On-surface synthesized magnetic nanoclusters of ferrocene derivatives
Abstract
On-surface molecular self-assembly in solution can produce two-dimensional (2D) materials with unique surface nanostructures that have the potential to create new functionalities. The surface completely differs from the uniform flat surface of conventional 2D materials such as graphene, MoS2, and 2D van der Waals nanosheets. The recently developed on-surface chemical synthesis of amino-ferrocene (AFc) nanoclusters on a graphene oxide (GO) nanosheet is a technique based on molecular self-assembly. Here, this method is applied to other ferrocene derivatives whose ferrocene units are covalently bonded to an amino group and several other molecules. The structure of the on-surface synthesized nanoclusters is analyzed by high-resolution transmission electron microscopy and atomic force microscopy. The molecules in the nanoclusters are densely and regularly arranged, and the distance between the Fe ions of the constituent molecules is longer than that in the AFc nanoclusters. Band-through electron transfer occurs between the Fe ions and the GO nanosheet, generating unpaired 3d electrons whose magnetic state is in the high spin state (S = 5/2). The present study demonstrates the feasibility of the design and synthesis of functional molecular nanostructures with molecular precision by on-surface chemistry, leading to the fabrication of nanoscale building blocks with molecular precision and 2D platforms for next-generation molecular spintronic and neuromorphic devices.
Keywords
INTRODUCTION
Low-dimensional magnetism involves fundamental issues related to the formation of magnetic order and new phases due to the reduction of its dimensionality[1,2] and has been studied both experimentally and theoretically for many years[3-6]. Recently, two-dimensional (2D) materials, such as 2D van der Waals magnets[6-8], have been associated with the elucidation of order formation in the 2D limit[9] and have been applied to topological quantum computation[10]; they have revealed new physical phenomena such as the quantum Hall effect[11] and the quantum spin liquid[12]. These 2D materials are typically prepared by mechanical exfoliation of bulk layered materials[13]. On the other hand, the use of on-surface chemistry such as charge transfer, surface diffusion, and on-surface synthesis to fabricate functional nanosheets in solution is a bottom-up approach and is based on molecules diffusing on the surface in a self-assembled manner[14]. This method can arrange molecules with molecular-scale precision and form nanoscale molecular architectures whose precision and arrangement are difficult to fabricate using the top-down approach. The recently developed method of creating 2D materials using graphene oxide (GO) on-surface chemistry[15] is an example of this technique. Using coupling agents, an amino group attached to a ferrocene molecule and a carboxyl group on a GO nanosheet form covalent bonds, and the molecule bonded to the nanosheet acts as a nucleus for crystal growth of diffusing molecules on the nanosheets, leading to the formation of 2D materials with nanoscale molecular aggregates[15,16].
A ferrocene molecule is known to be a structurally and chemically stable unit whose Fe ion is located between two cyclopentadienyl rings and is in the zero magnetic state (S = 0) due to the paired 3d electrons[17]. An amino-ferrocene (AFc) molecule is formed by adding an amino group to the unit and has the same magnetic state (S = 0) as the ferrocene molecule[16]. After the on-surface chemical reaction, the Fe ions in the AFc molecules in the nanoclusters have unpaired electrons by charge transfer between the molecules and GO[15], and the 3d electrons of the Fe ions are in a high spin state (S = 5/2)[16]. The magnetic dipole interaction between the Fe ions in the nanoclusters affects their magnetic properties due to the short intermolecular distance and large magnetic moment[16]. In the present study, we applied this on-surface synthesis method to molecules whose ferrocene units are attached to amino groups and other additional molecules to investigate the growth, structure, and magnetic state of molecular assemblies on GO nanosheets, and to see how changing the Fe-Fe distance and nanocluster size affects the band-through charge transfer and the formation of the magnetic state of the ferrocene derivatives.
EXPERIMENTAL
Synthesis of ferrocene derivative-based assemblies
The on-surface chemical reaction of AFc molecules with GO nanosheets has already been reported[15]. In this process, nanoclusters of AFc molecules are formed on a GO nanosheet, where the coupling agents
Characterization of the assemblies
The structure of the molecular assemblies on a GO nanosheet was investigated using a transmission electron microscope (TEM) (V-7200, JEOL) equipped with energy dispersive X-ray spectroscopy (EDX) and an atomic force microscope (AFM) (Dimension Icon, Bruker). First, 1-2 μL of diluted pure water solution containing the chemically synthesized nanosheets was dropped onto the TEM grid. After drying, the grid was placed on the TEM stage of the ultra-high vacuum (UHV) chamber for TEM observation [Figure 1A]. Additionally, 1-2 μL of dilute ethanol solution containing the nanosheets was dropped onto the SiO (90 nm)/Si substrate, and the dried substrate was used for AFM observation. The electronic states of the atoms forming the nanoclusters on the GO nanosheets were characterized by X-ray photoelectron spectroscopy (XPS) (Thermo Fisher Scientific Thera Probe system). The molecular components of the sheets were characterized by time-of-flight (TOF)-secondary ion mass spectrometry (SIMS) (PHI TRIFT V nanoTOF, Ulvac-Phi). A dense water solution containing the nanosheets was poured into a small hole in a clean copper thin plate. After drying, the aggregation of the sheets at the hole was measured by XPS and SIMS. The static magnetization and magnetic susceptibility under the application of a magnetic field were measured for aggregated nanosheets with a weight of 5-30 mg by using a superconducting quantum interference device (SQUID) magnetometer (MPMS-7T, Quantum Design).
Figure 1. (A) A TEM image of several C24H24FeNP-GO sheets (reaction time: 96 h) on a supporting carbon network of the TEM grid; (B) The amount of Fe atoms adsorbed on the GO nanosheets as a function of reaction time for chemical reaction of C24H24FeNP-GO sheets with (red open triangle) and without coupling agents (red open triangle) and AFc-GO sheets with coupling agents (blue open circle); (C) Inset: Molecular structures of the C24H24FeNP molecule. A TEM image of a GO nanosheet after chemical reaction of C24H24FeNP with coupling agents for 96 h; (D) Inset: Molecular structures of AFc molecule. A TEM image of a GO nanosheet after chemical reaction of AFc molecules with coupling agents for 24 h. Dark regions in (C and D) correspond to the nanoclusters of ferrocene derivatives; (E) Histogram of the diameter of C24H24FeNP-GO sheets (reaction time: 96 h). Red and blue solid lines show the Gaussian fit to the data for C24H24FeNP-GO and AFc-GO sheets; (F) Histogram of the number of nanoclusters in small areas of the C24H24FeNP-GO sheets using the quadra-count method; (G) TOF mass spectrum of C24H24FeNP-GO sheets. Inset: magnified spectrum at about 413 amu. TEM: Transmission electron microscope; GO: graphene oxide; AFc: amino-ferrocene; TOF: time-of-flight.
RESULTS AND DISCUSSION
The GO nanosheets chemically reacted with ferrocene derivatives on a supporting carbon network of a TEM grid was observed by a TEM [Figure 1A]. Each sheet in the image is 2-10 μm long, and many folded areas and wrinkles are created during the on-surface chemical reaction and the subsequent separation, washing, and drying processes. The progress of the chemical reaction with ferrocene derivatives was monitored by measuring the amount of Fe atoms in the solution. After removing the chemically synthesized nanosheets from the solution at each reaction time by centrifugation, the amount of Fe atoms in the residual solution was measured using inductively coupled plasma optical emission spectroscopy (ICP-OES)[15]. The amount of Fe atoms measured by this method corresponds to the amount of unreacted ferrocene derivatives in the solution. The difference of this amount of Fe atoms from that in the initial solution corresponds to rough estimation of the amount of Fe atoms adsorbed on the GO nanosheets and is plotted as a function of reaction time [Figure 1B]. There is a large difference in the change between the reactions of C24H24FeNP molecules (inset of Figure 1C), in which aminoethyl and diphenylphosphino groups are attached to the ferrocene unit, with and without coupling agents. The increase in the amount of Fe atoms in the reaction of C24H24FeNP molecules with coupling agents (red open triangle) indicates that the reaction progresses and forms molecular assemblies on the GO nanosheets, similar to the reaction with AFc molecules (inset of Figure 1D) using the agents (blue open circle)[15]. The negligible change in the reaction without the agents (red open circle) indicates negligible adsorption of the molecules on the GO nanosheets. Hereinafter, the GO nanosheets reacted with C24H24FeNP molecules and coupling agents will be abbreviated as C24H24FeNP-GO sheets, and the nanosheets reacted with AFc molecules (inset of Figure 1D) and coupling agents for 24 h will be abbreviated as AFc-GO sheets.
The structure of a C24H24FeNP-GO sheet (reaction time: 96 h) and an AFc-GO sheet (reaction time: 24 h) was investigated by a TEM [Figure 1C and D]. In their TEM images, there were several dark regions on the GO nanosheet. A TEM-EDX analysis of the C24H24FeNP-GO sheet showed that the Fe signal originated only from the dark regions [Supplementary Figure 1]. In the histogram of the lateral size of the regions measured from several TEM images of C24H24FeNP-GO sheets (reaction time: 96 h) [Figure 1E], the data are fitted by a Gaussian curve. The distribution in the C24H24FeNP-GO sheets (red curve) is broader than that in the AFc-GO sheets (blue curve). The distribution in the C24H24FeNP-GO sheets is further analyzed using the quadra-count method[18], where a large area TEM image of the C24H24FeNP-GO sheet is divided into small equal areas and a histogram is constructed by counting the number of the dark regions in each small area. The distribution is well fitted by a Poisson distribution (red solid line in Figure 1F), indicating that the regions are uniformly distributed on the nanosheet[19]. This is similar to that of the AFc assemblies on the GO nanosheets[15]. The TOF mass spectrum of the C24H24FeNP-GO sheets (reaction time: 96 h) had a peak at
To confirm the shape of the molecular assemblies, the height of the C24H24FeNP molecular assemblies on the GO nanosheets (reaction time: 96 h) was measured using an AFM. The white dots in the topological AFM image correspond to the assemblies [Figure 2A]. The cross-sectional line profile of the C24H24FeNP assembly marked with the yellow triangle in Figure 2A is shown in Figure 2B. The lateral width of the dots in the AFM image was much larger than that in the TEM images due to the tip effect[21], because the curvature of the AFM tip (100-500 nm) is much larger than that of the assemblies. The histogram of the diameter and height of C24H24FeNP assembly synthesized using the coupling agents for 46 h is shown in Supplementary Figure 2. The height of the C24H24FeNP assembly has a wider distribution than that of AFc assembly[15]
Figure 2. (A) A topological AFM image of C24H24FeNP nanoclusters on the GO nanosheet (reaction time: 96 h); (B) A cross-sectional line profile of the nanocluster marked with yellow triangle in (A); (C) Histogram of the heights of C24H24FeNP nanoclusters on the GO nanosheets (reaction time: 96 h). Red and blue solid lines show the Gaussian distribution fitted to the data for the C24H24FeNP-GO and AFc-GO sheets; (D) Schematic sequential illustration of the on-surface chemical reaction in which the molecule covalently bonded to the GO nanosheet acts as a nucleus for crystal growth. AFM: Atomic force microscope; GO: graphene oxide; AFc: amino-ferrocene.
The distance between the Fe ions of the ferrocene derivatives in the nanocluster on a GO nanosheet was investigated by taking high-resolution (HR)-TEM images [Figure 3A and B] and performing a fast Fourier transform (FFT) [Figure 3C and D]. The atom-resolved patterns in the TEM images show a regular arrangement of molecules in the nanocluster. The arrangement is completely different from that of the bulk AFc crystal[23], because the surface of the GO nanosheet plays an important role in the formation of a nanocluster. The Fe atoms in the ferrocene derivatives have larger structure factors with respect to the incident electron beams of the TEM than those of the C, O, H, N, and P atoms in the ferrocene derivatives, the GO nanosheet, and the thin carbon support layers of the TEM grid. Thus, the atom-resolved patterns in the HR-TEM images [Figure 3A and B] reflect the regular arrangement of Fe ions in the ferrocene derivatives forming a nanocluster. From the spacing of the main spots in the FFT patterns
Figure 3. (A) High-resolution TEM images and (B) corresponding FFT patterns of a C24H24FeNP nanocluster on a GO nanosheet; (C) High-resolution TEM images and (D) corresponding FFT patterns of an AFc nanocluster on a GO nanosheet; The main spots of the FFT patterns correspond to the lateral molecular arrangement of Fe ions in the molecules shown schematically in (E) the C24H24FeNP and (F) the AFc nanocluster; (G) The fundamental unit of the lateral arrangement is formed three-dimensionally by the corresponding Fe ions (orange and blue dots). TEM: Transmission electron microscope; FFT: fast Fourier transform; GO: graphene oxide; AFc: amino-ferrocene.
The investigation of the ionized state of Fe ions in the on-surface synthesized nanoclusters is instructive to study how the change in the Fe-Fe distance affects the band-through change transfer and the formation of the magnetic state. The electronic states of C and Fe atoms in the C24H24FeNP-GO sheets (reaction time:
Figure 4. (A and B) A series of XPS spectra of C1s and Fe2p core levels of the C24H24FeNP-GO sheets as a function of reaction time; (C) The Fe/C ratios obtained from the XPS spectra of GO nanosheets reacted with C24H24FeNP (red open circle) and AFc molecules (blue open circle) as a function of reaction time; (D) Magnetization curves of C24H24FeNP-GO sheets (reaction time: 96 h), GO sheets, and solid C24H24FeNP molecular powder at 2 K; (E)
The magnetic state of the Fe ions in the C24H24FeNP nanoclusters on a GO nanosheet was studied using the magnetic susceptibility χM,FD of the ferrocene derivative, which is simply expressed as MFD/H where magnetization MFD of the molecule is estimated using Equation (1) in Supplementary Materials. The
CONCLUSIONS
This study demonstrates the feasibility of self-assembled fabrication of functional nanoclusters using ferrocene derivatives in which an amino group and several other molecules are covalently bonded to the ferrocene unit. The present study shows that the molecule bonded by the covalent immobilization between the amino group of the C24H24FeNP molecule and the carboxyl group of the GO nanosheet under the coupling agents acts as a nucleus in the formation of on-surface synthesized nanoclusters with the regular molecular arrangement. A HR-TEM analysis shows that the Fe-Fe distance in the molecular nanoclusters can be tuned by using the ferrocene derivatives while maintaining their crystallinity. The present approach shows that band-through charge transfer occurs across several ferrocene derivatives to the GO nanosheet and that the 3d electrons of the Fe ions in the derivatives are in the high spin state (S = 5/2) for the nanoclusters whose size and Fe-Fe distance are increased by the on-surface chemical reaction of the ferrocene derivatives. The present results could provide guidance for the design and synthesis of attractive 2D materials using GO on-surface chemistry.
DECLARATIONS
Authors’ contributions
The author contributed solely to the article.
Availability of data and materials
Data supporting the results of the paper are available from the corresponding author upon reasonable request.
Financial support and sponsorship
This work was supported by JSPS KAKENHI Grant name JP21K04821 and in part by the International Center of Materials Nanoarchitectonics (WPI-MANA), MEXT, Japan.
Conflicts of interest
The author declared that there are no conflicts of interest.
Ethical approval and consent to participate
Not applicable.
Consent for publication
Not applicable.
Copyright
© The Author(s) 2024.
Supplementary Materials
REFERENCES
1. Mermin ND, Wagner H. Absence of ferromagnetism or antiferromagnetism in one- or two-dimensional isotropic Heisenberg models. Phys Rev Lett 1966:17;1133-6.
3. Bruno P. Magnetization and Curie temperature of ferromagnetic ultrathin films: the influence of magnetic anisotropy and dipolar interactions (invited). Proc MRS 1991:231;299-310.
5. Rong C, Li D, Nandwana V, et al. Size-dependent chemical and magnetic ordering in L10-FePt nanoparticles. Adv Mater 2006:18;2984-8.
6. Lee JU, Lee S, Ryoo JH, et al. Ising-type magnetic ordering in atomically thin FePS3. Nano Lett 2016:16;7433-8.
7. Gong C, Li L, Li Z, et al. Discovery of intrinsic ferromagnetism in two-dimensional van der Waals crystals. Nature 2017;546:265-9.
8. Huang B, Clark G, Navarro-Moratalla E, et al. Layer-dependent ferromagnetism in a van der Waals crystal down to the monolayer limit. Nature 2017:546;270-3.
9. Deng Y, Yu Y, Song Y, et al. Gate-tunable room-temperature ferromagnetism in two-dimensional Fe3GeTe2. Nature 2018:563;94-9.
10. Li J, Li Y, Du S, et al. Intrinsic magnetic topological insulators in van der Waals layered MnBi2Te4-family materials. Sci Adv 2019:5;eaaw5685.
11. Xue F, Hou Y, Wang Z, et al. Tunable quantum anomalous Hall effects in ferromagnetic van der Waals heterostructures. Natl Sci Rev 2023:11;nwad151.
13. Liu F. Mechanical exfoliation of large area 2D materials from vdW crystals. Prog Surf Sci 2021:96;100626.
14. Dreyer DR, Todd AD, Bielawski CW. Harnessing the chemistry of graphene oxide. Chem Soc Rev 2014;43;5288-301.
15. Sakurai M, Koley P, Aono M. Morphological change of molecular assemblies through on-surface chemical reaction. J Phys Chem C 2019:123;29679-85.
16. Sakurai M, Ueta T, Joachim C. Weekly interacting molecular spins in on-surface synthesized nanoclusters on a graphene oxide nanosheet. Adv Electron Mater 2023;9;2300347.
17. Crabtree RH. Pi-complexes. In: The organometallic chemistry of the transition metals. Wiley; 2014. pp. 134-62.
18. Ripley BD. 2 - Likelihood analysis for spatial Gaussian processes. In: Statistical inference for spatial process. Cambridge University Press; 1991. pp. 9-21.
20. Cervetti C, Rettori A, Pini MG, et al. The classical and quantum dynamics of molecular spins on graphene. Nat Mater 2016;15;164-8.
21. Keller DJ, Franke FS. Envelope reconstruction of probe microscope images. Sur Sci 1993:294;409-19.
22. Zangwill A. 15 - Surface reactions. In: Physics at surfaces. Cambridge univ. Press; 1988. pp. 400-20.
23. Perrine CL, Zeller M, Woolcock J, Hunter AD. Crystal structure of ferrocenyl amine. J Chem Crystal 2005:35;717-21.
25. Ren PG, Yan DX, Ji X, Chen T, Li ZM. Temperature dependence of graphene oxide reduced by hydrazine hydrate. Nanotechnology 2011;22:055705.
26. Perrozzi F, Prezioso S, Ottaviano L. Graphene oxide: from fundamentals to applications. J Phys Cond Matter 2015;27:013002.
27. Lüth H. Electronic surface states. In: Surfaces and interfaces of solid materials. Berlin: Springer. 1995. pp. 254-315.
28. Yamashita T, Hayes P. Analysis of XPS spectra of Fe2+ and Fe3+ ions in oxide materials. Appl Surf Sci 2008;254;2441-9.
29. Kahn O. Chapter 2 - Molecules containing a unique magnetic center without first-order orbital momentum. In: Molecular magnetism. VCH; 1992. Available from: https://www.scribd.com/document/382790790/Kahn-Molecular-Magnetism. [Last accessed on 12 Jul 2024].
30. Morrish AH. Chapter 3 - Thermal, relaxation, and resonance phenomena in paramagnetic materials. In: The physical principles of magnetism. Wiley; 1965. pp. 78-148. Available from: https://onlinelibrary.wiley.com/doi/10.1002/9780470546581.ch3. [Last accessed on 12 Jul 2024]
31. Miller JS, Epstein AJ, Reiff WM. Ferromagnetic molecular charge-transfer complexes. Chem Rev 1988:88;201-20.
Cite This Article
How to Cite
Sakurai, M. On-surface synthesized magnetic nanoclusters of ferrocene derivatives. Chem. Synth. 2024, 4, 42. http://dx.doi.org/10.20517/cs.2023.76
Download Citation
Export Citation File:
Type of Import
Tips on Downloading Citation
Citation Manager File Format
Type of Import
Direct Import: When the Direct Import option is selected (the default state), a dialogue box will give you the option to Save or Open the downloaded citation data. Choosing Open will either launch your citation manager or give you a choice of applications with which to use the metadata. The Save option saves the file locally for later use.
Indirect Import: When the Indirect Import option is selected, the metadata is displayed and may be copied and pasted as needed.
About This Article
Special Issue
Copyright
Author Biographies
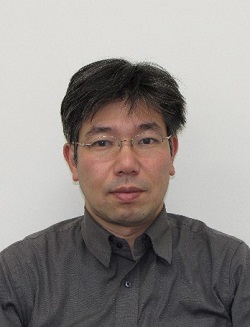
Data & Comments
Data
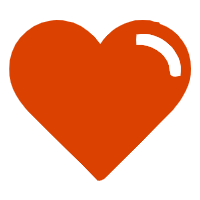
Comments
Comments must be written in English. Spam, offensive content, impersonation, and private information will not be permitted. If any comment is reported and identified as inappropriate content by OAE staff, the comment will be removed without notice. If you have any queries or need any help, please contact us at support@oaepublish.com.