Development of robotic surgical devices and its application in colorectal surgery
Abstract
Due to greater accessibility, robotic technology is becoming increasingly common in a wide range of general surgical procedures. In relation to traditional laparoscopic techniques, robotic surgery has four significant advantages: greater accessibility, visibility, accuracy, and comfortability. For example, robotic surgery is particularly beneficial for procedures in hard-to-reach areas (e.g., pelvis, rectum, and endoluminal areas) because it offers a greater range of motion and precision. In addition, surgical visibility and accuracy can be improved by providing 3D and magnified visualization of the surgical field. Robotic systems are also designed for surgeons’ comfort, allowing the operator to sit while working. For these reasons, robotic surgery is preferable for colorectal and other abdominal surgical procedures. As interest in minimally invasive surgery increases, so does the need to develop new approaches and procedures in colorectal surgery. While robotic surgery has great potential for improving outcomes, there may be disadvantages over traditional laparoscopic and open surgical procedures. For example, possible disadvantages include increased maintenance, training, and cost. This review discusses the evolution of robot-assisted surgery with respect to short-term and long-term outcomes. The development of robotic surgical devices, the new devices entering the market, and the possible future directions of robotic surgery will also be discussed.
Keywords
INTRODUCTION
Surgical procedures that utilize robotic technology are becoming more prevalent[1]. This uptrend has been partially driven by greater accessibility to robotic technology; moreover, a greater number of hospitals have been adopting robotic surgical systems. In comparison to 2021, for instance, the worldwide number of da Vinci® (Intuitive Surgical, Sunnyvale, CA, USA) procedures grew by approximately 18% in 2022[2].
Robotic surgery has significant advantages over laparoscopic approaches with straight instruments. Given the practical and visual benefits conferred by robotic surgery, it is preferred when performing colorectal and abdominal surgical procedures. Notably, it is advantageous when performing surgery in relatively difficult-to-reach areas (e.g., pelvis). Moreover, ergonomics is prioritized because the surgeon is able to operate the device while seated.
As interest in minimally invasive surgery increases, so does the need to develop new approaches and procedures in colorectal surgery. As robot-assisted colorectal surgery continues to evolve, it will become a more suitable option for a variety of surgical approaches. Currently, robot-assisted platforms are used when performing transabdominal, trans-anal, and endoluminal surgeries. During these surgeries, a variety of surgical techniques may be employed: dissection, resection, and anastomosis. It is particularly advantageous in confined spaces such as the rectum and endoluminal areas. Most importantly, robot-assisted colorectal surgery has the potential to improve patient outcomes[3-5].
While robotic surgery has great potential for improving outcomes, possible disadvantages over traditional laparoscopy and open surgery are still being debated. For example, there remain additional maintenance and training requirements; moreover, robotic surgery is costly[6]. This review discusses the evolution of robot-assisted surgery with respect to short- and long-term outcomes. We aim to bridge this gap by offering an all-encompassing insight into the subject. By collating and analyzing existing literature in conjunction with emerging technologies and practices, this review seeks to provide a clearer picture for those invested in the future of colorectal surgery.
SHORT-TERM OUTCOMES
The preponderance of studies have investigated the short-term outcomes (e.g., efficiency and reliability) of robotic surgery. Recent studies show that short-term outcomes are similar or superior to laparoscopic and open surgical approaches; more recently, robust data indicate that robotic surgery is safe and effective[7].
Initially, robotic surgery was not considered superior to laparoscopic surgery when laparoscopic surgery was the standard of care[8,9]. With respect to operation time, there were disparate findings between the distinct surgical techniques. In comparison to open and laparoscopic techniques, operative time was either longer or similar for robotic colorectal surgery. For example, one study found that robotic-assisted surgery for lower rectal cancers increased operative time by 21.6 min[10]. However, this isolated finding was not substantiated by a meta-analysis; the aggregated data noted that operative time was not significantly different between robotic and conventional laparoscopic surgery in the surgical treatment of rectal cancers[11]. Similarly, Baik et al. found that the operative time for mesorectal excision was similar to laparoscopy[12]. Typically, docking the patient cart is one of the time-consuming phases of robotic rectal surgery[13]. Operation time is further prolonged when multiple dockings are needed. However, with the introduction of new platforms and strategic port placement, the approach now encompasses multiple quadrants without the need for multiple dockings. Additionally, with rapid assimilation of surgical techniques by surgeons, there has been a notable reduction in operation durations. At the outset, surgeons were determining the optimal strategies for docking and executing the surgery; moreover, earlier platforms were engineered to function in a singular quadrant and remained stationary once docked, which further elongated operation time. As the surgical teams gained experience, both operative and docking times progressively reduced[13,14]. Indeed, more recent studies show that robotic surgery has a shorter operative time, a shorter length of stay, and a lower conversion rate in comparison to open surgery[14]. Furthermore, they show a progressive decrease in operative time in uncomplicated patients who are undergoing robotic surgery for the resection of malignant and benign tumors[15,16].
In addition to assessing safety and feasibility, assessing conversion rates and length of stays is crucial. Conversion rates, in particular, are predictive of longer hospital stays, higher complication rates, and higher recurrence rates. For example, the ROLARR trial demonstrated that conversion rates were lower than with laparoscopic surgery, especially in obese and male patients[3,4,17]. Similarly, another randomized controlled study demonstrated that robotic surgery decreased length of stays and conversion rates compared to laparoscopic surgery[18]. However, a post-hoc analysis revealed that statistical significance was achieved because low intraperitoneal pressure was used. In addition, this study included patients with colon cancer, not rectal cancer, unlike the ROLARR study. Although this study was conducted at a high-volume colorectal cancer center, the number of patients in this study was lower than in the ROLARR study. Another advantage of robot-assisted surgery is that it has better short-term outcomes when rectal cancer surgery is performed; this advantage is particularly evident among men; likewise, this advantage is conferred when there is a diagnosis of obesity or when there is medical history of radiation therapy; finally, short-term outcomes are improved when rectal tumors are inferiorly located[19,20]. This may be due to instrument articulation and improved operator dexterity and comfort, which can lead to reduced operating times and lower conversion rates. Additionally, the conversion rate is lower in managing complicated diverticulitis, which makes robotic surgery an appropriate option[21]. Nonetheless, we still believe robotic surgery can be associated with slightly longer operative times. However, improved efficiency and shortening of these durations can be achieved by gaining experience and building up teams.
There are three other pertinent short-term outcomes: 30-day morbidity, estimated blood loss, and gut function. The REAL trial demonstrated that the robotic group had better postoperative gastrointestinal recovery, shorter postoperative hospital stays, lower estimated blood loss, and fewer intraoperative complications than patients in the laparoscopic group[22]. Additionally, Waters et al. revealed that overall morbidity and 30-day mortality were comparable for both approaches. Nevertheless, the patients within the robotic group experienced fewer anastomotic complications, increased lymph node harvest, shorter hospital stays, lower conversion rates to open surgery, and decreased incisional hernia rates than those undergoing the laparoscopic approach in right hemicolectomy[23]. Similarly, a randomized controlled trial by Kim et al. demonstrated that robotic rectal cancer surgery had similar postoperative morbidity, bowel function recovery, and quality of life compared to laparoscopic surgery[4]. On the other hand, Bianchi et al. found that the overall 30-day complication rate was 16.1%, while major postoperative complications occurred in 3.7% of patients with a low intracorporeal anastomotic leak rate[24]. In a meta-analysis of seven randomized controlled trials, anastomotic leak rates were comparable between intracorporeal and extracorporeal anastomosis techniques in robotic right hemicolectomy[25]. Robotic intracorporeal anastomosis is a safe and effective technique with increasing evidence, such as faster gastrointestinal recovery, decreased hospital stays, and postoperative hernia rates for both right and left-sided colon resections[26-28].
Robotic approaches can provide higher rates of intersphincteric resections compared to the open approach, with a significantly lower anastomotic level[29]. Robotic intersphincteric resection ensures adequate surgical margins, making it a safe, anus-preserving alternative to abdominoperineal resection. However, some patients may experience significant bowel and genitourinary dysfunction post-procedure, which can affect their quality of life[30].
Over the years, there have been improvements in the short-term outcomes of robotic colorectal surgery. However, there remain areas in which further developments are anticipated.
ONCOLOGICAL OUTCOMES
From an oncological standpoint, some requisite steps must be completed before the operation is successfully completed. Firstly, achieving a negative surgical margin and acceptable lymph node harvest is paramount[31]. The American Joint Committee on Cancer recommends that a minimum of 12 lymph nodes be removed when colorectal surgery is performed[32]. Studies demonstrate no significant difference in the number of harvested lymph nodes with various surgical approaches in rectal cancers[33,34]. However, there is some early evidence that lymph node harvest is superior in colectomies[35]. The COLOR II study showed that the rates of positive surgical margins were similar for laparoscopic surgery and open surgery[36]. Additionally, recent high-profile trials, ALaCaRT and ACOSOG Z6051, failed to show non-inferiority of laparoscopy to open proctectomy[37,38]. A recent randomized controlled trial published in the Annals of Surgery revealed that robotic-assisted surgery did not significantly improve the total mesorectal excision (TME) quality compared with conventional laparoscopic surgery regarding circumferential resection margin and the harvested lymph nodes[39]. Robotic platforms may allow for more precise dissection, better identification, and preservation of small structures in the pelvic cavity. In confined spaces, negative circumferential resection margins and improved lymphadenectomy can be achieved with the advantage of articulating instruments[40]. Thus, this approach can potentially reduce local recurrence and improve survival rates in patients with rectal cancer. However, as demonstrated by the most comprehensive randomized controlled trial conducted to date, the ROLARR study, there has been no validation of the lymph node harvest and resection margin benefits of robotic surgery[3].
Although long-term survival after robotic colorectal surgery has not yet been fully confirmed, the limited data suggest that survival rates are generally similar to those of laparoscopic and open surgery[15]. The correlation between robotic surgical systems and improvements of the survival rate in colorectal cancer patients remains controversial. A retrospective two-center study by Feroci et al. evaluated three-year overall survival rates and disease-free survival rates of patients with middle and lower rectal cancers[41]. The oncologic outcomes were not significantly different between laparoscopic and robotic approaches. Likewise, Cho et al. found no difference in five-year overall survival rates between the two surgical approaches in a case-match study[42]. Another study from a high-volume center reported a lower five-year overall survival rate in the laparoscopic group, although the difference was insignificant[43]. A recent study compared 101 patients undergoing robotic complete mesocolic excision to the same number of patients undergoing laparoscopic resection for the right-sided colon tumors[44]. After the analysis of the prospectively maintained database, five-year overall and disease-free survival rates were higher in the robotic group, although not significant. Only a few studies, such as by Mirkin et al., suggest that robotic surgery increases survival in stage II and III colon cancer[45]. However, this retrospective study was conducted using a national database and does not provide information on chemotherapy regimens, quality of life, and disease-specific recurrence. Additionally, this database lacks surgeon-specific data to differentiate between novice and expert robotic surgeons. In contrast, in a retrospective single-center study, long-term survival was observed in the laparoscopic group for left-sided colon cancers, according to Xu et al.[46]. However, this was attributed to the fact that the laparoscopic group included a larger number of patients in the early stages. In summary, while the long-term oncological benefits of robotic surgery for rectal and colon cancers have not been demonstrated, some short-term advantages are bestowed by robotic surgery. For example, the advantages of colon surgery are primarily related to short-term outcomes such as shorter hospital stays, lower estimated blood loss, and faster return of bowel functions; additionally, more lymph nodes are removed[44,47,48].
Regardless of the surgical technique, careful consideration must be given to the patient’s anatomy. However, when performing rectal surgery, the robotic approach is preferred for patients with a narrow pelvis; notably, the robotic approach has practical advantages over other techniques (e.g., comfort and dexterity).
ADVANCES IN ROBOTIC DEVICES
Robotic surgical devices were first developed for neurosurgery and orthopedics. For example, the PUMA 200 (Westinghouse Electric, Pittsburgh, PA, USA) was used for needle placement in a CT-guided brain biopsy[49]. In contrast, the ROBODOC® surgical system (Integrated Surgical Systems, Inc., Sacramento, CA, USA) was used for total hip arthroplasty[50]. The integration of robotic surgical devices into abdominal surgery was driven by two factors: the advent of laparoscopic surgery and the heightened interest in minimally invasive surgery. There are two notable robotic surgical systems that are used for abdominal surgery: the AESOP® (Computer Motion, Inc., Goleta, CA, USA) and the ZEUS® (Computer Motion, Inc., Goleta, CA, USA). While the AESOP®, a robotic camera, is controlled by voice commands, the ZEUS®, a three-arm robotic device, is mechanically controlled[49,51,52]. Unfortunately, the early adoption of these systems in colorectal surgery was low, and many were not fully automated[53]. The monumental shift occurred when the da Vinci® surgical system was developed. In the year 2002, robotic systems gained popularity when the first colorectal surgery was performed[54].
In the year 2014, a new da Vinci® platform, da Vinci Xi® (Intuitive Surgical, Sunnyvale, CA, USA), was launched. This newer model possesses more advanced features than the previous model, da Vinci Si® (Intuitive Surgical, Sunnyvale, CA, USA). For instance, the working space was limited with da Vinci Si® because the patients remain in a fixed position once they are docked. However, the maneuverability of the da Vinci Xi® system may be superior to that of its predecessor[55]. The da Vinci Xi® platform has a novel overhead architecture that combines a boom-mounted system with a flexible and mobile platform[56,57]. Thus, the da Vinci Xi® surgical system has a significant advantage over the da Vinci Si® platform due to its ability to perform multi-quadrant surgeries, such as rectal surgery, without requiring a second docking procedure for splenic flexure takedown[58]. In doing so, this beneficial functionality saves time and makes it possible to perform multi-quadrant surgeries. Even though the splenic flexure is easier to mobilize using the da Vinci Xi® system[59], it remains complex and challenging. Some centers prefer double-docking, but it can be time-consuming. To overcome this barrier, the operation table can be tilted 30 degrees Trendelenburg and slightly to the right to facilitate mobilization[55]. In our practice, we move the standard port placement line 15-20 degrees counterclockwise and prefer a cross-armed single-docking approach [Figure 1]. A tip-up fenestrated grasper inserted through the port number one retracts the descending colon to the medial and inferior toward the caecum [Figure 2A]. Afterward, we cross the arms either from the lateral aspect of arm one and take down the flexure without sword fighting [Figure 2B]. After the lateral side dissection is completed, we change the position of the instruments to mobilize the transverse colon. This time, the tip-up grasping instrument is used to retract the colon through the left lower quadrant, which enables us to work in the medial aspect of the grasping instrument [Figure 2C]. This method allows us to work freely without port number one (typically tip-up fenestrated grasper) running into restricted mobility. Additionally, the single-docking approach saves time.
Figure 2. (A) Traction of descending colon; (B) Medial aspect of arm one; (C) Lateral aspect of arm one.
A dual robotic console refers to a sophisticated setup in robotic surgery where two separate surgeon consoles are integrated into a single robotic surgical system, allowing for simultaneous active engagement by two surgeons. Systems such as the da Vinci Surgical System by Intuitive Surgical have championed this approach, especially valued in the realm of collaborative surgeries and academic settings. This dual setup not only facilitates a seamless melding of expertise during intricate surgeries but also serves as an invaluable tool for training and mentorship. In an educational context, trainees can operate under the watchful eye of seasoned surgeons, benefiting from real-time guidance. Furthermore, in extended surgical procedures, the presence of two consoles allows surgeons to alternate, potentially reducing fatigue. A pivotal advantage is the ability to swiftly switch control during challenging surgical steps, enhancing both procedure efficiency and patient safety. In essence, the integration of a dual console in robotic surgery augments its versatility and opens avenues for collaborative and educational advancements. The dual console system further demonstrates its prowess in multispecialty operations, enabling a seamless transition of control between specialists from different disciplines[60]. For instance, in complex cases such as pelvic endometriosis or T4 low pelvic tumors, a colorectal surgeon and a urologist can collaboratively operate, each taking control at different stages in the procedure. This shared vision and control of the surgical field is a unique advantage of the dual console, offering an integration that is unparalleled in open and laparoscopic approaches.
Recently, additional refinements have been made to the new da Vinci Xi® system. The TruSystem® 7000dV (Trumpf Medizin Systeme GmbH & Co. KG, Saalfeld, Germany) is designed to interact wirelessly with the da Vinci Xi® surgical system and consists of a moving surgical table that reaches multiple quadrants[61]. In doing so, accessibility and range of motion were improved. Robotic surgery with table motion systems is safe and effective because it eliminates docking and redocking, allowing the procedure to continue without interruptions. Therefore, there is some anecdotal evidence that this system reduces operative time. The most crucial advantage is providing multi-quadrant operations in colorectal surgery[62].
The Single-Site® (Intuitive Surgical, Sunnyvale, CA, USA) platform, a software that repositions the two curved instruments, is another advancement that is relevant to robotic devices. The operation is performed through a single incision using the da Vinci Si® or da Vinci Xi® systems. Initially, single-site approaches were attempted using standard ports in conventional laparoscopy, but the proximity of the instruments limited the range of motion. The problem was generally believed to be caused by the straight nature of the trocars used in the standard single-site approach. However, the Single-Site® platform with curved and crossed-shaped trocars and silicone port overcomes the limitations of the standard laparoscopic single-site approach. The software allows the use of crossed trocars to adjust them to the correct position for the surgeon. Moreover, semi-flexible instruments increase the range of motion and articulation. Whereas using curved trocars and semi-flexible instruments has improved the range of motion, there are some hindrances. For instance, this system cannot use non-flexible instruments such as EndoWrist® (Intuitive Surgical, Sunnyvale, CA, USA) instruments and surgical energy devices. However, monopolar and bipolar coagulation systems can be used, and dissection and coagulation can be performed with scissors and fenestrated forceps. Depending on the surgeon’s preference, these instruments may need to be used for colorectal surgery. In this manner, developing this technology is necessary for using non-flexible instruments. Because of these technical difficulties, an additional port for non-flexible instruments can make the procedure more feasible[63]. It has been presented that additional ports facilitate TME[64]. The incision where the additional port is placed can also be used as a pelvic drain entry point for surgeons who prefer it. Another disadvantage of the Single-Site® platform is that although cosmetically pleasing, the existing incision is longer[65], with reported incision lengths of up to 4 cm[66]. Additionally, a single-site robotic approach is associated with a high risk of incisional hernia[67]. Furthermore, the Single-Site® platform makes it more challenging to use the assistant’s third instrument[68]. In addition, extra training is required for docking. The operative time may be longer because the docking procedure differs from previous robotic surgical systems. With experience, this time may be reduced to satisfactory levels.
Da Vinci SP® surgical system
The da Vinci SP® surgical system (Intuitive Surgical, Sunnyvale, CA, USA) is designed for single-port surgery. It has a single arm containing a shaft with three multi-jointed, fully articulated instruments and a jointed high-resolution camera. It is inserted through a 25 mm trocar to reach the target organ. The system is specifically designed to operate in narrow, hard-to-reach areas, such as anorectal surgery, prostatectomy, and tonsillectomy. One of the most important features is its 360-degree boom movement, which allows fully multi-quadrant operations in colorectal surgery. The single arm of the unit allows for flexible positioning of the patient cart in the operating room. A further advantage is that the single shaft makes the docking process easier to learn and quicker to perform[69]. The use of instruments within the same shaft prevents external collisions during surgery. The instruments are bent laterally to separate during surgery, creating a triangulation. The instruments are then turned inward to focus on the surgical site. In the series by Piozzi
The da Vinci SP® surgical system features on-screen holograms that show the positions and movements of instruments, enhancing the surgeon’s orientation. The system offers significant improvements over traditional single-incision laparoscopy and single-site robotic surgery, with enhanced vision, exposure, and range of motion. The advantages of this system over previous platforms include superior ergonomics and the opportunity for the operator to complete a series of procedures with reduced fatigue. The single-port system offers cosmetic benefits and less postoperative pain[71].
However, some devices and instruments are not integrated with the single port system. Surgical energy devices and the EndoWrist® instruments cannot be used with the single port system as with the Single-Site® platform. The shaft has no suction irrigation, but alternative external suction irrigation devices are currently on the market. Additionally, the grasping and traction force of the grasper is weaker than that of previous models, which limits the use of the grasper in major surgery[72]. Furthermore, the collaboration between the surgeon and an assistant underscores the vital role of teamwork in the procedure’s success and safety. For colorectal surgery, an additional port outside the main access is sometimes required for the assistant, which is called the “reduced port approach”.
Pioneering studies have demonstrated the broad application of the da Vinci SP® Surgical System in colorectal surgery, including right hemicolectomy, inter-sphincteric resection, and mid-transverse colectomy[69-71,73-75]. Its flexible, subtle, and lightweight platform can also be used for trans-anal and endoluminal surgery[76,77]. In addition to cancer, a single-port system is also used for benign lesions or procedures such as trans-anal mucosal rectal graft for urethroplasty[78]. We believe the single-port surgical system in colorectal surgery will increase in the near future.
New devices entering the market
Technological advances in minimally invasive surgery spurred interest in robotic surgical systems. More than 5,000 computer-assisted systems have been developed for robotic surgery, but only a few have been approved and made available for clinical use[79].
Revo-i robotic surgical solution (Meere Company Inc., Hwaseong-si, Republic of Korea) is a robotic platform with one control unit and four robotic arms; there are seven degrees of freedom. It was approved by the Korean Ministry of Food and Drug Safety in 2018[80]. Revo-i has been tested in pre-clinical and clinical studies in urology, gynecology, and hepatobiliary surgery[81-83]. The advantage over the da Vinci® surgical system is the haptic feedback. It has been launched in Korea, Russia, Kazakhstan, and Uzbekistan. Another platform with a similar design is the avatera® system (Avateramedical GmbH, Jena, Germany). Pre-clinical studies in Europe have shown it to be safe and feasible for urological surgery[84,85]. The hinotori™ surgical robot system (Medicaroid Corporation, Kobe, Japan) is another four-arm system. The monitor and control units are separate. The system consists of three parts, including the robotic arms. The robotic arms have a docking-free design[86]. It has been reported to be used for gastrectomy and rectal resection in pigs[86,87].
The Senhance® Surgical System (Asensus Surgical, North Carolina, USA) has two to four separate robotic arms and control units. The modular design and lightweight robotic arms of the system provide greater flexibility and range of motion. The robotic arms can be in various positions for different surgical procedures. The remote-control unit of the Senhance® Surgical System is designed in an open console format, which improves ergonomics and communication with the surgical team. The system has eye-tracking technology that reduces fatigue and improves focus by allowing the surgeon to control the camera unit with eye movements. Moreover, the system’s robotic arms provide haptic feedback to enhance precision. While the Senhance® Surgical System employs laparoscopic hand-helds with non-articulated end-effectors, which can be seen as a limitation, the presence of 3 mm and 5 mm instruments offers a significant advantage in terms of surgical versatility. It also significantly reduces costs by enabling instrument reuse. It is used for all abdominal surgery in Europe and has been used for colorectal surgery since 2017[88]. The Senhance® Surgical System is safe and feasible, with low conversion rates and reasonable docking times for various colorectal procedures[89,90]. Another modular robotic system is the Versius® Surgical Robotic System (CMR Surgical, Cambridge, UK). The system allows for up to five robotic arms. It has an open console cart with joystick controls that provide ergonomic advantages and haptic feedback. The camera unit is manually controlled with a joystick. This allows surgeons to use the system while standing if desired. The Versius boasts a minimal physical footprint, affording increased maneuverability and flexibility within the surgical suite. Its compact design facilitates more efficient surgical teamwork. Each arm of Versius is approximately 1.5 times the size of a human arm, occupying a compact area of 38 cm × 38 cm. The first studies demonstrating the safety and feasibility of the Versius® Surgical Robotic System were conducted in the United Kingdom for colorectal surgery[91] and are now being used in the Middle East, Europe, India, and Australia. The Senhance® Surgical System and the Versius® Surgical Robotic System are approved by the Food and Drug Administration (FDA), including da Vinci® systems. The SSi Mantra (SS Innovations International from Fort Lauderdale, FL, USA) is another modular robotic system. It features three to five independent, flexible robotic arms and an open-face console design that enhances surgeon ergonomics. The distinct modular arms allow for versatile patient docking, and they come with stable parking locks for assured steadiness. To further improve safety and efficiency during surgical procedures, the system incorporates machine learning models.
Modular patient carts offer a higher degree of flexibility and customization, as they allow surgeons to select specific tools and instruments tailored to particular procedures. They can be upgraded individually, potentially making them more cost-effective in the long run. Moreover, their design might provide operational continuity even if one module fails. However, they introduce an element of complexity to the surgical setup, might pose integration issues, and typically demand more space. On the other hand, a single patient cart is often more streamlined, making it easier for surgical teams to learn and operate. Its design provides consistency in performance and is usually more space-efficient. Nevertheless, it might offer limited customization options and can be a challenge if updates or changes to the system are required, as the entire cart may need revision.
Hugo™ Robotic-Assisted Surgery (Medtronic PLC, Dublin, Ireland) is another modular platform. This system is approved in Europe and has been used for gynecologic and urologic procedures since March 2022. Pre-clinical cadaver studies have reported right and left hemicolectomies[92]. FDA approval is pending. The Bitrack system (Rob Surgical, Barcelona, Spain) is one of the modular robotic surgical systems. It consists of three robotic arms and an open surgical console. The robotic arms are mounted on a flexible floating base designed to increase surgical accessibility and reach in various positions. It is advantageous due to its smaller size. Pre-clinical studies are underway for the Bitrack system. Another robotic surgery platform is the DLR MIRO (DLR Institute of Robotics and Mechatronics, Wessling, Germany), a robotic console with three to five separate arms. The arms are an integral part of the operating table, and no separate patient cart exists. As a result, it takes up less space in the operating room. It can be used in different combinations. Another advantage of the DLR MIRO is the haptic feedback demonstrated by force vectors on the monitor. Pre-clinical studies are underway. The Dexter robot (Distalmotion, Epalinges, Switzerland) consists of two instrument arms and a camera arm. The first radical prostatectomy was performed with the Dexter robot, but no colorectal studies have been reported[93].
The MIRA® (Miniaturized In Vivo Robotic Assistant) platform (Virtual Incision, Lincoln, NE, USA) is a miniaturized robotic-assisted surgical system. It is small and weighs approximately two pounds. This makes it easy to transport and requires less space than other robotic systems. The MIRA® platform has two instruments on a single shaft and an integrated camera. It can be integrated into the operating table and does not require a separate drape. The first right hemicolectomy was reported using the MIRA® platform[94].
With the recognition of the importance of colorectal cancer screening and early treatment, the endoluminal area is another area of focus for robotic systems. The Invendoscopy™ E210 system (Ambu, Ballerup, Denmark) and the Neoguide™ Endoscopy system (Intuitive Surgical Inc., Sunnyvale, CA, USA) are flexible, self-propelled robotic colonoscopes that are connected to the scope control unit. Both systems apply less force to the colon walls, resulting in less pain and allowing the procedure to be performed without sedation. Clinical trials have shown them to be safe and feasible[95]. The Flex® Robotic System (Medrobotics Corp., Raynham, MA, USA) is a flexible, highly articulated platform integrated into the operating table. It is controlled with a joystick from the on-screen control unit. The FDA-approved device is used for transoral and trans-anal procedures. The STRAS system (i-cube, Strasbourg, France) is a modular flexible endoscopy system suitable for endoluminal procedures. It features a flexible endoscope and instruments that provide ten degrees of freedom. The system is smaller than other laparo-endoscopic systems, which offers advantages in both setup time and cost. It has been used in pre-clinical studies for endoluminal submucosal dissection[96]. The EndoLuminal Surgical System (ELS) (ColubrisMX, Houston, TX, USA) is designed explicitly for trans-anal and endoluminal procedures. It appears to be suitable for endoluminal excision and suturing[97]. While robots for endoluminal surgery have been developed, they are not yet utilized in the proximal part of the rectum. However, we anticipate that the development of fully flexible endoluminal platforms will enable surgical procedures such as endoscopic mucosal resection and endoscopic submucosal dissection to be performed robotically throughout the colon [Figure 3].
Technological developments will accelerate as the market becomes competitive. As technology improves and competition intensifies, many new devices and products will be put into clinical use. As a result, robotic surgery is expected to become widespread and improve outcomes.
FUTURE DIRECTIONS OF ROBOTIC SURGERY
Staplers and vessel sealing devices
The limited use of staplers and vessel sealers is a significant drawback of many robotic surgical platforms. The da Vinci® surgical system currently overcomes these shortcomings with the Xi model. The EndoWrist® Stapler and SureForm® Stapler (Intuitive Surgical, Sunnyvale, CA, USA) are available and use SmartClamp Technology to adjust tissue compression and optimize the stapling line. The software monitors the stapler’s movements and provides feedback to the surgeon regarding the percentage of stapling completed. Firing is allowed when 100% clamp completion is achieved; otherwise, repositioning may be required. Studies show that this technology can be helpful with intraoperative decision-making; also, clinical outcomes are improved[98].
The EndoWrist® Stapler, which provides full wrist articulation in horizontal and vertical directions, is controlled by the surgeon through the da Vinci Xi® surgical system. It is available in 30- and 45-mm staple line lengths and features a 108-degree wrist cone. The EndoWrist® Stapler is feasible and safe to use[99]. However, there is an ongoing debate about whether it reduces the number of staplers used during rectal transection[100,101]. On the other hand, the SureForm® Stapler, available in line lengths of 45- and 60-mm, has a 120-degree cone of articulation that allows for easier access to the tissue. Improvements are currently being made to increase the cone of articulation, particularly for patients with a narrow pelvis.
Recent systematic reviews shed light on the potential advantages of robotic staplers in rectal cancer surgeries. Robotic staplers, compared to laparoscopic staplers, have shown a trend towards reduced numbers of firings necessary for rectal transection[102]. This reduction may have implications for decreasing the incidence of anastomotic leakage post-surgery. However, while the data suggests fewer firings and a potential reduction in anastomotic leakage[103], these findings were not statistically significant. Despite the inconclusiveness, the robotic stapler’s precision and improved tissue compression technology can play a pivotal role in optimizing surgical outcomes, especially in patients with narrow pelvises.
The da Vinci Xi® surgical system offers several options for vessel sealing devices, including the EndoWrist® One Vessel Sealer, Endowrist® Vessel Sealer Extend, and SynchroSeal® (Intuitive Surgical, Sunnyvale, CA, USA). With its extended body design, the Vessel Sealer Extend can help seal and cut vessels up to 7 mm in length. Compared to the EndoWrist® One Vessel Sealer, it has a 30% thinner design, allowing for more precise tissue dissection and manipulation. The Synchroseal® can seal and cut vessels up to 5 mm in length. The advantage is a shorter cycle time. The Vessel Sealer Extend and SynchroSeal® models operate at a cooler jaw temperature. However, because these vessel sealers are not flexible, they cannot be used on a Single-Site® platform or integrated into the da Vinci SP® surgical system. Future improvements are expected to address these limitations.
Visual improvements
To improve visual clarity, the AirSeal® intelligent Flow System (iFS) (CONMED, Utica, NY, USA) can remove smoke during surgery, or software on advanced laparoscopic platforms can render cleaner images by reducing visual artifact that is associated with smoke[104]. To further enhance visual clarity, preoperative computed tomography images can be rendered in 3D and transferred to TilePro™ on the da Vinci® surgical system before the operation. The surgeon can re-evaluate these images in real time during the procedure to facilitate surgical planning and improve orientation. In addition, the da Vinci® fluorescence imaging system, Firefly®, is intended for real-time near-infrared fluorescence imaging that can visualize bile ducts, blood vessels, and lymphatic tissue. Indocyanine green dye is used to visualize lymphatic dissection and blood supply of the residual bowel after resection in colorectal surgery[105,106]. The fluorescence imaging system has also been reported to be used in endometriosis surgery[107].
Haptic feedback
There is one principal criticism of remote-controlled robotic platforms: a lack of haptic feedback. Innovations related to direct haptic feedback lag behind visual innovations. Although there is some tactile sensation when laparoscopic surgery is performed, there is no tactile sensation when first-generation robotic surgical platforms are used. However, newer systems provide indirect feedback to the surgeon through on-screen force vectors, vibration feedback, excessive force warnings, and color or sound alerts. Technological advances to improve haptic feedback aims to provide real-time and direct feedback[108,109].
Artificial intelligence
The use of robotic platforms also fosters an opportunity to adapt artificial intelligence. Robotic technology can be used for disease screening, diagnosis, staging, and surgery. The Smart Tissue Autonomous Robot (STAR) (Omniboros Team, Washington, DC, USA), developed for autonomous surgery, has been shown in pre-clinical studies to perform surgery with greater consistency and fewer errors[110]. When used in conjunction with a surgeon, the STAR has been shown to increase incision accuracy and reduce surgeon work time[111]. We believe surgeons’ jobs will be made easier by the development of robotic surgery with artificial intelligence.
CONCLUSION
In conclusion, robotic surgery is a rapidly evolving field that offers many benefits over traditional surgical methods. Robotic platforms enable surgeons to perform procedures with greater precision, dexterity, and flexibility. Additionally, robotic surgery reduces pain and recovery time; therefore, robotic surgery reduces hospital stays and improves clinical outcomes. However, despite its advantages, there are some limitations associated with robotic surgery. For instance, long-term oncologic outcomes remain ill-defined. Moreover, robotic surgery requires specialized training due to the use of incompatible instruments and the lack of haptic feedback. However, ongoing technological advancements and studies are addressing these limitations and opening new possibilities for the future of surgical robotics. From staplers and vessel-sealing devices to visual improvements and artificial intelligence, the future of robotic surgery is promising and has the potential to revolutionize the field of medicine.
DECLARATIONS
Acknowledgments
The authors would like to acknowledge Karim Kheniser for editing the manuscript.
Authors’ contributions
Made substantial contributions to the conception and design of the study, literature review, and writing of the article: Erozkan K
Made substantial contributions to the conception and design of the study and review of the article, provided administrative, technical, and material support: Gorgun E
Availability of data and materials
Not applicable.
Financial support and sponsorship
None.
Conflicts of interest
Dr. Kamil Erozkan declared that there are no conflicts of interest. Dr. Emre Gorgun receives consultancy fees from Boston Scientific, Olympus, DiLumen, and Vascular Technology.
Ethical approval and consent to participate
Not applicable.
Consent for publication
Not applicable.
Copyright
© The Author(s) 2023.
REFERENCES
1. Sheetz KH, Claflin J, Dimick JB. Trends in the adoption of robotic surgery for common surgical procedures. JAMA Netw Open 2020;3:e1918911.
2. Intuitive announces preliminary fourth quarter and full year 2022 results. Available from: https://isrg.intuitive.com/news-releases/news-release-details/intuitive-announces-preliminary-fourth-quarter-and-full-year-2. [Last accessed on 15 Nov 2023].
3. Jayne D, Pigazzi A, Marshall H, et al. Effect of robotic-assisted vs conventional laparoscopic surgery on risk of conversion to open laparotomy among patients undergoing resection for rectal cancer: the ROLARR randomized clinical trial. JAMA 2017;318:1569-80.
4. Kim MJ, Park SC, Park JW, et al. Robot-assisted versus laparoscopic surgery for rectal cancer: a phase II open label prospective randomized controlled trial. Ann Surg 2018;267:243-51.
5. Crippa J, Grass F, Dozois EJ, et al. Robotic surgery for rectal cancer provides advantageous outcomes over laparoscopic approach: results from a large retrospective cohort. Ann Surg 2021;274:e1218-22.
6. Kim CW, Kim CH, Baik SH. Outcomes of robotic-assisted colorectal surgery compared with laparoscopic and open surgery: a systematic review. J Gastrointest Surg 2014;18:816-30.
7. Vilsan J, Maddineni SA, Ahsan N, et al. Open, laparoscopic, and robotic approaches to treat colorectal cancer: a comprehensive review of literature. Cureus 2023;15:e38956.
8. Anderson JE, Chang DC, Parsons JK, Talamini MA. The first national examination of outcomes and trends in robotic surgery in the United States. J Am Coll Surg 2012;215:107-14.
9. Maeso S, Reza M, Mayol JA, et al. Efficacy of the da Vinci surgical system in abdominal surgery compared with that of laparoscopy: a systematic review and meta-analysis. Ann Surg 2010;252:254-62.
10. Fung AKY, Aly EH. Robotic colonic surgery: is it advisable to commence a new learning curve? Dis Colon Rectum 2013;56:786-96.
11. Zhang J, Qi X, Yi F, Cao R, Gao G, Zhang C. Comparison of clinical efficacy and safety between da Vinci robotic and laparoscopic intersphincteric resection for low rectal cancer: a meta-analysis. Front Surg 2021;8:752009.
12. Baik SH, Ko YT, Kang CM, et al. Robotic tumor-specific mesorectal excision of rectal cancer: short-term outcome of a pilot randomized trial. Surg Endosc 2008;22:1601-8.
13. Huang CW, Wei PL, Chen CC, Kuo LJ, Wang JY. Clinical safety and effectiveness of robotic-assisted surgery in patients with rectal cancer: real-world experience over 8 years of multiple institutions with high-volume robotic-assisted surgery. Cancers 2022;14:4175.
14. Konstantinidis IT, Ituarte P, Woo Y, et al. Trends and outcomes of robotic surgery for gastrointestinal (GI) cancers in the USA: maintaining perioperative and oncologic safety. Surg Endosc 2020;34:4932-42.
15. Huang Z, Li T, Zhang G, et al. Comparison of open, laparoscopic, and robotic left colectomy for radical treatment of colon cancer: a retrospective analysis in a consecutive series of 211 patients. World J Surg Oncol 2022;20:345.
16. Bao X, Wang H, Song W, Chen Y, Luo Y. Meta-analysis on current status, efficacy, and safety of laparoscopic and robotic ventral mesh rectopexy for rectal prolapse treatment: can robotic surgery become the gold standard? Int J Colorectal Dis 2021;36:1685-94.
17. Allaix ME, Furnée EJB, Mistrangelo M, Arezzo A, Morino M. Conversion of laparoscopic colorectal resection for cancer: what is the impact on short-term outcomes and survival? World J Gastroenterol 2016;22:8304-13.
18. Fleming CA, Celarier S, Fernandez B, Cauvin T, Célérier B, Denost Q. An analysis of feasibility of robotic colectomy: post hoc analysis of a phase III randomised controlled trial. J Robot Surg 2023;17:1057-63.
19. Keller DS, Flores-Gonzalez JR, Ibarra S, Haas EM. Review of 500 single incision laparoscopic colorectal surgery cases - Lessons learned. World J Gastroenterol 2016;22:659-67.
20. Scarpinata R, Aly EH. Does robotic rectal cancer surgery offer improved early postoperative outcomes? Dis Colon Rectum 2013;56:253-62.
21. Panin SI, Nechay TV, Sazhin AV, et al. Should we encourage the use of robotic technologies in complicated diverticulitis? Results of systematic review and meta-analysis. Front Robot AI 2023;10:1208611.
22. Feng Q, Yuan W, Li T, et al. Robotic versus laparoscopic surgery for middle and low rectal cancer (REAL): short-term outcomes of a multicentre randomised controlled trial. Lancet Gastroenterol Hepatol 2022;7:991-1004.
23. Waters PS, Cheung FP, Peacock O, et al. Successful patient-oriented surgical outcomes in robotic vs laparoscopic right hemicolectomy for cancer - a systematic review. Colorectal Dis 2020;22:488-99.
24. Bianchi PP, Salaj A, Giuliani G, Ferraro L, Formisano G. Feasibility of robotic right colectomy with complete mesocolic excision and intracorporeal anastomosis: short-term outcomes of 161 consecutive patients. Updates Surg 2021;73:1065-72.
25. Zhang T, Sun Y, Mao W. Meta-analysis of randomized controlled trials comparing intracorporeal versus extracorporeal anastomosis in minimally invasive right hemicolectomy: upgrading the level of evidence. Int J Colorectal Dis 2023;38:147.
26. Akram WM, Al-Natour RH, Albright J, et al. A propensity score-matched comparison of intracorporeal and extracorporeal techniques for robotic-assisted right colectomy in an Enhanced Recovery Pathway. Am J Surg 2018;216:1095-100.
27. Al Natour RH, Obias V, Albright J, et al. A propensity score matched comparison of intracorporeal and extracorporeal techniques for robotic-assisted sigmoidectomy in an enhanced recovery pathway. J Robot Surg 2019;13:649-56.
28. Cleary RK, Silviera M, Reidy TJ, et al. Intracorporeal and extracorporeal anastomosis for robotic-assisted and laparoscopic right colectomy: short-term outcomes of a multi-center prospective trial. Surg Endosc 2022;36:4349-58.
29. Kim JC, Lee JL, Alotaibi AM, Yoon YS, Kim CW, Park IJ. Robot-assisted intersphincteric resection facilitates an efficient sphincter-saving in patients with low rectal cancer. Int J Colorectal Dis 2017;32:1137-45.
30. Piozzi GN, Kim SH. Robotic intersphincteric resection for low rectal cancer: technical controversies and a systematic review on the perioperative, oncological, and functional outcomes. Ann Coloproctol 2021;37:351-67.
31. Yang Y, Wang F, Zhang P, et al. Robot-assisted versus conventional laparoscopic surgery for colorectal disease, focusing on rectal cancer: a meta-analysis. Ann Surg Oncol 2012;19:3727-36.
32. Compton CC, Fielding LP, Burgart LJ, et al. Prognostic factors in colorectal cancer: College of American Pathologists consensus statement 1999. Arch Pathol Lab Med 2000;124:979-94.
33. Kim NK, Baik SH, Seong JS, et al. Oncologic outcomes after neoadjuvant chemoradiation followed by curative resection with tumor-specific mesorectal excision for fixed locally advanced rectal cancer: impact of postirradiated pathologic downstaging on local recurrence and survival. Ann Surg 2006;244:1024-30.
34. Trastulli S, Farinella E, Cirocchi R, et al. Robotic resection compared with laparoscopic rectal resection for cancer: systematic review and meta-analysis of short-term outcome. Colorectal Dis 2012;14:e134-56.
35. Zheng J, Zhao S, Chen W, Zhang M, Wu J. Comparison of robotic right colectomy and laparoscopic right colectomy: a systematic review and meta-analysis. Tech Coloproctol 2023;27:521-35.
36. van der Pas MH, Haglind E, Cuesta MA, et al. Laparoscopic versus open surgery for rectal cancer (COLOR II): short-term outcomes of a randomised, phase 3 trial. Lancet Oncol 2013;14:210-8.
37. Stevenson ARL, Solomon MJ, Lumley JW, et al. Effect of laparoscopic-assisted resection vs open resection on pathological outcomes in rectal cancer: the ALaCaRT randomized clinical trial. JAMA 2015;314:1356-63.
38. Fleshman J, Branda M, Sargent DJ, et al. Effect of laparoscopic-assisted resection vs open resection of stage II or III rectal cancer on pathologic outcomes: the ACOSOG Z6051 randomized clinical trial. JAMA 2015;314:1346-55.
39. Park JS, Lee SM, Choi GS, et al. Comparison of laparoscopic versus robot-assisted surgery for rectal cancers: the COLRAR randomized controlled trial. Ann Surg 2023;278:31-8.
40. Guo Y, Guo Y, Luo Y, Song X, Zhao H, Li L. Comparison of pathologic outcomes of robotic and open resections for rectal cancer: a systematic review and meta-analysis. PLoS One 2021;16:e0245154.
41. Feroci F, Vannucchi A, Bianchi PP, et al. Total mesorectal excision for mid and low rectal cancer: laparoscopic vs robotic surgery. World J Gastroenterol 2016;22:3602-10.
42. Cho MS, Baek SJ, Hur H, et al. Short and long-term outcomes of robotic versus laparoscopic total mesorectal excision for rectal cancer: a case-matched retrospective study. Medicine 2015;94:e522.
43. Kim J, Baek SJ, Kang DW, et al. Robotic resection is a good prognostic factor in rectal cancer compared with laparoscopic resection: long-term survival analysis using propensity score matching. Dis Colon Rectum 2017;60:266-73.
44. Spinoglio G, Bianchi PP, Marano A, et al. Robotic versus laparoscopic right colectomy with complete mesocolic excision for the treatment of colon cancer: perioperative outcomes and 5-year survival in a consecutive series of 202 patients. Ann Surg Oncol 2018;25:3580-6.
45. Mirkin KA, Kulaylat AS, Hollenbeak CS, Messaris E. Robotic versus laparoscopic colectomy for stage I-III colon cancer: oncologic and long-term survival outcomes. Surg Endosc 2018;32:2894-901.
46. Xu M, Zhao Z, Jia B, Liu R, Liu H. Perioperative and long-term outcomes of robot-assisted versus laparoscopy-assisted hemicolectomy for left-sided colon cancers: a retrospective study. Updates Surg 2021;73:1049-56.
47. Ozben V, de Muijnck C, Sengun B, et al. Robotic complete mesocolic excision for transverse colon cancer can be performed with a morbidity profile similar to that of conventional laparoscopic colectomy. Tech Coloproctol 2020;24:1035-42.
48. Ceccarelli G, Costa G, Ferraro V, De Rosa M, Rondelli F, Bugiantella W. Robotic or three-dimensional (3D) laparoscopy for right colectomy with complete mesocolic excision (CME) and intracorporeal anastomosis? A propensity score-matching study comparison. Surg Endosc 2021;35:2039-48.
49. Shah J, Vyas A, Vyas D. The history of robotics in surgical specialties. Am J Robot Surg 2014;1:12-20.
50. Cain P, Kazanzides P, Zuhars J, Mittelstadt B, Paul H. Safety considerations in a surgical robot. Biomed Sci Instrum 1993;29:291-4.
51. Sackier JM, Wooters C, Jacobs L, Halverson A, Uecker D, Wang Y. Voice activation of a surgical robotic assistant. Am J Surg 1997;174:406-9.
52. Falcone T, Goldberg J, Garcia-Ruiz A, Margossian H, Stevens L. Full robotic assistance for laparoscopic tubal anastomosis: a case report. J Laparoendosc Adv Surg Tech A 1999;9:107-13.
53. Melzer A, Schurr MO, Kunert W, Buess G, Voges U, Meyer JU. Intelligent surgical instrument system ISIS. Concept and preliminary experimental application of components and prototypes. Endosc Surg Allied Technol 1993;1:165-70.
54. Weber PA, Merola S, Wasielewski A, Ballantyne GH. Telerobotic-assisted laparoscopic right and sigmoid colectomies for benign disease. Dis Colon Rectum 2002;45:1689-96.
55. Morelli L, Guadagni S, Di Franco G, et al. Use of the new da Vinci Xi® during robotic rectal resection for cancer: a pilot matched-case comparison with the da Vinci Si®. Int J Med Robot 2017;13:e1728.
56. Baek SJ, Kim CH, Cho MS, et al. Robotic surgery for rectal cancer can overcome difficulties associated with pelvic anatomy. Surg Endosc 2015;29:1419-24.
57. Luca F, Valvo M, Ghezzi TL, et al. Impact of robotic surgery on sexual and urinary functions after fully robotic nerve-sparing total mesorectal excision for rectal cancer. Ann Surg 2013;257:672-8.
58. Ozben V, Cengiz TB, Atasoy D, et al. Is da Vinci Xi better than da Vinci Si in robotic rectal cancer surgery? Comparison of the 2 generations of da Vinci systems. Surg Laparosc Endosc Percutan Tech 2016;26:417-23.
59. Protyniak B, Jorden J, Farmer R. Multiquadrant robotic colorectal surgery: the da Vinci Xi vs Si comparison. J Robot Surg 2018;12:67-74.
60. Piozzi GN, Khan JS. Dual console operating in robotic surgery - a show off or a real necessity? Colorectal Dis 2023;25:1327-9.
61. Palmeri M, Gianardi D, Guadagni S, et al. Robotic colorectal resection with and without the use of the new Da Vinci table motion: a case-matched study. Surg Innov 2018;25:251-7.
62. Morelli L, Palmeri M, Simoncini T, et al. A prospective, single-arm study on the use of the da Vinci® table motion with the Trumpf TS7000dV operating table. Surg Endosc 2018;32:4165-72.
63. Bae SU, Jeong WK, Bae OS, Baek SK. Reduced-port robotic anterior resection for left-sided colon cancer using the Da Vinci single-site® platform. Int J Med Robot 2016;12:517-23.
64. Hamzaoglu I, Karahasanoglu T, Baca B, Karatas A, Aytac E, Kahya AS. Single-port laparoscopic sphincter-saving mesorectal excision for rectal cancer: report of the first 4 human cases. Arch Surg 2011;146:75-81.
65. Morelli L, Guadagni S, Caprili G, Di Candio G, Boggi U, Mosca F. Robotic right colectomy using the Da Vinci Single-Site® platform: case report. Int J Med Robot 2013;9:258-61.
66. Ostrowitz MB, Eschete D, Zemon H, DeNoto G. Robotic-assisted single-incision right colectomy: early experience. Int J Med Robot 2009;5:465-70.
67. Sun N, Zhang J, Zhang C, Shi Y. Single-site robotic cholecystectomy versus multi-port laparoscopic cholecystectomy: a systematic review and meta-analysis. Am J Surg 2018;216:1205-11.
68. Haber GP, White MA, Autorino R, et al. Novel robotic da Vinci instruments for laparoendoscopic single-site surgery. Urology 2010;76:1279-82.
69. Kim HJ, Choi GS, Song SH, et al. An initial experience with a novel technique of single-port robotic resection for rectal cancer. Tech Coloproctol 2021;25:857-64.
70. Piozzi GN, Kim JS, Choo JM, et al. Da Vinci SP robotic approach to colorectal surgery: two specific indications and short-term results. Tech Coloproctol 2022;26:461-70.
71. Song SH, Kim HJ, Choi GS, et al. Initial experience with a suprapubic single-port robotic right hemicolectomy in patients with colon cancer. Tech Coloproctol 2021;25:1065-71.
72. Baek SJ, Piozzi GN, Kim SH. Optimizing outcomes of colorectal cancer surgery with robotic platforms. Surg Oncol 2021;37:101559.
73. Piozzi GN, Lee DY, Kim JS, Kim SH. Da Vinci Single-Port (SP) robotic transverse colectomy for mid-transverse colon cancer. Tech Coloproctol 2022;26:681-2.
74. Noh GT, Oh BY, Han M, Chung SS, Lee RA, Kim KH. Initial clinical experience of single-incision robotic colorectal surgery with da Vinci SP platform. Int J Med Robot 2020;16:e2091.
75. Cheong JY, Choo JM, Kim JS, Rusli SM, Kim J, Kim SH. Da Vinci SP system optimized for intersphincteric resection of very low rectal cancer. Dis Colon Rectum 2022;65:e174.
76. Marks JH, Perez RE, Salem JF. Robotic transanal surgery for rectal cancer. Clin Colon Rectal Surg 2021;34:317-24.
77. Marks JH, Kunkel E, Salem JF, Martin C, Anderson B, Agarwal S. First clinical experience with single-port robotic transanal minimally invasive surgery (SP rTAMIS) for benign rectal neoplasms. Tech Coloproctol 2021;25:117-24.
78. Emrich Accioly JP, Zhao H, Ozgur I, Lee GC, Gorgun E, Wood HM. Single-port, robot-assisted transanal harvest of rectal mucosa grafts for substitution urethroplasty. Urology 2022;166:1-5.
79. Schleer P, Drobinsky S, de la Fuente M, Radermacher K. Toward versatile cooperative surgical robotics: a review and future challenges. Int J Comput Assist Radiol Surg 2019;14:1673-86.
80. Chang KD, Abdel Raheem A, Choi YD, Chung BH, Rha KH. Retzius-sparing robot-assisted radical prostatectomy using the Revo-i robotic surgical system: surgical technique and results of the first human trial. BJU Int 2018;122:441-8.
81. Ku G, Kang I, Lee WJ, Kang CM. Revo-i assisted robotic central pancreatectomy. Ann Hepatobiliary Pancreat Surg 2020;24:547-50.
82. Alip S, Koukourikis P, Han WK, Rha KH, Na JC. Comparing revo-i and da Vinci in retzius-sparing robot-assisted radical prostatectomy: a preliminary propensity score analysis of outcomes. J Endourol 2022;36:104-10.
83. Abdel Raheem A, Troya IS, Kim DK, et al. Robot-assisted Fallopian tube transection and anastomosis using the new REVO-I robotic surgical system: feasibility in a chronic porcine model. BJU Int 2016;118:604-9.
84. Rassweiler JJ, Autorino R, Klein J, et al. Future of robotic surgery in urology. BJU Int 2017;120:822-41.
85. Gkeka K, Tsaturyan A, Faitatziadis S, et al. Robot-assisted radical nephrectomy using the novel avatera robotic surgical system: a feasibility study in a porcine model. J Endourol 2023;37:273-8.
86. Nakauchi M, Suda K, Nakamura K, et al. Establishment of a new practical telesurgical platform using the hinotoriTM surgical robot system: a pre-clinical study. Langenbecks Arch Surg 2022;407:3783-91.
87. Takahashi Y, Hakamada K, Morohashi H, et al. Verification of delay time and image compression thresholds for telesurgery. Asian J Endosc Surg 2023;16:255-61.
88. Spinelli A, David G, Gidaro S, et al. First experience in colorectal surgery with a new robotic platform with haptic feedback. Colorectal Dis 2018;20:228-35.
89. McKechnie T, Khamar J, Daniel R, et al. The senhance surgical system in colorectal surgery: a systematic review. J Robot Surg 2023;17:325-34.
90. Samalavicius NE, Dulskas A, Janusonis V, et al. Robotic colorectal surgery using the Senhance® robotic system: a single center experience. Tech Coloproctol 2022;26:437-42.
91. Dixon F, O’Hara R, Ghuman N, Strachan J, Khanna A, Keeler BD. Major colorectal resection is feasible using a new robotic surgical platform: the first report of a case series. Tech Coloproctol 2021;25:285-9.
92. Bianchi PP, Salaj A, Rocco B, Formisano G. First worldwide report on Hugo RASTM surgical platform in right and left colectomy. Updates Surg 2023;75:775-80.
93. Böhlen D, Gerber R. First ever radical prostatectomy performed with the new dexter robotic systemTM. Eur Urol 2023;83:479-80.
94. Virtual Incision. World’s first surgery using virtual incision’s MIRA miniature robotic system performed at bryan medical center in Lincoln, Nebraska. Available from: https://virtualincision.com/worlds-first-surgery-using-mira/. [Last accessed on 15 Nov 2023].
95. Prendergast JM, Rentschler ME. Towards autonomous motion control in minimally invasive robotic surgery. Expert Rev Med Devices 2016;13:741-8.
96. Zorn L, Nageotte F, Zanne P, et al. A novel telemanipulated robotic assistant for surgical endoscopy: preclinical application to ESD. IEEE Trans Biomed Eng 2018;65:797-808.
97. Atallah S, Sanchez A, Bianchi E, Larach SW. Envisioning the future of colorectal surgery: preclinical assessment and detailed description of an endoluminal robotic system (ColubrisMX ELS). Tech Coloproctol 2021;25:1199-207.
98. Johnson CS, Kassir A, Marx DS, Soliman MK. Performance of da Vinci Stapler during robotic-assisted right colectomy with intracorporeal anastomosis. J Robot Surg 2019;13:115-9.
99. Ohi M, Toiyama Y, Ichikawa T, et al. Billroth-I reconstruction with overlap anastomosis using an EndoWrist linear stapler after robotic distal gastrectomy. J Laparoendosc Adv Surg Tech A 2020;30:1117-21.
100. Teo NZ, Ngu JCY. A comparison between the da Vinci Xi EndoWrist Stapler and a conventional laparoscopic stapler in rectal transection: a randomized controlled trial. Int J Med Robot 2023;19:e2501.
101. Guadagni S, Di Franco G, Gianardi D, et al. Control comparison of the new endowrist and traditional laparoscopic staplers for anterior rectal resection with the da Vinci Xi: a case study. J Laparoendosc Adv Surg Tech A 2018;28:1422-7.
102. Tejedor P, Sagias F, Flashman K, Kandala NL, Khan J. The use of robotic or laparoscopic stapler in rectal cancer surgery: a systematic review and meta-analysis. J Robot Surg 2020;14:829-33.
103. Tejedor P, Sagias F, Nock D, Flashman K, Naqvi S, Kandala NL, Khan JS. Advantages of using a robotic stapler in rectal cancer surgery. J Robot Surg 2020;14:365-70.
104. Schols RM, Bouvy ND, Van Dam RM, Stassen LPS. Advanced intraoperative imaging methods for laparoscopic anatomy navigation: an overview. Surg Endosc 2013;27:1851-9.
105. Takahashi H, Yanagita TE, Suzuki T, et al. Accurate localization of rectal cancer using near infrared ray-guided surgery with intra-operative colonoscopy and da Vinci Firefly technology. Surg Today 2020;50:205-8.
106. Spinoglio G, Petz W, Borin S, Piccioli AN, Bertani E. Robotic right colectomy with complete mesocolic excision and indocyanine green guidance. Minerva Chir 2019;74:165-9.
107. Al-Taher M, van den Bos J, Terink I, et al. Near-infrared fluorescence imaging for the intraoperative detection of endometriosis: a pilot study. Life 2022;12:15.
108. Giannini A, Bianchi M, Doria D, et al. Wearable haptic interfaces for applications in gynecologic robotic surgery: a proof of concept in robotic myomectomy. J Robot Surg 2019;13:585-8.
109. Bae SU, Baek SJ, Hur H, Baik SH, Kim NK, Min BS. Intraoperative near infrared fluorescence imaging in robotic low anterior resection: three case reports. Yonsei Med J 2013;54:1066-9.
110. Saeidi H, Opfermann JD, Kam M, et al. Autonomous robotic laparoscopic surgery for intestinal anastomosis. Sci Robot 2022;7:eabj2908.
Cite This Article
Export citation file: BibTeX | EndNote | RIS
OAE Style
Erozkan K, Gorgun E. Development of robotic surgical devices and its application in colorectal surgery. Mini-invasive Surg 2023;7:37. http://dx.doi.org/10.20517/2574-1225.2023.56
AMA Style
Erozkan K, Gorgun E. Development of robotic surgical devices and its application in colorectal surgery. Mini-invasive Surgery. 2023; 7: 37. http://dx.doi.org/10.20517/2574-1225.2023.56
Chicago/Turabian Style
Kamil Erozkan, Emre Gorgun. 2023. "Development of robotic surgical devices and its application in colorectal surgery" Mini-invasive Surgery. 7: 37. http://dx.doi.org/10.20517/2574-1225.2023.56
ACS Style
Erozkan, K.; Gorgun E. Development of robotic surgical devices and its application in colorectal surgery. Mini-invasive. Surg. 2023, 7, 37. http://dx.doi.org/10.20517/2574-1225.2023.56
About This Article
Copyright
Data & Comments
Data
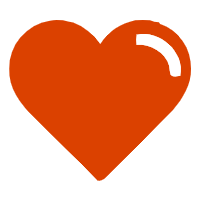
Comments
Comments must be written in English. Spam, offensive content, impersonation, and private information will not be permitted. If any comment is reported and identified as inappropriate content by OAE staff, the comment will be removed without notice. If you have any queries or need any help, please contact us at support@oaepublish.com.