Catalytic mechanism and biomedical applications of diatomic nanozymes
Abstract
The diatomic nanozymes (DANs) represent a class of nanomaterials containing dual metals as active centers with enzyme-like activity inspired by natural enzymes. They hold unique catalytic properties caused by their dual-atom structure, which have attracted significant attention. The catalytic mechanism of DANs may involve synergistic interactions between neighboring metal atoms and the regulation of electron arrangement near the active center, enhancing catalytic activity and specificity. The excellent catalytic activity and exceptional stability make DANs promising candidates for developing sensitive biosensors capable of precisely detecting disease markers. Furthermore, DANs show great promise as antitumor therapeutic agents, offering enhanced efficacy while minimizing side effects. This review outlines the catalytic mechanism and biomedical applications of DANs. The discussion section highlights the challenges and prospects in the development of DANs, offering insights for future research endeavors in this field.
Keywords
INTRODUCTION
Natural enzymes are often directly derived from various plants, animals, and microorganisms. They are important catalysts in living organisms. Their function is to lower the activation energy of chemical reactions, thereby significantly accelerating these reactions within living organisms[1-3]. The excellent biocompatibility and sustainability of natural enzymes contribute to indispensable tools in modern science and industry, bridging the gap between biology and technology. Especially, natural enzymes exhibit significant advantages in industrial applications such as the food industry, pharmaceuticals, biotechnology, and environmental protection due to their exceptional catalytic efficiency and outstanding selectivity[4-8]. Though natural enzymes hold some advantages in catalysis, there are still some intrinsic defects, such as sensitivity to environmental conditions (temperature and pH), high production cost and purification process, and limited stability and shelf-life, hindering their practical application[9].
Nanozymes, nanomaterials with intrinsic biological effects, have developed rapidly since first reported in 2007[10]. With the remarkable enzyme-like properties of nanozymes, the field of catalysis has been revolutionized. It is truly impressive that nanozymes have the ability to mimic natural enzymes while offering enhanced stability and tunable activity. This is because a single nanozyme typically contains multiple catalytic active centers, whereas the catalytic active center of natural enzymes is usually singular. To date, numerous nanomaterials, such as noble metals, metal oxides, carbon-based nanomaterials, and metal-organic frameworks (MOFs), have been found to perform enzyme-like catalytic activities[11-17]. These nanoscale materials hold significant promise for diverse applications, ranging from biomedical diagnostics to environmental remediation[18-23]. Their innovative design and exceptional performance highlight the exciting potential of nanotechnology in advancing science and technology.
Single-atom catalysts (SACs), an intriguing subset in the field of catalysis, have emerged with the advancements in nanotechnology, materials science, and catalytic chemistry. The active sites of SACs are typically derived from isolated metal centers, mostly transition metals, supported on various substrates, such as carbon-based materials, metal oxides, and polymers[24,25]. The SACs can maximize catalytic efficiency by fully utilizing the active sites of each metal atom. The atomic-level dispersion enhances the catalytic activity and provides a high degree of control over the catalytic process. Moreover, SACs hold the advantages of diverse, controllable and easily adjustable structures conducive to mimicking natural enzymes, suggesting that SACs could be promising candidates for bioinspired nanozymes[26,27]. Based on nanozymes, the emergence of single-atom nanozymes (SANs) has greatly increased the density of active centers, thereby further boosting catalytic performance[28-30]. In early research, the rise of SANs laid the foundation for diatomic design.
In factual, the catalytic centers of some natural enzymes, including Cu, Zn-Superoxide Dismutase (Cu, Zn-SOD)[31], nitrogenase[32-34], and cytochrome c oxidase (CcO)[35], contain two or more metals to achieve efficient catalytic conversion reactions through the synergistic interactions between the polynuclear metals. It is the multinucleated clusters that act as the active center in both of heterogeneous and homogeneous metal enzymes. Research shows that interactions among multi-metallic species, particularly bi-(poly)-nuclear metal species, significantly influence the catalytic process, which inspired the study of diatomic catalysts (DACs). In 2014, He et al. embedded the Fe DACs within graphene vacancies, which represents a milestone in the field of catalysis[36].
Diatomic catalysts or dual-metal-atom catalysts (DACs) are a growing area of enzyme-mimicking catalysis where adjacent metal atoms interact with each other, leading to higher catalytic activity compared to SACs. Meanwhile, DACs can be tailored to exhibit specific catalytic properties through bimetallic synergies. Thus, to simulate diverse and complex enzyme-like activities, DACs are better candidates as nanozymes for biomedical applications[37-39]. In 2024, Yang et al. made a distinction between the dual-atom and dual-single-atom catalysts, clearly stating that diatomic realization demonstrates the effect of “1 + 1 > 2”[40]. Figure 1 illustrates the structural differences between dual-atom and dual-single-atom catalysts. The term “Dual-atom nanozyme” or “Diatomic nanozymes” was initially used in 2020[41-43], which is a new chapter of diatomic nanozymes (DANs). Here, DANs are a class of nanomaterials that boast enzyme-like activity through synergistic interaction between contiguous metal active centers. DANs are characterized by their dual-atom structure, typically formed by neighboring metal atoms from the same or different elements. These paired metal atoms interact synergistically to create unique catalytic sites, enhancing catalytic activity and selectivity of the nanozyme. Besides, DANs usually possessed higher content of metal atoms; the metal content of some DANs was summarized in Table 1. Figure 2 showed developments from natural enzymes to dual-atom nanozymes (DANs). Combing the benefits of single-atom catalysis with the cooperative effects between the paired metal atoms makes DANs a promising and innovative milestone in the field of nano-catalysis.
Figure 1. Diagram of evolution from single-atom catalyst to diatomic catalyst. Ref.[40] Copyright 2024, Elsevier.
Overview of documented DANs for potential biomedical uses
DANs | Supports type | Mimic-function | Applications | Loading ratio in DANs | Increase of activity | Ref. |
Fe2NC | ZIF-8 derived N-doped/C | NADH-POD AA-POD | Biosensing | 0.38 wt% | 23.33 | Jiao et al.[37] |
Fe1Mn1-NCe | N-doped/C | POD-like OXD-like | Cancer treatment | Fe 1.16 wt% Mn 1.09 wt% | 16.64 | Zeng et al.[44] |
IC-DAN | ZIF-8 derived N-doped/C | POD-like | Antibacteria | Fe 2.84 % | 2.02-2.31 | Shi et al.[42] |
Zn/Mo DSAC-SMA | macroscope aerogel | POD-like | Biosensing | Zn 1.5 wt% Mo 7.3 wt% | over 100 | Ma et al.[45] |
FeBi-NC SAzyme | MOF | Oxidase-like | Degrade dyes | Fe 2.61 wt% Bi 8.01 wt% | 100 | Chen et al.[46] |
Fe2 DAC | ZIF-8 derived N-doped/C | Lipoxidase (LOX)-like POD-like | Antivirus | Fe 1.19 wt% | 6.5 | Li et al.[47] |
Cu/Zn NC | ZIF-8 derived N-doped/C | Laccase-like | Biosensing | Cu 0.11 wt% Zn 7.6 wt% | 3 | Li et al.[48] |
Fe2NC | ZIF-8 derived N-doped C | SOD-like CAT-like OXD-like | Protect against cerebral Ischemic reperfusion injury | Fe 0.38 wt% | Over 100 | Tian et al.[49] |
CuMn-DANs | MOF | CAT-like OXD-like | Cancer treatment | Cu 5.3 wt% Mn 4.1 wt% | 3.3 | Li et al.[50] |
FeCo-NC DANs | N-doped C | POD-like | Cancer treatment | - | 1.2-4.5 | Zeng et al.[51] |
FeCu DANs | Carbon support | POD-like GSH-OXD-like CAT-like | Cancer treatment | Fe 0.8% Cu 0.4% | 2.8 | Ning et al.[52] |
Despite the increasing number of studies on DACs and DANs, a comprehensive review focusing on the catalytic mechanisms and biomedical applications of DANs is currently lacking. Existing reviews primarily cover the design and characterization of DACs[40,54,55] or focus on electrocatalytic applications[39,56], rather than their enzyme-like functions and potential in biomedical fields. This review summarized the catalytic mechanisms underlying the enzyme-mimicking behavior of DANs, highlighted the biomedical applications of DANs in biosensing, antimicrobial therapy, and disease treatment, and discussed the challenges and future prospects to guide further research and application development. By focusing on both catalytic principles and biomedical relevance, this review offers a unique and timely contribution to the emerging field of DANs.
THE SYNTHETIC AND CATALYTIC MECHANISM OF DANs
The synthesis of DANs
The preparation methods of DANs are similar to those of DACs and involve various strategies to optimize the synthesis of highly active catalytic centers with two metal atoms, typically supported on carbon-based or other porous materials[57]. These methods generally aim to precisely control the coordination environment, atomic distribution, and electronic structure of the dual-metal sites. Some commonly used approaches for the synthesis of DANs include:
Atomically precise synthesis via atomic layer deposition (ALD): ALD is a powerful technique used to achieve precise control over the atomic layer-by-layer deposition of metals onto supports. In the case of dual-atom catalysts, ALD can be employed to deposit metal atoms onto a support material, followed by post-synthetic modification to achieve dual-atomic configurations[58]. This method ensures uniform distribution and precise placement of metal atoms at the atomic scale, which is essential for catalytic activity. However, this method has some limitations, such as high cost, complex equipment, and limited scalability for large-scale production.
Co-embedding of dual metals onto carbon-based supports: One widely used method is co-embedding dual metal atoms onto N-doped carbon or other porous carbon-based supports[59-61]. This method typically involves the use of metal precursors for the respective metals (e.g., Fe and Mn) followed by pyrolysis, which leads to the formation of dual-atom metal sites. The carbon support not only provides a stable platform for the metal atoms but also enhances the conductivity and overall catalytic activity. However, this method makes it difficult to precisely control atomic dispersion and coordination environment, leading to potential site heterogeneity.
Direct synthesis through molecular precursor methods: In this approach, molecular precursors containing the two metal atoms are directly synthesized and then incorporated into a support material[47,62]. The metal precursors can be designed to ensure that the two metals are in close proximity to each other, promoting the formation of dual-metal sites during the synthesis process. This method allows for precise control over the chemical bonding and electronic properties of the dual-atom sites. However, challenges include complex precursor design, potential metal aggregation during pyrolysis, and difficulties in achieving high metal loading while maintaining atomic dispersion.
These preparation techniques collectively contribute to the development of highly efficient DANs for various applications, including catalysis, sensing, and therapeutic purposes, especially in biomedical fields such as tumor therapy and inflammation treatment.
The catalytic mechanism of DANs
The catalytic mechanism of DANs is characterized by the synergistic interactions between the paired metal atoms within their dual-atom structure. These adjacent metal atoms create cooperative catalytic sites that facilitate enhanced catalytic activity and selectivity. The interaction between the two metal atoms can be achieved through direct metal-metal bonds or through interactions mediated by a supporting substrate or ligands.
In the initial attempts to develop bifunctional monoatomic nanozymes, an additional atom was introduced into SANs to enable cascade functions between the two metals. These nanozymes exhibited enhanced properties compared to SANs[63-66]. The two metal atoms were randomly distributed, lacking direct interaction with each other. Even so, DANs play an important role in the catalytic process.
Several advanced techniques are commonly used to study the catalytic mechanisms of DANs, providing insights into their electronic structures, charge distributions, and reaction pathways: Density functional theory (DFT) Calculations and X-ray absorption spectroscopy (XAS) are used to predict or analyze electronic structures, charge transfer, and adsorption energies, helping to understand reaction mechanisms at the atomic level. High-resolution transmission electron microscopy (HR-TEM) & Scanning transmission electron microscopy (STEM) were used to visualize the atomic dispersion of dual-metal sites and structural changes during catalysis. Gibbs Free Energy and Molecular dynamics (MD) Simulations were used to evaluate reaction thermodynamics, energy barriers, and the stability of catalytic sites under reaction conditions. Electron paramagnetic resonance (EPR), X-ray Photoelectron Spectroscopy (XPS) and Raman Spectroscopy were used as auxiliary tools to help analyze the catalytic mechanism.
Synergistic effect of the directed bimetallic active center with improved substrate affinity
A versatile CuMn DAN was created by Li et al., which was loaded with doxorubicin (DOX@CuMn-DANs) for synergistic trimodal therapy by simultaneously silencing P-gp and heat shock proteins [50]. They prepared CuMn-DANs by chemical precipitation method combined with pyrolysis under nitrogen, and further loaded doxorubicin (DOX) and obtained DOX@CuMn-DANs. The final nanozyme exhibits high antitumor efficacy because cascading of OXD-like and CAT-like activity. For further verification, CuCu-DANs and MnMn-DANs served as control materials. The catalytic mechanism of CuMn-DANs was analyzed. As depicted in Figure 3, Studies showed that Cu and Mn atoms formed Cu-N and Mn-N bonds, confirming their structure [Figure 3A-D]. Further analysis revealed that these atoms grouped into Cu2N6 and Mn2N6 units, enhancing their catalytic activity [Figure 3E and F]. Unlike some SACs where copper mainly exists as Cu+, CuMn-DANs had a nearly equal mix of Cu2+ and Cu+, influenced by the presence of manganese [Figure 3G][67]. In contrast, manganese in CuMn-DANs existed in +2, +3, and +4 oxidation states, with Mn3+ levels increasing compared to MnMn-DANs [Figure 3H][68,69]. This shift in oxidation states was due to a strong interaction between Cu+ and Mn4+ [Figure 3I], leading to the reaction, which plays a key role in the enhanced catalytic properties of CuMn-DANs:
Figure 3. (A-F) In CuMn-DANs, Fourier-transformed (FT) and Wavelet-transformed (WT) k3-weighted extended X-ray absorption fine structure (EXAFS) spectra of the Cu K-edge (A and C) and the Mn K-edge (B and D); FT of the k3-weighted EXAFS spectra and the corresponding fit in R space of Cu K-edge (E) and Mn K-edge (F). (G and H) The deconvoluted X-ray photoelectron spectroscopy (XPS) spectra of Cu 2p3/2 (G) and Mn 2p3/2 (H) for three DANs. (I) diagrammatic representation of the super-exchange interaction between Mn and Cu atoms in CuMn-DANs. Reproduced with permission from Ref.[50] Copyright 2023, Elsevier.
Some dual single-atomic nanozymes are also considered to be DANs according to the definition of DANs. Ma et al. used the non-covalent nano-assembly of polyoxometalates to create a peroxidase (POD)-like dual SAN made up of Zn and Mo[45]. with supramolecular coordination complexes as metal-atom precursors and macroscopic amphiphilic aerogels as the supporting substrate. Theoretical studies suggested that the adsorption energy of hydroxyl (Eads,OH) could serve as an indicator of the catalytic performance of POD-like nanozymes[73] [Figure 4A]. Eads,OH was calculated using the density functional theory (DFT) and result indicated that the dual Zn/Mo sites outperformed two isolated metal-atom sites. The reason was the Eads,OH (~3.01 eV) of the dual Zn/Mo sites was closer to the optimal value compared to the Zn SAC (~2.17 eV) and Mo SAC (~3.44 eV) sites [Figure 4B-D]. These results suggest that the synergistic interaction of Zn and Mo sites enhances the POD-like activity.
Figure 4. DFT calculation of the Zn/Mo DSAC-SMA. (A) Suggested reaction process for POD-like nanozymes. (B-D) Computational models and optimized hydroxyl adsorption configurations at the active sites of (B) Zn-SAC, (C) Mo-SAC, and (D) Zn/Mo DSAC-SMA. Reproduced with permission from Ref.[45] Copyright 2022, John Wiley and Sons.
Chen et al. developed FeBi-NC DANs with Fe-N4 and Bi-N4 active centers, supported by carbon from Fe-Bi bimetallic MOFs[46]. which contained 2.61 wt% Fe and 8.01 wt% Bi. Compared to single-metal Bi-NC and Fe-NC SAzymes, FeBi-NC SAzyme exhibited 9.8× and 5.9× higher oxidase-like activity, respectively. The synergistic effect between Fe and Bi played a crucial role, with Fe acting as both an active site and a spacer, preventing Bi atom clustering during preparation.
It is essential to develop a model that can be utilized to accurately mimic the bimetallic sites of natural enzymes. Jiao et al. synthesized Fe2NC nanozymes using Fe2 dimers encapsulated in ZIF-8, followed by calcination. Compared to Fe1NC, Fe2NC adsorbed oxygen differently, forming a peroxo-like structure with a longer O-O bond, which enhanced oxygen cleavage and catalytic activity [Figure 5A][53]. Charge analysis showed Fe2NC had a lower oxidation state, making it more effective in activating O2 [Figure 5B]. The projected density of states (PDOS) of oxygen adsorption state [Figure 5C], including the d-orbit centers of Fe and the p-orbit centers of O, demonstrated the strong adsorption of O2. Further calculations confirmed that Fe2NC followed an oxygen dissociation mechanism (ODM) instead of an adsorption evolution mechanism (AEM), improving oxygen reduction efficiency [Figure 5D and E]. As a result, Fe2NC facilitated a 4e-/4H+ oxygen reduction, unlike Fe1NC, which followed a less efficient 2e-/2H+ pathway. This distinction contributed to its superior enzyme-like activity.
Figure 5. The catalytic mechanism of Fe2NC. (A-D) On Fe1NC and Fe2NC nanozymes, (A) the structure diagram for adsorbed oxygen; (B) the charge density (yellow isosurfaces: the increase of 0.02 e/Å-3 for electronic density; blue isosurfaces: the decrease of 0.02 e/Å-3 for electronic density); (C) PDOS of the oxygen adsorption state, with the dashed line indicating the Fermi level and the solid lines representing the d-band centers of Fe and the p-band centers of O for each sample. (D) The free energy diagram for oxygen reduction pathways via AEM and ODM. (E) the diagram illustration of O2 reduction process, with AEM shown on the left and ODM on the right. Reproduced with permission from Ref.[53] Copyright 2021, Springer Nature.
The natural lipoxidase (LOX) is characterized by its ability to catalyze the cleavage of ester bonds in triglycerides, phospholipids, and other lipid substrates, and thus possesses antivirus ability to envelope viruses. However, the limited interaction between LOX and the viral envelope, along with the complexity of the substrate being catalyzed, results in the suboptimal antiviral performance of LOX[74,75]. Li et al. first reported the LOX-like activity of Fe2 DACs[47], which were prepared through an encapsulation-pyrolysis approach mediated by macrocyclic precursor[62], and showed versatile enzyme-like catalytic activity. Among them, the Fe2 DAC showed superior LOX-like activity and killed enveloped viruses with high efficiency. To calculate the LOX-like catalytic activity, they chose the Fe2N4O2 model and the cis, cis-1,4-pentadiene moiety to serve as active centers and model substrates, respectively. The mechanism of the LOX-like activity for Fe2 DAC nanozyme is elucidated through the established four-step mechanism [Figure 6A]. The feasibility of the catalytic cycle had been verified by the calculation of Gibbs free energy [Figure 6B]. In addition, to clarify the factors of substrate activation, the densities of states (DOS) for the cis, cis-1,4-pentadiene moiety, Fe2N4O2 model, and their interaction configuration were calculated. They found the energy levels of β-spin d orbitals for Fe2 minority were well matched with π* orbitals of pentadiene moiety, and the energy levels of α-spin d orbitals for Fe2 minority were well-aligned with π orbitals of pentadiene moiety through the electronic structure analysis [Figure 6C]. The two factors could significantly reduce the bonding strength within the pentadiene moiety, leading to substrate activation. In contrast, Fe SACs did not adhere to this principle, resulting in reduced LOX-like activity and lower antiviral efficacy, which indicate the importance of cooperation between diatoms.
Figure 6. (A) the suggested reaction process for LOX-like activity, including the peroxidatic reaction of cis, cis-1,4-pentadiene moiety with Fe2N4O2 model (unit: Å). (B) Gibbs free energy profile of crucial intermediates and transition states during the LOX-like catalytic chain. Energy unit: eV. (C) pDOS for cis, cis-1,4-pentadiene moiety, Fe2 DAC structure, and their interaction configuration. The species adsorbed on Fe2 DAC was signed by asterisk (*). Reproduced with permission from Ref.[47] Copyright 2023, Springer Nature.
As confirmed, active centers of natural laccase are multiple Cu-N ligand structures. Inspired by this, Li et al. demonstrated CuZn-N/C DANs. Through experiments, they found that the ligand structure was acted as an efficient laccase mimic and further used to catalyze oxidation of epinephrine (EP)[48]. To uncover catalytic mechanism of CuZn-N/C DANs for their excellent performance, the calculation of DFT was conducted. Results were as follows: (1) CuZn-N/C possessed proper distance between Cu and Zn atoms, which was different from Cu-N/C or Zn-N/C and optimal for adsorbing the EP molecule [Figure 7A-F]. Additionally, in the CuZn-N/C, the existence of another hydrogen bond could contribute to stability of EP molecular adsorption [Figure 7G-I]; (2) Analyses of molecular orbitals and DOS show that the strong effect on the EP molecule and the active metal sites of CuZn-N/C was crucial for the catalytic oxidization of EP molecule
Figure 7. (A-C) Structure schematic of Cu-N/C (A), Zn-N/C (B), and CuZn-N/C (C). (D-F) Models of EP molecule adsorbed onto Cu-N/C (D), Zn-N/C (E), and CuZn-N/C (F). (G-I) the highest occupied molecular orbitals of (G) CuN4, (H) ZnN4, and (I) CuZn-N/C with the adsorption of EP molecule. (J-L) pDOS of CuN4 (J), ZnN4 (K), and CuZn-N/C (L) with the adsorption of EP molecule. Reproduced with permission from Ref.[48] Copyright 2023, American Chemical Society.
Shi et al. constructed an interlayer coupling DAN (IC-DAN) through atomic-level engineering to modulate the coordination environment[42]. Synchrotron radiation photoelectron spectroscopy indicated that the IC-DAN formed N4-Fe-Fe-N4 active structures, which significantly improved the catalytic performance. DFT calculation indicated stronger interaction and adsorption between FeN4-FeN4 DAN, with enhanced charge transfer throughout the POD catalysis process. The root cause of the synergistic effect between the courtyards was further explained from the structure and electron density of the active centers was further explained by Zeng et al., who introduced a novel approach to fine-tune the d-band center of dual-atomic sites on N-doped porous carbon dual-atomic sites Fe-Mn (Fe1Mn1-NCe), enhancing significantly boosted multiple-enzyme-like catalytic activities[44]. DFT calculations revealed that introducing Mn shifted the d-band center closer to the Fermi level (-0.564 vs. -1.113 eV for Fe1-NCe), improving substrate interactions and catalytic efficiency [Figure 8A and B]. Charge analysis confirmed that dual-atomic-site interactions modulate electronic structure of Fe, increasing intermediate affinity [Figure 8C-F]. Gibbs free energy calculations showed Fe1Mn1-NCe had stronger H2O2 adsorption, facilitating H2O* formation and desorption with lower energy (2.67 eV vs. 2.94 Ev, Figure 8G and H). Additionally, Fe1Mn1-NCe exhibited stronger O2 adsorption, weakening O-O bonds[76], and enhancing OOH* cleavage, boosting its enzyme-like activity.
Figure 8. (A and B) DOS of (A) Fe1-NCe and (B) Fe1Mn1-NCe. (C) Schematic illustration of the upshift of the d-band center by a synergistic interaction between metal atoms. (D-F) Differential charge density of (D) Fe1Mn1-NCe and H2O2 dissociated to 2OH* in (E) Fe1-NCe and (F) Fe1Mn1-NCe (the isosurface level is 0.002 e/Å3). Yellow and blue regions indicate areas where electrons are gained and lost, respectively. (G and H) Free-energy diagrams illustrating the POD-like (G) and OXD-like (H) mechanisms on Fe1-NCe and Fe1Mn1-NCe in an acidic environment (inset: optimized geometries of intermediates). Reprinted with permission from Ref.[44], Copyright 2024, American Chemical Society.
Besides, Some DACs can also serve as promising candidates for DANs. Lv et al. employed the first principles high-throughput screening and discovered that Fe-Fe sites on graphite carbon nitride modulate the binding strength of reaction species, such as N2H and NH2[77]. This approach achieved the highest nitrogen reduction reaction performance among 23 transition metals. The DACs attained high theoretical Faradaic efficiency and accelerated catalyst discovery for ammonia synthesis; thus, it was speculated that these DACs might have some promise in ammonia synthesis simulating the activities of enzymes related to ammonia synthesis, such as nitrogenase.
In a word, when substrate molecules approach the catalytic sites of DANs, the paired metal atoms work in tandem to activate the substrate, lowering the activation energy of the reaction. This cooperative effect allows for efficient conversion of reactants into products with improved efficiency and specificity. Furthermore, the electronic configuration and spatial structure of metal atom pairs are crucial in defining the catalytic properties of DANs. The electronic configuration influences the redox potentials and binding affinities, while the geometric arrangement dictates the accessibility and orientation of the catalytic sites of substrate molecules. Overall, the unique dual-atom structure of DANs enables a multifaceted catalytic mechanism that combines the advantages of single-atom catalysis with cooperative interactions between the paired metal atoms. This synergistic approach provides DANs with enhanced performance and versatility in various catalytic applications. However, the catalytic mechanism has not been elucidated[49,78]. With the continuous expansion of the research field of nanozymes, more and more DANs have been developed, their properties will be improved, and the catalytic mechanism will be more comprehensive and clear.
THE BIOMEDICAL APPLICATION OF DANs
The catalytic mechanisms of DANs are intrinsically linked to their biomedical applications, as their enzyme-mimicking activities directly influence therapeutic efficacy. For instance, DANs with POD-like activity generate reactive oxygen species (ROS) to induce oxidative stress, making them highly effective for tumor therapy via ferroptosis. Similarly, DANs exhibiting CAT-like activity efficiently decompose hydrogen peroxide into oxygen and water, alleviating oxidative stress in inflammation-related diseases. Moreover, their OXD-like activity enables antibacterial applications by promoting bacterial membrane disruption through oxidative damage. The precise tuning of dual-metal active sites not only enhances catalytic efficiency but also tailors their function for specific biomedical needs, demonstrating the strong interplay between fundamental catalytic mechanisms and therapeutic applications.
DANs have promising applications in biomedicine, particularly in areas of diagnostics and therapeutics. Their enhanced catalytic activity and stability make them ideal candidates for developing sensitive biosensors for disease markers. Furthermore, DANs have a place in the bioimaging, assisting in the detection and monitoring of diseases at the molecular level. Overall, the biomedical potential of DANs highlights their significance in advancing healthcare technologies.
DANs used for biosensing
DANs offer promising potential in biosensing due to their enhanced catalytic activity and stability although their development is still in infancy. Their unique properties could enable the development of sensitive biosensors capable of detecting disease markers with high precision, advancing early diagnosis and treatment monitoring in healthcare[79]. Li et al. utilized the laccase-like activity of CuZn-N/C, combined with an online optical detection platform (OODP), to construct a highly selective and sensitive system for EP detection [Figure 9A][48]. This system was also used to continuously monitor EP in brain microdialysis samples [Figure 9B-G]. The detection ability was comparable to previously reported methods[35].
Figure 9. (A) Schematic illustration of using CuZn-N/C for in vivo microdialysis and online optical detection platform (OODP) to measure EP. (B) Time-dependent changes in light intensity reflecting the EP levels. (C) Comparison of the Km of CuZn-N/C and LOD of OODP in different studies. (D and E), Selectivity (D) and stability (E) detection of EP using CuZn-N/C in the OODP. (F and G), Continuous monitoring of striatal EP levels before and after ischemia in the living rat brain using OODP, where (F) represents the saline group and (G) represents the drug administration group. Reprinted with permission from Ref.[48], Copyright 2023, American Chemical Society.
Jiao et al. used the Fe2 NC to detect S- in water for Proof of concept, achieving a broad detection range of
DANs used for antimicrobial
DANs have demonstrated significant potential in antimicrobial applications due to their intrinsic enzyme-mimicking activities. Their OXD-like, POD-like, and SOD-like catalytic properties enable the generation of ROS, which effectively disrupt bacterial membranes, damage intracellular biomolecules, and ultimately lead to microbial cell death.
Additionally, the dual-metal synergy in DANs enhances their catalytic efficiency, allowing for lower concentrations to achieve potent antimicrobial effects while minimizing cytotoxicity to mammalian cells. Moreover, DANs can combat antibiotic-resistant bacteria, such as methicillin-resistant Staphylococcus aureus (MRSA), by bypassing conventional resistance mechanisms and triggering oxidative stress-induced bacterial apoptosis. Shi et al. constructed an IC-DAN, which exhibits higher POD-like activity than Fe SANs in the presence of H2O2 because of the formation of Nx-Fe-Fe-Nx, generating a large amount of hydroxyl radicals and demonstrating high photothermal conversion capability[42]. They created MRSA-infected wounds on the epidermis of both normal and type 2 diabetic mice [Figure 10A and B], and the results showed that the nanozyme exhibited efficient healing effects in both models [Figure 10C-F].
Figure 10. (A) Treatment plan using IC-DAN for normal mouse models infected with MRSA. (B) Treatment plan for type 2 diabetic mouse models infected with MRSA. (C) Images of the wound sites in normal mice infected with MRSA. Scale bar: 5 mm. (D) Images showing wound healing in type 2 diabetic mouse models after MRSA infection treatment. (E and F) Relative healing area in normal mice infected with MRSA (E) and type 2 diabetes mice (F) (n = 5). *P < 0.0001, **P < 0.001, ***P < 0.01, ****P < 0.05. Reproduced with permission from Ref.[42], Copyright 2024, John Wiley and Sons.
Furthermore, surface modifications and hybrid nanoplatforms incorporating DANs have been explored to enhance their biocompatibility, stability, and targeted antimicrobial efficacy. These advances position DANs as promising candidates for next-generation antibacterial agents in biomedical applications, including wound healing, infection control, and antiviral effects. Li et al. used the prepared Fe2 DAC to effectively eliminate the capsular virus, which has great application potential in fresh air systems and cold chain transportation[47]. The presence of dual-atomic active sites uniquely endows Fe2 DAC with LOX enzyme activity, a capability unattainable by SANs.
DANs used for biological degradation
DANs demonstrate excellent potential for biological degradation by catalyzing the breakdown of harmful biomolecules and environmental pollutants through enzyme-mimetic reactions. Their enhanced catalytic activity enables efficient degradation of organic contaminants, toxins, and bacterial biofilms, contributing to biomedical and environmental applications. Liu et al. established a cascade enzyme-nanozyme platform by combining AChE with FeBi-NC for detecting AChE activity, achieving a LOD of 1 × 10-4 mU mL-1[18]. In the FeBi-NC, Bi-N4 and Fe-N4 exhibit strong electron-donating and binding energy capabilities, enhancing peroxymonosulfate activation and generating highly active intermediates. Through peroxymonosulfate activation by FeBi-NC, rhodamine B is completely degraded within five minutes. Importantly, we speculate that the prepared FeBi-NC material may also be used to degrade other dyes containing benzene rings, which have broad application prospects in the biomedical.
DANs used for tumor/inflammation therapy
DANs exhibit potential anti-inflammatory properties by mimicking natural antioxidant enzymes to effectively scavenge excessive ROS and reduce oxidative stress. Additionally, DANs can modulate immune responses by inhibiting pro-inflammatory cytokine production and suppressing NLRP3 inflammasome activation, thereby alleviating inflammation-related diseases[61,78,82].
Wang et al. verified the potential of DANs in anti-inflammatory action using Caenorhabditis elegans Parkinson’s disease models[83]. This was attributed to the enhanced catalase-like catalytic activity of DANs. Tian et al. developed a multi-enzyme system by embedding a Fe2NC within a selenium-containing MOF (Se-MOF) shell for cascade antioxidants[49]. Compared to the Fe1NC nanozyme, the designed Fe2NC nanozyme exhibited superior redox enzyme-like activities. Additionally, the Se-MOF shell significantly enhanced the biocompatibility of Fe2NC nanozyme because of the mimicked glutathione POD (GPx) activity. DFT calculations revealed the synergistic effect of the Fe-Fe atoms in Fe2NC is the key reason for its enhanced activity. Further mechanistic studies demonstrated that this multifunctional system can eliminate intracellular ROS and may inhibit the signaling pathway of ASK1/JNK apoptotic, thereby effectively reducing oxidative damage and alleviating neuronal apoptosis after cerebral ischemia reperfusion injury.
Liu et al. developed a nanoplatform comprising phospholipase A2 (PLA2), lipoxygenase (LOX), and a FeCo DANs/PL[84]. This system induces two forms of immunogenic tumor ferroptosis. Firstly, because of the multi-enzyme mimetic activities, it can induce the initiating immunogenic tumor ferroptosis; secondly, it can induce ACSL4-mediated immunogenic tumor ferroptosis. In the second form, FeCo DAN/PL generates ROS and depletes GSH and GPX4, thereby promoting lipid peroxidation (LPO) at tumor sites. Additionally, under interferon-gamma stimulation, ACSL4 is activated, converting free AA released from PLA2 catalysis process into arachidonyl-CoA. Then, the converted arachidonyl-CoA was further integrated into membrane phospholipids and undergoes peroxidation catalyzed by LOX, ultimately inducing irreversible cascade immunogenic ferroptosis. This approach provides an effective pathway to immunotherapies [Figure 11].
Figure 11. Diagram illustrating the preparation process and therapeutic mechanism of the FeCo/Fe-Co DAN/PL. Reproduced with permission of Ref.[84], Copyright 2023, American Chemical Society.
Zeng et al. developed Fe1Mn1-NC DANs, which exhibit excellent enzyme-like catalytic activity and strong photothermal performance[44]. In vivo experiments demonstrated that these DANs achieved an impressive tumor inhibition efficiency of 95.74%. Ning et al. developed FeCu DANs with glutathione-oxidase (GSH-OXD)-like, POD-like and catalase-like activity, enabling cascade catalysis to maintain the generation of ·OH and eliminate ROS continuously[52]. They utilized the FeCu DANs combined with photothermal therapy for tumor treatment, achieving a tumor inhibition rate as high as 98.4%, the highest inhibition rate reported to date.
In summary, DANs have demonstrated significant potential in tumor and inflammation therapy by leveraging their superior enzyme-mimetic activities. From alleviating oxidative stress in inflammatory diseases such as dry eye disease and cerebral ischemia-reperfusion injury to inducing ferroptosis in tumor cells, DAN-based systems exhibit remarkable catalytic efficiency and biocompatibility. Their ability to modulate ROS, regulate apoptotic pathways, and enhance immunogenic responses positions them as promising candidates for next-generation therapeutic strategies. These advancements underscore the versatility of DANs in biomedical applications, paving the way for more effective and targeted treatments.
BIOLOGICAL SAFETY OF DANS
Biological safety assessment is crucial for the clinical translation of DANs. Comprehensive evaluations, including cytotoxicity, hemocompatibility, immunogenicity, and long-term biodistribution studies, are essential to ensure their biocompatibility. Despite their promising catalytic activities, similar to other nanozymes, there are several challenges in biomedical applications, such as potential cytotoxicity and oxidative stress, hemocompatibility and immunogenicity, and long-term biodistribution and clearance, which directly affect the transformation and application of DANs. Here are strategies to enhance the biological safety of DANs.
Surface modification and functionalization: Coating DANs with biocompatible polymers, such as polyethylene glycol or lipid-based encapsulation[50,78,85], can reduce cytotoxicity and improve blood circulation. Besides, zwitterionic modifications can prevent protein adsorption, reducing immune recognition and inflammatory responses.
Optimizing composition and dosage: Selecting biodegradable or bioresorbable materials[52,86] can facilitate natural clearance and minimize toxicity. Dose-dependent studies and controlled ROS generation strategies can prevent oxidative damage while maintaining therapeutic efficacy.
Enhancing clearance pathways: Engineering DANs with renal-clearable designs such as ultrasmall nanoparticles < 5 nm promotes rapid excretion via the kidneys, reducing long-term toxicity.
Comprehensive in vivo toxicity assessments: In animal models to evaluate biodistribution, metabolism, and excretion pathways[52], multi-omics approaches can provide a deeper understanding of cellular responses to DAN exposure.
By addressing these challenges through rational design, surface engineering, and rigorous biological evaluations, DANs can be optimized for safe and effective biomedical applications.
CONCLUSION AND PERSPECTIVE
In conclusion, DANs have emerged as a captivating category of nanomaterials, known for their versatile catalytic properties and potential in biomedical applications. Their unique dual-atom structure and synergistic interactions are beneficial to enhancing catalytic activity and specificity. The catalytic mechanism of DANs involves the regulation of electron arrangement near the active center, contributing to their remarkable performance across diverse catalytic processes.
In the biomedical field, DANs hold tremendous potential for applications, such as biosensing, disease diagnosis and therapeutics. Their enhanced catalytic activity and stability make them ideal candidates for developing sensitive biosensors. Moreover, DANs offer opportunities for prevention and treatment of microbial infections. Despite the promising prospects, the development and application of DANs still face several challenges. One major challenge is the synthesis of DANs with precisely controlled structures and compositions, as well as scalable production methods. The present results show that, the preparation of DANs from diatomic precursors may be easier to obtain higher yield than the direct preparation of DANs by one-pot method. In addition, understanding the complex catalytic mechanism and optimizing the performance of DANs for specific biomedical applications are still worthy of further research.
(1) Advanced synthetic techniques: The future of DANs lies in the advancement of synthetic techniques. Developing precise, scalable, and cost-effective methods for synthesizing DANs with controlled structures and compositions is essential. Innovations in chemical vapor deposition, ALD, could lead to more efficient production methods. Moreover, exploring green synthesis routes could reduce environmental impact and enhance the sustainability of DAN production. Utilizing emerging technologies, such as data-driven material screening, machine learning-guided predictive synthesis optimization, real-time process monitoring, real-time process monitoring and automated high-throughput experimentation, will lead to more efficient, scalable, and environmentally friendly production methods. These innovations will unlock new opportunities for next-generation biomedical treatments, energy conversion technologies, and environmental remediation solutions.
Data-driven material screening: AI models analyze vast datasets of metal combinations, coordination environments, and support materials to predict optimal dual-atom configurations for enhanced catalytic activity.
Predictive synthesis optimization: Machine learning algorithms process experimental and computational data to determine ideal precursor selection, temperature control, and synthesis pathways, reducing trial-and-error experiments.
Real-time process monitoring: AI-integrated spectroscopy and imaging techniques (e.g., XAS, STEM, energy-dispersive X-ray spectroscopy) provide feedback on atomic dispersion and stability, allowing dynamic adjustments to synthesis conditions.
Theoretical and computational simulations: DFT and MD simulations, coupled with big data analysis, help refine electronic structures, reaction mechanisms, and catalytic performance predictions.
Automated high-throughput experimentation: AI-driven robotic platforms execute and analyze multiple synthesis trials simultaneously, accelerating the discovery of high-performance DANs with optimized structures and functions.
(2) In-depth mechanistic studies: Further elucidation of the catalytic mechanisms of DANs is crucial. Advanced characterization techniques, such as synchrotron radiation, operando spectroscopy and cryo-electron microscopy, can provide real-time insights into the behavior of DANs under reaction conditions. Coupled with computational modeling and machine learning approaches, these studies can unravel the precise roles of each metal atom and their interactions, guiding the rational design of more efficient nanozymes.
(3) Integration with other technologies: Integrating DANs with other emerging technologies can open new avenues for biomedical applications. For instance, combining DANs with nanorobots or microfluidic devices can enhance targeted drug delivery and diagnostic precision. Additionally, leveraging DANs in conjunction with gene editing tools such as the clustered regularly interspaced short palindromic repeats could lead to innovative therapeutic strategies for genetic disorders.
(4) Interdisciplinary collaborations: Interdisciplinary collaborations among nanotechnology, catalysis, and biomedicine will drive innovation and accelerate the translation of DANs into practical biomedical solutions. Collaborative efforts can foster the development of multifunctional DANs that combine catalytic activity with other therapeutic modalities, such as photothermal or photodynamic therapy, for enhanced treatment efficacy.
(5) Regulatory and safety considerations: As DANs move closer to clinical applications, addressing regulatory and safety considerations becomes paramount. To confirm the safety and efficacy of DANs in medical applications, comprehensive biocompatibility evaluation, long-term toxicity assessments, and clinical trials are required. Establishing standardized protocols for evaluating and reporting the performance of DANs will facilitate their acceptance and integration into existing healthcare systems.
(6) Commercialization and industrial application: To realize the full potential of DANs, the transfer of laboratory research to commercial applications is one of the directions of future efforts. Collaborations with industry partners can expedite the development of DAN-based products and facilitate their entry into the market. Additionally, exploring applications beyond biomedicine, such as environmental remediation and energy conversion, can expand the impact of DANs across various sectors.
Overall, the development of DANs is still in its infancy, although diatomic catalysts have been available since 2014. However, the advancement of DANs marks a paradigm shift in nanozyme research, offering unprecedented catalytic performance, versatility, and application potential across multiple scientific and technological fields. It should not be ignored that, although different from the definition of the new DANs, Dual-single atom nanozyme also played an indispensable role in the development of DANs. The future of DANs holds exciting possibilities; continuous development of DANs with better performance, further exploration of their catalytic mechanism, and promotion of their future biomedical applications are key research directions. Realizing these visions positions DANs as promising candidates for revolutionizing medical technologies and meeting diverse medical needs.
DECLARATIONS
Authors’ contributions
Conceptualization, investigation, and writing-original draft: Wang, X.; Hou, L.; Dang, M.
Editing and supervision: Li, B.; Wu, J.
Writing-review & editing, supervision, and funding acquisition: Li, H.; Li, B.; Wu, J.; Gao, L.
Availability of data and materials
Not applicable.
Financial support and sponsorship
This work was supported by the National Key R&D Program of China (2019YFA0709200), National Natural Science Foundation of China Foundation of Innovative Research Group (22121003), National Natural Science Foundation of China (81930050, 22401076), the China Postdoctoral Science Foundation (2023M740999, 2021M691759, and 2021TQ0169), Beijing Natural Science Foundation (2224103), Science Foundation of State Key Laboratory of Structural Chemistry (20240017), Program for Guangdong Introducing Innovative and Entrepreneurial Teams (2019ZT08L101), University stability support program of Shenzhen (2023SC0027), Shenzhen Science and Technology Innovation Commission (JSGGKQTD20221101115701006), and Henan Provincial Natural Science Foundation youth project (242300420537).
Conflicts of interest
All authors declared that there are no conflicts of interest.
Ethical approval and consent to participate
Not applicable.
Consent for publication
Not applicable.
Copyright
© The Author(s) 2025.
REFERENCES
2. Garcia-Viloca, M.; Gao, J.; Karplus, M.; Truhlar, D. G. How enzymes work: analysis by modern rate theory and computer simulations. Science 2004, 303, 186-95.
3. Benini, S.; Rypniewski, W. R.; Wilson, K. S.; Miletti, S.; Ciurli, S.; Mangani, S. A new proposal for urease mechanism based on the crystal structures of the native and inhibited enzyme from Bacillus pasteurii: why urea hydrolysis costs two nickels. Structure 1999, 7, 205-16.
4. Lin, Y.; Ren, J.; Qu, X. Catalytically active nanomaterials: a promising candidate for artificial enzymes. ACC. Chem. Res. 2014, 47, 1097-105.
5. Planas-Iglesias, J.; Marques, S. M.; Pinto, G. P.; et al. Computational design of enzymes for biotechnological applications. Biotechnol. Adv. 2021, 47, 107696.
6. Shahidi, F.; Janak Kamil, Y. V. A. Enzymes from fish and aquatic invertebrates and their application in the food industry. Trends. Food. Sci. Technol. 2001, 12, 435-64.
7. Kelly, S. A.; Pohle, S.; Wharry, S.; et al. Application of ω-transaminases in the pharmaceutical industry. Chem. Rev. 2018, 118, 349-67.
8. Lin, Y. W. Biodegradation of aromatic pollutants by metalloenzymes: a structural-functional-environmental perspective. Coord. Chem. Rev. 2021, 434, 213774.
9. Pinney, M. M.; Mokhtari, D. A.; Akiva, E.; et al. Parallel molecular mechanisms for enzyme temperature adaptation. Science 2021, 371, eaay2784.
10. Gao, L.; Zhuang, J.; Nie, L.; et al. Intrinsic peroxidase-like activity of ferromagnetic nanoparticles. Nat. Nanotechnol. 2007, 2, 577-83.
11. Liu, M.; Wang, L.; Zhao, K.; et al. Atomically dispersed metal catalysts for the oxygen reduction reaction: synthesis, characterization, reaction mechanisms and electrochemical energy applications. Energy. Environ. Sci. 2019, 12, 2890-923.
12. Huo, M.; Wang, L.; Wang, Y.; Chen, Y.; Shi, J. Nanocatalytic tumor therapy by single-atom catalysts. ACS. Nano. 2019, 13, 2643-53.
13. Hu, F. X.; Hu, T.; Chen, S.; et al. Single-atom cobalt-based electrochemical biomimetic uric acid sensor with wide linear range and ultralow detection limit. Nanomicro. Lett. 2020, 13, 7.
14. Wang, D.; Wu, H.; Phua, S. Z. F.; et al. Self-assembled single-atom nanozyme for enhanced photodynamic therapy treatment of tumor. Nat. Commun. 2020, 11, 357.
15. Cao, F.; Sang, Y.; Liu, C.; et al. Self-adaptive single-atom catalyst boosting selective ferroptosis in tumor cells. ACS. Nano. 2022, 16, 855-68.
16. Fan, K.; Xi, J.; Fan, L.; et al. In vivo guiding nitrogen-doped carbon nanozyme for tumor catalytic therapy. Nat. Commun. 2018, 9, 1440.
17. Lyu, Z.; Ding, S.; Wang, M.; et al. Iron-imprinted single-atomic site catalyst-based nanoprobe for detection of hydrogen peroxide in living cells. Nanomicro. Lett. 2021, 13, 146.
18. Liu, Y.; Ding, D.; Zhen, Y.; Guo, R. Amino acid-mediated 'turn-off/turn-on' nanozyme activity of gold nanoclusters for sensitive and selective detection of copper ions and histidine. Biosens. Bioelectron. 2017, 92, 140-6.
19. Karim, M. N.; Anderson, S. R.; Singh, S.; Ramanathan, R.; Bansal, V. Nanostructured silver fabric as a free-standing NanoZyme for colorimetric detection of glucose in urine. Biosens. Bioelectron. 2018, 110, 8-15.
20. Wu, J.; Zhou, H.; Li, Q.; et al. Densely populated isolated single Co-N site for efficient oxygen electrocatalysis. Adv. Energy. Mater. 2019, 9, 1900149.
21. Zhang, J.; Huang, Q. A.; Wang, J.; Wang, J.; Zhang, J.; Zhao, Y. Supported dual-atom catalysts: preparation, characterization, and potential applications. Chin. J. Catal. 2020, 41, 783-98.
22. Zhang, W.; Fu, Q.; Luo, Q.; Sheng, L.; Yang, J. Understanding single-atom catalysis in view of theory. JACS. Au. 2021, 1, 2130-45.
23. Li, Z.; Ji, S.; Liu, Y.; et al. Well-defined materials for heterogeneous catalysis: from nanoparticles to isolated single-atom sites. Chem. Rev. 2020, 120, 623-82.
24. Zhao, X.; He, D.; Xia, B. Y.; Sun, Y.; You, B. Ambient electrosynthesis toward single-atom sites for electrocatalytic green hydrogen cycling. Adv. Mater. 2023, 35, e2210703.
25. Singh, B.; Gawande, M. B.; Kute, A. D.; et al. Single-atom (iron-based) catalysts: synthesis and applications. Chem. Rev. 2021, 121, 13620-97.
26. Xiang, H.; Feng, W.; Chen, Y. Single-atom catalysts in catalytic biomedicine. Adv. Mater. 2020, 32, e1905994.
27. Wu, J.; Zhu, X.; Li, Q.; et al. Enhancing radiation-resistance and peroxidase-like activity of single-atom copper nanozyme via local coordination manipulation. Nat. Commun. 2024, 15, 6174.
28. Yang, X. F.; Wang, A.; Qiao, B.; Li, J.; Liu, J.; Zhang, T. Single-atom catalysts: a new frontier in heterogeneous catalysis. ACC. Chem. Res. 2013, 46, 1740-8.
29. Jiang, B.; Guo, Z.; Liang, M. Recent progress in single-atom nanozymes research. Nano. Res. 2023, 16, 1878-89.
30. Chang, Q.; Wu, J.; Zhang, R.; et al. Optimizing single-atom cerium nanozyme activity to function in a sequential catalytic system for colorimetric biosensing. Nano. Today. 2024, 56, 102236.
31. Lumsden, J.; Hall, D. O. Superoxide dismutase in photosynthetic organisms provides an evolutionary hypothesis. Nature 1975, 257, 670-2.
32. Seefeldt, L. C.; Hoffman, B. M.; Peters, J. W.; et al. Energy transduction in nitrogenase. ACC. Chem. Res. 2018, 51, 2179-86.
34. Van, S. C.; Decamps, L.; Cutsail, G. E.; et al. The spectroscopy of nitrogenases. Chem. Rev. 2020, 120, 5005-81.
35. Wikström, M.; Krab, K.; Sharma, V. Oxygen activation and energy conservation by cytochrome c oxidase. Chem. Rev. 2018, 118, 2469-90.
36. He, Z.; He, K.; Robertson, A. W.; et al. Atomic structure and dynamics of metal dopant pairs in graphene. Nano. Lett. 2014, 14, 3766-72.
37. Ye, W.; Chen, S.; Lin, Y.; et al. Precisely tuning the number of Fe atoms in clusters on N-doped carbon toward acidic oxygen reduction reaction. Chem 2019, 5, 2865-78.
38. Zhang, N.; Zhou, T.; Ge, J.; et al. High-density planar-like Fe2N6 structure catalyzes efficient oxygen reduction. Matter 2020, 3, 509-21.
39. Zhang, S.; Wu, Y.; Zhang, Y. X.; Niu, Z. Dual-atom catalysts: controllable synthesis and electrocatalytic applications. Sci. China. Chem. 2021, 64, 1908-22.
40. Yang, X.; Xu, L.; Li, Y. Do we achieve “1 + 1 > 2” in dual-atom or dual-single-atom catalysts? Coord. Chem. Rev. 2024, 516, 215961.
41. Du, C.; Gao, Y.; Chen, H.; et al. A Cu and Fe dual-atom nanozyme mimicking cytochrome c oxidase to boost the oxygen reduction reaction. J. Mater. Chem. A. 2020, 8, 16994-7001.
42. Shi, X.; Lv, J.; Deng, S.; et al. Construction of interlayer coupling diatomic nanozyme with peroxidase-like and photothermal activities for efficient synergistic antibacteria. Adv. Sci. 2024, 11, e2305823.
43. Wang, S.; Hu, Z.; Wei, Q.; et al. Diatomic active sites nanozymes: enhanced peroxidase-like activity for dopamine and intracellular
44. Zeng, R.; Gao, Q.; Xiao, L.; et al. Precise tuning of the D-band center of dual-atomic enzymes for catalytic therapy. J. Am. Chem. Soc. 2024, 146, 10023-31.
45. Ma, C. B.; Xu, Y.; Wu, L.; et al. Guided synthesis of a Mo/Zn dual single-atom nanozyme with synergistic effect and peroxidase-like activity. Angew. Chem. Int. Ed. 2022, 61, e202116170.
46. Chen, Q.; Liu, Y.; Lu, Y.; et al. Atomically dispersed Fe/Bi dual active sites single-atom nanozymes for cascade catalysis and peroxymonosulfate activation to degrade dyes. J. Hazard. Mater. 2022, 422, 126929.
47. Li, B.; Ma, R.; Chen, L.; et al. Diatomic iron nanozyme with lipoxidase-like activity for efficient inactivation of enveloped virus. Nat. Commun. 2023, 14, 7312.
48. Li, M.; Wang, G.; Dai, J.; et al. Bioinspired CuZn-N/C single-atom nanozyme with high substrate specificity for selective online monitoring of epinephrine in living brain. Anal. Chem. 2023, 95, 14365-74.
49. Tian, R.; Ma, H.; Ye, W.; et al. Se-containing MOF coated dual-Fe-atom nanozymes with multi-enzyme cascade activities protect against cerebral ischemic reperfusion injury. Adv. Funct. Mater. 2022, 32, 2204025.
50. Li, K.; Miao, Y.; Song, K.; et al. Collaborative CuMn diatomic nanozyme to boost nanocatalytic/mild photothermal/chemo-therapy through overcoming therapeutic resistance. Chem. Eng. J. 2023, 471, 144693.
51. Zeng, R.; Li, Y.; Hu, X.; et al. Atomically site synergistic effects of dual-atom nanozyme enhances peroxidase-like properties. Nano. Lett. 2023, 23, 6073-80.
52. Ning, S.; Zhang, Z.; Ren, Y.; et al. A synergistic dual-atom sites nanozyme augments immunogenic cell death for efficient immunotherapy. Adv. Sci. 2025, 12, e2414734.
53. Jiao, L.; Ye, W.; Kang, Y.; et al. Atomically dispersed N-coordinated Fe-Fe dual-sites with enhanced enzyme-like activities. Nano. Res. 2022, 15, 959-64.
54. He, T.; Santiago, A. R. P.; Kong, Y.; et al. Atomically dispersed heteronuclear dual-atom catalysts: a new rising star in atomic catalysis. Small 2022, 18, e2106091.
55. Wang, Y.; Wang, Y.; Lee, L. Y. S.; Wong, K. Y. An emerging direction for nanozyme design: from single-atom to dual-atomic-site catalysts. Nanoscale 2023, 15, 18173-83.
56. Liu, M.; Li, N.; Cao, S.; et al. A "pre-constrained metal twins" strategy to prepare efficient dual-metal-atom catalysts for cooperative oxygen electrocatalysis. Adv. Mater. 2022, 34, e2107421.
57. Zhang, M.; Lu, X.; Wu, Z.; et al. Advanced development of dual-atom catalysts: From synthesis methods to versatile electrocatalytic applications. J. Power. Sources. 2024, 613, 234923.
58. Johnson, R. W.; Hultqvist, A.; Bent, S. F. A brief review of atomic layer deposition: from fundamentals to applications. Mater. Today. 2014, 17, 236-46.
59. Cong, B. T.; Zhao, W. H.; Liang, Z.; et al. Understanding the synergistic catalysis effect on the dual-metal-N4 embedding single-walled carbon nanotubes from first principles. Mater. Today. Commun. 2024, 38, 107800.
60. Li, J.; Xue, H.; Xu, N.; et al. Co/Ni dual-metal embedded in heteroatom doped porous carbon core-shell bifunctional electrocatalyst for rechargeable Zn-air batteries. Mater. Rep. Energy. 2022, 2, 100090.
61. Li, Z.; Ding, B.; Li, J.; et al. Multi-enzyme mimetic MoCu dual-atom nanozyme triggering oxidative stress cascade amplification for high-efficiency synergistic cancer therapy. Angew. Chem. Int. Ed. 2025, 64, e202413661.
62. Zhang, Y. X.; Zhang, S.; Huang, H.; et al. General synthesis of a diatomic catalyst library via a macrocyclic precursor-mediated approach. J. Am. Chem. Soc. 2023, 145, 4819-27.
63. Yang, X.; Xiang, J.; Su, W.; et al. Modulating Pt nanozyme by using isolated cobalt atoms to enhance catalytic activity for alleviating osteoarthritis. Nano. Today. 2023, 49, 101809.
64. Zhang, M.; Xu, W.; Gao, Y.; Zhou, N.; Wang, W. Manganese-iron dual single-atom catalyst with enhanced nanozyme activity for wound and pustule disinfection. ACS. Appl. Mater. Interfaces. 2023, 15, 42227-40.
65. Chen, J.; Zhong, J.; Lai, J.; et al. Enhancing catalytic performance of Fe and Mo co-doped dual single-atom catalysts with dual-enzyme activities for sensitive detection of hydrogen peroxide and uric acid. Anal. Chim. Acta. 2023, 1273, 341543.
66. Qu, Y.; Zhuang, L.; Bao, W.; et al. Atomically dispersed nanozyme-based synergistic mild photothermal/nanocatalytic therapy for eradicating multidrug-resistant bacteria and accelerating infected wound healing. RSC. Adv. 2024, 14, 7157-71.
67. Xu, J.; Zheng, X.; Feng, Z.; et al. Organic wastewater treatment by a single-atom catalyst and electrolytically produced H2O2. Nat. Sustain. 2021, 4, 233-41.
68. Baer, D. R.; Blanchard, D. L.; Engelhard, M. H.; Zachara, J. M. The interaction of water and Mn with surfaces of CaCO3: an XPS study. Surf. Interface. Anal. 1991, 17, 25-30.
69. Ramana, C. V.; Massot, M.; Julien, C. M. XPS and Raman spectroscopic characterization of LiMn2O4 spinels. Surf. Interface. Anal. 2005, 37, 412-6.
70. Tong, Y.; Wu, J.; Chen, P.; et al. Vibronic superexchange in double perovskite electrocatalyst for efficient electrocatalytic oxygen evolution. J. Am. Chem. Soc. 2018, 140, 11165-9.
71. Li, J.; Chu, D.; Dong, H.; Baker, D. R.; Jiang, R. Boosted oxygen evolution reactivity by igniting double exchange interaction in spinel oxides. J. Am. Chem. Soc. 2020, 142, 50-4.
72. Yang, G.; Zhu, J.; Yuan, P.; et al. Regulating Fe-spin state by atomically dispersed Mn-N in Fe-N-C catalysts with high oxygen reduction activity. Nat. Commun. 2021, 12, 1734.
73. Shen, X.; Wang, Z.; Gao, X.; Zhao, Y. Density functional theory-based method to predict the activities of nanomaterials as peroxidase mimics. ACS. Catal. 2020, 10, 12657-65.
74. Schewe, T.; Halangk, W.; Hiebsch, C.; Rapoport, S. M. A lipoxygenase in rabbit reticulocytes which attacks phospholipids and intact mitochondria. FEBS. Lett. 1975, 60, 149-52.
75. Belkner, J.; Wiesner, R.; Kühn, H.; Lankin, V. Z. The oxygenation of cholesterol esters by the reticulocyte lipoxygenase. FEBS. Lett. 1991, 279, 110-4.
76. Luo, M.; Koper, M. T. M. A kinetic descriptor for the electrolyte effect on the oxygen reduction kinetics on Pt(111). Nat. Catal. 2022, 5, 615-23.
77. Lv, X.; Wei, W.; Huang, B.; Dai, Y.; Frauenheim, T. High-throughput screening of synergistic transition metal dual-atom catalysts for efficient nitrogen fixation. Nano. Lett. 2021, 21, 1871-8.
78. Chu, D.; Zhao, M.; Rong, S.; et al. Dual-atom nanozyme eye drops attenuate inflammation and break the vicious cycle in dry eye disease. Nanomicro. Lett. 2024, 16, 120.
79. Song, Z.; Ou, J.; Zhu, F.; et al. Novel enzyme cascade colorimetric nanosensing platform based on 3D diatomic nanozymes synergistically enhancing peroxidase-like activity for the detection of xanthine. Microchem. J. 2024, 207, 111735.
80. Li, J. Q.; Mao, Y. W.; Zhang, R.; Wang, A. J.; Feng, J. J. Fe-Ni dual-single atoms nanozyme with high peroxidase-like activity for sensitive colorimetric and fluorometric dual-mode detection of cholesterol. Colloids. Surf. B. Biointerfaces. 2023, 232, 113589.
81. Jin, X.; Feng, X.; Wang, G.; et al. Zn-Y dual atomic site catalyst featuring metal-metal interactions as a nanozyme with peroxidase-like activity. J. Mater. Chem. A. 2023, 11, 2326-33.
82. Liu, S.; Wei, Y.; Liang, Y.; et al. Engineering nanozymes for tumor therapy via ferroptosis self-amplification. Adv. Healthc. Mater. 2024, 13, e2400307.
83. Wang, Z.; Wen, H.; Zheng, C.; et al. Synergistic Co-Cu dual-atom nanozyme with promoted catalase-like activity for parkinson’s disease treatment. ACS. Appl. Mater. Interfaces. 2025, 17, 583-93.
84. Liu, Y.; Niu, R.; Deng, R.; Song, S.; Wang, Y.; Zhang, H. Multi-enzyme co-expressed dual-atom nanozymes induce cascade immunogenic ferroptosis via activating interferon-γ and targeting arachidonic acid metabolism. J. Am. Chem. Soc. 2023, 145, 8965-78.
85. Wang, S.; Hu, Z.; Wei, Q.; et al. Precise design of atomically dispersed Fe, Pt dinuclear catalysts and their synergistic application for tumor catalytic therapy. ACS. Appl. Mater. Interfaces. 2022, 14, 20669-81.
Cite This Article

How to Cite
Download Citation
Export Citation File:
Type of Import
Tips on Downloading Citation
Citation Manager File Format
Type of Import
Direct Import: When the Direct Import option is selected (the default state), a dialogue box will give you the option to Save or Open the downloaded citation data. Choosing Open will either launch your citation manager or give you a choice of applications with which to use the metadata. The Save option saves the file locally for later use.
Indirect Import: When the Indirect Import option is selected, the metadata is displayed and may be copied and pasted as needed.
About This Article
Copyright
Data & Comments
Data
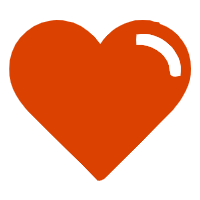
Comments
Comments must be written in English. Spam, offensive content, impersonation, and private information will not be permitted. If any comment is reported and identified as inappropriate content by OAE staff, the comment will be removed without notice. If you have any queries or need any help, please contact us at [email protected].