Shape-deformable Micro-LEDs for advanced displays and healthcare
Abstract
Recently, flexible/stretchable micro-scale light-emitting diodes (LEDs), with dimensions significantly smaller than conventional diodes used for illuminations, have emerged for promising applications in areas such as deformable displays, wearable devices for healthcare, etc. For such applications, these devices must have some unusual features that common inorganic LEDs do not intrinsically own, including conformability, biocompatibility, mechanical flexibility, etc. This Perspective focuses on summarizing the most recent progress in developing such flexible emitters based on inorganic semiconductors, followed by reviewing their potential applications. Finally, major challenges and future research directions of deformable micro-scale LEDs are presented.
Keywords
INTRODUCTION
Micro-scale light-emitting diode (Micro-LED) displays made from p-n junctions of III-V inorganic epitaxial semiconductors, such as gallium nitride (GaN) and aluminum gallium indium phosphide (AlGaInP), have stood out as a competing technology over organic LED (OLED) and liquid crystal displays (LCD)[1-7]. Despite the reduced physical dimensions into a regime of a few tens of microns or below, they exhibit unusual properties such as fast switching speed, high brightness, long lifetime, and large current withstanding capability[8], making them particularly suited for low-power consumption and high-resolution display. Indeed, significant progress of Micro-LEDs has been made in areas such as smart watches, VR/AR, and high-resolution TVs. Their huge commercial prospect has been partially confirmed, accompanied by a booming display market worth tens of billions of dollars.
In contrast to the tremendous progress of using planar Micro-LEDs for conventional rigid displays, the development of flexible Micro-LED counterparts is currently actively explored, evidenced by intensive research interest from both academia and industry[9-18]. Benefiting from their extraordinary luminescent property, deformable displays made from inorganic Micro-LEDs can not only significantly improve visual experience of users but also potentially provide more convenience, better portability, and easier connectivity with their surroundings. Furthermore, they allow more versatile design with various forms that are difficult to achieve using conventional planar devices. Examples of such innovations have led to the recent demonstration of distortion-free displays[10], foldable three-dimensional (3D) displays[19], etc.
Apart from high-resolution deformable displays, some emerging applications, such as optogenetics[20-28] and smart contact lenses[29-33], also drive the development of highly efficient, biocompatible light sources that can be conformally attachable to skins or implantable inside the human body for healthcare [34]. Micro-LEDs in flexible/stretchable formats are well suited for these purposes. Here, we highlight the recent technology advancements in developing such flexible emitters and then discuss their potential applications for uncommon displays and healthcare. Finally, future research trends of flexible inorganic emitters are also given.
STRATEGIES TOWARD DEFORMABLE MICRO-LEDS
Conventional inorganic semiconductors used for Micro-LEDs have higher conductivities and mobilities, leading to higher brightness and better operation stability. However, high-performance inorganic semiconductors are usually grown on rigid, planar substrates, which means they are not intrinsically flexible. In order to render Micro-LEDs with flexibility, several strategies must be considered, including substrate removal, chip transfer printing, and certain mechanical design for enhanced stretchability [Figure 1].
Figure 1. Strategies toward the fabrication of deformable Micro-LEDs, including substrate removal (A-C), micro-assembly (D-F), and mechanical design (E-I). Schematics for the substrate removal methods, including (A) laser lift-off, (B) chemical lift-off, and (C) mechanical lift-off[35]; Representative transfer methods for Micro-LED integration on curvilinear substrates reported recently, including (D) ballon-shaped stamp transfer[60], (E) roller transfer[11], and (F) photosensitive tape-assisted transfer[17]; Typical mechanical structure designs for improved stretchability of Micro-LED devices, including (G) island-bridge design[61], (H) buckling structures[16], and (I) Kirigami structures[9]. Figure 1A-C reproduced under the terms of the CC-BY Creative Commons Attribution 4.0 International
Substrate removal
Thickness reduction by substrate removal can impart certain flexibility to Micro-LEDs. Two major strategies have been developed to take off the growth substrate: laser lift-off (LLO)[6,35,36] and epitaxial lift-off (ELO)[37-43]. LLO exploits the laser energy absorption at the LED layer/substrate interface, which leads to the release of Micro-LEDs from the substrate due to the high-temperature induced material decomposition at the interface[35] [Figure 1A]. Prior to LLO, the LED wafer is temporarily bonded to a supporting carrier. High-energy laser scanning leads to the transfer of a thin membrane to the supporting substrate. ELO, on the other hand, is a technique based on a modified epitaxial structure, where a sacrificial layer is incorporated in the epi-stack to assist the release of Micro-LEDs grown on the sacrificial layer[38]. Depending on specific release layers, two ELO principles have been established: chemical (CLO)[43-46] and mechanical lift-off (MLO)[37,38]. In the case of CLO, the sacrificial layer can be removed by chemical solvents, resulting in released thin film Micro-LEDs [Figure 1B]. For MLO, a handling carrier (for instance, a tape) is used to peel off the epi-stack grown on the release layer directly due to the significantly weakened bonding of the epi-layer to the substrate [Figure 1C]. Two-dimensional (2D) material-assisted epitaxy or remote epitaxy has emerged to fulfill this purpose[39-42,47-49]. Growth on voids or porous templates can also lead to successful MLO[50,51]. However, MLO is usually done manually, making it difficult to accurately control the process with good reproducibility. By contrast, CLO is more controllable, which has witnessed commercial success, especially for the fabrication of solar cell modules[52,53].
Micro-assembly
System-scale flexibility can be partially enhanced by micro-assembling Micro-LEDs onto soft substrates[35,54-56]. With shrinking the Micro-LED size and thickness into the micro-scale regime, fast and accurate manipulation of Micro-LEDs in a reliable manner becomes a critical challenge. Several micro-assembly techniques have been established to partially address this issue, including the pioneering stamp transfer printing methods[15,16,56,57], where an elastic polydimethylsiloxane (PDMS) stamp picks up the microscale device from the source wafer and then releases it to a receiver. To assist the transfer, Micro-LEDs are commonly patterned in a manner such that they are weakly suspended on the growth substrate by using anchors or undercut microstructures[7]. Other popular methods include laser-based[6,58] or fluid-assisted micro-assembly[55,59], which have competing advantages in terms of the transfer speed. While most of them do work for transfer printing microscale devices to planar surfaces, some present poor combability for integrating Micro-LEDs onto a flexible or curvy substrate, primarily because the conventional planar stamps are difficult to be in conformal contact with the curvy/flexible surface. The issue can be partially alleviated by micro-assembling Micro-LEDs onto a planar soft substrate fixed on a temporary hard substrate. The temporary supporting substrate is then removed, enabling the integration of Micro-LEDs onto soft substrates[6]. Alternatively, several modified micro-assembly methods have been developed to address the challenge of Micro-LED integration onto curved substrates directly [Figure 1D-F]. Rao et al. developed a ballon-shaped stamp for printing micro-scale devices onto a curvy surface[60] [Figure 1D]. Hu et al. proposed using a roller stamp for flexible Micro-LED integration[11] [Figure 1E]. Guo et al. developed a photosensitive polymer adhesive stamp-based method[17] [Figure 1F]. These approaches exhibit excellent capability for micro-assembling micro-scale chips onto both planar and curvilinear surfaces due to the improved conformity with the receiver and adhesion switchability. However, transfer speed, printing yield, and placement accuracy are key factors that need continuous improvement, especially for the device integration onto curvy substrates.
Mechanical structure design
The brittle nature of Micro-LEDs prevents the system (i.e., Micro-LEDs and integrated substrates) from accommodating large strain induced by bending, especially in the localized areas close to Micro-LEDs. Extra mechanical design can provide further room to enhance the system performance and stretchability. Representative strategies toward enhanced deformation of Micro-LEDs include island-bridge[15,61,62], buckling[16,63], and kirigami/origami structures[9,60]. Stretchable Micro-LEDs with the island-bridge configuration have rigid island arrays (where these LEDs are fixed) and meandering metallic interconnects connected to individual islands[61] [Figure 1G]. In this structure, the Micro-LED devices on the rigid islands are hardly affected by external strain, whereas the meandering interconnects, which are commonly patterned into serpentine or noncoplanar arc-shapes, can tolerate most of the strain and geometric deformation. Stretchable Micro-LED devices can also be achieved by incorporating buckled structures[16] [Figure 1H], inspired by the fact that a flexible film on a pre-stretched soft substrate (e.g., PDMS) can turn into periodically wrinkled structures once the pre-stressed substrate is relaxed. Such wrinkle structures can be flattened if the substrate is re-stretched, which allows the flexible devices to be stretched without mechanical failure. Micro-LED integrated onto a substrate with kirigami/origami structures is another smart strategy for fabricating stretchable Micro-LEDs[9] [Figure 1I]. The 2D stress exerted on the Micro-LED device can be significantly reduced via 3D shape transformation induced by the cutting/folding lines, thereby mitigating stress concentrations around the Micro-LED devices.
EMERGING APPLICATIONS
With the above innovations in material epitaxy, chip transfer, device fabrication, and mechanical designs, great progress has been made toward developing advanced deformable Micro-LEDs with expanded functionalities for applications such as advanced displays and healthcare.
Deformable displays
Currently, most display electronics take the rigid, planar shape, but in the future, foldable/flexible displays may become the mainstream products. To this end, Micro-LEDs in deformable formats have undergone intensive research recently due to their higher luminescent efficiency and longer lifetime than their organic counterparts. Industrial research in this field has led to great progress in developing deformable Micro-LED display prototypes with encouraging performances. For instance, AU Optronics successfully demonstrated a 9.4-inch 228-ppi flexible Micro-LED display integrated onto a flexible low-temperature polysilicon thin-film transistor (LTPS-TFT) backplane based on fine-pitch flip-chip bonding, followed by LLO to take off the temporary supporting carrier[12] [Figure 2A]. The display features a high contrast ratio of > 1,000,000:1, a brightness of up to 700 nits, good display uniformity, and minimized color shift at any off-axis viewing angles, revealing its potential for high-resolution flexible automotive applications. Royole Co. demonstrated a 2.7-inch 42-PPI stretchable Micro-LED display based on an island-bridge structure in combination with buckling[64] [Figure 2B]. The obtained device panel can be normally operated under biaxial stretching, free from twisting and poking, revealing its excellent capability for tolerating large deformation.
Figure 2. Deformable Micro-LEDs for applications in (A-D) advanced displays and (E and F) healthcare. (A) 4-inch flexible full-color active-matrix display integrated onto TFT backplane by LLO[12]; (B) Stretchable display based on island-bridge structures[64]; (C) Distortion-free stretchable display based on 2D Kirigami structures defined by laser cutting[10]; (D) 3D foldable display based on an origami structure design[19]; (E) Wearable contact lenses for the treatment of diabetic retinopathy[29]; (F) Wirelessly powered, dual-color implantable probes for optogenetics[28]. Figure 2A adapted with permission from ref.[12]. Copyright 2021, Wiley-VCH; Figure 2B adapted with permission from ref.[64]. Copyright 2021, Wiley-VCH; Figure 2C adapted with permission from ref.[10]. Copyright 2022, Wiley-VCH; Figure 2D adapted with permission from ref.[19]. Copyright 2020, Wiley-VCH; Figure 2E reproduced under the terms of the CC-BY Creative Commons Attribution 4.0 International license[29]; Figure 2F reproduced under the terms of the CC-BY Creative Commons Attribution 4.0 International license[28]. Micro-LED: Micro-scale light-emitting diode; TFT: Thin-film transistor; 2D: two-dimensional; 3D: three-dimensional.
Academic research, on the other hand, has led to the development of some unusual deformable displays with expanded functionalities that common ones cannot provide. Jang et al. demonstrated using Micro-LEDs fabricated on a 2D kirigami electrical circuit board to realize an auxetic distortion-free meta-display with a stretchability of 24.5% and Poisson’s ratio of -1 under uniaxial stretching[10] [Figure 2C]. The concept constitutes a remarkable improvement over the common stretchable displays, which can lead to the deterioration of the reduced resolution per unit area and the blurred display image quality when subject to large mechanical stretch. Kim et al. demonstrated a hexahedral LED array with general row and column control lines by laminating Micro-LED devices to an acrylonitrile butadiene styrene (ABS) film, followed by selective plasticization and transformation, forming nondisruptive tucking-based origami at the electronics level[19] [Figure 2D]. The novel origami structure and fabrication process, combined with laminated Micro-LEDs, pave the way toward developing 3D foldable displays.
Wearable devices for healthcare
Apart from displays, deformable Micro-LEDs attachable to skins or implantable to human/animal bodies can be used as wearable light sources for healthcare purposes. For instance, Lee et al. demonstrated a wirelessly powered smart contact lens based on infrared Micro-LEDs [Figure 2E][29]. The contact lens with integrated Micro-LEDs can be conformally attached to the eyeball, effectively treating diabetic retinopathy that can cause vision loss and blindness in people with diabetes. Li et al. developed an implantable, wireless-powered dual-color Micro-LED probe for bidirectional optogenetic modulations[28] [Figure 2F]. The lightweight device has good biocompatibility, reduced dimensions and good portability, constituting remarkable improvements over conventional implantable LEDs driven by external wires and batteries, which restrict the natural motion and social interactions of animals. Self-powered, battery-free flexible Micro-LED-based Optogenetic Systems have also been reported to further reduce the system weight[20,65]. Zhang et al. demonstrated a wirelessly powered Micro-LED patch for local tissue oximetry, which allows effective monitoring of the regional tissue oxygenation in animal models[66]. Such a Micro-LED-based tissue oximeter can create many opportunities for studying various O2-mediated processes in naturally behaving subjects, with implications in biomedical research and clinical practice. Phan et al. reported a flexible and wireless Micro-LED patch with an internet of things (IoT) healthcare platform for wound healing applications, which opens tremendous opportunities for remote healthcare with cost-effectiveness in the future[67].
CONCLUSIONS AND REMARKS
Technological advancements in substrate removal, transfer printing and mechanical designs have enabled the fabrication of deformable Micro-LEDs in flexible/stretchable formats. These devices not only reserve their excellent optoelectronic performance but also exhibit substantially improved flexibility and better reliability than rigid counterparts, making them suited for potential application in areas such as unusual deformable displays and wearable devices for healthcare. It is envisioned that deformable Micro-LEDs will lead to a technological revolution in the future display industry.
However, before practical applications of deformable Micro-LEDs, several issues need to be addressed. First, further improving the mechanical properties of Micro-LEDs is urgently required. While some novel mechanical designs, such as island-bridge and buckling structures, can be adopted to enhance the system stretchability, these concepts lead to reduced pixel resolution and compromised filling factors. Innovations in intrinsically stretchable conductors/substrates[68] (for instance, replacing the meandering interconnects using intrinsically stretchable conductors/substrates) and more accurate micro-assembly processes should be considered to simultaneously achieve excellent stretchability and resolution. Vertical stacking of multi-color Micro-LED chips may be another useful strategy toward further enhanced resolution of the flexible device[38]. Second, the long-term operation stability and biocompatibility of such deformable Micro-LEDs in bio-environments needs to be clearly established, especially for healthcare purposes. These devices encapsulated by bio-polymer generally can tolerate harsh environment attacks. However, whether they have any long-term side effects on the skin or human organs needs to be confirmed. It has been known, for example, that wearable devices with poor air permeability can affect the long-term wearing comfort[69]. Bonding failure at the Micro-LED/soft substrate interface is another issue worth future investigation. It may be worthwhile to develop intrinsically stretchable solders that can enhance the interfacial bonding strength. It is also urgently needed to develop flexible Micro-LEDs with full-color emitting capability. Monochromic Micro-LEDs combined with flexible quantum dot patterns[70], for example, may be a practical approach toward full-color wearable displays in the future. Finally, a system-scale, universal fabrication process for wearable Micro-LEDs is required. Currently, most fabrication techniques are only demonstrated at the laboratory, lacking consistent reproducibility. There is still a long way to extend the lab concept to large-scale fabrication.
DECLARATIONS
Authors’ contributions
Wrote the original draft: Zou S, Li Y, Gong Z
Supervised, reviewed and revised the manuscript: Gong Z
Availability of data and materials
Not applicable.
Financial support and sponsorship
This work was supported by the National Key Research and Development Program of China (Grant No. 2021YFB3600100); the National Natural Science Foundation of China (Grant Nos. 62104049 and 62304055); the Key-Area Research and Development Program of Guangdong Province (Grant Nos. 2019B010925001, 2020B010183001, and 2020B0101320002); the Guangdong Academy of Sciences’ Project of Science and Technology Development (Grant Nos. 2022GDASZH-2022010111, 2019GDASYL-0103070, 2019GDASYL-0103071, 2020GDASYL-20200103118, and 2021GDASYL-20210103067); the Guangzhong Basic and Applied Basic Research Foundation (Grant No. 202201010572); the Guangdong Basic and Applied Basic Research Foundation (Grant Nos. 2022A1515110397, 2020B1515120020, 2020A1515110509 and 2019A1515110682).
Conflicts of interest
All authors declared that there are no conflicts of interest.
Ethical approval and consent to participate
Not applicable.
Consent for publication
Not applicable.
Copyright
© The Author(s) 2024.
REFERENCES
1. Jiang HX, Jin SX, Li J, Shakya J, Lin JY. III-nitride blue microdisplays. Appl Phys Lett 2001;78:1303-5.
2. Gong Z, Zhang HX, Gu E, et al. Matrix-addressable micropixellated InGaN light-emitting diodes with uniform emission and increased light output. IEEE Trans Electron Dev 2007;54:2650-8.
3. Liu Z, Lin CH, Hyun BR, et al. Micro-light-emitting diodes with quantum dots in display technology. Light Sci Appl 2020;9:83.
4. Chen D, Chen YC, Zeng G, Zhang DW, Lu HL. Integration technology of micro-LED for next-generation display. Research 2023;6:0047.
5. Lee HE, Shin JH, Park JH, et al. Micro light-emitting diodes for display and flexible biomedical applications. Adv Funct Mater 2019;29:1808075.
6. Pan Z, Guo C, Wang X, et al. Wafer-scale micro-LEDs transferred onto an adhesive film for planar and flexible displays. Adv Mater Technol 2020;5:2000549.
7. Li C, Pan Z, Guo C, et al. Transfer printed, vertical GaN-on-silicon micro-LED arrays with individually addressable cathodes. IEEE Trans Electron Dev 2022;69:5630-6.
8. Gong Z, Jin S, Chen Y, et al. Size-dependent light output, spectral shift, and self-heating of 400 nm InGaN light-emitting diodes. J Appl Phys 2010;107:013103.
9. Liu H, Li H, Wang Z, et al. Robust and multifunctional kirigami electronics with a tough and permeable aramid nanofiber framework. Adv Mater 2022;34:e2207350.
10. Jang B, Won S, Kim J, et al. Auxetic meta-display: stretchable display without image distortion. Adv Funct Mater 2022;32:2113299.
11. Hu L, Choi J, Hwangbo S, et al. Flexible micro-LED display and its application in Gbps multi-channel visible light communication. npj Flex Electron 2022;6:100.
12. Lee S, Cheng C, Liu C, Yeh C, Lin Y. 9.4-inch 228-ppi flexible micro-LED display. J Soc Info Display 2021;29:360-9.
13. Choi M, Jang B, Lee W, et al. Stretchable active matrix inorganic light-emitting diode display enabled by overlay-aligned roll-transfer printing. Adv Funct Mater 2017;27:1606005.
14. Lee SY, Park K, Huh C, et al. Water-resistant flexible GaN LED on a liquid crystal polymer substrate for implantable biomedical applications. Nano Energy 2012;1:145-51.
15. Kim RH, Kim DH, Xiao J, et al. Waterproof AlInGaP optoelectronics on stretchable substrates with applications in biomedicine and robotics. Nat Mater 2010;9:929-37.
16. Park SI, Xiong Y, Kim RH, et al. Printed assemblies of inorganic light-emitting diodes for deformable and semitransparent displays. Science 2009;325:977-81.
17. Guo C, Pan Z, Li C, et al. Large-scale programmable assembly of functional micro-components for advanced electronics via light-regulated adhesion and polymer growth. npj Flex Electron 2022;6:44.
18. Zhang H, Rogers JA. Recent advances in flexible inorganic light emitting diodes: from materials design to integrated optoelectronic platforms. Adv Opt Mater 2019;7:1800936.
19. Kim G, Kim Y, Yoo S, Jang HS, Ko HC. Hexahedral LED arrays with row and column control lines formed by selective liquid-phase plasticization and nondisruptive tucking-based origami. Adv Mater Technol 2020;5:2000010.
20. Zhou L, Zhang Y, Cao G, et al. Wireless self-powered optogenetic system for long-term cardiac neuromodulation to improve post-MI cardiac remodeling and malignant arrhythmia. Adv Sci 2023;10:e2205551.
21. Guan S, Tian H, Yang Y, et al. Self-assembled ultraflexible probes for long-term neural recordings and neuromodulation. Nat Protoc 2023;18:1712-44.
22. Yang Y, Wu M, Wegener AJ, et al. Preparation and use of wireless reprogrammable multilateral optogenetic devices for behavioral neuroscience. Nat Protoc 2022;17:1073-96.
23. Wu Y, Wu M, Vázquez-Guardado A, et al. Wireless multi-lateral optofluidic microsystems for real-time programmable optogenetics and photopharmacology. Nat Commun 2022;13:5571.
24. Kathe C, Michoud F, Schönle P, et al. Wireless closed-loop optogenetics across the entire dorsoventral spinal cord in mice. Nat Biotechnol 2022;40:198-208.
25. Lee J, Lee S, Kim D, Jae Lee K. Implantable Micro-Light-Emitting Diode (µLED)-based optogenetic interfaces toward human applications. Adv Drug Deliv Rev 2022;187:114399.
26. Rajalingham R, Sorenson M, Azadi R, Bohn S, DiCarlo JJ, Afraz A. Chronically implantable LED arrays for behavioral optogenetics in primates. Nat Methods 2021;18:1112-6.
27. Lee HE, Choi J, Lee SH, et al. Monolithic flexible vertical GaN light-emitting diodes for a transparent wireless brain optical stimulator. Adv Mater 2018;30:e1800649.
28. Li L, Lu L, Ren Y, et al. Colocalized, bidirectional optogenetic modulations in freely behaving mice with a wireless dual-color optoelectronic probe. Nat Commun 2022;13:839.
29. Lee GH, Jeon C, Mok JW, et al. Smart wireless near-infrared light emitting contact lens for the treatment of diabetic retinopathy. Adv Sci 2022;9:e2103254.
30. Lingley AR, Ali M, Liao Y, et al. A single-pixel wireless contact lens display. J Micromech Microeng 2011;21:125014.
31. Park Y, Cha E, An HS, et al. Wireless phototherapeutic contact lenses and glasses with red light-emitting diodes. Nano Res 2020;13:1347-53.
32. Park J, Kim J, Kim SY, et al. Soft, smart contact lenses with integrations of wireless circuits, glucose sensors, and displays. Sci Adv 2018;4:eaap9841.
33. Takamatsu T, Chen Y, Yoshimasu T, Nishizawa M, Miyake T. Highly efficient, flexible wireless-powered circuit printed on a moist, soft contact lens. Adv Mater Technol 2019;4:1800671.
34. Lee GH, Moon H, Kim H, et al. Multifunctional materials for implantable and wearable photonic healthcare devices. Nat Rev Mater 2020;5:149-65.
35. Gong Z. Layer-scale and chip-scale transfer techniques for functional devices and systems: a review. Nanomaterials 2021;11:842.
36. Sun W, Ji L, Lin Z, et al. 20 µm micro-LEDs mass transfer via laser-induced in situ nanoparticles resonance enhancement. Small 2024:e2309877.
37. Wang L, Yang S, Zhou F, et al. Wafer-scale transferrable GaN enabled by hexagonal boron nitride for flexible light-emitting diode. Small 2024;20:e2306132.
38. Shin J, Kim H, Sundaram S, et al. Vertical full-colour micro-LEDs via 2D materials-based layer transfer. Nature 2023;614:81-7.
39. Zhang S, Liu B, Ren F, et al. Graphene-nanorod enhanced quasi-Van Der Waals epitaxy for high indium composition nitride films. Small 2021;17:e2100098.
40. Yu J, Wang L, Hao Z, et al. Van der Waals epitaxy of III-nitride semiconductors based on 2D materials for flexible applications. Adv Mater 2020;32:e1903407.
41. Lee CH, Kim YJ, Hong YJ, et al. Flexible inorganic nanostructure light-emitting diodes fabricated on graphene films. Adv Mater 2011;23:4614-9.
42. Kim Y, Cruz SS, Lee K, et al. Remote epitaxy through graphene enables two-dimensional material-based layer transfer. Nature 2017;544:340-3.
43. Cheng CW, Shiu KT, Li N, Han SJ, Shi L, Sadana DK. Epitaxial lift-off process for gallium arsenide substrate reuse and flexible electronics. Nat Commun 2013;4:1577.
44. Schermer JJ, Mulder P, Bauhuis GJ, et al. Epitaxial Lift-Off for large area thin film III/V devices. Phys Status Solidi A 2005;202:501-8.
45. Lin C, Dai J, Wang G, Lin M. Chemical lift-off process for blue light-emitting diodes. Appl Phys Express 2010;3:092101.
46. Lin M, Lin C, Huang W, et al. Chemical-mechanical lift-off process for InGaN epitaxial layers. Appl Phys Express 2011;4:062101.
47. Chen Q, Yang K, Shi B, et al. Principles for 2D-material-assisted nitrides epitaxial growth. Adv Mater 2023;35:e2211075.
48. Liang D, Wei T, Wang J, Li J. Quasi van der Waals epitaxy nitride materials and devices on two dimension materials. Nano Energy 2020;69:104463.
49. Chung K, In Park S, Baek H, Chung J, Yi G. High-quality GaN films grown on chemical vapor-deposited graphene films. NPG Asia Mater 2012;4:e24.
50. Choi JH, Cho EH, Lee YS, et al. Fully flexible GaN light-emitting diodes through nanovoid-mediated transfer. Adv Opt Mater 2014;2:267-74.
51. Huang S, Zhang Y, Leung B, et al. Mechanical properties of nanoporous GaN and its application for separation and transfer of GaN thin films. ACS Appl Mater Interfaces 2013;5:11074-9.
52. Choi W, Kim CZ, Kim CS, et al. A repeatable epitaxial lift-off process from a single GaAs substrate for low-cost and high-efficiency III-V solar cells. Adv Energy Mater 2014;4:1400589.
53. Kirk AP, Cardwell DW, Wood JD, et al. Recent progress in epitaxial lift-off solar cells. In: 2018 IEEE 7th World Conference on Photovoltaic Energy Conversion (WCPEC) (A Joint Conference of 45th IEEE PVSC, 28th PVSEC & 34th EU PVSEC); 2018 Jun 10-15; Waikoloa, HI, USA. IEEE; 2018. pp. 32-5.
54. Park SH, Kim TJ, Lee HE, et al. Universal selective transfer printing via micro-vacuum force. Nat Commun 2023;14:7744.
55. Chang W, Kim J, Kim M, et al. Concurrent self-assembly of RGB microLEDs for next-generation displays. Nature 2023;617:287-91.
56. Meitl MA, Zhu Z, Kumar V, et al. Transfer printing by kinetic control of adhesion to an elastomeric stamp. Nature Mater 2006;5:33-8.
57. Carlson A, Bowen AM, Huang Y, Nuzzo RG, Rogers JA. Transfer printing techniques for materials assembly and micro/nanodevice fabrication. Adv Mater 2012;24:5284-318.
58. Gong Y, Gong Z. Laser-based micro/nano-processing techniques for microscale LEDs and full-color displays. Adv Mater Technol 2023;8:2200949.
59. Lee D, Cho S, Park C, et al. Fluidic self-assembly for MicroLED displays by controlled viscosity. Nature 2023;619:755-60.
60. Rao Z, Lu Y, Li Z, et al. Curvy, shape-adaptive imagers based on printed optoelectronic pixels with a kirigami design. Nat Electron 2021;4:513-21.
61. Jiao R, Wang R, Wang Y, et al. Vertical serpentine interconnect-enabled stretchable and curved electronics. Microsyst Nanoeng 2023;9:149.
62. Biswas S, Schoeberl A, Hao Y, et al. Integrated multilayer stretchable printed circuit boards paving the way for deformable active matrix. Nat Commun 2019;10:4909.
63. Yu S, Deng Y, Cheung YK, Yu H. A biaxially stretchable and washable LED Display enabled by a wavy-structured metal grid. J Microelectromech Syst 2022;31:771-6.
64. Kang J, Luo H, Tang W, et al. 71-2: enabling processes and designs for tight-pitch micro-LED based stretchable display. Symp Dig Tech Pap 2021;52:1056-9.
65. Liu Z, Zhou Y, Qu X, et al. A self-powered optogenetic system for implantable blood glucose control. Research 2022;2022:9864734.
66. Zhang H, Gutruf P, Meacham K, et al. Wireless, battery-free optoelectronic systems as subdermal implants for local tissue oximetry. Sci Adv 2019;5:eaaw0873.
67. Phan DT, Mondal S, Tran LH, et al. A flexible, and wireless LED therapy patch for skin wound photomedicine with IoT-connected healthcare application. Flex Print Electron 2021;6:045002.
68. Zhao Z, Liu K, Liu Y, Guo Y, Liu Y. Intrinsically flexible displays: key materials and devices. Natl Sci Rev 2022;9:nwac090.
69. Chen F, Huang Q, Zheng Z. Permeable conductors for wearable and on-skin electronics. Small Struct 2022;3:2100135.
Cite This Article
Export citation file: BibTeX | EndNote | RIS
OAE Style
Zou S, Li Y, Gong Z. Shape-deformable Micro-LEDs for advanced displays and healthcare. Soft Sci 2024;4:19. http://dx.doi.org/10.20517/ss.2024.13
AMA Style
Zou S, Li Y, Gong Z. Shape-deformable Micro-LEDs for advanced displays and healthcare. Soft Science. 2024; 4(2): 19. http://dx.doi.org/10.20517/ss.2024.13
Chicago/Turabian Style
Shenghan Zou, Yuzhi Li, Zheng Gong. 2024. "Shape-deformable Micro-LEDs for advanced displays and healthcare" Soft Science. 4, no.2: 19. http://dx.doi.org/10.20517/ss.2024.13
ACS Style
Zou, S.; Li Y.; Gong Z. Shape-deformable Micro-LEDs for advanced displays and healthcare. Soft. Sci. 2024, 4, 19. http://dx.doi.org/10.20517/ss.2024.13
About This Article
Special Issue
Copyright
Data & Comments
Data
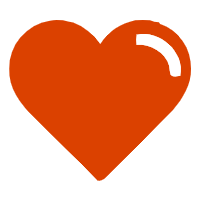
Comments
Comments must be written in English. Spam, offensive content, impersonation, and private information will not be permitted. If any comment is reported and identified as inappropriate content by OAE staff, the comment will be removed without notice. If you have any queries or need any help, please contact us at support@oaepublish.com.