Chemosensitizing effect of pentoxifylline in sensitive and multidrug-resistant non-small cell lung cancer cells
Abstract
Aim: Multidrug resistance (MDR) is frequent in non-small cell lung cancer (NSCLC) patients, which can be due to its fibrotic stroma. This work explores the combination of pentoxifylline, an anti-fibrotic and chitinase 3-like-1 (CHI3L1) inhibitor drug, with conventional chemotherapy to improve NSCLC treatment.
Methods: The effect of pentoxifylline in the expression levels of P-glycoprotein (P-gp), CHI3L1 and its main downstream proteins, as well as on cell death, cell cycle profile, and P-gp activity was studied in two pairs of sensitive and MDR counterpart NSCLC cell lines (NCI-H460/NCI-H460/R and A549/A549-CDR2). Association studies between CHI3L1 gene expression and NSCLC patients’ survival were performed using The Cancer Genome Atlas (TCGA) analysis. The sensitizing effect of pentoxifylline to different drug regimens was evaluated in both sensitive and MDR NSCLC cell lines. The cytotoxicity of the drug combinations was assessed in MCF10A non-tumorigenic cells.
Results: Pentoxifylline slightly decreased the expression levels of CHI3L1, β-catenin and signal transducer and activator of transcription 3 (STAT3), and caused a significant increase in the G1 phase of the cell cycle in both pairs of NSCLC cell lines. A significant increase in the % of cell death was observed in the sensitive NCI-H460 cell line. TCGA analysis revealed that high levels of CHI3L1 are associated with low overall survival (OS) in NSCLC patients treated with vinorelbine. Moreover, pentoxifylline sensitized both pairs of sensitive and MDR NSCLC cell lines to the different drug regimens, without causing significant toxicity to non-tumorigenic cells.
Conclusion: This study suggests the possibility of combining pentoxifylline with chemotherapy to increase NSCLC therapeutic response, even in cases of MDR.
Keywords
INTRODUCTION
Despite recent advances in the treatment of non-small cell lung cancer (NSCLC), platinum-based chemotherapy, consisting of cisplatin or carboplatin in combination with paclitaxel, gemcitabine or vinorelbine, is still commonly used[1-5]. Nevertheless, multidrug resistance (MDR) to chemotherapy is frequently observed in patients with NSCLC[6,7]. A high expression of efflux pumps, particularly
Our recent work discovered that pentoxifylline, an anti-fibrotic drug approved for the treatment of vascular diseases[15,16], increased the response of pancreatic cancer cells to gemcitabine treatment[17]. The chemosensitizing effect of pentoxifylline has also been described in cervical[18,19], prostate[20], and human pancreatic tumor xenografts[21]. In NSCLC cells, Ohsaki et al. reported the benefit of combining pentoxifylline with cisplatin and etoposide by interfering with the cell cycle[22]. Thus, pentoxifylline, in combination therapies, may be a promising drug candidate for drug repurposing to improve NSCLC treatment. Mechanistically, pentoxifylline showed anticancer activity by reducing tumorigenesis and cell proliferation via modulation of the mitogen-activated protein kinase/extracellular signal-regulated kinase (MAPK/ERK) and protein kinase B (Akt) pathways[23], and inducing apoptosis and cell cycle arrest in breast[24], liver[25], and colorectal[26] cancer cells.
Interestingly, pentoxifylline is also known as a chitinase 3-like-1 (CHI3L1) inhibitor[17,27]. Notably, CHI3L1 is a promising therapeutic target in cancer. For instance, CHI3L1 has been implicated in reducing the response of pancreatic cancer cells to gemcitabine[17]. Several studies have also reported that high serum levels of CHI3L1 are associated with low overall survival (OS) rates in patients with different types of cancer[28-30]. In NSCLC, Jefri et al. demonstrated that CHI3L1 mediates the expression of numerous epithelial-to-mesenchymal transition factors, providing an invasive phenotype to cancer cells[31]. CHI3L1 is associated with tissue remodeling through stimulation of fibroblast growth and matrix deposition, thus contributing to establishing a highly fibrotic stroma in NSCLC[32,33]. CHI3L1 is also known to be secreted by tumor and stromal cells, such as macrophages and fibroblasts[33]. Most importantly, CHI3L activates several pro-survival signaling pathways, and regulates cell growth, differentiation, and apoptosis in NSCLC. For instance, CHI3L1 interacts with IL-13Rα2 and/or the receptor for advanced glycation end products (RAGE), which are transmembrane receptors, activating ERK, Akt, and signal transducer and activator of transcription 3 (STAT3) signaling pathways, and inducing
Therefore, this work intends to disclose the mechanism of action of pentoxifylline and to explore the chemosensitizing effects of pentoxifylline when combined with conventional chemotherapeutic drugs currently used to treat NSCLC (paclitaxel, vinorelbine, carboplatin, and vinorelbine plus carboplatin), in pairs of sensitive and MDR NSCLC cell lines.
METHODS
Cell lines and culture
Two pairs of counterpart NSCLC cell lines, NCI-H460 (sensitive) and NCI-H460/R (MDR) and A549 (sensitive) and A549-CDR2 (MDR), were used in this work. The human NSCLC large cell carcinoma NCI-H460 and NCI-H460/R cell lines were kindly provided by Dr. Milica Pešić, Belgrade, Serbia[37,38]. The NCI-H460/R cell line is resistant to several drugs, including doxorubicin, paclitaxel, etoposide, vinblastine, and epirubicin[37]. Both cell lines were cultured in RPMI-1640 medium supplemented with Ultraglutamine I and 25 mM 4-(2-hydroxyethyl)-1-piperazineethanesulfonic acid (HEPES) and 10% fetal bovine serum (FBS; Biowest). The sensitive human NSCLC adenocarcinoma cell line, A549, was obtained from American Type Culture Collection (ATCC), and its MDR counterpart cell line, A549-CDR2, was established by our research group by continuous treatment with increased concentrations of paclitaxel. This cell line is resistant to paclitaxel, vinorelbine, and doxorubicin [Supplementary Figure 1] as well as to docetaxel, etoposide, and gemcitabine (unpublished data). The A549 and A549-CDR2 cells were cultured in DMEM with 4.5 g/L Glucose (Lonza; BE12-604F) and 10% FBS. To maintain the resistant phenotype of the MDR cell lines, doxorubicin and paclitaxel were added every three weeks to NCI-H460/R and A549-CDR2, respectively. For each assay, doxorubicin was added six days and paclitaxel was added three days before starting each experiment to NCI-H460/R and A549-CDR2 cells, respectively. During the performance of the Sulforhodamine B (SRB) assay, all cell lines were supplemented with 5% FBS. The non-tumorigenic breast cell line, MCF-10A (from ATCC), was cultured in DMEM/F12 Medium (Thermo Fischer Scientific), as previously described[39]. All cell lines were kept in a humidified incubator at 37 °C with 5% CO2.
Reagents
Carboplatin (BP711), doxorubicin (D1515), paclitaxel (T7402), pentoxifylline (P1784), verapamil (v-4629), and vinorelbine (Y0000463) were obtained from Merck Life Science. Dimethyl-sulfoxide (DMSO) was used to dissolve paclitaxel and doxorubicin, whereas sterile water was used to dissolve carboplatin, pentoxifylline, and vinorelbine. Carboplatin was stored at 4 °C while the other drugs stored at -20 °C.
Drug treatments
The NSCLC cell lines were treated with different concentrations of pentoxifylline (4, 2, 1 and 0.5 mM), H2O (the vehicle, control), or culture medium (blank). To study the cytotoxic effect of the combined therapies in both sensitive and MDR pairs of cell lines, as well as in the MCF-10A cell line, cells were treated with: (1) each drug individually; (2) drug combinations consisting of pentoxifylline together with paclitaxel, vinorelbine, carboplatin, or vinorelbine plus carboplatin; (3) H2O and/or DMSO (vehicles, control).
Cell growth inhibition - SRB assay
SRB assay was performed according to Vichai and Kirtikara[40] and as previously described[17,41]. The cells (at 5.0 × 104 cells/mL) were plated in 96 wells and, after 24 h, treated with different drugs. After 48 h, cells were fixed with 50 µL ice-cold 10% (w/v) trichloroacetic acid, washed with distilled water, and then stained with 0.4% (w/v) SRB (Merck Life Science) in 1% (v/v) acetic acid for 30 min in the dark, and at room temperature. Cells were washed with 1% (v/v) acetic acid and then 10 mM Tris-Base was added for 5 min and absorbance measured (Synergy TM BioTek Instruments, Inc.) using Gen5TM software. Drug concentration-response curves were determined and the GI50 concentration (which causes 50% of cell growth inhibition) was calculated.
Protein expression - Western Blot analysis
Cells were plated in 6-well plates (at 5.0 × 104 cells/mL) for 24 h and then treated with pentoxifylline at
Cell cycle profile analysis
The effect of pentoxifylline on the cell cycle profile was analyzed using propidium iodide (PI) staining, according to the previously described protocol[41-43]. After 24 h of cell seeding (5.0 × 104 cells/mL), cells were exposed for 48 h to the following conditions: (1) culture medium (blank); (2) medium with H2O (vehicle); (3) pentoxifylline at 1 or 2 mM. After this period, cells were collected, centrifuged and the pellets fixed with cold ethanol. After at least 12 h at 4 °C, cells were centrifuged and resuspended in phosphate buffered saline (PBS) with 5 g/mL PI (Merck Life Science) and 0.1 mg/mL RNase (Invitrogen), for 30 min at 4 °C, protected from the light. All samples were analyzed by flow cytometry (BD AccuriTM C6 Plus Flow Cytometer, BD Biosciences). A minimum of 15,000 events per sample were plotted after the exclusion of cell debris and aggregates. Data were analyzed using the FlowJo 10.8. Software.
Cell death analysis
The effect of pentoxifylline on cell death was evaluated using the Annexin V-FITC Apoptosis Detection Kit (Invitrogen), as previously described[41,42]. After 24 h of seeding (at 5.0 × 104 cells/mL), cells were treated with: (1) culture medium (blank); (2) medium with H2O (vehicle); or (3) pentoxifylline at 1 and 2 mM. Following 48 h of treatment, and 1 h before collecting the cells, ethanol was added to the blank condition (positive control). Cells were centrifuged and the pellet resuspended in Binding Buffer. Cell suspensions were divided into two samples: (a) auto-fluorescence; and (b) labeled samples, which were incubated with the Annexin V-FITC conjugate, followed by incubation with PI on ice. Samples were analyzed by flow cytometry (at least 15,000 events per sample were plotted, excluding cell debris and aggregates) and the percentage of dead cells was determined using the FlowJo program.
Rhodamine-123 accumulation assay
The P-gp inhibitory activity of pentoxifylline was assessed with the Rhodamine-123 accumulation assay[43,44]. Cells were cultured for 24 h in 12-well plates (at 5.0 × 104 cells/mL) and then treated with: (1) vehicle (H2O) or pentoxifylline at 1, 2, 5, or 10 mM during 1 h; (2) vehicle or pentoxifylline at 1 or 2 mM during 48 h. The sensitive cell line NCI-H460 was used as a negative control. The MDR NCI-H460/R cells under 20 µM Verapamil treatment (P-gp inhibitor) were used as a positive control. Cells were treated with or without rhodamine-123 (Merck Life Science) at 1 µM. In the end, cells were collected, centrifuged, and resuspended in cold PBS. A minimum of 15,000 events per sample were acquired (excluding cell debris and aggregates) using the Flow Cytometer.
Association studies between CHI3L1 gene expression and OS and drug response
Bioinformatic studies using The Cancer Genome Atlas (TCGA) database (https://www.cancer.gov/tcga) were performed to assess the possible association between CHI3L1 gene expression and OS of 994 patients with NSCLC. Moreover, possible associations between CHI3L1 gene expression and OS of NSCLC patients under different drug treatments (carboplatin, vinorelbine and carboplatin plus vinorelbine) were analyzed.
Determination of the fold change of the combined therapies vs. conventional chemotherapies
The fold change was determined for each drug treatment and in each pair of sensitive and MDR cell lines (NCI-H460 vs. NCI-H460/R and A549 vs. A549-CDR2), at the concentrations used for the drug combinations and using the following formula: % of Cell growth inhibition of the combined treatment of a chemotherapeutic drug with pentoxifylline/% of Cell growth inhibition of the conventional chemotherapeutic drug.
Statistical analysis
The two-tailed unpaired t-test was applied using the GraphPad Prism 8.0 software. Results represent the mean ± standard error of the mean (SEM), and a minimum of three independent experiments were performed. Statistical significance was considered whenever P ≤ 0.05. Non-statistical significance (ns) whenever P > 0.05.
RESULTS
Pentoxifylline decreased the growth of sensitive and MDR counterpart NSCLC cell lines
The effect of pentoxifylline (48 h treatment) on NSCLC cell growth was assessed using the SRB assay, and the GI50 concentration calculated based on the determination of the drug concentration-response curves
GI50 concentrations of pentoxifylline in two pairs of sensitive and MDR counterpart NSCLC cell lines
Drug | GI50* (mM) | |||
NCI-H460 | NCI-H460/R | A549 | A549-CDR2 | |
Pentoxifylline | 0.96 ± 0.08 | 1.17 ± 0.17 | 1.23 ± 0.07 | 1.53 ± 0.04 |
Pentoxifylline decreased the expression levels of P-gp, CHI3L1 and STAT3 in NSCLC cell lines
To unravel the mechanism of action of pentoxifylline, the effect of this drug (at 1 mM for 48 h) on the expression levels of P-gp (MDR-related protein), CHI3L1 (known target of pentoxifylline) and its main downstream proteins, such as β-catenin, phospho(p)-Akt, phospho(p)-ERK, and phospho(p)-STAT3 was studied. As expected, the results obtained [Figure 1] confirmed the higher expression levels of P-gp in the NCI-H460/R cell line, compared to NCI-H460 cells[45,46]. The same trend was found in the A549/A549-CDR cell lines. Pentoxifylline slightly decreased P-gp expression, although not significantly, in both resistant cell lines.
Figure 1. Effect of pentoxifylline on the expression levels of P-gp, determined by Western Blot, on (A) NCI-H460 vs. NCI-H460/R and (B) A549 vs. A549-CDR2 cell lines, treated with vehicle (H2O) or pentoxifylline at 1.0 mM after 48 h. β-Actin was used as a loading control. Data are presented as a percentage of expression.
Results also showed that pentoxifylline significantly decreased CHI3L1 expression in the NCI-H460/R cells
Figure 2. Effect of 48-h treatment with 1 mM pentoxifylline on the expression levels of CHI3L1 and its downstream proteins (β-Catenin, p-Akt and p-ERK), determined by Western Blot on (A-D) NCI-H460 vs. NCI-H460/R and on (E-H) A549 vs. A549-CDR2 cell lines. The effect of the vehicle (H2O) was also analyzed. β-Actin was used as a loading control. Data are presented as a percentage of expression. *P ≤ 0.05. CHI3L1: Chitinase 3-like-1; ERK: extracellular signal-regulated kinase.
Moreover, pentoxifylline significantly decreased the total STAT3 expression levels in the resistant NCI-H460/R cell line. In the A549-CDR2 cell line, pentoxifylline decreased STAT3 phosphorylation (p-STAT3) levels, although not significantly [Figure 3].
Figure 3. Effect of 48-h treatment with 1 mM pentoxifylline on the expression levels of total and phosphorylated STAT3, determined by Western Blot on (A) NCI-H460 vs. NCI-H460/R and (B) A549 vs. A549-CDR2 cell lines. The effect of the vehicle (H2O) was also analyzed. β-Actin was used as a loading control. Data are presented as a percentage of expression. *P ≤ 0.05. STAT3: signal transducer and activator of transcription 3.
Pentoxifylline caused an increase in the percentage of cells in the G0/G1 phase of the cell cycle
The effect of pentoxifylline on the cell cycle profile was assessed. As shown in Figure 4, pentoxifylline significantly increased the percentage of cells in the G0/G1 phase of the cell cycle in NCI-H460 and NCI-H460/R cell lines. In the NCI-H460/R cell line, a significant decrease in the percentage of cells in the S and G2/M phases was also observed. Consistently, pentoxifylline, at the higher concentration tested, significantly increased the percentage of cells in the G0/G1 phase in A549 and A549-CDR2 cell lines. Moreover, pentoxifylline slightly increased the percentage of cells in the sub-G1 phase (indicative of apoptosis) in all the cell lines.
Figure 4. Effect of 1 or 2 mM pentoxifylline 48 h treatment on the cell cycle profile of (A) NCI-H460 vs. (B) NCI-H460/R and (C) A549 vs. (D) A549-CDR2 cell lines, analyzed by flow cytometry. The frequency of the cell cycle phases (top panels), as well as the representative cell cycle histograms (bottom panels), are presented. *P ≤ 0.05; **P < 0.01.
Pentoxifylline increased cell death of the sensitive NCI-H460 NSCLC cell line
The effect of pentoxifylline on cell death was evaluated. Results demonstrated that pentoxifylline significantly increased the percentage of cell death in the NCI-H460 cells in a concentration-dependent manner [Figure 5]. This effect, however, was not observed in the resistant NCI-H460/R cells.
Figure 5. Effect of 48 h treatment with 1 or 2 mM pentoxifylline on the percentage of cell death of (A) sensitive NCI-H460 and (B) MDR NCI-H460/R NSCLC cells, determined by flow cytometry. The effect of the vehicle at the highest concentration was also tested. The frequency of dead cells for each condition (top panels) and the representative dot plot histograms (bottom panels) are shown. *P ≤ 0.05. MDR: Multidrug resistance; NSCLC: non-small cell lung cancer.
Pentoxifylline did not affect P-gp activity
Previous data revealed that pentoxifylline reduced the expression levels of the drug efflux pump P-gp in both MDR NSCLC cell lines. As shown in Figure 6, pentoxifylline did not cause alteration in P-gp activity following 1 or 48 h drug treatment, as there was no increase in the amount of intracellular rhodamine-123 accumulated on NCI-H460/R cells under drug treatment.
Figure 6. Effect of (A) 1-h and (B) 48-h treatments with different concentrations of pentoxifylline on P-gp activity of the NCI-H460/R cells, determined by the Rhodamine-123 accumulation assay. NCI-H460 cells were used as a negative control. The positive control consisted of NCI-H460/R cells treated with 20 μM verapamil. **P < 0.01; ***P < 0.001; ****P < 0.0001. P-gp: P-glycoprotein.
CHI3L1 gene expression was associated with the low response of NSCLC patients to vinorelbine treatment
The association of CHI3L1 gene expression with the OS of 994 patients with NSCLC, and the response of those patients to different chemotherapeutic regimens was studied using the TCGA database. Figure 7 demonstrates that high expression of CHI3L1 is not associated with poor OS of NSCLC patients. Importantly, NSCLC patients under vinorelbine treatment, and with high expression levels of CHI3L1, do not benefit from this therapy. The same tendency is observed when NSCLC patients are under carboplatin treatment, although in this case, the difference is not statistically significant. In NSCLC patients treated with carboplatin plus vinorelbine, the expression of CHI3L1 does not affect the response to the drug treatment.
Figure 7. OS and CHI3L1 gene expression in 994 NSCLC cases, using Kaplan-Meier curves. Comparison between low and high expression of CHI3L1 in (A) NSCLC patients and (B) under vinorelbine, (C) carboplatin, or (D) vinorelbine plus carboplatin treatments. OS: Overall survival; CHI3L1: chitinase 3-like-1; NSCLC: non-small cell lung cancer.
Pentoxifylline sensitized all NSCLC cell lines to different drug regimens
The combination treatment consisting of pentoxifylline with different drug regimens, namely paclitaxel, vinorelbine, carboplatin, and vinorelbine plus carboplatin, was evaluated in sensitive and MDR NSCLC cell lines using the SRB assay. The results [Figure 8] showed that pentoxifylline in combination with paclitaxel significantly decreased the percentage of cell growth in the sensitive NCI-H460 cell line compared with pentoxifylline or paclitaxel alone, and in the resistant NCI-H460/R cell line but only compared to paclitaxel alone. Regarding the combined treatment consisting of pentoxifylline with carboplatin, a significant decrease in the percentage of cell growth was observed in both sensitive cell lines and in the resistant NCI-H460/R cell line, compared to treatment with pentoxifylline or carboplatin alone. When pentoxifylline was combined with vinorelbine, a slight reduction in the percentage of NCI-H460 cell growth, and a significant effect on the percentage of NCI-H460/R cell growth, were observed compared with vinorelbine alone. Finally, both NCI-H460 and NCI-H460/R cell lines responded significantly better to treatment consisting of pentoxifylline in combination with vinorelbine plus carboplatin, compared with these drugs individually.
Figure 8. Effect of pentoxifylline in combination with different anticancer drugs on the percentage of cell growth of NCI-H460 and NCI-H460/R cell lines, assessed by the SRB assay. Cells were treated for 48 h with pentoxifylline at 1.0 mM and (A) paclitaxel at 5.7 nM, (B) carboplatin at 17.7 µM, (C) vinorelbine at 35 nM, and (D) vinorelbine at 35 nM plus carboplatin at 17.7 μM. The effect of the vehicle at the highest concentration was also tested. Data are presented as a percentage of cell growth. *,#P ≤ 0.05; **,##P < 0.01; ***,###P < 0.001; ****,####P < 0.0001; ns: P > 0.05. SRB: Sulforhodamine B.
Further, the chemosensitizing effect of pentoxifylline was validated in another pair of sensitive/MDR NSCLC cell lines, A549/A549-CDR2. The results [Figure 9] demonstrated that pentoxifylline combined with paclitaxel significantly decreased the percentage of cell growth in both cell lines, compared with paclitaxel alone. However, compared with pentoxifylline alone, the effect was only significant in the sensitive cell line. Moreover, pentoxifylline combined with carboplatin significantly decreased the percentage of cell growth in A549 cells, compared with carboplatin alone or with pentoxifylline alone. In A549-CDR2 cells, the effect was only significant compared with pentoxifylline alone. Additionally, pentoxifylline, in combination with vinorelbine, significantly decreased the percentage of cell growth in both A549 and A549-CDR2 cells. Finally, the inhibition of cell growth exhibited a significantly greater magnitude when pentoxifylline was combined with vinorelbine plus carboplatin, in both sensitive and MDR cells, as opposed to when vinorelbine plus carboplatin was used. However, in the sensitive cells, the effect of the triple drug combination was not significantly different from the effect of treatment with vinorelbine alone.
Figure 9. Effect of pentoxifylline in combination with different anticancer drugs on the percentage of cell growth of A549 and A549-CDR2 cell lines, assessed by the SRB assay. Cells were treated for 48 h with pentoxifylline at 1.0 mM and (A) paclitaxel at 2.7 nM, (B) carboplatin at 83.7 μM, (C) vinorelbine at 25 nM, or (D) vinorelbine at 25 nM plus carboplatin at 83.7 μM. Data are presented as a percentage of cell growth. *,#P ≤ 0.05; **,##P < 0.01; ***,###P < 0.001; ****,####P < 0.0001; ns: P > 0.05.
Importantly, the fold change of the percentage of cell growth inhibition of the different combination therapies (conventional chemotherapy with pentoxifylline) vs. conventional chemotherapy, for both pairs of NSCLC cell lines, was calculated [Table 2]. Values above 1 mean that the combination of a particular chemotherapeutic drug with pentoxifylline was more effective in decreasing cell growth than the chemotherapeutic drug alone. Thus, higher fold changes suggest more potent combination treatments. Table 2 shows that pentoxifylline combined with the different chemotherapeutic drugs decreased the percentage of cell growth more efficiently than the chemotherapeutic drugs alone. Interestingly, in the NCI-H460/R cell line, pentoxifylline in combination with vinorelbine and with vinorelbine plus carboplatin have the highest fold changes (values above 2.0).
Fold change of the percentage of growth inhibition of the NSCLC cell lines following treatment with chemotherapeutic drugs combined with pentoxifylline vs. without pentoxifylline
NSCLC cell lines | Combination treatment consisting of pentoxifylline with | |||
Paclitaxel | Vinorelbine | Carboplatin | Vinorelbine plus carboplatin | |
NCI-H460 | 2.14 | 1.33 | 1.86 | 1.78 |
NCI-H460/R | 1.64 | 2.36 | 1.74 | 2.42 |
A549 | 1.5 | 1.25 | 1.41 | 1.62 |
A549-CDR2 | 1.23 | 1.53 | 1.19 | 1.43 |
The cytotoxic effect of pentoxifylline combined with conventional chemotherapeutic drugs (paclitaxel, vinorelbine, carboplatin, and vinorelbine plus carboplatin) was also evaluated in the MCF-10A cells using the SRB assay. Results [Supplementary Figure 4] demonstrated that, at least at the concentrations tested, none of the combination treatments caused a significant increase in cytotoxicity, compared to the conventional therapy alone.
DISCUSSION
MDR is commonly observed in patients with NSCLC treated with different drug regimens currently applied in the clinical practice[7], and one of the causes has been associated with the presence of a highly resistant fibrotic stroma[12]. Thus, combining an anti-fibrotic drug with conventional chemotherapeutic drugs may improve therapeutic response of NSCLC cells.
Recently, our research group demonstrated that pentoxifylline, an approved drug for the treatment of vascular diseases with anti-fibrotic properties, sensitized pancreatic cancer cells to gemcitabine treatment[17]. Importantly, pentoxifylline reversed drug resistance induced by CHI3L1 in pancreatic cancer cells[17]. In fact, pentoxifylline is described as a CHI3L1 inhibitor, and overexpression of CHI3L1 has been associated with poor survival in different types of cancer[47]. Therefore, this work aimed to evaluate the effect of pentoxifylline on sensitive and MDR NSCLC cells and disclose its mechanism of action, as well as to verify the sensitizing effect of pentoxifylline to different chemotherapeutic regimens.
This work revealed that pentoxifylline reduced the growth of two pairs of sensitive and MDR NSCLC cell lines at concentrations below 2 mM. The mechanism of action of pentoxifylline was then explored in NSCLC cells. Pentoxifylline reduced the expression levels of P-gp but did not affect its activity. Barancik
Additionally, this work demonstrated that pentoxifylline decreased STAT3 expression levels in one of the MDR cell lines, which corroborates previous studies demonstrating that pentoxifylline impairs cancer cells’ ability to migrate through the downregulation of STAT3 signaling in B16-F10 murine melanoma[51] and in A375 human melanoma cells[52]. Interestingly, STAT3 is also a downstream signaling protein to CHI3L1[53]. Although the exact mechanism of STAT3 activation by CHI3L1 is not fully understood, one possibility is that CHI3L1 activates STAT3 through receptor interaction[36]. In fact, Lee et al. reported that CHI3L1 knockdown decreased STAT3 activity, which in turn decreased cancer cell growth in lung cancer[54]. Moreover, there are some studies that demonstrate a positive feedback loop between CHI3L1 and STAT3, since STAT3 also mediates the transcription of the CHI3L1 gene[55,56]. Interestingly, the results presented in the present study demonstrated that pentoxifylline significantly decreased both CHI3L1 and STAT3 expression levels in the NCI-H460/R cell line. In the other NSCLC cell lines, a slight decrease in CHI3L1 and STAT3 expression levels was also observed. Altogether, our results suggest that pentoxifylline might also exert its effect on NSCLC through inhibition of the CHI3L1/STAT3 signaling.
Pentoxifylline was able to interfere with the cell cycle profile of both sensitive and MDR NSCLC cells by increasing the percentage of cells in the G0/G1 phase. Moreover, this drug caused cell death in the sensitive NCI-H460 cells. Other studies have also reported the effect of pentoxifylline on the cell cycle profile and cell death in other cancer types. For instance, Castellanos-Esparza et al.[57] and Wang et al.[25] demonstrated that pentoxifylline caused cell cycle arrest in G0/G1 phase and increased cell death, in a triple-negative breast cancer cell line and in hepatocellular carcinoma cells, respectively. In the present work, pentoxifylline caused apoptosis in the NCI-H460 cells but not in the resistant counterpart cells. The explanation for this difference is unknown but may be due to the presence of gene mutations responsible for escape from apoptosis in the MDR cell line[38].
The TCGA data analysis revealed that high expression of CHI3L1 gene is not associated with low OS of NSCLC patients, even though several studies have reported that high serum levels of CHI3L1 are associated with low OS in patients with several types of cancers[33,34,47,58]. Notably, by analyzing this database, NSCLC patients with high expression levels of CHI3L1 and treated with vinorelbine have a worse OS than NSCLC patients with low CHI3L1 expression levels. These findings propose that high CHI3L1 gene expression is associated with lower response to vinorelbine in NSCLC patients. Thus, combining conventional chemotherapy with CHI3L1 inhibitors may improve the therapeutic outcome of NSCLC patients. In line with this data, Chiang et al.[59] and Xavier et al.[17] demonstrated that CHI3L1 overexpression negatively affects the response of epithelial ovarian carcinoma cells to paclitaxel and pancreatic cancer cells to gemcitabine treatment, respectively.
Interestingly, the data presented in this study revealed that pentoxifylline sensitized NCI-H460 cells to paclitaxel treatment, and both NCI-H460 and NCI-H460/R cells to carboplatin and to vinorelbine plus carboplatin treatments. Moreover, pentoxifylline sensitized the A549 cell line to paclitaxel, vinorelbine, and carboplatin treatments, and sensitized the A549-CDR2 cell line to vinorelbine and to vinorelbine plus carboplatin treatments. Thus, the combination of pentoxifylline with some chemotherapeutic drugs increased the inhibition of cell growth, compared to the individual treatments, without presenting increased cytotoxicity to non-tumorigenic cells. Interestingly, Ohsaki et al. also demonstrated the benefit of combining pentoxifylline with conventional chemotherapy, namely cisplatin and etoposide, in other NSCLC cell lines, PC-9 and PC-14[22].
Altogether, this work demonstrated the advantage of combining pentoxifylline with different conventional drug regimens in both sensitive and MDR NSCLC cell lines. Nevertheless, we are aware that this study has some limitations. For instance, different mechanisms of action of pentoxifylline were detected in the different NSCLC cell lines studied, particularly regarding the effect of pentoxifylline on cell death and on the expression levels of CHI3L1 and its downstream proteins. These may be due to the different histological features of both counterpart pairs of NSCLC cell lines tested, together with differences between sensitive and resistant phenotypes. In the future, it would be interesting to further understand the effect of pentoxifylline in cells with different genetic backgrounds. Another point to consider is that A549 and NCI-H460 cell lines have the Nfr2-Keap1 antioxidant response pathway activated[60]. Several studies demonstrated that overactivation of this pathway supports cancer progression and protects cancer cells from oxidative damage, leading to chemoresistance[61,62]. In fact, it is known that ERK and Akt signaling pathways upregulate Nrf2 and thus increase the Nrf2-Keap1 signaling[62]. Since ERK and Akt pathways are downstream of CHI3L1, therapies targeting CHI3L1 may be beneficial in downregulating the Nrf2-Keap1 pathway. Although this work was not able to observe a significant decrease in phospho-Akt and phospho-ERK levels following pentoxifylline treatment, other studies reported that CHI3L1 inhibition led to decreased activity in these pathways[34], and thus may have an impact on the Nfr2-Keap1 pathway. On the other hand, a recent publication reports that pentoxifylline can increase Nrf2 in non-cancer cells[63]. However, in the context of tumor cells, the effect of pentoxifylline on the Nfr2-Keap1 pathway is not yet known and therefore more studies are needed. Finally, another important issue is that the effect of the drug combinations should be exploited in more advanced cell models, better resembling tumors and their microenvironment, such as in 3D advanced cell-based models (spheroids and/or patient-derived tumoroids) co-cultured with cells from the tumor microenvironment (TME). This is particularly important since CHI3L1 has been found overexpressed both in tumor cells and in cells from the TME, namely tumor-associated macrophages and cancer-associated fibroblasts. This would enable us to verify whether pentoxifylline can also counteract the effect of CHI3L1 released by these TME cells.
In conclusion, this study provides insights into the possibility of combining pentoxifylline with different chemotherapeutic drug regimens to improve the therapeutic response of NSCLC patients, even in cases of MDR. Moreover, the presented data reinforce the potential of CHI3L1 as a promising therapeutic target in NSCLC.
DECLARATIONS
Acknowledgments
The authors acknowledge the support of the i3S Bioinformatics Service for the TCGA analysis. The results published are in part based upon data generated by the TCGA Research Network: https://www.cancer.gov/tcga. The Flow Cytometry technique was performed at the Translational Cytometry i3S Scientific Platform, with the assistance of Emília Cardoso and Catarina Meireles.
Authors’ contributions
Conception and study design: Vasconcelos MH, Xavier CPR
Acquisition of data: Matos BS, Peixoto da Silva S
Analysis and interpretation of data: Matos BS, Peixoto da Silva S, Xavier CPR
Statistical analysis: Matos BS, Peixoto da Silva S, Xavier CPR
Manuscript preparation: Matos BS, Xavier CPR
Manuscript revision: Matos BS, Vasconcelos MH, Xavier CPR
Availability of data and materials
All data supporting our findings are available from the corresponding author upon request.
Financial support and sponsorship
This work was funded (in part) by Programa Operacional Regional do Norte and co-funded by the European Regional Development Fund under the project “The Porto Comprehensive Cancer Center” with the reference NORTE-01-0145-FEDER-072678-Consórcio PORTO.CCC - Porto.Comprehensive Cancer Center. Xavier CPR is supported by the Fundação para a Ciência e Tecnologia (FCT) and Fundo Social Europeu (FSE), Portugal, through the post-doc grant SFRH/BPD/122871/2016.
Conflicts of interest
Xavier CPR is a Junior Editorial Board Member of the journal Cancer Drug Resistance, while the other authors have declared that they have no conflicts of interest.
Ethical approval and consent to participate
Not applicable.
Consent for publication
Not applicable.
Copyright
© The Author(s) 2024.
Supplementary Materials
REFERENCES
1. Alexander M, Kim SY, Cheng H. Update 2020: management of non-small cell lung cancer. Lung 2020;198:897-907.
2. Duma N, Santana-Davila R, Molina JR. Non-small cell lung cancer: epidemiology, screening, diagnosis, and treatment. Mayo Clin Proc 2019;94:1623-40.
3. Indini A, Rijavec E, Bareggi C, Grossi F. Novel treatment strategies for early-stage lung cancer: the oncologist’s perspective. J Thorac Dis 2020;12:3390-8.
4. Lim JU, Yeo CD. Update on adjuvant therapy in completely resected NSCLC patients. Thorac Cancer 2022;13:277-83.
5. Soon YY, Furnback W, Kim J, et al. Clinical trial and real-world outcomes of patients with metastatic NSCLC in the post-platinum-based chemotherapy failure setting. JTO Clin Res Rep 2023;4:100579.
6. Liu WJ, Du Y, Wen R, Yang M, Xu J. Drug resistance to targeted therapeutic strategies in non-small cell lung cancer. Pharmacol Ther 2020;206:107438.
7. Rotow J, Bivona TG. Understanding and targeting resistance mechanisms in NSCLC. Nat Rev Cancer 2017;17:637-58.
8. Assaraf YG, Brozovic A, Gonçalves AC, et al. The multi-factorial nature of clinical multidrug resistance in cancer. Drug Resist Updat 2019;46:100645.
9. Maloney SM, Hoover CA, Morejon-Lasso LV, Prosperi JR. Mechanisms of taxane resistance. Cancers 2020;12:3323.
10. Tian Y, Lei Y, Wang Y, Lai J, Wang J, Xia F. Mechanism of multidrug resistance to chemotherapy mediated by P-glycoprotein (Review). Int J Oncol 2023;63:119.
11. Wang C, Ding S, Sun B, et al. Hsa-miR-4271 downregulates the expression of constitutive androstane receptor and enhances in vivo the sensitivity of non-small cell lung cancer to gefitinib. Pharmacol Res 2020;161:105110.
12. Chen C, Hou J, Yu S, et al. Role of cancer-associated fibroblasts in the resistance to antitumor therapy, and their potential therapeutic mechanisms in non-small cell lung cancer. Oncol Lett 2021;21:413.
13. Han X, Xu Y, Geranpayehvaghei M, Anderson GJ, Li Y, Nie G. Emerging nanomedicines for anti-stromal therapy against desmoplastic tumors. Biomaterials 2020;232:119745.
14. Zhang H, Jiang H, Zhu L, Li J, Ma S. Cancer-associated fibroblasts in non-small cell lung cancer: recent advances and future perspectives. Cancer Lett 2021;514:38-47.
15. Lin Y, Xu Z, Zhou B, Ma K, Jiang M. Pentoxifylline inhibits pulmonary fibrosis by regulating cellular senescence in mice. Front Pharmacol 2022;13:848263.
16. Wen WX, Lee SY, Siang R, Koh RY. Repurposing pentoxifylline for the treatment of fibrosis: an overview. Adv Ther 2017;34:1245-69.
17. Xavier CPR, Castro I, Caires HR, et al. Chitinase 3-like-1 and fibronectin in the cargo of extracellular vesicles shed by human macrophages influence pancreatic cancer cellular response to gemcitabine. Cancer Lett 2021;501:210-23.
18. Bravo-Cuellar A, Ortiz-Lazareno PC, Lerma-Diaz JM, et al. Sensitization of cervix cancer cells to adriamycin by pentoxifylline induces an increase in apoptosis and decrease senescence. Mol Cancer 2010;9:114.
19. Bravo-Cuellar A, Ortiz-Lazareno PC, Sierra-Díaz E, et al. Pentoxifylline sensitizes cisplatin-resistant human cervical cancer cells to cisplatin treatment: involvement of mitochondrial and NF-kappa B pathways. Front Oncol 2020;10:592706.
20. Cancino-Marentes ME, Hernández-Flores G, Ortiz-Lazareno PC, et al. Sensitizing the cytotoxic action of docetaxel induced by pentoxifylline in a PC3 prostate cancer cell line. BMC Urol 2021;21:38.
21. Kim JH, Shin BC, Park WS, Lee J, Kuh HJ. Antifibrotic effects of pentoxifylline improve the efficacy of gemcitabine in human pancreatic tumor xenografts. Cancer Sci 2017;108:2470-7.
22. Ohsaki Y, Ishida S, Fujikane T, Kikuchi K. Pentoxifylline potentiates the antitumor effect of cisplatin and etoposide on human lung cancer cell lines. Oncology 1996;53:327-33.
23. Goel PN, Gude RP. Curbing the focal adhesion kinase and its associated signaling events by pentoxifylline in MDA-MB-231 human breast cancer cells. Eur J Pharmacol 2013;714:432-41.
24. Goel PN, Gude RP. Unravelling the antimetastatic potential of pentoxifylline, a methylxanthine derivative in human MDA-MB-231 breast cancer cells. Mol Cell Biochem 2011;358:141-51.
25. Wang Y, Dong L, Li J, Luo M, Shang B. Pentoxifylline induces apoptosis of HepG2 cells by reducing reactive oxygen species production and activating the MAPK signaling. Life Sci 2017;183:60-8.
26. Al-husein BA, Mhaidat NM, Alzoubi KH, et al. Pentoxifylline induces caspase-dependent apoptosis in colorectal cancer cells. Inform Med Unlocked 2022;31:100997.
27. Rao FV, Andersen OA, Vora KA, Demartino JA, van Aalten DM. Methylxanthine drugs are chitinase inhibitors: investigation of inhibition and binding modes. Chem Biol 2005;12:973-80.
28. Chen HT, Zheng JM, Zhang YZ, et al. Overexpression of YKL-40 predicts poor prognosis in patients undergoing curative resection of pancreatic cancer. Pancreas 2017;46:323-34.
29. De Robertis M, Greco MR, Cardone RA, et al. Upregulation of YKL-40 promotes metastatic phenotype and correlates with poor prognosis and therapy response in patients with colorectal cancer. Cells 2022;11:3568.
30. Lee YE, Chan TC, Tian YF, et al. High expression of chitinase 3-like-1 is an unfavorable prognostic factor in urothelial carcinoma of upper urinary tract and urinary bladder. Urol Oncol 2019;37:299.e7-18.
31. Jefri M, Huang YN, Huang WC, Tai CS, Chen WL. YKL-40 regulated epithelial-mesenchymal transition and migration/invasion enhancement in non-small cell lung cancer. BMC Cancer 2015;15:590.
32. Park KR, Yun HM, Yoo K, Ham YW, Han SB, Hong JT. Chitinase 3 like 1 suppresses the stability and activity of p53 to promote lung tumorigenesis. Cell Commun Signal 2020;18:5.
33. Zhao T, Su Z, Li Y, Zhang X, You Q. Chitinase-3 like-protein-1 function and its role in diseases. Signal Transduct Target Ther 2020;5:201.
34. Geng B, Pan J, Zhao T, et al. Chitinase 3-like 1-CD44 interaction promotes metastasis and epithelial-to-mesenchymal transition through β-catenin/Erk/Akt signaling in gastric cancer. J Exp Clin Cancer Res 2018;37:208.
35. Jaén M, Martín-Regalado Á, Bartolomé RA, Robles J, Casal JI. Interleukin 13 receptor alpha 2 (IL13Rα2): expression, signaling pathways and therapeutic applications in cancer. Biochim Biophys Acta Rev Cancer 2022;1877:188802.
36. Yu JE, Yeo IJ, Han SB, et al. Significance of chitinase-3-like protein 1 in the pathogenesis of inflammatory diseases and cancer. Exp Mol Med 2024;56:1-18.
37. Pesic M, Markovic JZ, Jankovic D, et al. Induced resistance in the human non small cell lung carcinoma (NCI-H460) cell line in vitro by anticancer drugs. J Chemother 2006;18:66-73.
38. Podolski-Renić A, Jadranin M, Stanković T, et al. Molecular and cytogenetic changes in multi-drug resistant cancer cells and their influence on new compounds testing. Cancer Chemother Pharmacol 2013;72:683-97.
39. Silva BR, Rebelo R, Rodrigues JM, Xavier CPR, Vasconcelos MH, Queiroz MRP. Synthesis of novel methyl 3-(hetero)arylthieno[3,2-b]pyridine-2-carboxylates and antitumor activity evaluation: studies in vitro and in ovo grafts of chick chorioallantoic membrane (CAM) with a triple negative breast cancer cell line. Molecules 2021;26:1594.
40. Vichai V, Kirtikara K. Sulforhodamine B colorimetric assay for cytotoxicity screening. Nat Protoc 2006;1:1112-6.
41. Branco H, Oliveira J, Antunes C, Santos LL, Vasconcelos MH, Xavier CPR. Pirfenidone sensitizes NCI-H460 non-small cell lung cancer cells to paclitaxel and to a combination of paclitaxel with carboplatin. Int J Mol Sci 2022;23:3631.
42. Teixeira A, DaCunha DC, Barros L, et al. Eucalyptus globulus Labill. decoction extract inhibits the growth of NCI-H460 cells by increasing the p53 levels and altering the cell cycle profile. Food Funct 2019;10:3188-97.
43. Castro I, Lopes-Rodrigues V, Branco H, Vasconcelos MH, Xavier CPR. Establishing and characterizing a novel doxorubicin-resistant acute myeloid leukaemia cell line. J Chemother 2023;35:307-21.
44. Lopes-Rodrigues V, Oliveira A, Correia-da-Silva M, et al. A novel curcumin derivative which inhibits P-glycoprotein, arrests cell cycle and induces apoptosis in multidrug resistance cells. Bioorg Med Chem 2017;25:581-96.
45. Lopes-Rodrigues V, Di Luca A, Mleczko J, et al. Identification of the metabolic alterations associated with the multidrug resistant phenotype in cancer and their intercellular transfer mediated by extracellular vesicles. Sci Rep 2017;7:44541.
46. Lopes-Rodrigues V, Di Luca A, Sousa D, et al. Multidrug resistant tumour cells shed more microvesicle-like EVs and less exosomes than their drug-sensitive counterpart cells. Biochim Biophys Acta 2016;1860:618-27.
47. Bian B, Li L, Yang J, et al. Prognostic value of YKL-40 in solid tumors: a meta-analysis of 41 cohort studies. Cancer Cell Int 2019;19:259.
48. Barancik M, Bohacova V, Gibalova L, Sedlak J, Sulova Z, Breier A. Potentiation of anticancer drugs: effects of pentoxifylline on neoplastic cells. Int J Mol Sci 2012;13:369-82.
49. Drobná Z, Stein U, Walther W, Barancík M, Breier A. Pentoxifylline influences drug transport activity of P-glycoprotein and decreases mdrl gene expression in multidrug resistant mouse leukemic L1210/VCR cells. Gen Physiol Biophys 2002;21:103-9.
50. Feng C, Zhang M, Zhang S, Zhang J, Li C, Zhou J. Therapeutic effects of pentoxifylline on invasive pulmonary aspergillosis in immunosuppressed mice. BMC Pulm Med 2021;21:31.
51. Gude RP, Binda MM, Presas HL, Klein-Szanto AJ, Bonfil RD. Studies on the mechanisms responsible for inhibition of experimental metastasis of B16-F10 murine melanoma by pentoxifylline. J Biomed Sci 1999;6:133-41.
52. Kamran MZ, Gude RP. Pentoxifylline inhibits melanoma tumor growth and angiogenesis by targeting STAT3 signaling pathway. Biomed Pharmacother 2013;67:399-405.
53. Park KR, Yun HM, Hong JT. G721-0282 inhibits cell growth and induces apoptosis in human osteosarcoma through down-regulation of the STAT3 pathway. Int J Biol Sci 2020;16:330-41.
54. Lee DH, Kim KC, Hwang CJ, et al. Decreased lung tumor development in SwAPP mice through the downregulation of CHI3L1 and STAT 3 activity via the upregulation of miRNA342-3p. Mol Ther Nucleic Acids 2019;16:63-72.
55. Singh SK, Bhardwaj R, Wilczynska KM, Dumur CI, Kordula T. A complex of nuclear factor I-X3 and STAT3 regulates astrocyte and glioma migration through the secreted glycoprotein YKL-40. J Biol Chem 2011;286:39893-903.
56. Yan C, Ding X, Wu L, Yu M, Qu P, Du H. Stat3 downstream gene product chitinase 3-like 1 is a potential biomarker of inflammation-induced lung cancer in multiple mouse lung tumor models and humans. PLoS One 2013;8:e61984.
57. Castellanos-Esparza YC, Wu S, Huang L, et al. Synergistic promoting effects of pentoxifylline and simvastatin on the apoptosis of triple-negative MDA-MB-231 breast cancer cells. Int J Oncol 2018;52:1246-54.
58. Yeo IJ, Lee CK, Han SB, Yun J, Hong JT. Roles of chitinase 3-like 1 in the development of cancer, neurodegenerative diseases, and inflammatory diseases. Pharmacol Ther 2019;203:107394.
59. Chiang YC, Lin HW, Chang CF, et al. Overexpression of CHI3L1 is associated with chemoresistance and poor outcome of epithelial ovarian carcinoma. Oncotarget 2015;6:39740-55.
60. Gong M, Li Y, Ye X, et al. Loss-of-function mutations in KEAP1 drive lung cancer progression via KEAP1/NRF2 pathway activation. Cell Commun Signal 2020;18:98.
61. Adinolfi S, Patinen T, Jawahar Deen A, et al. The KEAP1-NRF2 pathway: targets for therapy and role in cancer. Redox Biol 2023;63:102726.
62. Telkoparan-Akillilar P, Panieri E, Cevik D, Suzen S, Saso L. Therapeutic targeting of the NRF2 signaling pathway in cancer. Molecules 2021;26:1417.
Cite This Article
Export citation file: BibTeX | EndNote | RIS
OAE Style
Matos BS, Peixoto da Silva S, Vasconcelos MH, Xavier CPR. Chemosensitizing effect of pentoxifylline in sensitive and multidrug-resistant non-small cell lung cancer cells. Cancer Drug Resist 2024;7:19. http://dx.doi.org/10.20517/cdr.2024.04
AMA Style
Matos BS, Peixoto da Silva S, Vasconcelos MH, Xavier CPR. Chemosensitizing effect of pentoxifylline in sensitive and multidrug-resistant non-small cell lung cancer cells. Cancer Drug Resistance. 2024; 7: 19. http://dx.doi.org/10.20517/cdr.2024.04
Chicago/Turabian Style
Beatriz S. Matos, Sara Peixoto da Silva, M. Helena Vasconcelos, Cristina P. R. Xavier. 2024. "Chemosensitizing effect of pentoxifylline in sensitive and multidrug-resistant non-small cell lung cancer cells" Cancer Drug Resistance. 7: 19. http://dx.doi.org/10.20517/cdr.2024.04
ACS Style
Matos, BS.; Peixoto da Silva S.; Vasconcelos MH.; Xavier CPR. Chemosensitizing effect of pentoxifylline in sensitive and multidrug-resistant non-small cell lung cancer cells. Cancer Drug Resist. 2024, 7, 19. http://dx.doi.org/10.20517/cdr.2024.04
About This Article
Special Issue
Copyright
Data & Comments
Data
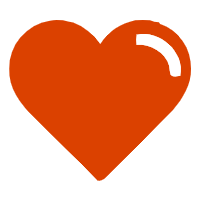
Comments
Comments must be written in English. Spam, offensive content, impersonation, and private information will not be permitted. If any comment is reported and identified as inappropriate content by OAE staff, the comment will be removed without notice. If you have any queries or need any help, please contact us at support@oaepublish.com.