Lipid and protein oxidation in Cyprinus carpio muscle by environmentally relevant concentrations of glibenclamide
Abstract
Diabetes mellitus (DM2) was considered a more common comorbidity and was associated with high mortality due to metabolic disease in the COVID-19 pandemic. For this reason, glibenclamide (GLI) was commonly prescribed for its control. However, it has been found in environmentally relevant concentrations in various water reservoirs due to its high consumption. Common carp (Cyprinus carpio), besides its nutritional properties and economic importance, is also considered a good bioindicator for assessing environmental health and pollutants presence; nonetheless, there are currently insufficient studies on the effect of GLI on the physicochemical and textural properties of the muscle of this bioindicator species. In this study, the effect of this drug at two environmentally relevant concentrations (50 and 1,000 ng/L) at five exposure times (every 24 up to 96 h) on the quality of carp muscle was investigated. Parameters such as carbonylated proteins, lipid peroxidation, total sulfhydryl content, water holding capacity, pH, electrophoretic profile, and texture profile analysis were determined. Regardless of the concentration of GLI used, the evaluated parameters showed significant muscle damage; therefore, it must be emphasized that this emerging pollutant not only damages environment, but also affects edible species present in different water reservoirs.
Keywords
INTRODUCTION
The presence of pharmaceuticals and their metabolites in the environment has emerged as a burgeoning concern owing to their potential deleterious impacts on aquatic ecosystems and human health. The contemporary lifestyle prevalent in our society has contributed to a surge in the prevalence of overweight and obesity, leading to the escalation of chronic degenerative ailments, including Type II Diabetes Mellitus (DM2), coronary heart disease, arterial hypertension, and even cancer. Furthermore, there exists a direct correlation between DM2 and the mortality rate associated with COVID-19[1,2].
According to the International Diabetes Federation[3], it is projected that the number of adults affected by diabetes will increase from 463 million to 578 million by the year 2030. This anticipated rise indicates a significant surge in the utilization of disease control medications. Among these medications, the sulfonylurea hypoglycemic drug Glibenclamide (GLI) holds a prominent position, being widely prescribed globally. Consequently, the presence of Glibenclamide has been detected in hospital effluents, with concentrations of 2.03 µg/L observed in Toluca (Mexico), ranging from 0.6 to 4.6 × 10-3 µg/L in Catalonia, 2.0 µg/L in the Ebro River, and 15.9 µg/L in Barcelona (Spain) within hospital wastewater that had undergone conventional disposal treatments. Furthermore, Glibenclamide was found at a concentration of 70 ng/L in the Ahar River (India), which is present in urban and industrial wastewater effluents[4-8]. This position GLI as an emerging contaminant of ecotoxicological significance, as its impact on aquatic life has been evidenced through biomarkers of oxidative stress, including lipoperoxidation (LPx) and carbonylated proteins (PCC), as well as the evaluation of superoxide dismutase and catalase, revealing damage to organs such as the brain, liver, gills, and blood of common carp (Cyprinus carpio) at concentrations of 50, 100, and 1,000 ng/L, as well as in Danio rerio and male albino rat neonates exposed to GLI[9-12]. These effects are correlated with oxidation processes, occurring both in its active form and in its biotransformed states, specifically 3 cis and 4 trans-hydroxy derivatives[13]. When deposited in tissues susceptible to peroxidation, such as cell membranes, this alteration disrupts the physiological functions of organisms[14].
The oxidation of unsaturated lipids in fish results in the generation of unstable hydroperoxides, which, upon fragmentation, liberate ketones, fatty acids, and low-molecular-weight aldehydes, notably malondialdehyde (MDA). This MDA serves as an intermediate for quantifying the extent of damage via this pathway, leading to nutritional degradation and the emergence of unpalatable tastes and odors. In addition, these intermediates (MDA and ketones) can exert an impact on proteins, potentially manifesting in changes in texture[15-17].
Cyprinus carpio holds significant global economic value[18]. It is classified as a semi-fat fish, characterized by its fat content reaching as high as 14%, consisting of polyunsaturated fatty acids, along with a protein content of 16%[19,20]. Despite the abundance of evidence showcasing the impact of emerging contaminants on the lipid and protein components in aquaculture species, there remains a paucity of studies investigating the oxidative effects on muscle quality, particularly at concentrations typically encountered within aquatic environments. Hence, the aim of this study was to assess the impact of GLI at concentrations prevalent in aquatic environments, specifically targeting the quantification of LPx, PCC and their effects on the textural and physicochemical properties of Cyprinus carpio. This evaluation was conducted under exposure conditions of 50 and 1,000 ng/L, representing the minimum and maximum environmental concentrations found in effluents and water bodies, with discernible and evident toxicological consequences.
EXPERIMENTAL
Test xenobiotic
A 1 L solution of GLI was prepared using glibenclamide sourced from Vertec, Sigma-Aldrich (Toluca, State of Mexico), purity exceeding 99%, and CAS number 10236-21-8. Preparation was performed by simply mixing the glibenclamide in the dilution water by mechanical means in distilled water containing 0.02%
Transport and acclimatization of the bioindicator
For the present study, Cyprinus carpio, four-month-old juvenile stage (nonsexual maturity nor final growth size), with an average weight of 145 ± 9.3 g and a length of 19 ± 0.8 cm (n = 50), from the Tiacaque Aquatic Center in the State of Mexico were used, fish were transported in vivo in chlorine-free water in polyethylene bags to ensure adequate oxygenation to maintain life. The bioindicators were acclimated for approximately 15 days in aquaria featuring the following water parameters: hardness of 18.5 ± 0.6 mg/L, alkalinity of
GLI exposure
Two concentrations were chosen from a range identified in various aquatic environments: 50 and
Oxidized compounds
Lipid oxidation
The level of oxidation was quantified based on malondialdehyde intermediate formation during lipid oxidation reactions. To accomplish this, the 2-thiobarbituric acid assay (TBARS using reagent from Fluka, Sigma-Aldrich, Toluca, State of Mexico, Mexico) following Büege and Aust[25] method was employed. 50 µL of the sample was mixed with a reagent combination of thiobarbituric acid-trichloroacetic acid (TCA) 0.37%-15% (Sigma-Aldrich, St. Louis, MO, USA) and Tris-HCL buffer (J.T. Baker, Pennsylvania, PA, USA). This mixture was heated in water bath for 15 min, followed by rapid cooling (-4 °C/3 min) and subsequent incubation (37 °C/30 min). The supernatant obtained via centrifugation (10 min/3,500 rpm) was then analyzed at a ƛ 353 nm using a GENESYS™ 10S Vis spectrophotometer (Thermo Scientific, Madison, WI, USA). Results were reported as nM MDA (malondialdehyde) per mg, utilizing an extinction coefficient (CEM) of 1.56 × 105 M-1·cm-1.
Oxidation of proteins
PCC were quantified by reacting the supernatant with 10 mM dinitrophenylhydrazine from Sigma-Aldrich, St Louis, MO, USA, as described in Burcham[26], with some modifications. The button resulting from 20% TCA precipitation was washed and dissolved in 6 M guanidine from Sigma-Aldrich, St Louis, MO, USA. Measurements were made at 366 nm, expressed as nM reactive carbonyls (C=O)/mg proteins in wet tissue using CEM 21,000 M-1·cm-1.
SDS-PAGE
Into the polyacrylamide gels prepared according to García-Carreño et al., 20 µL of the samples with high and low GLI concentrations at different time points and 10 µL of the Biorad tracer (CAT No. 161-0375) were injected in the Mini-PROTEAM system (CAT No. 1658050), Bio-Rad Laboratories, Inc., Hercules, CA, USA at 200 v/ 4 °C followed by staining with Coomassie Brilliant Blue R-250 from Sigma-Aldrich, St Louis, MO, USA, Subsequently, gels were stained using Coomassie Brilliant Blue R-250 sourced from Sigma-Aldrich, St. Louis, MO, USA, allowing for identification of protein bands based on molecular weight[27].
Texture properties
Warner-Bratzler Share force
The Warner-Bratzler knife (HDP/BSW, Stable MicroSystems, Surrey, UK) was adapted to the texture meter TA-XT2 v 2.63 (Texture Technology Corporation, Scarsdale, New York, USA), where the resistance (Newtons) was determined when cutting muscle (3 cm2) at 1 mm/s according to de Huidobro et al.[28].
Texture profile analysis
Using a TA-XT2 Texutrometer, hardness, cohesiveness, elasticity, gumminess, and chewiness were determined according to Jafarpour and Gorczyca[29], applying a 5 K load cell to protein gels (50 mg/mL) obtained by gelation using temperature ramping according to Silva-Ríos et al.[30].
Physicochemical and textural properties
Sulfhydryl group
A total of 1 mL of protein concentrate (PC) was prepared using the method described by Morachis-Valdez et al. and subsequently dissolved in 8M urea (J.T. Baker, Pennsylvania, PA, USA)[31]. The reaction was initiated with the addition of 5,5'-dithio-bis-2-nitrobenzoic acid (Sigma-Aldrich, St. Louis, MO, USA), and the absorbance was measured at 412 nm. The results are presented as µM of SH (sulfhydryl) per milligram of protein using the Ellman method[32].
pH
The pH was determined using a digital potentiometer (Hanna instrument pH 210, Ukraine) according to the method AOAC 981.12/2002.
Water holding capacity
Five g of muscle were shaken with a glass rod for 1 min with 0.6 M NaCl, allowed to settle for 30 min, shaken again, and centrifuged (3,500 rpm/15 min) in Eppendorf Centrifuge 5810R, Madrid, Spain, indicating NaCl/100 g retention, according to Dublán-García[33].
Statistical analysis
ANOVA and Tukey’s test were used for mean differences (P < 0.05) using the program SPSS, v17 Martínez-Viveros et al.[9].
RESULTS AND DISCUSSION
Oxidized compounds
A significant increase (P < 0.05) was observed in MDA and PCC at different exposure times, from 1,268% to 1,176% in malondialdehyde at 50 ng/L and from 67% to 608% at 1,000 ng/L, while carbonylated products increased from 256% to 439% at 50 ng/L and decreased from 117% to 115% at 1,000 ng/L starting from 48 h
Figure 1. (A) Changes in LPx of Cyprinus carpio muscle expressed as nM MDA/mg protein exposed to two concentrations of GLI (50 and 1,000 ng/L) for 12, 24, 48, 72 and 96 h. Values are the means of three replicates ± SE. *Significant differences from control group;
SDS-PAGE
In the electrophoretic profile, the appearance of low-molecular-weight bands with increasing exposure time is observed for both GLI concentrations compared with the control [Figure 1C].
Texture properties
For the shear force [Figure 1D], a significant decrease (P < 0.05) was observed starting at 48 h for the high concentration, dropping from 38% to 18%. However, this decrease was not observed for the low concentration, which increased from 24% to 28% in 72 h. The results of the texture profile analysis (TPA) presented in Table 1 indicate that, following the exposure period, all response variables for both concentrations exhibited a significant decrease (P < 0.05) compared with the control and the previous exposure time.
Results of texture profile analysis in Cyprinus carpio muscle exposed to two concentrations of GLB (50 ng/L and 1,000 ng/L) for 0, 24, 48, 72 and 96 h
Exposure time | ||||||
0 h | 24 h | 48 h | 72 h | 96 h | ||
Hardness | Control | 1.087 ± 0.021 | 1.022 ± 0.005 | 0.980 ± 0.009a | 0.946 ± 0.008a | 0.929 ± 0.003a |
50 ng/L | 0.955 ± 0.007 | 0.092 ± 0.006*a | 0.040 ± 0.003*a | 0.029 ± 0.002*a | 0.010 ± 0.000*a | |
100 ng/L | 0.922 ± 0.005 | 0.066 ± 0.001*a | 0.024 ± 0.002*a | 0.011 ± 0.001*a | 0.005 ± 0.001*a | |
Cohesiveness | Control | 0.015 ± 0.001 | 0.013 ± 0.001 | 0.012 ± 0.000a | 0.012 ± 0.000a | 0.010 ± 0.000a |
50 ng/L | 0.014 ± 0.001 | 0.001 ± 0.000*a | 0.001 ± 0.000*a | 0.001 ± 0.000*a | 0.001 ± 0.000*a | |
100 ng/L | 0.014 ± 0.000 | 0.001 ± 0.000*a | 0.001 ± 0.000*a | 0.001 ± 0.000*a | 0.001 ± 0.000*a | |
Elasticity | Control | 0.367 ± 0.015 | 0.347 ± 0.015 | 0.333 ± 0.015a | 0.277 ± 0.006a | 0.253 ± 0.001a |
50 ng/L | 0.363 ± 0.006 | 0.001 ± 0.000*a | 0.001 ± 0.000*a | 0.001 ± 0.000*a | 0.001 ± 0.000*a | |
100 ng/L | 0.330 ± 0.010 | 0.001 ± 0.000*a | 0.001 ± 0.000*a | 0.001± 0.000*a | 0.001 ± 0.000*a | |
Chewiness | Control | 0.006 ± 0.000 | 0.005 ± 0.000 | 0.004 ± 0.000a | 0.003 ± 0.000a | 0.002 ± 0.000a |
50 ng/L | 0.005 ± 0.000 | 0.000*a | 0.000*a | 0.000*a | 0.000*a | |
100 ng/L | 0.005 ± 0.000 | 0.000*a | 0.000*a | 0.000*a | 0.000*a | |
Gumminess | Control | 0.016 ± 0.000 | 0.013 ± 0.000 | 0.012 ± 0.000a | 0.011 ± 0.000a | 0.009 ± 0.000a |
50 ng/L | 0.013 ± 0.000 | 0.000*a | 0.000*a | 0.000*a | 0.000*a | |
100 ng/L | 0.012 ± 0.000 | 0.000*a | 0.000*a | 0.000*a | 0.000*a |
Physicochemical properties
For the sulfhydryl groups, there was a significant decrease (P < 0.05) in both GLI concentrations (high and low) at the different exposure times compared with the control; specifically, at 50 ng/L, there was a reduction of 45.5%, while at 1,000 ng/L, the decrease was even more pronounced at 90.9% [Figure 2A].
Figure 2. (A) Changes in sulfhydryl group (- SH) in Cyprinus carpio muscle expressed as µM - SH/mg protein exposed to two different concentrations of GLB (C1: 50 ng/L and C2: 1,000 ng/L) for 0, 24, 48, 72, and 96 h. Values are the means of three replicates ± SE. *Significant differences from control group; aDifferent letters represent statistically significant differences between concentrations ANOVA, and Tukey (P < 0.05); (B) Changes in water holding capacity (WHC) in Cyprinus carpio muscle expressed as NaCl/100 g retention exposed to two different concentrations of GLB (C1: 50 ng/L and C2: 1,000 ng/L) for 0, 24, 48, 72, and 96 h. Values are the means of three replicates ± SE. *Significant differences from control group; aDifferent letters represent statistically significant differences between concentrations (P < 0.05) ANOVA, and Tukey (P < 0.05); (C) Changes in pH in Cyprinus carpio muscle exposed to two different concentrations of GLB (C1: 50 ng/L and C2:1000 ng/L) for 0, 24, 48, 72, and 96 h. Values are the means of three replicates ± SE.
A significant decrease in water holding capacity (P < 0.05) was observed for both the high and low concentrations of GLI after 48 h, from 22% to 43% and from 20% to 37%, respectively [Figure 2B].
pH showed a decrease for both concentrations (high and low) of GLI until 72 h, of one and two percentual points [Figure 2C].
Discussion
Prior studies conducted within the research group have shown the impact of xenobiotic presence, including antidepressants (fluoxetine)[34]; hormones (17-β-estradiol)[35]; antibiotics (amoxicillin)[36]; plastic precursors (bisphenol A)[37,38]; sweeteners (Acesulfame potassium)[39] and sucralose[40,41]; caffeine[42]; nonsteroidal anti-inflammatory drugs (ibuprofen and diclofenac)[43]; competitive inhibitors (captopril)[44]; hospital effluents[45]; and reservoir contaminants[46]. These compounds have been found at environmentally relevant concentrations in various water bodies, stemming from anthropogenic and industrial activities, causing harmful effects, notably oxidative stress, in different organs (brain, gills, liver, and blood) of environmentally significant bioindicators such as Daphnia magna, Danio rerio, Xenopus laevis, and economically important species such as the common carp (Cyprinus carpio). While damage has been documented, investigations into fish muscle are of particular interest because of its role as an important source of high-biological-value protein.
In addition, previous studies have demonstrated protein damage resulting from oxidation caused by the presence of oxidizing agents (mentioned earlier), ionizing radiation, ultrasonication[47], and peroxide radical initiation[48], with observations of modifications in the amino acid chains[47], particularly in the essential ones. This phenomenon reduces nutritional properties[31]. Therefore, the identification of environmentally relevant levels of various xenobiotics can potentially result in a decline in nutritional quality.
Glibenclamide, a drug belonging to the sulfonylurea family, has been documented as having systemic phototoxic properties[49], a phenomenon attributed to the presence of aryl-halogen bonds, which can undergo photofragmentation upon direct UV light exposure, generating aryl radicals that can be replaced by a hydrogen atom or other molecular segments provided by the surrounding medium, or even undergo dimerization. In addition, the molecular segments possessing the highest electronic density are susceptible to interactions with electrophilic species. For instance, the aromatic segment with the alkyl substituent exhibits reactivity that can be influenced by the sulfamoyl group [Figure 3]. In essence, the photochemical transformation of the drug can form free radicals and participate as a photosensitizer in the formation of reactive oxygen species such as superoxide anions, hydroxyl radicals, and molecular singlet oxygen[50], in addition to those formed by the biotransformation of GLI by CYP450[10,51], revealing oxidative stress on biomarkers in the brain, gill, liver, and blood upon acute exposure of common carp to GLI.
Figure 3. Structure of glibenclamide (GLI), 5-chloro-N-(2-{4-[(cyclohexylcarbamoyl)sulfamoyl]phenyl}ethyl)-2-methoxybenzenecarboxamide.
The current study revealed an elevated concentration of MDA in muscle tissue because of acute exposure to both GLI concentrations (50 and 1,000 ng/L) [Figure 1A]. This increase in MDA facilitates the oxidation of unsaturated lipids in Cyprinus carpio muscle, and Dominguez emphasizes the high susceptibility of this muscle tissue to oxidation, which occurs primarily through the autoxidation mechanism - a chain reaction involving the formation of free radicals, yielding various derivative compounds such as hydroperoxides, aldehydes, ketones, and ultimately, end products such as alkanes, alcohols, and carbonyl compounds[52]. This process results in the deterioration and loss of textural and physicochemical properties, including gelling and water-holding capacity[31].
Furthermore, these by-products, along with reactive oxygen species (ROS), are widely acknowledged to induce protein oxidation reactions[53,54]. In the present study, an increase in the formation of protein carbonyl compounds (PCC) was demonstrated, showing a dependency on the exposure time and both concentrations of GLI. This increase in PCC formation is attributed to radical formation reactions, elongation reactions, branching reactions, and termination reactions. These mechanisms differ from lipid oxidation reactions and glycation processes[53]. Notably, these mechanisms have been extensively described in prior studies by Lund et al., Estévez, and Domínguez et al.[15,16,52].
The oxidation induced by GLI in both concentrations resulted in a noticeable alteration of the electrophoretic profile of myofibrillar proteins, as illustrated in Figure 1C. This alteration is characterized by a reduction in the intensity of the protein bands, coupled with the emergence of low-molecular-weight bands in both myosin and actin. It is worth noting that actin, which is typically more stable, was also affected by these changes[55]. This occurrence of protein fragmentation coincides with the findings of Liu and Xiong[41], who induced oxidation through FeCl3/H2O2/ascorbate. The fragmentation of proteins may arise due to the direct impact of hydroxyl radicals (•OH) on the polypeptide backbone or on the side chains of glutamyl or prolyl residues.
In addition, it has been reported that the decrease in myofibrillar protein bands could be linked to the formation of high-molecular-weight aggregates, which, under the pore size limitations of SDS-PAGE, are unable to permeate the gel network and consequently accumulate on its surface. Carbonyls formed during oxidation can react with the free amino groups of unoxidized amino acids, creating amide bonds[56]. The formation of bityrosine between protein molecules can also prompt polymerization and aggregation, whereas Schiff bases arising from oxidized myofibrils can generate aggregates[55-58].
Fish myosin is characterized by a substantial abundance of thiol groups, which, when oxidized, can form disulfide bonds, playing a crucial role in maintaining the structure of myofibrillar protein-based gels[59]. Our present study observed a decrease in sulfhydryl groups (-SH) for both GLI concentrations compared with control. This finding aligns with the observations of Liu et al., Qian et al., and Eymard et al., who noted similar results in the muscle tissues of grass carp, silver carp, and mackerel, respectively[48,58,60]. It is widely acknowledged that the presence of reactive oxygen species (ROS), reactive nitrogen species (RNS), and/or reactive chlorine species (RCS) can trigger a diverse range of modifications, impacting enzyme activities and protein-protein interactions. -SH groups are particularly vulnerable to oxidation, especially in the presence of hydrogen peroxide, which accumulates within cells and is generated through the phase 1 biotransformation of GLI. Notably, the thiol groups in cysteine (amino acid prominently present in fish muscle along with the entirety of essential amino acids, as indicated by the FAO standard protein) are particularly susceptible to oxidation. This susceptibility leads to a series of oxidized products, including sulfenic acid (RSOH), sulfinic acid (RSOOH), and the cross-linking of disulfide groups (RSSR)[15,31,56,61,62]. Structural modification of the protein results in compromised functionality, affecting aspects such as gelling capacity and water holding capacity, as indicated in reference[47].
The water holding capacity serves as a quantitative indicator of retained water, which could reflect the structure of the protein gel network. In this study, a decrease in water holding capacity (WHC) was observed with increased exposure time at both concentrations [Figure 2B]. This decrease may be attributed to the degradation of myosin at higher oxidant concentrations, resulting in an unstable gel network. The instability of the gel network is further evidenced by the deterioration in textural properties observed after 24 h of exposure for both concentrations [Table 1], aligning with findings reported by Liu et al. and Zhou
CONCLUSIONS
GLI concentrations of 50 and 1,000 ng/L induced an escalation in the extent of lipoperoxidation and carbonylated proteins, resulting in substantial repercussions on Cyprinus carpio muscle. This led to the attenuation of its physicochemical and textural attributes, thereby diminishing its functional integrity and presumably affecting its nutritional value. The inability to establish a three-dimensional network further compromised its potential technological utility. This investigation underscores that the presence of this xenobiotic exerts not only ecological ramifications but also influences species of economic and nutritional significance. For forthcoming inquiries, it is imperative to discover the driving forces behind myofibrillar protein oxidation and their implications for fragmentation, aggregation, and polymerization. This entails considering the oxidant’s inherent properties, exposure duration, and the appropriateness of SDS-PAGE parameters concerning pore dimensions to comprehensively elucidate these phenomena. Furthermore, the integration of other methodologies, such as proteomics, can provide a more comprehensive understanding.
DECLARATIONS
AcknowledgmentsMartínez-Viveros EMG thanks the National Council of Science and Technology (Consejo Nacional de Ciencia y Tecnología, Mexico) for a graduate scholarship.
Authors’ contributionsConceptualization: Gómez-Oliván LM, Dublán-García O, Martínez-Viveros EMG, Morachis-Valdez AG
Methodology: Dublán-García O, Martínez-Viveros EMG, Saucedo-Vence K, Morachis-Valdez AG
Software: Saucedo-Vence K, Morachis-Valdez AG, Cira-Chávez LA
Validation: Dublán-García O, Morachis-Valdez AG, Cira-Chávez LA
Formal analysis: Dublán-García O, Martínez-Viveros EMG, Saucedo-Vence K, Gómez-Oliván LM, Morachis-Valdez AG
Investigation: Dublán-García O, Morachis-Valdez AG, Saucedo-Vence K, Martínez-Viveros EMG,
Resources: Dublán-García O
Data curation: Cira-Chávez LA, Martínez-Viveros EMG, Gómez-Oliván LM
Writing - original draft preparation: Dublán-García O, Morachis-Valdez AG, Saucedo-Vence K, Martínez-Viveros EMG
Writing - review and editing: Dublán-García O, Morachis-Valdez AG, Saucedo-Vence K
Visualization: Cira-Chávez LA, Saucedo-Vence K
Supervision: Dublán-García O, Gómez-Oliván, LM
Project administration: Dublán-García O, Saucedo-Vence K, Martínez-Viveros EMG, Morachis-Valdez AG
All authors have read and agreed to the published version of the manuscript.
Availability of data and materialsAll generated data on this study are included in this article. All materials are listed in the experimental section.
Financial support and sponsorshipNone.
Conflict of interestAll authors declared that there are no conflicts of interest.
Ethical approval and consent to participateThe study was conducted in accordance with the Organization for Economic Cooperation and Development. OECD guidelines for testing of chemicals. Guideline no. 203: fish, acute toxicity test. 1992.
Consent for publicationNot applicable.
Copyright© The Author(s) 2023.
REFERENCES
1. Xie J, Tong Z, Guan X, Du B, Qiu H. Clinical characteristics of patients who died of coronavirus disease 2019 in China. JAMA Netw Open 2020;3:e205619.
2. Moodie R, Stuckler D, Monteiro C, et al. Profits and pandemics: prevention of harmful effects of tobacco, alcohol, and ultra-processed food and drink industries. Lancet 2013;381:670-9.
3. Saeedi P, Petersohn I, Salpea P, et al. Global and regional diabetes prevalence estimates for 2019 and projections for 2030 and 2045: results from the International Diabetes Federation Diabetes Atlas, 9th edition. Diabetes Res Clin Pract 2019;157:107843.
4. Radjenovic J, Petrovic M, Barceló D. Analysis of pharmaceuticals in wastewater and removal using a membrane bioreactor. Anal Bioanal Chem 2007;387:1365-77.
5. Rebollo CP, Calvo MG, de Alda Villaizán MJL, Petrovic M, Martí AG, Culleres DB. Repercusiones sanitarias de la calidad del agua: los residuos de medicamentos en el agua. Revista de Salud Ambiental 2011;11:17-26. (in Spanish). Available from: https://www.ojs.diffundit.com/index.php/rsa/article/view/170. [Last accessed on 14 Sep 2023].
6. Ginebreda A, Jelić A, Petrović M, de Alda ML, Barceló D. New indexes for compound prioritization and complexity quantification on environmental monitoring inventories. Environ Sci Pollut Res Int 2012;19:958-70.
7. Luja-Mondragón M, Gómez-Oliván LM, SanJuan-Reyes N, et al. Alterations to embryonic development and teratogenic effects induced by a hospital effluent on Cyprinus carpio oocytes. Sci Total Environ 2019;660:751-64.
8. Williams M, Kookana RS, Mehta A, Yadav SK, Tailor BL, Maheshwari B. Emerging contaminants in a river receiving untreated wastewater from an Indian urban centre. Sci Total Environ 2019;647:1256-65.
9. Martínez-Viveros EMG, Islas-Flores H, Dublán-García O, et al. Environmentally relevant concentrations of glibenclamide induce oxidative stress in common carp (Cyprinus carpio). Chemosphere 2018;197:105-16.
10. Martínez Viveros EMG. Evaluation of the toxicity induced by glibenclamide and its influence on the quality of the meat of Cyprus carpio. (in Spanish). Available from: http://hdl.handle.net/20.500.11799/94982. [Last accessed on 15 Sep 2023].
11. Souza EQ, Bittencourt TQM, Ferreira RCB, et al. Exposição crônica ao cloridrato de metformina e à glibenclamida causa alterações comportamentais, glicêmicas e de mortalidade em Hemigrammus caudovittatus e Danio rerio. Arq Bras Med Vet Zootec 2019;71:1582-90. (in Spanish).
12. Tüzün S, Girgin FK, Sözmen EY, Menteş G, Ersöz B. Antioxidant status in experimental type 2 diabetes mellitus: effects of glibenclamide and glipizide on various rat tissues. Exp Toxicol Pathol 1999;51:436-41.
13. Radjenović J, Pérez S, Petrović M, Barceló D. Identification and structural characterization of biodegradation products of atenolol and glibenclamide by liquid chromatography coupled to hybrid quadrupole time-of-flight and quadrupole ion trap mass spectrometry. J Chromatogr A 2008;1210:142-53.
14. Livingstone DR. Oxidative stress in aquatic organisms in relation to pollution and aquaculture. Rev Med Vet 2003;154:427-30. Available from: https://www.revmedvet.com/2003/RMV154_427_430.pdf. [Last accessed on 14 Sep 2023]
15. Lund MN, Heinonen M, Baron CP, Estévez M. Protein oxidation in muscle foods: a review. Mol Nutr Food Res 2011;55:83-95.
17. Liu Y, Zhang L, Gao S, et al. Effect of protein oxidation in meat and exudates on the water holding capacity in bighead carp
18. Chen L, Xu J, Sun X, Xu P. Research advances and future perspectives of genomics and genetic improvement in allotetraploid common carp. Rev Aquac 2022;14:957-78.
19. Huss HH. Fresh fish: its quality and changes in quality. (in Spanish). Available from: https://www.fao.org/3/v7180s/v7180s00.htm#Contents. [Last accessed on 15 Sep 2023].
20. Ljubojević D, Đorđević V, Ćirković M. Evaluation of nutritive quality of common carp, Cyprinus carpio L. IOP Conf Ser Earth Environ Sci 2017;85:012013.
21. Radjenović J, Petrović M, Barceló D. Fate and distribution of pharmaceuticals in wastewater and sewage sludge of the conventional activated sludge (CAS) and advanced membrane bioreactor (MBR) treatment. Water Res 2009;43:831-41.
22. Verlicchi P, Al Aukidy M, Zambello E. Occurrence of pharmaceutical compounds in urban wastewater: removal, mass load and environmental risk after a secondary treatment - a review. Sci Total Environ 2012;429:123-55.
23. Osorio V, Larrañaga A, Aceña J, Pérez S, Barceló D. Concentration and risk of pharmaceuticals in freshwater systems are related to the population density and the livestock units in Iberian Rivers. Sci Total Environ 2016;540:267-77.
24. OECD guideline for testing of chemicals. Available from: https://www.oecd.org/chemicalsafety/risk-assessment/1948241.pdf. [Last accessed on 14 Sep 2023].
26. Burcham PC. Modified protein carbonyl assay detects oxidised membrane proteins: a new tool for assessing drug- and chemically-induced oxidative cell injury. J Pharmacol Toxicol Methods 2007;56:18-22.
27. García-Carreño FL, Dimes LE, Haard NF. Substrate-gel electrophoresis for composition and molecular weight of proteinases or proteinaceous proteinase inhibitors. Anal Biochem 1993;214:65-9.
28. Ruiz de Huidobro F, Miguel E, Blázquez B, Onega E. A comparison between two methods (Warner-Bratzler and texture profile analysis) for testing either raw meat or cooked meat. Meat Sci 2005;69:527-36.
29. Jafarpour A, Gorczyca EM. Characteristics of sarcoplasmic proteins and their interaction with surimi and kamaboko gel. J Food Sci 2009;74:N16-22.
30. Silva-Ríos A, Dublán-García O, Quintero-Salazar B, et al. Evaluation of physicochemical, functional and textural properties of rainbow trout (Oncorhynchus mykiss) stored at low temperatures. Afr J Biotechnol 2013;12:5087-96.
31. Morachis-Valdez AG, Gómez-Oliván LM, García-Argueta I, Hernández-Navarro MD, Díaz-Bandera D, Dublán-García O. Effect of chitosan edible coating on the biochemical and physical characteristics of carp fillet (Cyprinus carpio) stored at -18°C. Int J Food Sci 2017;2017:2812483.
33. Dublan-garcia O, Cruz-camarillo R, Guerrero-legarreta I, Ponce-alquicira E. Effect of refrigerated storage on proteolytic activity and physicochemical and microstructural properties of giant squid (Dosidicus gigas) mantle muscle. J Muscle Foods 2006;17:291-310.
34. Orozco-Hernández JM, Gómez-Oliván LM, Elizalde-Velázquez GA, et al. Fluoxetine-induced neurotoxicity at environmentally relevant concentrations in adult zebrafish Danio rerio. Neurotoxicology 2022;90:121-9.
35. Orozco-Hernández L, Gutiérrez-Gómez AA, SanJuan-Reyes N, et al. 17β-Estradiol induces cyto-genotoxicity on blood cells of common carp (Cyprinus carpio). Chemosphere 2018;191:118-27.
36. Elizalde-velázquez A, Gómez-oliván LM, Islas-flores H, et al. Ecotoxicological studies of pharmaceuticals in aquatic organisms. In:
37. Heredia-García G, Gómez-Oliván LM, Elizalde-Velázquez GA, et al. Multi-biomarker approach and IBR index to evaluate the effects of bisphenol A on embryonic stages of zebrafish (Danio rerio). Environ Toxicol Pharmacol 2022;94:103925.
38. Heredia-García G, Elizalde-Velázquez GA, Gómez-Oliván LM, et al. Realistic concentrations of Bisphenol-A trigger a neurotoxic response in the brain of zebrafish: oxidative stress, behavioral impairment, acetylcholinesterase inhibition, and gene expression disruption. Chemosphere 2023;330:138729.
39. Cruz-Rojas C, SanJuan-Reyes N, Fuentes-Benites MPAG, et al. Acesulfame potassium: its ecotoxicity measured through oxidative stress biomarkers in common carp (Cyprinus carpio). Sci Total Environ 2019;647:772-84.
40. Saucedo-Vence K, Elizalde-Velázquez A, Dublán-García O, et al. Toxicological hazard induced by sucralose to environmentally relevant concentrations in common carp (Cyprinus carpio). Sci Total Environ 2017;575:347-57.
41. Heredia-García G, Gómez-Oliván LM, Orozco-Hernández JM, et al. Alterations to DNA, apoptosis and oxidative damage induced by sucralose in blood cells of Cyprinus carpio. Sci Total Environ 2019;692:411-21.
42. Casas-Hinojosa I, Gómez-Oliván LM, Gutierrez-Noya VM, et al. Integrative approach to elucidate the embryological effects of caffeine in Cyprinus carpio: bioconcentration and alteration of oxidative stress-related gene expression patterns. Sci Total Environ 2023;894:165016.
43. Islas-Flores H, Gómez-Oliván LM, Galar-Martínez M, et al. Cyto-genotoxicity and oxidative stress in common carp (Cyprinus carpio) exposed to a mixture of ibuprofen and diclofenac. Environ Toxicol 2017;32:1637-50.
44. Cortes-Diaz MJA, Rodríguez-Flores J, Castañeda-Peñalvo G, et al. Sublethal effects induced by captopril on Cyprinus carpio as determined by oxidative stress biomarkers. Sci Total Environ 2017;605-6:811-23.
45. Luja-Mondragón M, Gómez-Oliván LM, SanJuan-Reyes N, et al. Alterations to embryonic development and teratogenic effects induced by a hospital effluent on Cyprinus carpio oocytes. Sci Total Environ 2019;660:751-64.
46. Morachis-Valdez G, Dublán-García O, López-Martínez LX, Galar-Martínez M, Saucedo-Vence K, Gómez-Oliván LM. Chronic exposure to pollutants in Madín Reservoir (Mexico) alters oxidative stress status and flesh quality in the common carp Cyprinus carpio. Environ Sci Pollut Res Int 2015;22:9159-72.
47. Liu G, Xiong YL. Electrophoretic pattern, thermal denaturation, and in vitro digestibility of oxidized myosin†. J Agric Food Chem 2000;48:624-30.
48. Liu C, Li W, Zhou M, et al. Effect of oxidation modification induced by peroxyl radicals on the physicochemical and gel characteristics of grass carp myofibrillar protein. Food Measure 2021;15:5572-83.
49. Moore DE. Drug-induced cutaneous photosensitivity: incidence, mechanism, prevention and management. Drug Saf 2002;25:345-72.
50. Albani A, Fasini E. Drugs, photochemistry and photostability. Cambridge: The Royal Society of Chemistry; 1998. cap. 1-4. Available from: https://www.semanticscholar.org/paper/Drugs%2C-photochemistry-and-photostability-Albini-Fasani/acee90616b776a627da4c86b68ac03abc19e3ebd. [Last accessed on 14 Sep 2023].
51. Luzi L, Pozza G. Glibenclamide: an old drug with a novel mechanism of action? Acta Diabetol 1997;34:239-44.
52. Domínguez R, Pateiro M, Gagaoua M, Barba FJ, Zhang W, Lorenzo JM. A comprehensive review on lipid oxidation in meat and meat products. Antioxidants 2019;8:429.
54. Xiong YL, Guo A. Animal and plant protein oxidation: chemical and functional property significance. Foods 2020;10:40.
55. Sante-Lhoutellier V, Aubry L, Gatellier P. Effect of oxidation on in vitro digestibility of skeletal muscle myofibrillar proteins. J Agric Food Chem 2007;55:5343-8.
56. Morzel M, Gatellier P, Sayd T, Renerre M, Laville E. Chemical oxidation decreases proteolytic susceptibility of skeletal muscle myofibrillar proteins. Meat Sci 2006;73:536-43.
57. Ma J, Wang X, Li Q, et al. Oxidation of myofibrillar protein and crosslinking behavior during processing of traditional air-dried yak
58. Qian S, Dou P, Wang J, et al. Effect of MTGase on silver carp myofibrillar protein gelation behavior after peroxidation induced by peroxyl radicals. Food Chem 2021;349:129066.
59. An Y, You J, Xiong S, Yin T. Short-term frozen storage enhances cross-linking that was induced by transglutaminase in surimi gels from silver carp (Hypophthalmichthys molitrix). Food Chem 2018;257:216-22.
60. Eymard S, Baron CP, Jacobsen C. Oxidation of lipid and protein in horse mackerel (Trachurus trachurus) mince and washed minces during processing and storage. Food Chemistry 2009;114:57-65.
61. Ahmad S, Khan H, Shahab U, et al. Protein oxidation: an overview of metabolism of sulphur containing amino acid, cysteine. Front Biosci (Schol Ed) 2017;9:71-87.
62. Ezraty B, Gennaris A, Barras F, Collet JF. Oxidative stress, protein damage and repair in bacteria. Nat Rev Microbiol 2017;15:385-96.
63. Zhou F, Zhao M, Zhao H, Sun W, Cui C. Effects of oxidative modification on gel properties of isolated porcine myofibrillar protein by peroxyl radicals. Meat Sci 2014;96:1432-9.
Cite This Article
Export citation file: BibTeX | EndNote | RIS
OAE Style
Martínez-Viveros EMG, Dublán-García O, Saucedo-Vence K, Morachis-Valdez AG, Cira-Chávez LA, Gómez-Oliván LM. Lipid and protein oxidation in Cyprinus carpio muscle by environmentally relevant concentrations of glibenclamide. Water Emerg Contam Nanoplastics 2023;2:19. http://dx.doi.org/10.20517/wecn.2023.17
AMA Style
Martínez-Viveros EMG, Dublán-García O, Saucedo-Vence K, Morachis-Valdez AG, Cira-Chávez LA, Gómez-Oliván LM. Lipid and protein oxidation in Cyprinus carpio muscle by environmentally relevant concentrations of glibenclamide. Water Emerging Contaminants & Nanoplastics. 2023; 2(4): 19. http://dx.doi.org/10.20517/wecn.2023.17
Chicago/Turabian Style
Ericka María Guadalupe Martínez-Viveros, Octavio Dublán-García, Karinne Saucedo-Vence, Ana Gabriela Morachis-Valdez, Luis Alberto Cira-Chávez, Leobardo Manuel Gómez-Oliván. 2023. "Lipid and protein oxidation in Cyprinus carpio muscle by environmentally relevant concentrations of glibenclamide" Water Emerging Contaminants & Nanoplastics. 2, no.4: 19. http://dx.doi.org/10.20517/wecn.2023.17
ACS Style
Martínez-Viveros, EMG.; Dublán-García O.; Saucedo-Vence K.; Morachis-Valdez AG.; Cira-Chávez LA.; Gómez-Oliván LM. Lipid and protein oxidation in Cyprinus carpio muscle by environmentally relevant concentrations of glibenclamide. Water. Emerg. Contam. Nanoplastics. 2023, 2, 19. http://dx.doi.org/10.20517/wecn.2023.17
About This Article
Copyright
Data & Comments
Data
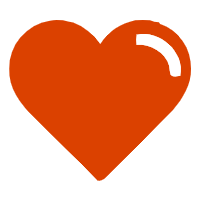
Comments
Comments must be written in English. Spam, offensive content, impersonation, and private information will not be permitted. If any comment is reported and identified as inappropriate content by OAE staff, the comment will be removed without notice. If you have any queries or need any help, please contact us at support@oaepublish.com.