Greenhouse gas emissions and offset potential from sugarcane straw for bioenergy production in Brazil
Abstract
This study aims to assess the additional Greenhouse gas (GHG) emissions affected by straw removal from the soil surface in sugarcane areas, including measurement of short-term soil CO2-C emissions plus emissions associated with the recovery and transport operations of straw bales until to the industry gate (diesel emissions) and estimated soil N2O emission, comparing with leaving all straw on the soil surface. Taking into account the main sources evaluated (soil CO2, diesel and N2O from straw), the total additional GHG emissions from the recovery of 6.9 Mg Dry Matter ha-1 (27%) was estimated at 1423 kg CO2eq ha-1, resulting in a carbon footprint of
Keywords
INTRODUCTION
Sugarcane (Saccharum spp.) is considered one of the main alternatives for replacing fossil fuels in the biofuel sector due to its great potential in the production of ethanol and its by-products. The sugarcane production in the 2021/22 harvest season in Brazil reached 585.2 million tons with a harvested area of 8317 thousand hectares, a reduction of 299 thousand hectares compared to the 2020/21 due to adverse climatic effects of the drought during the productive cycle[1]. In the global ethanol industry, sugarcane is one of the main crops with an abundant production of crop residue commonly denominated as “sugarcane straw”[2], which can be an option to be recovered to produce additional renewable energy or second-generation ethanol, depending on the management system adopted.
Bioenergy production could potentially mitigate greenhouse gas (GHG) emissions and climate change by avoiding the combustion of fossil energy, therefore safeguarding energy security. However, there are issues to be considered, such as the identification of crop and straw management systems with lower GHG emissions, including the sustainability of practices and the efficiency of bioenergy systems[3-6]. The increased attention on bioenergy occurs in conjunction with the global interest in transitioning from large-scale dependence upon fossil fuels to the use of renewable energy sources to curb GHG emissions[7]. Evidence suggests that bioenergy options with low lifecycle emissions can reduce GHG emissions; however, outcomes are site-specific and rely on efficient integrated “biomass-to-bioenergy systems”, and sustainable land use management and governance[3-5,7].
Several acts and standards have been developed in different countries to impose stringent requirements on biofuel producers to confirm the environment-friendly characteristics of their products and their ability to effectively reduce GHG emissions in comparison with corresponding fossil fuels[8]. Towards this goal, the National Biofuel Policy, officially known as RenovaBio Program, was established under Law 13.576/2017 in Brazil. This program provides a framework to certify biofuel production and allows biofuel producers to earn decarbonization certificates (CBIOs) for proven and independently verified reductions in GHG emissions from the production and use of biofuels (1 CBIO = 1 tonne of avoided CO2eq emissions)[9]. The decarbonization certificates are an important mechanism to price and stimulate management practices that reduce GHG emissions[9] and should be supported by research results. However, the effectiveness of such mechanisms rests on the fact that the amount of CBios will be linked to the reduction in GHG emissions associated with the production of a given biofuel in comparison to its fossil competitor[8], as investigated in this study.
Inventorying GHG balance of sugarcane-based by-products is critical to assess the degree of carbon (C) neutrality of biofuels production[9,10], and the advances to better support renewable energy sources and sustainability parameters, including the GHG balance of the bioenergy sources, should be better investigated to support public policies in Brazil and worldwide.
The impact of sugarcane straw removal on economic[11], environmental and agronomic factors[4,12] are well documented and discussed in terms of sugarcane growth and biomass production[4], nutrient recycling, soil conservation, soil biological attributes, greenhouse gas emissions[5,10,13], weed control and pest infestation[4,11,14,15]. Nevertheless, there is potential to withdraw part of the straw from the fields to produce bioelectricity and cellulosic ethanol (second generation-2G). However, further studies are needed to demonstrate the sustainability of different practices and the best options for industrial use of this crop residue and their GHG balance.
Regardless of the considerable potential for increased energy generation from the use of recovered straw, few studies worldwide have investigated the impact of sugarcane straw removal on soil CO2-C emissions and additional GHG emissions associated with straw removal and transport operations[13,16,17]. In a sugarcane area after 3 years of green mechanized harvesting adoption, de Figueiredo et al. demonstrated that 100% of straw removal from the soil surface compared with no removal management led to an additional emission of 927.7 kg of CO2 ha-1 to the atmosphere over a 25-day measurement period[13]. In terms of the GHG balance, emissions from straw management or recovery in sugarcane areas can lead to additional soil CO2 emission and annul the benefits of banning the pre-harvest burning, which is responsible for an estimated net emission (CH4 and N2O) of 941 kg CO2eq ha-1[18]. It is important to highlight that results for measurements of additional short-term soil CO2-C emission from different managements can support better cause-effect comprehension related to further impact on soil C accumulation.
This study aims to assess the additional GHG emissions affected by straw removal from the soil surface in sugarcane areas, including measurement of short-term soil CO2-C emissions plus emissions associated with the recovery and transport operations of straw bales to the factory gate (diesel emissions) and estimated soil N2O emission, in contrast to leaving all the straw on the soil surface.
The specific objective is to quantify the additional GHG emissions associated with straw recovery, assess the potential of straw for bioenergy production (electricity generation or 2G ethanol), and compare these total emissions with the possible avoided emissions if a producer uses electricity from the National energy grid (in the absence of all practice to recover and produce bioenergy-leaving all straw on the soil surface), based on GHG emission factors (Mg CO2eq MWh year-1) for the Brazilian energy matrix.
The hypotheses tested in the present study were: (1) The recovery and transport of large amounts of sugarcane straw from the field after mechanized harvesting without burning may result in enhanced soil CO2 and other GHG emissions (soil N2O and emissions from diesel combustion), greater than the potential to offset emissions through the use of straw for bioenergy (bioelectricity or 2G ethanol); and (2) Partial removal of sugarcane straw from the soil surface can be a sustainable alternative for its use as bioelectricity source or 2G ethanol production, taking into account their additional GHG emissions and offset potential.
MATERIALS AND METHODS
This study was conducted at a commercial sugar, ethanol and energy production plant located in the region of Ribeirão Preto, Sao Paulo, southern Brazil, where the company performs the removal of straw for bioenergy generation. The field experiment for measuring soil CO2-C emissions was in Serrana, Sao Paulo State, Brazil (21°06′ S-47°37′ W, 623 m of altitude). According to the data provided by the agricultural and industrial facility, the sugarcane variety in the experimental area was IACSP 95-5000, planted at 1.5 m inter-row spacing and with a first cutting stalk yield of 143 Mg ha-1. The field experiment started on 3 August 2016, immediately after the straw removal, in which all operations were carried out within 20 days of mechanized harvest without burning, following the standard procedure of the company. The regional climate is classified as B2rB′4a′ by Thornthwaite system[19], indicating a mesothermal region with rainy summers (B2, wet climate), with or without hydric deficiency (r), mesothermic (B′4, potential evapotranspiration < 1140 and ≥ 997) and thermal climate subtypes (a′). The soil is classified as a Eutroferric Red Latosol (Haplustox, USDA Soil Taxonomy).
Field treatments for measuring soil CO2-C emissions, soil water content and soil temperature
The experiment for measuring soil CO2-C emissions comprised of three treatments replicated three times (03 treatments × 03 times) in a commercial sugarcane plantation. The treatments consisted of a control (T1), which are areas of 12 m × 20 m plots (~240 m2) where all straw was left on the soil surface after sugarcane harvest without any straw management, and two field conditions (T2 and T3 explained below), according to the soil cover observed after the straw management (straw heaping and bailing) and recovery operation (bale picking and transport) [Figure 1].
Figure 1. Schematic figure showing T1 area, with 100% of straw maintained on the soil surface; T2, line plots where straw was heaped for subsequent baling (i.e., 17% of 01 hectare) and T3 area where straw was recovered from six lines per each pass of a tractor (i.e., 83% of 01 hectare)
In areas where straw is managed for recovery, straw from 6 crop rows (5 inter-row areas) was collected with each pass of a tractor and heaped between two crop rows (one inter-row area) to be removed later by baling [Figure 1]. After straw was baled and removed, there is still more straw left on the soil surface of the inter-row area where straw was heaped prior to baling than in the control area. This inter-row area where straw was accumulated was defined as T2 (line plots of 1.5 m × 20 m or 30 m2). The area of 6 crop rows from which straw was collected has less straw on the soil surface than in the control area, and this area was defined as T3
Short-term soil CO2-C emissions, soil water content (SWC) and soil temperature (12 cm depth) were monitored, starting 24 h after straw removal (4 August 2016) in different treatment plots where PVC collars were previously inserted into soil (15 replicates per treatment). Measurements were taken for 17 days over a 21-day period after straw removal in the morning between 8:00 to 11:00 AM on each sampling day. Soil

where, WAFCO2: weighted average CO2 flux; FCO2T3: CO2 flux from T3 area; and FCO2T2: CO2 flux from T2 area. In each treatment (3 replicates), the same 15 PVC collars were used for measurements of soil
The results of GHG emissions and offset potential for straw recovery were presented in terms of carbon dioxide equivalents (CO2eq) according to the global warming potentials of 1, 28 and 265 for carbon dioxide (CO2), methane (CH4) and nitrous oxide (N2O), respectively[20]. In addition, molar ratio of
Soil analysis, quantification of post-harvest straw and estimated N2O emissions
Soil samples were collected to a 0.20-m depth after measurements of soil CO2-C emissions. To obtain 1 kg of soil, 12 single samples were collected from the experimental area, and soil physical-chemical characteristics such as pH, soil organic matter (SOM) and soil texture were analyzed after sieving and adjusting the pH to 10-11 with 1 M NaOH; the particle size distribution (sand, silt, and clay) was determined by the pipette method and pH was determined by the pH SMP method measured in water and in the solution of 0.01 mol l-1 CaCl2. The samples were oven dried and sieved through a 2 mm mesh prior to analysis. These analyses include the determination of SOM content by oxidation with sulphuric acid[21], presented in Table 1.
Soil chemical and physical characterization of the experimental area (0-20 cm depth)
pH | SOM | P | Ca | Mg | K | CEC | BS | Clay | Silt | Sand |
g dm-3 | mg dm-3 | mmolc dm-3 | % | g kg-1 | ||||||
7 | 31 | 10 | 31 | 10 | 6.4 | 83 | 58 | 52 | 18 | 30 |
The amount of straw deposited on the soil surface after sugarcane green mechanized harvesting, as well as straw management, was determined by collecting samples using a metallic frame of 0.64 m2 (0.8 m × 0.8 m) in each of the three treatments (5 replications). The samples were oven dried for 24 h at 105 °C until reaching constant weight and then weighed[22], which resulted in: 25.8 Mg Dry Matter (DM) ha-1 after oven dried for T1; 44.3 Mg DM ha-1 for T2 and 18.9 Mg DM ha-1 for T3. The average amount of straw removed from the field after straw management and recovery operation was calculated as the difference of straw mulch dry matter left on the soil surface between T1 and T3 (T3-T1), which resulted in 6.9 Mg DM ha-1 or 27% of the total straw in the control area.
Crop residues such as sugarcane straw, when returned to the soil after harvest, are considered as a direct source of N2O from agricultural soils[23], and an average N2O-N emission factor of 1% is recommended for N content in any crop residues returned to soil for estimating the direct N2O emissions attributable to crop residue decomposition, which means that 1% of N from crop residues will be emitted as N2O[24].
The amount of total N returned to soil from sugarcane crop residues was estimated by applying green tops and dry leaves content of 65% and 35%, respectively of the total N present in sugarcane crop residues[6,25], which translate to an average rate of 7.5 and 3.4 g N kg-1 of dry matter, respectively. We considered that only 20% of sugarcane straw mass was reduced or mineralized during a period of 1 year[26], and applying a rate of 20% of straw N content available for N2O emission each year, we estimate an average amount of
In order to derive an N2O-N emission factor for crop residue removal, Gonzaga et al. carried out field experiments in commercial sugarcane fields in clay soils (64% clay content) similar to the experimental site of this study (52% clay; Table 1), reporting cumulative N2O-N emission of 1.45 kg N2O-N ha-1 associated with the maintenance of 15 Mg DM of straw ha-1 without N fertilizer applications over a 270-days period[27]. Furthermore, the same authors demonstrated that N2O-N emissions decreased to 0.91 kg N2O-N ha-1 with the total recovery of straw from the soil surface without N fertilizer. Considering the similarity with soils and climate conditions of this study, we applied the same cumulative N2O-N emissions[27] to be compared with the results calculated using the IPCC methodology, which consider emission factors of 0.01 kg
Estimation of greenhouse gas emissions from fossil fuel used in the recovery and transport of straw
In addition to industrial plants, further investments in tractors, trucks and other heavy machinery are required for the management and recovery of sugarcane straw after green mechanized harvest. For this experiment, the data collected and analyzed included truckload capacity of approximately 70 bales per trip, bales weighing on average about 350 kg bale-1 and 24.5 Mg of straw transported per trip (16 km round trip, Table 2), with a straw moisture content of around 6 to 10%.
Technical parameters of diesel consumption in mechanized operations for the amount of sugarcane straw recovered of
Operation | Equipment | Horse power (HP) | Consumption (L diesel ha-1) | Operation income (ha h-1) | L diesel ton-1 of straw |
(1) Straw heaping | Tractor | 110 | 7.00 | 8.2 | 0.9 |
(2) Baling | Tractor | 210 | 20.00 | 5.5 | 3.0 |
(3) Bales picking | Tractor | 210 | 13.7 | 5.4 | 3.0 |
(4) Bales loading | Tractor | 130 | 4.5 | 8.0 | 2.1 |
*(5) Bales transport | Truck | - | 1.4 km L-1 | - | 0.466 (0.233 × 2) |
(6) Bales unloading | Loader | 130 | 4.3 (L h-1) | - | 0.122 |
(7) Crusher feeding | Loader | 130 | 3.1 (L h-1) | - | 0.257 |
Total (L diesel ton-1 straw) | 9.895 | ||||
Total (L diesel ha-1) | 68.2 |
The technical parameters for mechanized operations considered in the straw management and recovery operation and the transport of straw until to the biorefinery gate are presented in Table 2. The fossil fuel typically used for sugarcane production systems is diesel oil, consumed mostly during agricultural operations by machinery, tractors and trucks[18]. In our study, the diesel consumption related to the final use of sugarcane straw was measured according to all field operations performed [Table 2].
Estimates of GHG emissions due to the use of fossil fuel for straw recovery in this study assumed CO2, CH4 and N2O gases[24]. The emission factors applied were those suggested by Air Pollution Control Program for Auto Engines)/CETESB-Brazil[29], in association with IBAMA (Brazilian Institute of the Environment), considering the types of fuel and vehicles. To calculate these emission factors, vehicles were considered as off-road and machinery, with 74,100 kg CO2 TJ-1 (TJ = Terajoule), 4.15 kg CH4 TJ-1 and 28.6 kg N2O TJ-1, respectively[24]. The GHG emissions related to diesel extraction and distribution were considered as
Quantification of energy, cellulosic ethanol (2G) production from straw recovered and metrics for their carbon footprints
The use of sugarcane bagasse as a fuel for combined heat and power (CHP) systems to meet energy needs of the mills for bioenergy generation is a common practice in the Brazilian sugarcane industry[31]. In this study, we present an additional energy generation potential of sugarcane straw recovered after green mechanized harvesting as per the technology used by the company. According to the information from the company's industrial sector, the straw used for energy generation is added to the sugarcane bagasse at a proportion of 5 to 7% of the volume, presenting a potential value for electricity generation of approximately
Analyzing the use of sugarcane straw for power generation, a higher heating value of cane straw of
Based on data from parameters for vehicle emissions in Brazil, an emission factor (EF) of 1.750 kg CO2eq L-1 of hydrated ethanol should be applied for a vehicle year 2010, flex (ethanol or gasoline), using ethanol, with an efficiency of 8.2 km L-1, considering emissions of CO2 (207 g km-1), CH4 (0.053 g km-1) and N2O
Parameters of emission factors (EF) for ethanol and gasoline in Brazil and total EF for each fuel and respective efficiency
Ethanol* | EF (g CO2eq L-1) | ||
Gas | EF (g km-1) | EF (g L-1) | |
CH4 | 0.053 | 0.4346 | 10.87 |
CO2 | 0.0 | 0.00 | |
N2O | 0.017 | 0.1394 | 36.94 |
Total EF | 47.81 | ||
Gasoline** | EF (g L-1) | EF (g CO2eq L-1) | |
CH4 | 0.009 | 0.0972 | 2.43 |
CO2 | 207 | 0.0 | 2235.60 |
N2O | 0.023 | 0.2484 | 65.83 |
Total EF | 2303.86 |
Carbon footprint is widely defined as the amount of carbon that is emitted during a process or by an organization or entity. It is a popular metric that appeared in media, conferences, and government and environmental institute’s reports as pressure from the public concerning pollution and its impact on human health, and it is generally used as a measure of atmospheric pollution due to anthropogenic activities[36]. The parameters used in our study to present the C footprints were based on the total additional amount of GHG emitted related to all practices applied to recover sugarcane straw from the field to produce bioenergy or 2G ethanol. Our metrics were based on these total additional GHG emissions (soil CO2-C emission, diesel from mechanized operations and N2O from straw management), divided by four main parameters assessed:
CO2 emission factors for electric energy production in Brazil
The CO2 emission factors of electric energy production used in life-cycle inventories in Brazil predict the amount of CO2 associated with the generation of a particular type or source of electric energy. These emission factors are calculated by the average of emissions sources, considering all the plants that are generating energy, and not only those that are working at the moment[37], presenting emission factors in terms of Mg CO2eq MWh-1.
The CO2 emission factors calculated according to the methodological approach “Tool to calculate the emission factor for an electricity system, version 04.0 and earlier” approved by the Clean Development Mechanism (CDM), Executive Board are intended to estimate the contribution, in terms of CO2 emission reductions, from a project that generates electricity for the grid[37]. Briefly, the interconnected system CDM emission factor is a combination of the operating margin emission factor, which reflects the intensity of CO2 emission of the energy dispatched at the margin, and the build margin emission factor, which reflects the intensity of CO2 emissions from the last plants built[37].
The emission factors (Mg CO2 MWh-1) for electricity generation in Brazil presented annual average values of 0.0740; 0.0927; 0.0817; 0.1244; 0.1355; 0.0960; 0.0653 and 0.0292 for the years 2018, 2017, 2016, 2015, 2014, 2013, 2012 and 2011, respectively[37]. However, in this study, the offset potential for straw recovered displacing energy produced by the Brazilian energy matrix was calculated based on the EF of
Statistical analysis
Data related to CO2-C emissions (µmol m-2 h-1), soil temperature (°C) and soil water content (SWC, %) were analyzed using a completely randomized experimental design with three treatments and three replicates per treatment (3 × 3) with 5 sub-samples within each experimental unit to compose 01 sample per treatment, totaling 45 sampling points and repeated measurements in time. For the analyses, the mixed-model procedure of SAS statistical software was used [SAS Institute Inc., Cary, North Carolina]. When statistically significant (P < 0.05), the means were compared by Tukey’s test at a significance level of 5%. In this case, we used SAS PROC MIXED procedure for repeated measurements to test for treatment effects and Tukey’s procedure was used to adjust for multiple mean comparisons using LSMEANS options. The variables
Linear coefficient of correlation between soil CO2-C emission and soil properties (soil temperature and soil water content)
Treatment | Soil temperature | Soil water content | ||||
r | P-value | R2 | r | P-value | R2 | |
1 | 0.18 | 0.48 | 0.034 | 0.57 | 0.01581 | 0.33 |
2 | 0.062 | 0.81 | 0.004 | 0.61 | 0.01010 | 0.37 |
3 | 0.17 | 0.51 | 0.029 | 0.75 | < 0.001 | 0.56 |
RESULTS
Short-term soil CO2-C emissions, soil water content and soil temperature
Results of Pearson’s linear correlation coefficients are presented in Table 4, together with the R2 values of the adjustment of a linear model to the soil CO2 emission data, individually adjusted for the studied treatments. In addition, the P-value associated with the linear regression analysis was presented, H and zero confirms that the estimated regression coefficient is equal to zero. Thus, for the treatments studied, a linear relationship was observed between soil CO2 emission and only soil water content (P < 0.05). The same was not observed for soil temperature, which shows no relationship between soil CO2 emission and soil temperature for all treatments studied.
Figure 2A presents the temporal soil CO2-C emissions comparing the management of recovering part of straw (T3; leaving 18.9 Mg DM ha-1) with the management of keeping all straw on the soil surface without removal (T1; keeping 25.8 Mg DM ha-1), which result in a straw removal rate of 6.9 Mg DM ha-1. A significant difference (P < 0.05) among treatments was observed only on day 20, showing that agricultural operations to recover around 27% of straw may not significantly impact the temporal soil CO2-C emissions during the measurements. The highest temporal emissions were observed in T2, except for day 17
Figure 2. (A) Soil CO2-C emissions (µmol m-2 h-1); (B) soil temperature (°C); (C) soil water content (%) plus standard errors (vertical bars) and (D) Accumulated soil CO2-C emission (plus standard error, kg CO2-C ha -1) for T1: Control area, with straw maintained on the soil surface; T2: Line plots where straw was recovered and accumulated in one line and later collected for baling (17% of harvest area); T3: Areas where the normal mechanized straw harvesting operations were performed (83% of the harvested area); WAFCO2: Weighted average of soil FCO2 (weighted average of emission in T2 and T3). Means followed by the same letters comparing straw management types did not differ by Tukey’s test (P < 0.05).
The impacts of straw management on soil temperature can be observed in Figure 2B when comparing T1 (100% straw maintained) with T3 (27% of straw recovered). For the 21-day period analyzed, there was a significant difference between these two treatments only on days 07 and 09 (P < 0.05), in which soil temperature was higher in T3, showing that recovering 27% of straw had a slight impact on soil temperature.
Comparing the management of removing 27% of straw (T3) with the management of keeping all straw on the soil surface (T1) during the dry period, there was no significant difference in soil water content
In terms of the cumulative short-term soil CO2-C emissions [Figure 2D], the emissions were lowest in T1 (100% straw) with 788 kg CO2-C or 2889 kg CO2 ha-1. However, the comparison of cumulative soil CO2-C emissions in the management of recovering 27% of straw (WAFCO2 - weighed average T2 and T3 - 963 kg
Emissions from fossil fuel used to recover and transport straw
Specific management options practiced by sugarcane mills for straw recovery systems certainly have distinct impacts on the economic and technical parameters. Taking into account all additional mechanized field operations for straw recovery and their respective machinery use and diesel consumption [Table 2], the total amount of diesel consumed to recover 1 Mg of straw under the experimental parameters was 9.9 L Mg-1 of straw or 68.2 L of diesel ha-1 (6.9 Mg ha-1, dry matter-basis), resulting in a carbon footprint for diesel of
Estimation of N2O emissions derived from post-harvest sugarcane straw
Applying the default methodology for N2O emissions[24] and measured straw dry mass in the present experiment, leaving all straw on the soil surface without management (T1 - 100% straw) led to an estimated N2O emission of 159 kg CO2eq ha-1. In contrast, recovering 6.9 Mg DM of straw ha-1 (27% straw removed-T3) resulted in an estimated N2O emission of 117 kg CO2eq ha-1. Thus, comparing the practice of leaving all straw on the soil surface against the practice of recovering 27% of straw (T3), there was an estimated avoided N2O emission of 42 kg CO2eq ha-1 [Figure 3].
Comparing the estimated N2O emissions with the IPCC methodology[24], previous research had reported higher cumulative N2O emission of 2.28 kg N2O ha-1 or 604.2 kg CO2eq ha-1 over a 270-day period[27] when sugarcane straw was left on soil at a rate of 15 Mg DM ha-1, which was used in calculating the GHG balance for the potential of straw for bioelectricity or 2G ethanol production in our study [Figure 3].
Potential for straw-based bioenergy and GHG balance of energy or cellulosic ethanol production
With 6.9 Mg of straw DM ha-1 recovered, it is possible to generate 4.1 MWh ha-1 of electricity (Company parameter), which means that for each Mg of recovered sugarcane straw, considering the emission factor from new projects and energy generation intensity[37] from Brazilian energy matrix (the base year 2018, 0.1370 Mg CO2 MWh-1), GHG emission of 81.5 kg CO2eq Mg-1 of straw or 0.5617 Mg CO2eq ha-1
The 2G ethanol produced from lignocellulosic biomass (LM) has been considered the biofuel with the greatest potential to replace fossil fuels[38,39]. Based on our experimental results, with the production potential of 240 L 2G ethanol Mg-1 LM (Dantas et al.[31], 2013), it would be possible to produce an additional 1656 L of ethanol ha-1 (6.9 Mg DM straw ha-1), which could promote an avoided GHG emission of 2.26 kg CO2eq L-1 of 2G ethanol by replacing 1 liter of gasoline or 3743 kg CO2eq ha-1 considering only CH4 and N2O emissions from 2G ethanol combustion [Figure 3].
Taking into account all mechanized operations to recover around 27% of sugarcane straw, the total additional GHG emissions were estimated at 1465 kg CO2eq ha-1 (for 6.9 Mg DM ha-1) minus
Applying the parameters cited in this study for electricity or 2G ethanol production, our results for their respective GHG balance (emissions and offset potential) showed that straw-based electricity would result in additional GHG emission of (+) 860 kg CO2eq ha-1 [Figure 3]. In contrast, avoided GHG emission of (-) 2320 kg CO2eq ha-1 could be achieved [Figure 3] when applying the same parameters for 2G ethanol production replacing gasoline.
DISCUSSION
Short-term soil CO2-C emissions, soil water content and soil temperature
The highest temporal CO2-C emissions observed in T2 (straw accumulated after harvest) could be explained by the straw management and accumulation of large amounts of straw between two crop lines (inter-row), which could promote higher soil aeration, higher O2 availability for microorganisms activity and higher C accessibility (straw carbon) to be decomposed through mineralization process. In another sugarcane field experiment, keeping all sugarcane straw on the soil surface without management led to a 253 kg CO2-C ha-1 reduction in emissions compared with recovering all straw[13], probably due to higher soil bulk density and lower soil aeration[40], showing that in a condition where soil and straw were managed, the mineralization of SOM (soil organic matter) increased significantly with higher aeration, thus supporting the findings observed in T2.
It is important to note that short-term soil CO2-C emissions in sugarcane areas may be higher soon after the straw management; however, these emissions tend to stabilize to similar levels compared with the emissions from areas with no straw management, as observed in T1 and T3 at the end of the experimental period [Figure 2A], supporting the importance of short-term measurements instead of longer measurement periods. Previous research on GHG responses to sugarcane straw removal at a rate of
Measurements of short-term soil CO2 emissions can contribute to a better knowledge of the impact of straw removal on long-term soil organic C storage as a result of short-term higher or lower emissions from different soil and straw management. Our study assessed the variance by comparing the practice of maintaining all straw on the soil surface with the practice of removing part of this straw, and the impact of those practices only on the additional soil CO2 emission caused by straw management, as straw decays over time and reduces its presence on the soil surface in the long term. Hence, before determining CO2 sequestration rates, presenting short-term emissions and their correlation with soil properties can help understand the possible impact on soil CO2 losses. Our results (Figure 2D, T1 x WAFCO2) show that soil CO2 emission increases with this removal rate (27% of straw), but there is no significant difference from keeping all straw on the surface, which could be an important effect that can explain the long-term changes and straw amount that could be recovered without impacting CO2 sequestration rate in the long term. This effect of straw management is observed in the short term, simply because after about 30 or 40 days after soil or straw management, those additional emissions tend to stabilize to the same condition as no straw removal as observed in T1, compared to WAFCO2 [Figure 2A]. Other long-term experiments to assess the correlations of the rate of straw removal with the soil organic carbon storage shall be evaluated, but due to the complexity of such evaluations in terms of time and spatial variance, modeling studies may be applied to detect SOC changes.
In a scenario with straw removal and possible additional SOC losses from this management, no-tillage or reduced tillage practices combined with other best management practices, such as vinasse and filter cake application, could reverse the potential SOC losses associated with residue removal[13,26,41], contributing to a long-term soil C increase.
Estimation of N2O emissions derived from post-harvest sugarcane straw
Gonzaga et al. have shown that N2O emissions were reduced to 1.43 kg N2O ha-1 or 379 kg CO2eq ha-1
Compared to the management to recover sugarcane straw from the soil surface for multiple uses, leaving sugarcane straw on the soil surface can lead to higher N2O emissions, which would strongly influence the extent to which biofuels derived from straw decrease their GHG emissions relative to fossil fuels[44,45]. Additionally, regardless of N fertilizer application, the retention of sugarcane crop residue increases N2O emissions[27], acting as a catalyst for increased soil microbial activity and soil N2O flux[46]. Thus, we should find an equilibrium for straw recovering rate and related N2O emissions once leaving all straw on the soil surface result in higher N2O emissions and lower CO2 emissions.
Potential for straw-based bioenergy and GHG balance of energy or cellulosic ethanol production
The difference of straw energy generation parameter informed by the company (0.6 MWh Mg-1 of straw, 6%-10% humidity) resulted in a production of 4.1 MWh ha-1 (6.9 Mg DM ha-1), and contrasting with the potential 23.28 MWh ha-1, applying a lower heating value of 12.15 MJ kg-1 DM[33], can result in
The RenovaBio Program presents itself as a promising and innovative policy in many aspects (biofuels demand planning and boosting energy security in Brazil), in which every single biorefinery in the country will pursue the production of biofuels with an ever-higher environmental responsibility[8]. The characteristics of each country’s energy matrix, based on fossil fuels or renewable sources as found in Brazil[37], with average carbon emission intensity of 0.08735 Mg CO2 MWh-1 from years 2011 to 2018 as reference[37], will determine the potential for each country to develop and use straw-based bioenergy sources to reach a low-carbon energy matrix.
The results from this research can support deriving environmental indicators to assess the sustainability of 2G ethanol by regulatory and certifying bodies, such as the CARB (California Air Resources Board) and the most recent State Program “RenovaBio”, whose objective is to stimulate the efficiency of the biofuels industry, in the agricultural and industrial sectors with an emphasis on total energy demand and the mitigation of GHG emissions (lower C intensity) associated with each stage of the life cycle of bioenergy production.
Finally, summarizing results of two large-scale projects aiming to provide quantitative data to support decision-making for straw management and establish rational plans for sustainable straw removal[5], and analyzing scientific findings addressing soil conservation, soil compaction, soil carbon (C) stocks, and biology; nutrient cycling and fertilizer management; GHG emissions; pest management; crop yield; engineering solutions; and industrial performance, this study showed that sustainable straw removal is feasible in Brazil, but the recommendations of optimum removal must not be designed using isolated factors. They must be developed using integrated knowledge to ensure the sugarcane straw will not only provide bioenergy but also sustain multiple soil ecosystem services and crop yield[5]. Therefore, other sustainability parameters related to sugarcane straw recovery should be considered, such as soil type (sand or clay soils), harvest crop cycle and respective crop productivity, impact on soil erosion and crop nutrition, the balance of soil CO2-C (emission and sequestration rate), as well as N2O balance.
Conclusions
Bioelectricity and cellulosic ethanol production using different sugarcane straw recovering rates may not be a sustainable management strategy to mitigate GHG emissions, depending not only on the average amount of straw recovered but also on the remaining amount and associated impacts on sugarcane-cultivated soils, related additional GHG emissions and the efficiency rate for straw conversion in bioenergy products.
The main additional GHG emission sources from straw management and agricultural processes include soil CO2-C emissions, soil N2O emissions and emissions from mechanized field operations (diesel use), which should be accounted for in the deployment of new sugarcane bioenergy projects that uses straw as a raw material to contribute to a low carbon intensity matrix.
The route of recovering around 27% of sugarcane straw from the soil surface through bale system for bioelectricity production using the technical parameters and industrial efficiency rate of this case study could not be a sustainable option because the additional GHG emissions from these processes can be higher than its potential to offset generated emissions compared to the emission factor from the national energy matrix, resulting in a C footprint of 347 kg CO2eq MWh-1. Improving the efficiency rate for sugarcane straw conversion in bioelectricity based on its lower heating value could reduce its C footprint to
Employing the same parameters for straw recovery at the first sugarcane cutting cycle in clay soil, the option of producing 2G ethanol with around 27% of the total sugarcane straw recovered could offset greenhouse gas emissions replacing fossil gasoline, resulting in a C footprint of 0.86 kg CO2eq L-1 of 2G ethanol in the agricultural phase, which can be an option to contribute to better sustainability of sugarcane straw recovery, supporting renewable and sustainable bioenergy systems, and reducing the impacts of Global Climate Change.
DECLARATIONS
AcknowledgmentsWe are grateful to SãoPaulo State University (UNESP), Department of Engineering and Exact Sciences, Via de Acesso Prof. Paulo Donato Castellane s/n, Jaboticabal, SP, Brazil, for financial support.
Authors’ contributionsProject administration, conceptualization, investigation methodology, data curation, writing (original draft): de Figueiredo EB
Methodology, review & editing, validation: Jayasundara S
Methodology, review & editing, validation: de Oliveira Bordonal R
Software, data analyse, review: Rodrigo Panosso A
Funding acquisition; investigation; project administration, review: La Scala Jr N
Availability of data and materialsAll base data and statistical analysis used for this research are available.
Financial support and sponsorshipNone.
Conflicts of interestAll authors declared that there are no conflicts of interest.
Ethics approval and consent to participateWritten consent to publish potentially identifying information, such as details or the case and photographs, was obtained from the patient(s) or their legal guardian(s).
Consent for publicationWe confirm that we have given due consideration to the protection of intellectual property associated with this work and that there are no impediments to publication, including the timing of publication, with respect to intellectual property. In so doing we confirm that we have followed the regulations of our institutions concerning intellectual property.
Copyright© The Author(s) 2023.
REFERENCES
1. CONAB, Brazilian national company of provision. 2022. (In Portuguese). Available from: https://www.conab.gov.br/info-agro/safras/cana/boletim-da-safra-de-cana-de-acucar/item/download/43933_4c496920bffcfbfb45deb447d553a497 [Last accessed on 15 Feb 2023].
2. Aguiar A, Milessi TS, Mulinari DR, Lopes MS, da Costa SM, Candido RG. Sugarcane straw as a potential second generation feedstock for biorefinery and white biotechnology applications. Biomass Bioenergy 2021;144:105896.
3. Pachauri RK, Allen MR, Barros VR. Climate change 2014: synthesis report. Contribution of working groups i, ii and iii to the fifth assessment report of the intergovernmental panel on climate change. Geneva, Switzerland: IPCC, 2014. 32p.
4. Cherubin MR, Oliveira DMD, Feigl BJ, et al. Crop residue harvest for bioenergy production and its implications on soil functioning and plant growth: A review. Sci Agric 2018;75:255-72.
5. Carvalho JLN, Cerri CEP, Karlen DL. Sustainable sugarcane straw special issue: considerations for brazilian bioenergy production. Bioenergy Res 2019;12:746-8.
6. Cherubin MR, Lisboa IP, Silva AGB, et al. Sugarcane straw removal: implications to soil fertility and fertilizer demand in Brazil. Bioenergy Res 2019;12:888-900.
7. Lazaro LL, Giatti LL, Puppim de Oliveira JA. Water-energy-food nexus approach at the core of businesses - how businesses in the bioenergy sector in Brazil are responding to integrated challenges? J Clean Prod 2021;303:127102.
8. Klein BC, Chagas MF, Watanabe MDB, Bonomi A, Maciel Filho R. Low carbon biofuels and the New Brazilian National Biofuel Policy (RenovaBio): a case study for sugarcane mills and integrated sugarcane-microalgae biorefineries. Renew Sustain Energy Rev 2019;115:109365.
9. Carvalho JLN, Oliveira BG, Cantarella H, et al. Implications of regional N2O-N emission factors on sugarcane ethanol emissions and granted decarbonization certificates. Renew Sustain Energy Rev 2021;149:111423.
10. de Oliveira Bordonal R, Lal R, Alves Aguiar D, et al. Greenhouse gas balance from cultivation and direct land use change of recently established sugarcane (Saccharum officinarum) plantation in south-central Brazil. Renew Sustain Energy Rev 2015;52:547-56.
11. Cardoso TDF, Cavalett O, Chagas MF, et al. Technical and economic assessment of trash recovery in the sugarcane bioenergy production system. Sci Agric 2013;70:353-60.
12. Carvalho JLN, Nogueirol RC, Menandro LMS, et al. Agronomic and environmental implications of sugarcane straw removal: a major review. GCB Bioenergy 2017;9:1181-95.
13. Figueiredo EB, Panosso AR, Reicosky DC, La Scala N. Short-term CO2-C emissions from soil prior to sugarcane (
14. Paula DT, Martins Filho MV, Farias VLD, Siqueira DS. Clay and phosphorus losses by erosion in oxisol with sugarcane residues. Eng Agríc 2016;36:1063-72.
15. Satiro LS, Cherubin MR, Safanelli JL, et al. Sugarcane straw removal effects on Ultisols and Oxisols in south-central Brazil. Geoderma Reg 2017;11:86-95.
16. Corradi MM, Panosso AR, Martins Filho MV, La Scala Junior N. Crop residues on short-term CO2 emissions in sugarcane production areas. Eng Agríc 2013;33:699-708.
17. Neto M, Galdos MV, Feigl BJ, Cerri CEP, Cerri CC. Direct N2O emission factors for synthetic N-fertilizer and organic residues applied on sugarcane for bioethanol production in Central-Southern Brazil. GCB Bioenergy 2016;8:269-80.
18. Figueiredo EB, La Scala N. Greenhouse gas balance due to the conversion of sugarcane areas from burned to green harvest in Brazil. Agric Ecosyst Environ 2011;141:77-85.
19. Rolim GDS, Camargo MBPD, Lania DG, Moraes JFLD. Classificação climática de Köppen e de Thornthwaite e sua aplicabilidade na determinação de zonas agroclimáticas para o estado de são Paulo. Bragantia 2007;66:711-20.
20. IPCC. The physical science basis. contribution of working group I to the fifth assessment report of the intergovernmental panel on climate change. Stocker TF, Qin D, Plattner GK, et al. (Ed.). Available from: https://www.ipcc.ch/report/ar5/wg1/ [Last accessed on 15 Feb 2023].
21. Raij BV, Quaggio JA, Cantarella H, et al. Análise química do solo para fins de fertilidade. Cargill F, editor; 1987, 170p. Available from: https://www.iac.sp.gov.br/produtoseservicos/analisedosolo/livro.php [Last accessed on 15 Feb 2023].
22. EMBRAPA, Centro nacional de pesquisa de solos. Manual de métodos de análise de solo. Brasília, Brazil: Ministério da Agricultura e do Abastecimento/EMBRAPA-CNPS; 1997. Available from: https://ainfo.cnptia.embrapa.br/digital/bitstream/item/169149/1/Manual-de-metodos-de-analise-de-solo-2-ed-1997.pdf [Last accessed on 15 Feb 2023].
23. Gonzaga LC, Zotelli LDC, de Castro SGQ, et al. Implications of sugarcane straw removal for soil greenhouse gas emissions in São Paulo State, Brazil. Bioenergy Res 2019;12:843-57.
24. IPCC. Chapter 2 generic methodologies. In: Guidelines for national green house gas inventories, prepared by the national greenhouse gas inventories programme. Eggleston LHS, Buendia K, Miwa T, et al. (Eds.). Japan: IGES; 2006.
25. Franco HCJ, Pimenta MTB, Carvalho JLN, et al. Assessment of sugarcane trash for agronomic and energy purposes in Brazil. Sci Agric 2013;70:305-12.
26. Oliveira DMS, Williams S, Cerri CEP, Paustian K. Predicting soil C changes over sugarcane expansion in Brazil using the DayCent model. GCB Bioenergy 2017;9:1436-46.
27. Gonzaga LC, Carvalho JLN, Oliveira BGD, Soares JR, Cantarella H. Crop residue removal and nitrification inhibitor application as strategies to mitigate N2O emissions in sugarcane fields. Biomass Bioenergy 2018;119:206-16.
28. Vasconcelos ALS, Cherubin MR, Feigl BJ, Cerri CE, Gmach MR, Siqueira-neto M. Greenhouse gas emission responses to sugarcane straw removal. Biomass Bioenergy 2018;113:15-21.
29. CETESB. Emissões veiculares no estado de são paulo. 2014. Available from: https://cetesb.sp.gov.br/veicular/wp-content/uploads/sites/6/2013/12/Relat%C3%B3rio-emiss%C3%B5es-veiculares-2014_VERS%C3%83O-DIGITAL_01.09.pdf [Last accessed on 15 Feb 2023].
30. Macedo IC, Seabra JE, Silva JE. Green house gases emissions in the production and use of ethanol from sugarcane in Brazil: The 2005/2006 averages and a prediction for 2020. Biomass Bioenergy 2008;32:582-95.
31. Dantas GA, Legey LF, Mazzone A. Energy from sugarcane bagasse in Brazil: An assessment of the productivity and cost of different technological routes. Renew Sustain Energy Rev 2013;21:356-64.
32. Leal MRL, Galdos MV, Scarpare FV, Seabra JE, Walter A, Oliveira CO. Sugarcane straw availability, quality, recovery and energy use: A literature review. Biomass Bioenergy 2013;53:11-9.
33. Carvalho DJ, Veiga JPS, Bizzo WA. Analysis of energy consumption in three systems for collecting sugarcane straw for use in power generation. Energy 2017;119:178-87.
34. Milanez AY. De promessa a realidade: como o etanol celulósico pode revolucionar a indústria da cana-de-açúcar: Uma avaliação do potencial competitivo e sugestões de política pública. BNDES; 2015. pp. 237-94.
35. Rosa LP, Ribeiro SK. Avoiding emissions of carbon dioxide through the use of fuels derived from sugar cane. Ambio 1998;27:465-70. Available from: http://www.jstor.org/stable/4314770 [Last accessed on 15 Feb 2023]
36. Harkiolakis N. In: Idowu SO, Capaldi N, Zu L, Gupta AD, editors. Encyclopedia of corporate social responsibility. Berlin: Springer; 2013. pp. 309-13.
37. MCTI. Emissões corporativas. ministério da ciência, tecnologia e inovação, Brazil; 2019. Available from: https://www.gov.br/mcti/pt-br/acompanhe-o-mcti/cgcl/clima/paginas/fator-medio-inventarios-corporativos [Last accessed on 15 Feb 2023].
38. Seabra JE, Tao L, Chum HL, Macedo IC. A techno-economic evaluation of the effects of centralized cellulosic ethanol and co-products refinery options with sugarcane mill clustering. Biomass Bioenergy 2010;34:1065-78.
39. Cavalett O, Chagas MF, Junqueira TL, Watanabe MD, Bonomi A. Environmental impacts of technology learning curve for cellulosic ethanol in Brazil. Ind Crops Prod 2017;106:31-9.
40. Vor T, Dyckmans J, Loftfield N, Beese F, Flessa H. Aeration effects on CO2, N2O, and CH4 emission and leachate composition of a forest soil. J Plant Nutr Soil Sci 2003;166:39-45.
41. Liska AJ, Yang H, Milner M, et al. Biofuels from crop residue can reduce soil carbon and increase CO2 emissions. Nat Clim Chang 2014;4:398-401.
42. Carmo JBD, Filoso S, Zotelli LC, et al. Infield greenhouse gas emissions from sugarcane soils in Brazil: effects from synthetic and organic fertilizer application and crop trash accumulation. GCB Bioenergy 2013;5:267-80.
43. Pitombo LM, Carmo JB, Hollander M, et al. Exploring soil microbial 16S rRNA sequence data to increase carbon yield and nitrogen efficiency of a bioenergy crop. GCB Bioenergy 2016;8:867-79.
44. Adler PR, Del Grosso SJ, Parton WJ. Life-cycle assessment of net greenhouse-gas flux for bioenergy cropping systems. Ecol Appl 2007;17:675-91.
45. Crutzen PJ, Mosier AR, Smith KA, Winiwarter W. N2O release from agro-biofuel production negates global warming reduction by replacing fossil fuels. Atmos Chem Phys 2008;8:389-95.
46. Firestone MK, Davidson EA. Microbiological basis of no and N2O production and consumption in soilsIn: Andreae MO, Schimel DS, editors, Exchange of trace gases between terrestrial ecosystems and the atmosphere. Chichester: John Wiley and Sons; 1989.
Cite This Article
Export citation file: BibTeX | EndNote | RIS
OAE Style
de Figueiredo EB, Jayasundara S, de Oliveira Bordonal R, Rodrigo Panosso A, La Scala Jr N. Greenhouse gas emissions and offset potential from sugarcane straw for bioenergy production in Brazil. Carbon Footprints 2023;2:9. http://dx.doi.org/10.20517/cf.2022.21
AMA Style
de Figueiredo EB, Jayasundara S, de Oliveira Bordonal R, Rodrigo Panosso A, La Scala Jr N. Greenhouse gas emissions and offset potential from sugarcane straw for bioenergy production in Brazil. Carbon Footprints. 2023; 2(2): 9. http://dx.doi.org/10.20517/cf.2022.21
Chicago/Turabian Style
Eduardo Barretto de Figueiredo, Susantha Jayasundara, Ricardo de Oliveira Bordonal, Alan Rodrigo Panosso, Newton La Scala Jr. 2023. "Greenhouse gas emissions and offset potential from sugarcane straw for bioenergy production in Brazil" Carbon Footprints. 2, no.2: 9. http://dx.doi.org/10.20517/cf.2022.21
ACS Style
de Figueiredo, EB.; Jayasundara S.; de Oliveira Bordonal R.; Rodrigo Panosso A.; La Scala Jr N. Greenhouse gas emissions and offset potential from sugarcane straw for bioenergy production in Brazil. Carbon. Footprints. 2023, 2, 9. http://dx.doi.org/10.20517/cf.2022.21
About This Article
Copyright
Data & Comments
Data
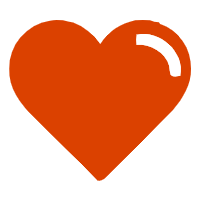
Comments
Comments must be written in English. Spam, offensive content, impersonation, and private information will not be permitted. If any comment is reported and identified as inappropriate content by OAE staff, the comment will be removed without notice. If you have any queries or need any help, please contact us at support@oaepublish.com.