3D augmented reality-guided robotic partial nephrectomy
Abstract
The present study aims to provide a contemporary overview of the use of augmented reality (AR) in robotic renal surgery from the renal pedicle management to the demolition and reconstructive phases thanks also to the preoperative planning obtained with three-dimensional virtual models (3DVMs). Recently, the increasing use of the robotic approach extends the indication to partial nephrectomy also in cases of complex or large renal masses and maximizing functional and surgical outcomes. With this goal, new imaging technologies have increased in popularity, especially for laparoscopic and robotic approaches. In this scenario, hyper-accuracy 3DVMs of the kidney and tumor, based on computed tomography (CT) scans, have been developed as a new tool for preoperative planning and intraoperative surgical navigation via AR technology. However, a standardized production process of 3DVMs and dedicated guidelines on their use and application are still needed. A recent systematic review and metanalysis has shed light on the impact of 3D models on minimally-invasive nephron-sparing surgery (NSS). Specifically, lower rates of global ischemia and collecting system violation were observed within AR-robot-assisted partial nephrectomy (RAPN). However, these rates did not translate into significant improvements in terms of oncological or functional outcomes. This review provides a contemporary overview of the use of AR in robotic renal surgery from the renal pedicle management to the demolition and reconstructive phases thanks also to the preoperative planning obtained with 3DVMs.
Keywords
INTRODUCTION
Current guidelines recommend nephron-sparing surgery (NSS) as the treatment of choice in small renal masses whenever technically feasible[1]. Specifically, minimally invasive approach (laparoscopic or robotic) should be preferred to minimize perioperative morbidity[2,3].
Many new technologies have been tested trying to broaden the indications for NSS to include larger and more surgically challenging renal masses, including the use of intravenous dyes [e.g., indocyanine green (ICG)] and hyper-accuracy three-dimensional virtual models (3DVMs)[4,5].
To improve planning and resection phase of the tumor, 3DVMs of the kidney and the tumor have been introduced.
These models are obtained from preoperative computed tomography (CT) images and can be used through different modalities such as holograms and intraoperative superimposition with augmented reality (AR) technology[6-8].
Considering the tumor resection phase, AR has proven to be the most attractive technology for the surgeon. This can be explained by the fact that this technology allows the real-time intraoperative superimposition of 3D models on real anatomy, enhancing the surgeon’s perception of anatomical relationship of the tumor with the surrounding anatomical structures.
Additionally, 3DVMs seem to improve trifecta rates of the procedure, also in highly complex renal tumors, by enhancing many surgical steps[6,9-12].
We aimed to summarize the clinical applications of AR technology during the different sections of robot-assisted partial nephrectomy (RAPN), from the renal pedicle approach to the resection and reconstructive techniques.
3DVM AND AR
Workflow to obtain a high-definition 3D virtual model
A dedicated team of bioengineers is needed to obtain the final model in about 24-48 h. It is mandatory that patients undergo four-phase (unenhanced corticomedullary, arterial, nephrographic, and urographic phase) contrast-enhanced CT[13,14]. This necessitates a specific scanning protocol, with distinct scan delays for each of the three phases: typically seven seconds for the arterial phase, 70 s for the nephrographic phase, and at least 10 min for the urographic phase[15]. Alternative imaging techniques such as magnetic resonance imaging (MRI), ultrasound, and non-contrast CT, along with advanced processing methods, can achieve similar levels of accuracy for patients with renal insufficiency. Studies have demonstrated the effectiveness of MRI for creating detailed 3D models of kidney cancers[6,15,16].
The following workflow [Table 1] helps summarize the step-by-step process for developing accurate and high-quality 3D models, from initial imaging to final visualization or printing[16]:
Standardized workflow for 3DVM creation
Stage | Key actions | Standards and guidelines |
1. Image acquisition | - Use standardized imaging protocols for CT, MRI, and Ultrasound - Set specific scan parameters | - Slice thickness: 1 mm for CT - MRI contrast sequences: T1, T2, DCE |
2. Data preprocessing | - Apply noise reduction and contrast enhancement - Align images using standardized software | - Use ISO-certified image processing software - Require predefined contrast thresholds |
3. Segmentation | - Use AI/ML algorithms for automatic segmentation - Refine manually when necessary | - Segmentation tools should have a ≤ 5% error margin - Follow ISO/IEC 62304 standards |
4. Model generation | - Construct 3D models based on processed images - Verify accuracy of organ and tumor shapes | - Accuracy margin: ≤ 0.5 mm for critical structures - Follow ISO/IEC 9001 for quality |
5. Validation | - Perform validation checks against reference standards (e.g., cadaver models) | - Validation protocol requiring ≤ 1 mm deviation from actual anatomy - Document deviations |
1. Image Acquisition: Multi-slice CT scans are preferred due to their detailed imaging and ability to export in DICOM format. The slice thickness should be ≤ 5 mm to ensure high-fidelity 3D reconstruction. MRI images can also be used, especially for soft tissue differentiation [Table 2].
Accuracy benchmarking for 3DVMs
Structure | Target accuracy margin | Validation methods | Benchmark example |
Kidney tumor | ≤ 0.5 mm | Validate against intraoperative ultrasound or MRI | Comparison with surgical specimen post-nephrectomy |
Renal arteries/veins | ≤ 1 mm | Validate with angiographic imaging or Doppler scan | Correlation with intraoperative vascular examination |
Surrounding organs | ≤ 2 mm | Use cross-sectional imaging for accuracy check | Compare with 2D CT or MRI results post-surgery |
2. Preprocessing: The 2D DICOM images are reviewed using specialized software, such as those that allow contrast and luminance adjustments. Images are chosen based on the phase that provides the most diagnostic value (e.g., arterial or late-phase CT scans).
3. Volume Rendering: The software automatically generates an initial 3D model from the voxels (the 3D counterpart of 2D pixels). This initial model gives an overview of the anatomical structure and highlights areas that may require further attention.
4. Segmentation: The segmentation process is performed using specialized software. Segmentation involves separating pixels that belong to regions or objects of interest (ROIs/OOIs), based on a chosen similarity criterion (e.g., color). One of the simplest methods for identifying different ROIs and OOIs is “thresholding”. By setting a specific parameter range (such as a gray scale, that represents the density of different tissues and structures in the CT image), the software can detect areas with the desired characteristics. However, as the ROIs could have similar gray values, the results could be inaccurate. This step is crucial in the creation of 3D models, as the software may not always accurately recognize and represent all elements, requiring manual adjustments. For example, a threshold of 1,330 GVs is used to segment the collecting system while avoiding the surrounding renal pyramids. Similarly, when it is necessary to include more surrounding structures, a threshold of 1,330 GVs could be used[6]. Since different phases are used to capture the collecting system and other anatomical parts, it is essential to align the segmented regions. Interactive translation and rotation tools are used to adjust the orientation and position of these different segments, ensuring that they accurately represent the kidney’s anatomy in the 3D model, and also in case of pyeloureteral malformation. At this stage, the engineer’s expertise is essential, allowing them to fine-tune the patient’s 3D model, much like an artisan perfects a finished product.
5. Refinement of the 3D Model: The segmented areas are refined to accurately represent the patient’s anatomy. The expertise of the engineer or clinician plays a significant role in ensuring the model is anatomically accurate.
6. Exporting the 3D Model: The final 3D model is exported in .stl (Standard Triangulation Language) format, a common format used in 3D printing and further modeling. This virtual model can then be reviewed, modified, or transferred for other purposes, such as surgical planning or patient consultation.
3D models: four different platforms
This interaction between the surgeon and the model can be utilized in different scenarios[17]:
• Virtual Reality: The 3D model is rendered within a fully immersive virtual setting.
• Mixed Reality: Through head-mounted display systems, both the virtual 3D model and the physical environment can exist and interact simultaneously.
• AR: The 3D model is projected onto the real world using a dedicated platform, such as Tilepro with the Da Vinci surgical console[18], enhancing the features of the real environment.
In the beginning, the superimposition of the images on the operative field was manual and static[17]. Subsequently, AR begins to incorporate the simulation of tissue deformation using nonlinear parametric techniques[6]. Finally, using a specific computer software vision algorithm, after injection of ICG, the primary reference point for superimposition is identified: the green image of the kidney, set against a dark surgical field[12]. After a registration time of ten seconds, an automatic AR driven-surgical navigation becomes possible[12]. However, extreme movements of the kidney (for example, kidney rotations) and posterior tumors still represent an obstacle for this technology.
Additionally, since 2022, a specific platform for 3D models, named ICON3DTM (Intraoperative Cognitive Navigation), has been available at https://www.medics3d.com. The ICON3DTM platform allows surgeons to independently access and manage 3D models via the cloud for use in robotic and laparoscopic surgeries. The platform supports preoperative planning, intraoperative navigation, and even AR surgical procedures, offering a bridge between traditional methods and advanced technologies such as AR and robotics[18].
Surgeons can access 3D models online, ensuring easy and fast retrieval regardless of location, and the minimally invasive modality used (laparoscopic or robotic). Surgeons can use 3D models for planning before surgery, allowing for an understanding of the patient’s anatomy in great detail. Moreover, during surgery, surgeons can navigate through the virtual models for real-time guidance and decision-making; otherwise, the platform also supports AR-based procedures, overlaying virtual models on real surgical views.
Several studies[8,19-23] have examined the effectiveness of 3D imaging models in the education of medical students, urology residents, and fellows. Rai et al. found that medical students using the interactive 3D virtual reality simulator (dV-Trainer) along with standard 2D images were better at identifying renal masses in 3D physical models compared to those who only used 2D planar images[22]. Additionally, two studies[20,21] assessed how well medical students could characterize renal tumors using the RENAL nephrometry scoring system, in comparison to a reference standard created by experienced surgeons. Both studies indicated that students were more precise in locating renal tumors with the 3D renal models than with 2D imaging alone. Knoedler et al. also observed that 3D-printed physical models enhanced students’ ability to correctly assign the R, N, and L components of the nephrometry score[20]. Accurately identifying the tumor’s location is crucial for minimizing both postoperative and major complications during partial nephrectomy.
Finally, several studies[8,20,23] have explored how 3D imaging models benefit urologists in presurgical planning, training and learning curves. Porpiglia et al. highlighted that 3D imaging provides a “hyper-accurate” view, offering a clearer understanding of surgical complexity compared to 2D images[9]. A recent study[18] showed the usability and users’ perception of this platform during a urology congress. Surgeons and attendees had the opportunity to test the new ICON3DTM platform and to fill out the Health Information Technology Usability Evaluation Scale (Health-ITUES) and the User-Experience Questionnaire (UEQ) questionnaire. The platform was helpful both for presurgical and surgical planning for the 43.4% and the 39.6% of the attendees.
Table 3 summarizes 3D application, training and competency framework for 3DVM use.
Training and competency framework for 3DVM use
Competency area | Training requirement | Certification process | Recertification |
Basic 3D anatomy interpretation | - Training on reading and interpreting 3DVMs - Use of simulation tools for practice | - Complete accredited training modules - Pass simulation-based test | Required every 2 years |
Surgical planning with 3DVMs | - Specific training for surgeons on tumor and organ resection planning - Hands-on workshops | - Certified by professional medical boards - Assessment during mock surgeries | Recertification through case studies |
Intraoperative navigation | - Use of 3DVMs in AR/VR-assisted surgery - Real-time imaging interpretation | - Pass competency test with AR/VR tools - Regular performance reviews | Ongoing training and assessments |
RENAL PEDICLE MANAGEMENT
Selective and superselective clamping
During the preoperative planning phase, the surgeon can explore various approaches to the hilum and determine the optimal clamping strategy to balance the risk of hypoxic damage with the potential for bleeding from the resection bed. Indeed, three-dimensional visualization of the pedicle enables the surgeon to more frequently use selective clamping or clampless NSS instead of global clamping. This approach, as highlighted in a recent systematic review by Piramide et al., significantly reduces global ischemia by 80%[23].
Indeed, 3DVM can reproduce not only the main renal artery and segmental branches, but also the interlobar vessels with specific attention to the feeding tumor artery. This can support a selective clamping approach, as initially demonstrated by Porpiglia et al.[15]. Additionally, the 3D group exhibited lower blood loss (mean difference of 23.1 mL, 95%CI: 31.8-14.4; P < 0.01). Finally, the same review indicated that the risk of incorrect selective clamping was reduced by approximately 10% in the ICG AR group compared to ICG guidance alone[12,13].
These encouraging results have pushed forward the research in this field.
Perfusion areas: the rainbow kidney
The latest generation of 3D kidney models can be enhanced with mathematical models that predict which regions of the renal parenchyma are supplied by each arterial branch, allowing for the individualization of specific perfusion areas. Specifically, each branch of the renal artery is considered as a set of seed points in a Voronoi diagram instead of using the endpoints of arteries[24,25]. The 3D reconstructions can then be divided by perfusion region and displayed in different colors[25], as a “rainbow kidney”. This means that, based on 3DVM perfusion areas, it is possible to balance the rate of renal tissue spared from ischemia and the feasibility of selective or superselective clamping. For example, the surgeon can put a bulldog clamp only on the segmental tumor feeding arteries or on the prepyelic or the retropyelic branch of the main renal artery[25].
RESECTION AND RECONSTRUCTIVE TECHNIQUE
Intraoperative real-time superimposition of 3D models over the real anatomy
Intraoperatively, the resection plane follows the lesion marked on the renal parenchyma surface under a 3D AR guidance overlay, allowing the surgeon to spare vascular or draining structure.
During robotic partial nephrectomy, various resection techniques are used to remove renal tumors while preserving as much healthy tissue as possible[26-28]. The ICON3DTM platform also allows the simulation of the resection bed reporting through different colors to indicate the depth of the resection. This feature helps simulate potential violations of the collecting system or feeding arteries, preventing complications[18].
Recently, a systematic review by Bertolo et al. reported a new classification of kidney resection based on the extension of healthy tumoral tissue removed: resection (1 cm), enucleoresection, divided in traditional enucleoresection (2 mm) and mini-enucleoresection (2 mm, before transitioning to the tumor’s pseudocapsule), enucleation (excision following its pseudocapsule, virtually no surrounding healthy tissue excised)[26].
Surgeons often adapt their approach based on the anatomical characteristics of the tumor and the surrounding kidney tissue. For example, an attempted enucleation might transition into mini-enucleoresection if following the pseudocapsule is not feasible.
The decision between these techniques balances the goals of maximizing tumor removal while preserving kidney function and minimizing complications such as positive margins or structural damage.
In conclusion, the choice between resection, enucleoresection, and enucleation depends on tumor characteristics, with increasing emphasis on kidney preservation and oncological safety.
Each of these techniques is chosen based on the tumor’s characteristics, the patient’s overall health, and the surgeon’s preference, with the aim of optimizing both oncological outcomes and functional kidney preservation.
Finally, during reconstructive phase, in case of violation of calyces or venous branches, the use of 3DVMs allows the surgeon to quickly identify structures that need to be repaired.
Enucleation rate and functional outcomes
Piramide et al. demonstrated that the use of 3D imaging during NSS resulted in higher rates of enucleation (OR: 2.54, 95%CI: 1.36-4.74; P < 0.01) and a lower incidence of collecting system injury (OR: 0.36, 95%CI: 0.15-0.89; P = 0.03) compared to 2D imaging[23]. The enucleation technique, which preserves more healthy renal parenchyma, is associated with improved postoperative functional outcomes[27], without the risk of a greater amount of positive surgical margins relative to enucleoresection, as showed by a large multinstitutional study[28].
However, the only metanalysis[23] available failed to show any difference in the change of renal function, despite the higher rate of enucleation in the 3DVMs group. However, using the estimated renal plasmatic flow (ERPF) derived renal scan as a functional renal parameter, Amparore et al. found a worrisome ERPF drop in the 2D group relative to the 3D group (-10% vs. -19.6%; P = 0.002)[29]. Additionally, in multivariate analysis, the use of 3DVMs was the only protective factor against ERPF drop (ERPF drop > 20%: OR: 0.3, 95%CI: 0.1-0.6; P = 0.002).
Moreover, a large institutional study[11] found that, regardless of the definition of Trifecta, the 3D model group consistently exhibited two-fold higher success rates (OR: 2.7, P < 0.001; OR: 2.0, P = 0.0008; OR: 2.8,
A Multinstitutional study[30] identified preoperative estimated glomerular filtration rate (eGFR), the type of ischemia technique used, and early postoperative renal function as key predictors of renal function one year after partial nephrectomy. This emphasizes the importance of precise surgical techniques and optimal intraoperative decision-making in ensuring favorable long-term outcomes for patients undergoing complex renal surgeries.
The role of 3DVMs and AR in this context is significant, as these technologies facilitate enhanced visualization of tumor margins and critical structures. This allows surgeons to perform precise resections while minimizing unnecessary damage to healthy renal parenchyma, which is crucial in reducing ischemia time and preserving kidney function. By minimizing surgical trauma, AR can positively influence these predictive factors - preoperative eGFR, ischemia technique, and early postoperative renal function - ultimately improving functional and oncologic outcomes in complex renal tumor cases.
Incorporating AR-guided RAPN could, therefore, be a game-changer in managing complex renal masses, ensuring better functional preservation and enhanced patient recovery.
VIRTUAL SURGERY AND ANATOMIC SURGICAL INSIGHTS
Another significant advantage of using 3DVMs is the ability to conduct “virtual” surgery before performing the actual procedure in the operating room. This approach not only provides valuable insights into the previously mentioned steps, such as renal pedicle management and resection-reconstruction techniques, but also helps predict potential intraoperative scenarios that may require intervention. For example, it aids in identifying aberrant renal vessels, planning selective clamping of renal artery branches, and improving control of the renal hilum in case of bleeding. This allows the surgeon to evaluate the potential for postoperative renal function more effectively, rather than focusing solely on tumor excision.
RAPN AND 3DVMS IN COMPLEX RENAL MASSES
RAPN has demonstrated its effectiveness as a treatment option for a range of complex renal tumors, including completely endophytic, large, and hilar masses, and recurrent tumors and cases involving a solitary kidney[31].
In this context, advances in robotic technology, AR, and 3D modeling have significantly improved outcomes, allowing for better preservation of renal function, reduced complications, and strong oncologic control[32,33].
Larger tumors have a greater risk of local invasion and complex vascular anatomy, increasing the difficulty of partial nephrectomy. However, RAPN, for large tumors, is feasible, but outcomes are highly dependent on surgeon experience[34]. A recent review[31] showed that, with careful planning and expertise, RAPN can achieve negative margins, maintain renal function, and manage complications effectively. In the attempt to provide the best surgical planning, 3DVMs could be a helpful tool for these patients[7].
Finally, for solitary kidneys, preserving renal function is of paramount importance, as the risk of post-surgical renal insufficiency is high. RAPN in solitary kidneys is associated with positive oncologic outcomes while prioritizing nephron-sparing techniques[35,36].
In the case of completely endophytic renal masses, the lack of visual clues and a large contact surface area make RAPN particularly difficult.
In this case, AR can overlay 3DVMs onto the actual anatomy during surgery, helping the surgeon visualize the hidden tumor in real time[37].
However, the complexity of these cases requires careful perioperative management to minimize ischemia time and preserve maximum renal function. Also in this case, 3DVMs could help give a comprehensive preoperative planning to optimize both functional and oncologic outcomes[38]. Specifically, Pecoraro et al. reported that 3DVM can be a valuable tool for managing highly complex renal masses with unusual anatomy and imperative indications for NSS[11].
They presented three clinical cases with similar renal anatomy and tumor characteristics on 3DVMs, and with potentially independent vascularization and drainage for the lower pole.
In the first case presented in the study, the infundibulum of the upper calyceal group was dislocated by the tumor, while an accessory artery fed the lower pole of the kidney. In this case, the treatment of choice was enucleoresection. However, after three months, MRI was performed showing a functionally excluded hydrocalyx. This was due to the damage of the infundibular connection to the upper calyceal system, resulting in loss of almost all the upper pole renal tissue, as showed at renal scan. In light of this experience, in the other two cases, an upper heminephrectomy was performed.
Indeed, the high risk of damage of the calyceal infundibulum pushed the surgeon to perform a hemi-nephrectomy, instead of enucleoresection, following the plane of the tumor and sparing the lower pole of the kidney with its arterial and venous pedicle of pertinence. This choice was made also considering the potential loss of renal function after surgery.
In this case, it is noteworthy that 3DVM defined a precise anatomical cluster that influenced and modulated the final surgical approach of the surgeon based on the previous AR-guided surgery.
Similarly, Campi et al. reported the 3DVM application in a patient harboring two complex renal masses in a horseshoe kidney[38].
Specifically, the 3D model allowed the identification of the presence of three renal arteries for the left kidney. This influenced the surgeon to perform a selective clamping strategy (two out of three feeding arteries) for the enucleation of the largest mass, after delineation of its contours via AR technology, and a clampless strategy was used for the resection of the smallest mass, preserving all vascular branches supplying the middle and upper pole of the left kidney.
REAL TIME DYNAMIC IMAGING DURING SURGERY
Hyper-accuracy 3DVMs are valuable for preoperative tumor evaluation and intraoperative navigation, but their use in real-time dynamic imaging during surgery presents additional challenges. During surgery, hyper-accuracy 3DVMs assist in guiding the surgeon by overlaying preoperative 3D models onto real-time imaging. However, real-time dynamic imaging requires continuous updates to reflect changes during surgery, such as tumor deformation or movement. Conversely, current Technology relies on techniques such as intraoperative ultrasound, fluoroscopy, or endoscopy. Integrating these with hyper-accuracy 3DVMs requires sophisticated systems that can synchronize the 3D model with real-time changes. These could be possible only through high-speed data acquisition and processing, along with advanced computational resources, to merge real-time data with preoperative models seamlessly.
STANDARDIZATION OF 3DVMS AND LIMITATIONS
Despite the progress made in this field, many technical and ethical concerns still need to be addressed.
A large part of the urological community is blind regarding the preconditions needed and the correct steps to produce 3DVMs[16].
The widespread of a standardized process is needed for the creation of 3DVMs which can be used by every surgeon as a certificated medical device. Several 3DVM companies have already gained recognition from Food and Drug Administration (FDA), along with the ICON3D system, for example, IRISTM (Intuitive Surgical; Sunnyvale, CA), a platform for surgical planning and navigation produces 3DVM following a specific workflow giving relatively standardized 3DVM. Surgeons can upload on this platform CT scan images and obtain the correspondent 3DVM in 24-48 h.
However, there is still inconsistency in the quality of the final delivered product due to the wide number and type of processes and different software packages available. Moreover, 3D modeling requires a trained team with a significant level of expertise.
Indeed, the risk is to deliver inaccurate 3DVM which could dramatically influence surgical approach or technique based on inexact information obtained from the model itself.
In an effort to streamline the process, a team of surgeons and engineers has begun developing guidelines for reporting criteria and workflow steps in research projects focused on producing 3DVMs. The goal is to review the methodology reporting on three-dimensional modeling (3DM) reconstruction to estimate the quality of the models used to guide the surgical act (https://www.crd.york.ac.uk/prospero/display_record.php?ID=CRD42021276893). The review will assess if the reported methodology in each study includes acquisition of DICOM images, segmentation procedure and software used, post-processing phase (including smoothing of the triangles), and 3D reconstruction validation and visualization.
Checcucci et al. have already submitted a proposal for 3D model reconstruction guidelines to the EQUATOR Network website (www.equator-network.org) to equip the scientific community with a new resource in the near future[16].
Concerning the most appealing 3DVM-derived surgical navigation systems, two major barriers still hinder their diffusion and implementation. First, current AR technologies lack the ability to adequately simulate elastic deformation of the model, and artifacts related to movement, such as breathing, require further refinement.
This necessitates ongoing and meticulous assistance from an expert urologist or engineer to manually adjust the alignment of the 3D model with the anatomy in real time during RAPN, with a 0.5-second delay through the TilePro window.
Secondly, the occlusion of robotic instruments by the 3DVM during surgery remains a concern. Not all instruments may be clearly visible in AR, as the superimposition of a 3D model can obscure parts of the surgical field, including the instruments, potentially creating a hazardous situation.
To address these issues, De Backer et al. proposed an algorithm leveraging deep learning networks to detect all non-anatomical items during AR-guided robot-assisted kidney transplantation[39]. In this context, artificial intelligence and deep learning algorithms could help resolve localization challenges by recognizing robotic instruments that may be obscured by the superimposed 3D model[40]. Additionally, numerical methods could assist in generating realistic model deformation sequences to account for motion artifacts or surgical manipulation.
In the near future, 3D models are expected to achieve automatic alignment with maximal accuracy and minimal delay. However, the cost of this technology remains a significant barrier to its widespread adoption in routine clinical practice[17].
CONCLUSION
This review provides a contemporary overview of the use of AR in robotic renal surgery from the renal pedicle management to the demolition and reconstructive phases thanks also to the preoperative planning obtained with 3DVMs.
Literature demonstrated that 3DVMs are an ally for surgeons in the form of a medical device to perform virtual tailored surgery before the operating room, with the aim of minimizing risks and complications.
However, specific guidelines and a standardization of 3DVM production process are needed in order to offer the best and accurate help for surgeons even in more challenging and complex cases.
DECLARATIONS
Authors’ contributions
Literature research, writing and editing: Pecoraro A, Piramide F, Amparore D
Availability of data and materials
Not applicable.
Financial support and sponsorship
None.
Conflicts of interest
All authors declared that there are no conflicts of interest.
Ethical approval and consent to participate
Not applicable.
Consent for publication
Not applicable.
Copyright
© The Author(s) 2024.
REFERENCES
1. Ljungberg B, Albiges L, Abu-Ghanem Y, et al. European Association of Urology Guidelines on Renal Cell Carcinoma: the 2022 update. Eur Urol 2022;82:399-410.
2. Bertolo R, Bove P, Sandri M, et al; AGILE Group (Italian Group for Advanced Laparoendoscopic Surgery). Randomized clinical trial comparing on-clamp versus off-clamp laparoscopic partial nephrectomy for small renal masses (CLOCK II Laparoscopic Study): a intention-to-treat analysis of perioperative outcomes. Eur Urol Open Sci 2022;46:75-81.
3. Bravi CA, Larcher A, Capitanio U, et al. Perioperative outcomes of open, laparoscopic, and robotic partial nephrectomy: a prospective multicenter observational study (The RECORd 2 Project). Eur Urol Focus 2021;7:390-6.
4. Wake N, Bjurlin MA, Rostami P, Chandarana H, Huang WC. Three-dimensional printing and augmented reality: enhanced precision for robotic assisted partial nephrectomy. Urology 2018;116:227-8.
5. Porpiglia F, Amparore D, Checcucci E, et al; for ESUT Research Group. Current use of three-dimensional model technology in urology: a road map for personalised surgical planning. Eur Urol Focus 2018;4:652-6.
6. Porpiglia F, Checcucci E, Amparore D, et al. Three-dimensional augmented reality robot-assisted partial nephrectomy in case of complex tumours (PADUA ≥ 10): a new intraoperative tool overcoming the ultrasound guidance. Eur Urol 2020;78:229-38.
7. Bertolo R, Autorino R, Fiori C, et al. Expanding the indications of robotic partial nephrectomy for highly complex renal tumors: urologists’ perception of the impact of hyperaccuracy three-dimensional reconstruction. J Laparoendosc Adv Surg Tech A 2019;29:233-9.
8. Checcucci E, Amparore D, Pecoraro A, et al. 3D mixed reality holograms for preoperative surgical planning of nephron-sparing surgery: evaluation of surgeons’ perception. Minerva Urol Nephrol 2021;73:367-75.
9. Porpiglia F, Amparore D, Checcucci E, et al. Three-dimensional virtual imaging of renal tumours: a new tool to improve the accuracy of nephrometry scores. BJU Int 2019;124:945-54.
10. Minervini A, Campi R, Lane BR, et al. Impact of resection technique on perioperative outcomes and surgical margins after partial nephrectomy for localized renal masses: a prospective multicenter study. J Urol 2020;203:496-504.
11. Pecoraro A, Amparore D, Checcucci E, et al. Three-dimensional virtual models assistance predicts higher rates of “successful” minimally invasive partial nephrectomy: an institutional analysis across the available trifecta definitions. World J Urol 2023;41:1093-100.
12. Amparore D, Checcucci E, Piazzolla P, et al. Indocyanine green drives computer vision based 3D augmented reality robot assisted partial nephrectomy: the beginning of “automatic” overlapping era. Urology 2022;164:e312-6.
13. Diana P, Buffi NM, Lughezzani G, et al. The role of intraoperative indocyanine green in robot-assisted partial nephrectomy: results from a large, multi-institutional series. Eur Urol 2020;78:743-9.
14. Shirk JD, Thiel DD, Wallen EM, et al. Effect of 3-dimensional virtual reality models for surgical planning of robotic-assisted partial nephrectomy on surgical outcomes: a randomized clinical trial. JAMA Netw Open 2019;2:e1911598.
15. Porpiglia F, Bertolo R, Checcucci E, et al. Development and validation of 3D printed virtual models for robot-assisted radical prostatectomy and partial nephrectomy: urologists’ and patients’ perception. World J Urol 2018;36:201-7.
16. Checcucci E, Piazza P, Micali S, et al; Uro-technology, SoMe Working Group of the Young Academic Urologists of the European Association of Urology. Three-dimensional model reconstruction: the need for standardization to drive tailored surgery. Eur Urol 2022;81:129-31.
17. Porpiglia F, Fiori C, Checcucci E, Amparore D, Bertolo R. Hyperaccuracy three-dimensional reconstruction is able to maximize the efficacy of selective clamping during robot-assisted partial nephrectomy for complex renal masses. Eur Urol 2018;74:651-60.
18. Checcucci E, Piramide F, De Cillis S, et al. Health Information Technology Usability Evaluation Scale (Health-ITUES) and User-Experience Questionnaire (UEQ) for 3D intraoperative cognitive navigation (ICON3DTM) system for urological procedures. Medicina 2023;59:624.
19. Smith B, Dasgupta P. 3D printing technology and its role in urological training. World J Urol 2020;38:2385-91.
20. Knoedler M, Feibus AH, Lange A, et al. Individualized physical 3-dimensional kidney tumor models constructed from 3-dimensional printers result in improved trainee anatomic understanding. Urology 2015;85:1257-61.
21. Bernhard JC, Isotani S, Matsugasumi T, et al. Personalized 3D printed model of kidney and tumor anatomy: a useful tool for patient education. World J Urol 2016;34:337-45.
22. Rai A, Scovell JM, Xu A, et al. Patient-specific virtual simulation - a state of the art approach to teach renal tumor localization. Urology 2018;120:42-8.
23. Piramide F, Kowalewski KF, Cacciamani G, et al; European Association of Urology Young Academic Urologists and the European Section of Uro-Technology. Three-dimensional model-assisted minimally invasive partial nephrectomy: a systematic review with meta-analysis of comparative studies. Eur Urol Oncol 2022;5:640-50.
24. Wang C, Roth HR, Kitasaka T, et al. Precise estimation of renal vascular dominant regions using spatially aware fully convolutional networks, tensor-cut and Voronoi diagrams. Comput Med Imaging Graph 2019;77:101642.
25. Amparore D, Piramide F, Checcucci E, et al. Three-dimensional virtual models of the kidney with colored perfusion regions: a new algorithm-based tool for optimizing the clamping strategy during robot-assisted partial nephrectomy. Eur Urol 2023;84:418-25.
26. Bertolo R, Pecoraro A, Carbonara U, et al; European Association of Urology Young Academic Urologists Renal Cancer Working Group. Resection techniques during robotic partial nephrectomy: a systematic review. Eur Urol Open Sci 2023;52:7-21.
27. Minervini A, Carini M. Tumor enucleation is appropriate during partial nephrectomy. Eur Urol Focus 2019;5:923-4.
28. Di Maida F, Campi R, Lane BR, et al. Predictors of positive surgical margins after robot-assisted partial nephrectomy for localized renal tumors: insights from a large multicenter international prospective observational project (the surface-intermediate-base margin score consortium). J Clin Med 2022;11:1765.
29. Amparore D, Pecoraro A, Checcucci E, et al. Three-dimensional virtual models’ assistance during minimally invasive partial nephrectomy minimizes the impairment of kidney function. Eur Urol Oncol 2022;5:104-8.
30. Crocerossa F, Fiori C, Capitanio U, et al. Estimated glomerular filtration rate decline at 1 year after minimally invasive partial nephrectomy: a multimodel comparison of predictors. Eur Urol Open Sci 2022;38:52-9.
31. Pandolfo SD, Cerrato C, Wu Z, et al. A systematic review of robot-assisted partial nephrectomy outcomes for advanced indications: large tumors (cT2-T3), solitary kidney, completely endophytic, hilar, recurrent, and multiple renal tumors. Asian J Urol 2023;10:390-406.
32. Checcucci E, Amparore D, Volpi G, Porpiglia F. A snapshot into the future of image-guided surgery for renal cancer. Asian J Urol 2022;9:201-3.
33. Puliatti S, Eissa A, Checcucci E, et al. New imaging technologies for robotic kidney cancer surgery. Asian J Urol 2022;9:253-62.
34. Malkoc E, Ramirez D, Kara O, et al. Robotic and open partial nephrectomy for localized renal tumors larger than 7 cm: a single-center experience. World J Urol 2017;35:781-7.
35. Hillyer SP, Bhayani SB, Allaf ME, et al. Robotic partial nephrectomy for solitary kidney: a multi-institutional analysis. Urology 2013;81:93-7.
36. Zargar H, Bhayani S, Allaf ME, et al. Comparison of perioperative outcomes of robot-assisted partial nephrectomy and open partial nephrectomy in patients with a solitary kidney. J Endourol 2014;28:1224-30.
37. Komninos C, Shin TY, Tuliao P, et al. Robotic partial nephrectomy for completely endophytic renal tumors: complications and functional and oncologic outcomes during a 4-year median period of follow-up. Urology 2014;84:1367-73.
38. Campi R, Sessa F, Rivetti A, et al. Case report: optimizing pre- and intraoperative planning with hyperaccuracy three-dimensional virtual models for a challenging case of robotic partial nephrectomy for two complex renal masses in a horseshoe kidney. Front Surg 2021;8:665328.
39. De Backer P, Van Praet C, Simoens J, et al. Improving augmented reality through deep learning: real-time instrument delineation in robotic renal surgery. Eur Urol 2023;84:86-91.
Cite This Article
How to Cite
Pecoraro, A.; Piramide, F.; Amparore, D. 3D augmented reality-guided robotic partial nephrectomy. Mini-invasive. Surg. 2024, 8, 25. http://dx.doi.org/10.20517/2574-1225.2023.134
Download Citation
Export Citation File:
Type of Import
Tips on Downloading Citation
Citation Manager File Format
Type of Import
Direct Import: When the Direct Import option is selected (the default state), a dialogue box will give you the option to Save or Open the downloaded citation data. Choosing Open will either launch your citation manager or give you a choice of applications with which to use the metadata. The Save option saves the file locally for later use.
Indirect Import: When the Indirect Import option is selected, the metadata is displayed and may be copied and pasted as needed.
About This Article
Special Issue
Copyright
Data & Comments
Data
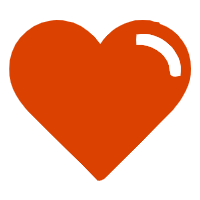
Comments
Comments must be written in English. Spam, offensive content, impersonation, and private information will not be permitted. If any comment is reported and identified as inappropriate content by OAE staff, the comment will be removed without notice. If you have any queries or need any help, please contact us at support@oaepublish.com.