Possible application of Akkermansia muciniphila in stress management
Abstract
Akkermansia muciniphila (A. muciniphila) is a promising candidate bacterium for stress management due to its beneficial effects on the microbiota-gut-brain axis (MGBA). As a well-known mucin-degrading bacterium in the digestive tract, A. muciniphila has demonstrated significant benefits for host physiology. Recent research highlights its potential in treating several neuropsychiatric disorders. Proposed mechanisms of action include the bacterium’s outer membrane protein Amuc_1100 and potentially its extracellular vesicles (EVs), which interact with host immune receptors and influence serotonin pathways, which are crucial for emotional regulation. Despite its potential, the administration of probiotics containing A. muciniphila faces technological challenges, prompting the development of pasteurized forms recognized as safe by the European Food Safety Authority (EFSA). This review systematically examines the existing literature on the role of A. muciniphila in stress management, emphasizing the need for further research to validate its efficacy. The review follows a structured methodology, including comprehensive database searches and thematic data analysis, to provide a detailed understanding of the relationship between stress, microbiota, and A. muciniphila therapeutic potential.
Keywords
INTRODUCTION
Akkermansia muciniphila mucin degradation and psychobiotic mechanisms of action
Generally, mucin degradation in the digestive tract has been considered harmful to host health. However, Akkermansia muciniphila (A. muciniphila), a mucus-degrading bacterium of human gut microbiota, benefits one’s physiology[1]. An increasing amount of evidence indicates that this bacterium has beneficial systemic effects on host health, primarily by enhancing immunological and metabolic functions, making it a promising potential probiotic[2]. Recent clinical and preclinical research has demonstrated that
Overall, the benefits of harboring A. muciniphila might not only be due to mucus degradation promoting the renewal of the mucus layer and maintaining gut barrier function, but also because of the production of EVs that can interact with the host’s gut epithelium, improving gut barrier function, reducing body weight gain, and enhancing glucose tolerance. Other mechanisms that have been proposed include[9]:
1. Pilus-associated signaling (PAS) protein (Amuc_1100), which can interact with host immune receptors like TLR2-, enhancing gut barrier function and having immunomodulatory effects, including reducing inflammation and promoting the production of beneficial cytokines.
2. Short-chain fatty acids (SCFAs) affecting gut health by interacting with host receptors (e.g., FFAR2, FFAR3) to regulate inflammation and gut hormone release.
3. Metabolite Harmaline modulating host immune responses, enhancing bile acid signaling and reducing inflammation.
4. Glucagon-like peptide-1 (GLP-1)-inducing protein (P9) involved in regulating glucose homeostasis and energy balance.
5. Peptidoglycan muropeptides activating immune receptors such as nucleotide-binding oligomerization domain 1 (NOD1) and NOD2, contributing to gut immune responses and maintaining gut health.
6. Membrane lipids (e.g., diacyl phosphatidylethanolamine), which can activate immune receptors like toll-like receptors (TLR2-TLR1) to induce anti-inflammatory cytokine production.
These modes of action collectively contribute to the beneficial effects of A. muciniphila on gut health, including enhancing gut barrier function, modulating immune responses, and improving metabolic conditions.
The psychobiotic potential of A. muciniphila is very promising; however, due to many technological problems, the administration of probiotics containing this strain is very complicated. For this reason, technology has been developed to produce pasteurized A. muciniphila, which has comparable or better properties than the live bacterium and has also been recognized by the European Food Safety Authority (EFSA) as a novel food that can be safely administered to humans[15]. As mentioned above, there are substantial data on the potential use of A. muciniphila in neuropsychiatric disorders; however, little is known about the effect of this bacterium on stress specifically, which plays a crucial role in the onset of many neurobiological disorders and has recently become a critical mental health issue[16]. Therefore, in this narrative review, we decided to describe the relationship between stress and the microbiota. To achieve this, we aimed to analyze existing work on the effects of A. muciniphila on stress to organize published knowledge and guide further research on this topic.
STRESS AND MICROBIOTA
Stress definition and phases
Current psychological descriptions of stress are dominated by the relational view, which states that “stress is a dynamic relationship between an individual and the environment that is judged by the individual to require adaptive effort or to be beyond the ability to cope. Recognizing a relationship as stressful is determined by the subjective evaluation of the individual rather than by the objective properties of the situation”[17].
Stress, in the initial phase, is a highly motivating factor leading to the activation of psychological processes, mediated by the hypothalamic-pituitary-adrenal (HPA) axis and sympathetic-adreno-medullar (SAM) axis, thus enabling effective work on a given problem. On the other hand, prolonged and more severe stress significantly decreases psychological activities. If this condition persists or if the intensity of the stress increases rapidly, a so-called destructive phase of stress occurs, in which cognitive and executive functions are impaired to a large extent[18]. The perception of stress is a highly subjective process, as is the individual’s stress resilience. Physiologically, however, the body’s response to stress follows specific patterns explained by biochemical pathways and processes[19]. With deeper analysis, it could be theorized that almost any event or stimulus can be a cause of stress subjectively. Physiological occurrences such as hunger, thirst, sleep deprivation or hormonal fluctuations can also be stressful for the body. Adding to the complexity, these stimuli are processed in different regions of the brain. As a response to a perceived stressful situation, various autonomic nervous system and emotional reactions are triggered[20,21]. In the scope of this review, stress is defined as a dynamic relationship requiring adaptive effort. Initial stress is motivating, but prolonged stress decreases psychological activities, leading to impaired cognitive and executive functions.
Physiological responses to stress
A stressful stimulus triggers an immediate sympathetic nervous system response that releases adrenaline and noradrenaline from the adrenal glands. The HPA axis is activated with prolonged stress, resulting in the adrenal glands secreting cortisol. This stress hormone affects lipid, carbohydrate and protein metabolism and immune function. These biochemical and physiological changes manifest as heart palpitations, accelerated breathing, trembling hands, dry mouth, sweating, and/or intestinal reactions such as abdominal cramps, pain, and diarrhea[22,23]. Consequently, the body, with the help of the CNS and hormones, not only minimizes intestinal blood supply and mucus production but also purges food by inducing diarrhea and vomiting. The blood, along with the energy supply, is redirected to the muscles and brain, coordinating the cascade of the “fight or flight response”. These processes allowed humans to survive throughout evolution and continue to impact the body even though stress stimuli have changed. In stress-sensitive people, public speaking or financial troubles activate the “catastrophic program” in the brain in the same way that the threat of a wild animal attack or a flood previously did. It is well established that chronic stress affects gastrointestinal, physical, and mental health[24]. Examples of these manifestations are disorders of gut-brain interactions (DGBIs)[25-27], including irritable bowel syndrome[28-30] or functional dyspepsia[31]. However, the majority of chronic gastrointestinal disorders are also frequently associated with high comorbidity of psychological disorders[32].
Chronic stress is one of the factors that play a role in the onset of psychiatric disorders[33]. This relationship is illustrated by the stress susceptibility model [Figure 1][34].
Figure 1. Stress vulnerability model. Created with BioRender software.
This model illustrates the psychological theory that there is a link between internal and external factors and stress susceptibility, which can impact the risk of various diseases, including mental disorders. In this theory, internal factors include genetics, biological and cellular factors, and gut microbiota composition[35]. External factors or buffering factors are psychosocial factors such as financial stability, a regulated lifestyle, a sense of security, or education that can impact the ability to respond to stress. These factors collectively determine how much stress an individual can endure before decompensation occurs. Decompensation manifests as atypical behavior when a person cannot regulate their emotions. It is a natural defense mechanism but can lead to mental and physical illness if persistent[36,37]. Standard therapies often overlook internal factors, like the gut microbiota, which are crucial for stress management. Stimuli affecting the brain are interpreted based on past experiences. If a stimulus is perceived as stressful, the brain triggers biochemical reactions, including cortisol release, impacting organs like the gut. The gut microbiota then signals the brain about the body’s state. Favorable internal factors help manage stress and prevent decompensation. Modulating these factors can break the vicious cycle and improve therapeutic outcomes[35].
MICROBIOTA AND STRESS
Microbiota and neuromodulation
The fact that various microorganisms inhabit the gut has been known for years. However, it is recent scientific research that has begun to elucidate the functions and significance of the intestinal “microbiota”, which refers to the collection of microorganisms populating the gut. It is known that the gut microbiota is a critical element in the functioning of the brain-gut axis and that it has an impact not only on physical processes but also on mental processes. The gut microbiota plays an essential role in the human body, such that some scientists call it a separate organ[38]. The composition of the gut microbiota is individual to each person and is constantly changing. The number and type of microorganisms in the different levels of the gastrointestinal tract vary. Environmental conditions such as the availability of oxygen and nutrients, the pH value, the intestinal transit time, and even the structure and immunological properties of the intestinal epithelium determine the number and type of microorganisms that populate them. In addition, gender and epigenetic factors such as diet, medications, stimulants, past medical history, latitude, and age influence the formation of each individual’s gut microbiota[39]. Despite this, scientists are discovering more and more common traits and are gaining a better understanding of the individual microbiota and its impact on bodily function and health, both physical and mental[40].
The microbiota significantly impacts the formation and modulation of the gut-brain axis[41]. Researchers have found that the gut microbiota is also involved in developing the hippocampus responsible for memory, the amygdala as the brain’s alarm center, and the myelination processes of prefrontal cortex neurons responsible for executive functions[42,43]. Furthermore, the metabolic activity of the microbiota is comparable to that of the liver. The microorganisms that make up the intestinal ecosystem break down undigested food residues by fermentation and actively and rapidly eliminate harmful antigens in the intestinal lumen. The intestinal microbiota produces vitamin K and B vitamins and, by producing hydrolase, aids in the efficient digestion of lipids. Pivotal metabolites are SCFAs, which are essential for adequately functioning of intestinal cells. Microbes stimulate the synthesis of mucins, which protect the endothelium from invasion by pathogens and toxins. In addition, they affect the CNS by producing and modulating the concentration of selected neuromediators such as serotonin, histamine, acetylcholine, norepinephrine, melatonin, and gamma-aminobutyric acid (GABA). They also affect the expression of receptors for these neurotransmitters in the brain by stimulating immune cells to release pro- and anti-inflammatory cytokines[44]. Furthermore, the gut microbiota impacts blood cortisol levels, affecting the functioning of various organs and exacerbating the stress spiral. Numerous scientific studies confirm the link between high levels of stress and the development of mental illnesses. Therefore, a favorable composition of the gut microbiota may be a factor that helps protect the body from mental decompensation and can contribute to one’s perception and tolerance of stress[35]. Overall, the gut microbiota is crucial for the brain-gut axis, affecting physical and mental processes. It influences brain development, immune responses, and the production of neuromediators and their receptors, impacting stress resilience.
Stress-induced microbiota alterations and mental health
The significance of stress impact on the composition and function of host gut microbiota has been demonstrated in several preclinical studies. For example, a decrease in Lactobacillus[45] and an increase in Clostridia[46] in mice exposed to different types of stress have been documented. In contrast to studies in rodents, human studies are scarce and focus mainly on naturalistic stressors such as academic examinations or negative events, but they provide conflicting results[47,48]. In one of the recent studies among COVID-19 frontline healthcare workers (FHWs), experiencing stressful events has been associated with a disrupted gut microbiome for at least half a year. Microbes positively associated with good mental health were Faecalibacterium spp. and [Eubacterium] eligens group spp., which have anti-inflammatory properties. It was found that a low abundance of [Eubacterium] hallii group and a high abundance of Bacteroides eggerthii shortly after frontline work stress exposure were associated with the reappearance of posttraumatic stress symptoms[49].
Numerous scientific papers also confirm the relationship between stress intensity and gut microbiota composition, a crucial part of the brain-gut axis[50]. It is also known that a specific composition of the gut microbiota, different from the healthy population, can be observed in mentally ill patients[51-54]. Thus, since stress takes part in the pathophysiology of mental illness and simultaneously has an impact on the gut microbiota, it seems essential to have an in-depth understanding of the gut microbiota as potential diagnostic and therapeutic options in case of mental disorders. Of note, psychiatric pharmacotherapy causes significant shifts in gut microbiome balance[55], which further increases the risk of cardiometabolic disorders. The problem becomes further magnified as these effects are one of the main reasons for discontinuing treatment. Consequently, a high risk of recurrence of symptoms and a renewed disintegration of life might occur[35,55].
Scientists have acknowledged the importance of microbiota balance in maintaining the integrity of the intestinal barrier and overall well-being. Animal experiments provided essential data on how stressful events affect the gut barrier integrity[56-58]. On the other hand, microbiota maintains gut barrier integrity and affects the blood-brain barrier (BBB). Microbial alterations, frequently called dysbiosis, might impair the BBB[59]. Stress-induced microbiota alterations lead to allostatic disturbances. The term allostasis was formed to explain the physiological adaptation mechanisms to environmental stressors, which were regulated by various mediators, including hormones, neurotransmitters, oxidative stress markers, and inflammatory processes. The short-term mobilization of allostatic mechanisms enables counteraction of negative outcomes of stress. However, chronic activation of these mediators [allostatic load (AL)] harms human health. Allostatic overload leads to negative physical and emotional outcomes and has been associated with mental and somatic disorders, including metabolic and cardiovascular diseases. It was proposed that an imbalance within gut microbiota might be a consequence of AL[60,61].
In conclusion, stress alters gut microbiota composition, influencing mental health. Specific microbiota compositions are linked to mental illnesses, suggesting potential diagnostic and therapeutic options for mental disorders.
Psychobiotics in stress management
Psychobiotics are defined as live organisms (probiotics) and prebiotics that, when ingested in adequate amounts, have a positive impact on the MGBA[62,63]. Animal studies have shown that gut microbiota modified by probiotics, antibiotics (ABX), and gnotobiosis (germ-free model) might significantly alter behavioral outcomes in rodents exposed to stress[64,65]. For instance, the intake of various probiotics was demonstrated to improve stress-induced anxiety and depressive-like behaviors in mice[63]. Therapeutic strategies aimed at modulation of the MGBA were proven effective as novel treatment practices in future psychiatric care. For instance, it has been well documented that administration of Lactobacillus helveticus Rosell-52 to animals exposed to stress is associated with diminished pathogen adherence to intestinal mucosa, decreased microbial translocation, and lowered synthesis of pro-inflammatory cytokines. These events have been associated with a protective effect on the limbic system altered by chronic exposure to stress[66,67]. In humans, such supplementation showed beneficial effects on mental health, including alleviating stress and anxiety. Clinical trials have documented that the administration of Lactobacillus helveticus Rosell-52 and Bifidobacterium longum Rosell-175 in healthy individuals has been associated with decreased stress-related gastrointestinal symptoms. The oral use of these probiotic strains was associated with better mood, lower perceived stress intensity, and decreased cortisol release[68,69]. Canadian Natural and Non-prescription Health Products Directorate issued the following license approvals related to psychobiotic use: a probiotic product composed of Lactobacillus helveticus Rosell-52 and Bifidobacterium longum Rosell-175: (i) aids in lowering overall symptoms of anxiety; (ii) supports emotional balance; (iii) aids in the management of stress-related gastrointestinal symptoms[70].
METHODS
The methodology for this study followed the scoping review framework initially developed by Arksey and O’Malley[71], with subsequent updates[72]. The study was conducted in five main phases:
1. Formulating the research question: The question posed was, “What is known about gut microbiota alterations under the influence of A. muciniphila regarding its impact on health conditions in case of prolonged exposure to stress?” This broad question covered as many aspects of the topic as possible.
2. Identifying relevant studies: We searched PubMed and Google Scholar databases using key terms such as A. muciniphila AND (emotions OR psychiatric diseases OR psychopathology OR stress. Additionally, we manually reviewed references from relevant reviews discussing the overall impact of A. muciniphila on health. Only studies in English available by December 1, 2023, were included, with no restrictions on publication date.
3. Selecting studies: We included studies involving animals and humans that provided data on the intervention with A. muciniphila (live or pasteurized A or its components) to confer mental health benefits. The first and senior authors conducted the selection process over two weeks.
4. Charting the data: Data were extracted from study protocols, including stress model type, intervention, analytical techniques, key outcomes, conclusions, and limitations.
5. Collating, summarizing, and reporting the results: Data were thematically organized as paragraphs and a Table.
At the beginning of the article, we added a paragraph describing stress response in humans and the impact of stress on gut microbiota, the latter one being followed by a short communication on psychobiotics.
A. MUCINIPHILA AND STRESS MANAGEMENT
Psychobiotic potential and challenges
Live psychobiotics have certain limitations concerning their standardization and shelf-life stability[73,74]. The alternative is using postbiotics, which contain inactivated microorganisms and/or their components[75]. One novel postbiotic that interests scientists and clinicians is pasteurized A. muciniphila[76]. These bacteria are produced by anaerobic growth followed by pasteurization and freeze-drying. A. muciniphila is an anaerobe and Gram-negative bacterium, with an approximate gut relative abundance of 1% to 3%; however, the abundance of this bacterium is variable and can be much lower in many individuals and some may not harbor it. A. muciniphila subsists in the intestinal mucus layer and contacts epithelial cells at the top of villi. Intestinal mucins are a significant carbon and nitrogen source for producing SCFAs like acetate and propionate[77].
Since its discovery, A. muciniphila has been used successfully in multiple clinical phenotypes, predominantly of metabolic origin[78]. Discovering the mechanism through which pasteurized
Review of experimental evidence
Pasteurized A. muciniphila was experimentally demonstrated to restore gut barrier integrity, a key element of the proper flow of signals within the MGBA[88]. Additionally, a body of evidence exists to link TLR signaling with stress response and psychiatric phenotypes[89-93]. Thus, a postulation on the potential use of
One of the very first analyses was conducted by Chen et al. in male C57BL/6N mice, which were subjected to chronic restraint stress (CRS), an established protocol to achieve a mouse model of depression, and further given dextran sodium sulfate (DSS) to induce colitis[94]. In the second stage of the experiment, mice, after microbiota depletion with the ABX cocktail, received fecal microbiota transplantation (FMT) with
Another study in an animal model by Ding et al. was conducted using C57BL/6 male mice aged 6-8 weeks[95]. Three groups of rodents were analyzed: mice subjected to CRS (3 weeks), mice not subjected to CRS, and mice treated with A. mucinphila after CRS (3 weeks, 5 × 108 CFU/mL via oral gavage). After the treatment protocol, mice were subjected to behavioral tests and hippocampal biospecimens were collected to analyze hormones, neurotransmitters, and brain-derived neurotrophic factor (BDNF) RNA expression. Blood was taken to evaluate serum untargeted metabolome and hepatic and renal biochemical parameters. Additionally, cecal microbiota was analyzed using the next generation sequencing (NGS) amplicon-based technique. It was observed that mice treated with A. muciniphila exhibited a significant improvement in the OFT, TST, and FST behavioral tests compared to mice with CRS exposure only. In gene expression analyses, A. muciniphila intervention diminished the level of corticosterone release after CRS exposure, along with elevation in serum dopamine and hippocampal BDNF but not serum serotonin levels.
The differences were significant regarding the CRS exposure group. As far as gut microbiota composition is concerned, differences in structure were found (A. muciniphila vs. CRS-only groups: higher abundance of Verrucomicrobia, lower of Epsilonbacteraeota, Patescibacteria, Chloroflexi, and Acidobacteria; genus level: lower of Helicobacter, Candidatus_Saccharimonas, Eubacterium_brachy_group, and Lachnoclostridium). However, the principal component analysis revealed no differences between the groups. In a functional prediction approach, pathways linked to neurodegenerative diseases were inhibited in the CRS +
The effects of the Amuc_1100 protein, located on the outer membrane of the A. muciniphila, in mouse models of depression were investigated in another experiment[96]. Depression-like behavior was induced in C57BL/6 mice by exposure to six-week-long chronic unpredictable mild stress (CUMS), which is meant to mimic daily life stressors. Four groups were analyzed: control, CUMS, CUMS + antidepressant fluoxetine (FLX) (20 mg/kg), and CUMS + Amuc_1100 (80 µg/day). Treatments were administered by oral gavage during or after CUMS. Treatment with Amuc_1100 at both time points improved the CUMS-induced behavioral deficits significantly, which was not observed with FLX treatment, but the authors did highlight a high statistical tendency toward such. 5-HT levels in the serum and colon increased in FLX and Amuc_1100 interventions compared to CUMS-only group. When the dorsal raphe nucleus (DRN) involved in serotonin synthesis was immunohistochemically analyzed, it was revealed that both interventions elevated the process. The neurocompetent factors: BDNF, CREB1, and 5-HTR1A expression were restored following CUMS exposure when A. muciniphila and FLX were administered.
Similarly, the hippocampal expression of pro-inflammatory cytokines (IL-6, IL-1B, TNF-α), initially elevated by CUMS, lowered significantly in both treatment groups. CUMS diminished the diversity of gut microbiota to a large, but not significant, extent as compared to control mice. The abundance of Bacteroidota was lowered, and the Firmicutes increased in the CUMS group, and both FLX and Amuc_1100 interventions ameliorated these changes. The other CUMS-mediated alterations (higher counts of Clostridia and lower of Bacteroidia) were only partly and non-significantly recapitulated by FLX and Amuc_1100[96].
Similarly, stress induction, in the form of CUMS, and chronic alcohol exposure were utilized in a study by Guo et al.[97]. C57BL/6J specific pathogen free (SPF) mice were treated with A. muciniphila (2.5 × 109 CFU/200 μL) via oral gavage for five weeks. A. muciniphila improved the loss of weight post alcohol exposure, immobility time in TST, and sucrose preference in the sucrose preference test (SPT). In addition, such bacterial supplementation diminished the liver enzyme elevation induced by alcohol. Stress induced via alcohol and CUMS was alleviated with A. muciniphila, which was confirmed by decreasing immobility time in FST and TST and an elevated preference toward sucrose in the SPT. However, A. muciniphila did not alter the mice’s global locomotor abilities, anxiety, or fear memory. The tested intervention increased 5-HT in the prefrontal cortex and the gut, as the expression of the rate-limiting enzyme of 5-HT was not altered between A. muciniphila and vehicle-treated mice exposed to alcohol. On the other hand, it was decreased in the case of CUMS. Consequently, this proved that A. muciniphila enlarges the serotonin pool independently of this limiting bio-catalyzer. A. muciniphila gavage also inhibited the expression of serotonin transporter in the gut but not in the brain for both stress model mice, which can further be responsible for elevated serotonin pool. A. muciniphila also decreased the expression of cFos in enteric nerves, pointing out that such intervention impacts gut-to-brain signaling through these nerves. To add, a very recent study[98] pointed out that modified Amuc_1100 (Amuc_1100Δ80 without 80 N-terminal amino acids with higher affinity for TLR2 as compared to Amuc_1100) in a mouse model of CUMS also upregulated a tryptophan hydroxylase 1 (Tph1) limiting serotonin expression in the gut. Furthermore, as evidenced by this group previously[96], the intervention downregulated the 5-HTR1A-CREB-BDNF signal pathway and restored gut microbiota composition post CUMS exposure. Interestingly, Amuc_1100Δ80 diminished the inflammatory state and downregulated the HPA axis, consequently improving rodent depressive-like behavioral deficits.
In a study by Sun et al., depressive and anxious behaviors were induced via an antibiotic cocktail (ABX; Ampicillin, Streptomycin, and Clindamycin;1 g/L) administration lasting three weeks[8]. One study group received A. muciniphila (1.5 × 109 CFU/200 μL) per day, while the other was gavaged with the Amuc_1100 protein (100 μg/200 μL) per day, and these periods lasted two weeks. ABX was administered continuously during the intervention period. During a series of behavioral tests, it was shown that A. muciniphila/Amuc_1100 alleviated depression-like behavioral deficits induced by the ABX. Specifically, in an OFT, the number of entries into the center and time spent there were significantly elevated in Amuc_1100-treated mice as compared to the group treated with ABX only. The similar observation was recorded in the case of time spent in a lightbox; however, in the case of the entries into the lightbox, a significant improvement was also found for the A. muciniphila-treated group compared to solely ABX-treated mice. As for immobility in the TST, similar improvements were noted, but in the FST, only Amuc_1100 treatment significantly reduced the immobility time. In the case of total distance traveled in the OFT and time in open arms in the elevated plus maze (EPM) test, both treatments showed improvements; however, these were not statistically significant compared to either control (healthy, vehicle-treated mice) or ABX-treated counterparts. When gut microbiota was evaluated, it was demonstrated that ACE, Chao, and Simpson indices decreased due to ABX exposure, with the first ones being recovered with A. muciniphila gavage. The abundance of
Finally, 5-HT levels in serum and hippocampus were increased in the case of both interventions as compared to ABX alone. In contrast, corticosterone levels in serum and mRNA of the glucocorticoid receptor in the hippocampus decreased. The study confirmed that A. muciniphila and Amuc_1100 diminish antibiotic-induced anxiety and depression, affecting different parts of the GMBA, including the BDNF/TrkB signaling pathway and astrocyte activation.
The summary of the above-described studies with their limitations is arranged in Table 1. Based on these studies, it can be stated that animal studies demonstrate that A. muciniphila has the ability to alleviate stress-induced behavioral deficits and improve gut microbiota diversity. Mechanisms include interactions with host receptors and modulation of various stress-related pathways.
Effects of A. muciniphila and its outer membrane protein Amuc_1100 on anxiety and depression-like behavior in mice
Model of stress | Tested animals (age, weight, number, sex) | Intervention (dosage, duration) | Analytical techniques | Samples tested | Outcomes | Conclusions | Limitations | Ref. |
CRS and DSS-induced colitis | Male C57BL/6N mice, 18-20 g, n = 32 (experiment 1) and n = 72 (experiment 2) | A. muciniphila MucT (DSM 22959), 1 × 108 CFU/100 µL, oral gavage for 14 days | Behavioral tests (OFT, TST, FST), ELISA, qRT-PCR, 16S rRNA gene sequencing, RNA sequencing, histology, immunohistochemistry, PAS/AB staining | Serum, colon tissue, cecal microbiota, fecal samples | Behavioral tests: increased distance in OFT, decreased immobility in TST and FST ELISA: decreased corticosterone, increased dopamine and serotonin in serum qRT-PCR: increased BDNF mRNA in hippocampus 16S rRNA gene sequencing: altered gut microbiota composition RNA sequencing: identified DEGs related to gut microbiota and colonic mucus Histology: improved colonic structure Immunohistochemistry: increased MUC2 expression PAS/AB staining: increased number of goblet cells | A. muciniphila supplementation protects against CRS-induced gut microbiota dysbiosis, colonic mucosal barrier damage, and aggravation of colitis | Limited to male mice; effects on females unknown Mechanisms of action not fully elucidated | Chen et al., 2021[94] |
CRS | Male C57BL/6 mice, 6-8 weeks, n = 6 per group | A. muciniphila (ATCC® BAA-835™) (1 × 108 CFU/µL), daily for 3 weeks | Behavioral tests (OFT, FST, TST), ELISA, qRT-PCR, 16S rRNA gene sequencing, untargeted metabolomics | Serum, hippocampus tissue, cecal microbiota | Behavioral tests: increased distance in OFT, decreased immobility in FST and TST ELISA: decreased corticosterone, increased dopamine and serotonin in serum qRT-PCR: increased BDNF mRNA in hippocampus 16S rRNA gene sequencing: altered gut microbiota composition Untargeted metabolomics: identified 23 potential biomarkers in serum | AKK ameliorates depressive-like behavior by regulating gut microbiota, metabolites and increasing BDNF levels | Limited to male mice; female response unknown Short duration of intervention | Ding et al., 2021[95] |
CUMS | Male C57BL/6 mice, 5-6 weeks, n = 4-8 per group | Amuc_1100, 80 µg/200µL, daily for 6 weeks | Behavioral tests (OFT, TST, FST), ELISA, qRT-PCR, 16S rRNA gene sequencing, immunohistochemistry | Serum, colon tissue, brain (hippocampus) tissue, fecal samples | Behavioral tests: increased distance in OFT, decreased immobility in TST and FST ELISA: increased 5-HT in serum and colon, decreased corticosterone qRT-PCR: increased Tph1 in colon, increased BDNF, CREB1, 5-HTR1A in hippocampus 16S rRNA gene sequencing: improved gut microbiota diversity and composition Immunohistochemistry: increased 5-HT in DRN | Amuc_1100 improves depression-like behaviors, increases 5-HT levels in CNS and periphery, modulates gut microbiota, and upregulates BDNF and CREB1 in the hippocampus | The study was only conducted on male mice; the effects on female mice were unknown Mechanisms of MGBA interaction not fully elucidated Limited sample size for some tests Short duration of intervention | Cheng et al., 2021[96] |
CUMS | Male C57BL/6J mice, 6-8 weeks, n = 45 | A. muciniphila (ATTC BAA-835) (2.5 × 109 CFU/200 µL), daily for 5 weeks | Behavioral tests (OFT, FST, TST, SPT), ELISA, qRT-PCR, 16S rRNA gene sequencing, histology, immunohistochemistry | Serum, gut tissue, brain (PFC) tissue | Behavioral tests: increased distance in OFT, decreased immobility in FST and TST, increased sucrose preference in SPT ELISA: increased 5-HT in gut and PFC, decreased corticosterone qRT-PCR: increased Tph1 in gut, increased BDNF in brain 16S rRNA gene sequencing: altered gut microbiota composition Histology: improved colonic structure Immunohistochemistry: increased MUC2 expression | A. muciniphila alleviates depressive-like behaviors by increasing 5-HT levels in the gut and brain, modulating gut microbiota, and improving colonic structure | The study was only conducted on male mice; the effects on female mice were unknown Mechanisms of MGBA interaction not fully elucidated | Guo et al., 2024[97] |
CAE | Male C57BL/6J mice, 8 weeks, n = 48 | A. muciniphila (2.5 × 109 CFU/200 µL), daily for 5 weeks | Behavioral tests (OFT, FST, TST, SPT), ELISA, qRT-PCR, 16S rRNA gene sequencing, histology, immunohistochemistry | Serum, gut tissue, brain (PFC) tissue | Behavioral tests: increased distance in OFT, decreased immobility in FST and TST, increased sucrose preference in SPT ELISA: increased 5-HT in gut and PFC, decreased corticosterone qRT-PCR: increased Tph1 in gut, increased BDNF in brain 16S rRNA gene sequencing: altered gut microbiota composition Histology: improved colonic structure Immunohistochemistry: increased MUC2 expression | A. muciniphila alleviates depressive-like behaviors by increasing 5-HT levels in the gut and brain, modulating gut microbiota, and improving colonic structure | The study was only conducted on male mice; the effects on female mice are unknown Mechanisms of MGBA interaction not fully elucidated | Guo et al., 2024[97] |
CUMS | Male C57BL/6 mice, 5-6 weeks, n = 6 per group | Amuc_1100Δ80 (80 μg/day) for 3 weeks | Behavioral tests (LDB, OFT, EPM, FST, TST), ELISA, qRT-PCR, 16S rRNA gene sequencing, immunohistochemistry | Serum, colon tissue, hippocampus tissue, fecal samples | Behavioral tests: increased entries and time in light chamber (LDB), increased distance traveled (OFT), increased open arm entries (EPM), decreased immobility (FST, TST) ELISA: increased 5-HT in serum and hippocampus, decreased corticosterone qRT-PCR: increased Tph1 in colon, increased BDNF, CREB1, 5-HTR1A in hippocampus 16S rRNA gene sequencing: improved gut microbiota diversity and composition Immunohistochemistry: increased MUC2 expression | Amuc_1100Δ80 alleviates anxiety and depression-like behaviors, modulates gut microbiota, increases 5-HT levels, and enhances the 5-HTR1A-CREB-BDNF signaling pathway | The study was only conducted on male mice; effects on females are unknown Short duration of intervention Mechanisms of MGBA interaction not fully elucidated | Cheng et al., 2022[98] |
Antibiotic-induced anxiety and depression-like behavior | Male C57BL/6 mice, 6 weeks, 20 ± 2 g, n = 32 (Exp 1) and n = 72 (Exp 2) | A. muciniphila (1.5 × 109 CFU/200 µL), Amuc_1100 (100 μg/200 µL), daily for 2 weeks | Behavioral tests (LDB, OFT, EPM, TST, FST), ELISA, qRT-PCR, 16S rRNA gene sequencing, immunohistochemistry | Serum, hippocampus, and fecal samples | Behavioral tests: increased entries and time in light chamber (LDB), increased distance traveled (OFT), increased open arm entries (EPM), decreased immobility (TST, FST) ELISA: increased 5-HT in serum and hippocampus, decreased corticosterone qRT-PCR: increased BDNF, TrkB, GFAP, c-Fos in hippocampus 16S rRNA gene sequencing: altered gut microbiota composition Immunohistochemistry: increased MUC2 expression | A. muciniphila and Amuc_1100 alleviate antibiotic-induced anxiety and depression by modulating the gut microbiota, increasing 5-HT levels, and affecting the BDNF/TrkB signaling pathway | The study was only conducted on male mice; the effects on females are unknown Short duration of intervention The potential impacts of antibiotic-induced gut microbiota changes on overall health are not fully explored | Sun et al., 2023[8] |
Current human studies and applications
To the best of our knowledge, no human studies have evaluated the efficacy of A. muciniphila in alleviating stress manifestations. One study is ongoing: The Efficacy of Pasteurized A. muciniphila in Healthy Medical Workers (MENTAkHEALTH)[99]. Changes in the gut microbiota secondary to chronic exposure to stress can be a trigger for its manifestation in the form of somatic and psychiatric symptoms. The homeostatic disturbances and changes in the intestinal bacterial ecosystem initiate a series of inflammatory disorders in the intestine and the increased synthesis of neurocompetent metabolites, which can negatively affect the enteric nervous system (ENS) and the CNS as described earlier in this review. Medical professionals are susceptible to burnout, anxiety, and depression, but also to irregular lifestyles (e.g., shift work) and functional disorders of the digestive tract[100]. It was also established that an increasing number of students at medical universities abide by psychiatric or psychological therapies and thus often take anxiolytics and antidepressants[101]. Furthermore, research has shown that the use of antipsychotic drugs, especially of the second-generation type, can significantly affect the composition of the intestinal microbiota, increasing the ratio of Firmicutes to Bacteroidetes phyla and affecting the status of A. muciniphila abundance in the gut[102]. Another ongoing project, funded by the European Union, named Imminent Disease Prediction and Prevention at the Environment Host Interface (IMMEDIATE), aims to assess the functioning of the diet-microbiome-immunometabolism axis, which is the gateway for the initiation of the disease process. An intervention study using a so-called novel food, i.e., the pasteurized A. muciniphila with potent neuro- and immunocompetent properties, will evaluate the efficacy of a non-invasive therapy for the homeostasis of clinical biomarkers and the maintenance of well-being. The researchers hypothesize that administration of pasteurized A. muciniphila MucT (commercially available strain) at a dose of 1010 TFU/day for three months may favorably affect gut microbiota and increase intestinal barrier integrity, which will lead to reduced inflammation and negative health effects of stress in healthy participants.
Another very interesting application of pasteurized A. muciniphila MucT is reducing stress in overweight and obese individuals. A proof-of-concept clinical trial showed that pasteurized A. muciniphila MucT improved insulin sensitivity and reduced insulinemia and plasma total cholesterol levels.
In addition, reductions in body weight (without lifestyle or diet modifications permitted), fat mass, and hip circumference were observed compared to those of the placebo group. After three months of supplementation with this postbiotic, a reduction in blood markers of liver dysfunction and inflammation was also observed[103]. The relationship between stress, obesity, and diet is very complex. It has been historically known that stress disturbs cognitive processes, among them executive function and self-regulation. Stress can induce overeating (and elevates leptin, ghrelin, and neuropeptide Y), increase the propensity to choose unhealthy food, and reduce physical activity and sleep, which are critical life behaviors in maintaining health.
Finally, stress activates the HPA and impact-reward processing in the brain and the gut microbiome composition. Complicating matters further, obesity itself can be a stressful condition due to the high prevalence of weight stigma[104]. Therefore, pasteurized A. muciniphila MucT may be a valuable component of stress therapy in overweight and obese individuals. Confirmation of this thesis requires more robust clinical research.
CONCLUSION
The role of the gut microbiota in the development of stress has been well documented and psychobiotics have gained a well-established place in its treatment. A. muciniphila is a Gram-negative mucus-degrading bacterium whose importance in the onset and symptomology of many diseases is increasingly well understood. Due to its action in supporting the function of the intestinal barrier, damage to which plays an essential role in the pathophysiology of psychiatric disorders, the psychobiotic properties of A. muciniphila provide an opportunity for novel applications and therapies. Particularly as the pasteurized bacterium has escaped the status of novel food and is finding increasing use in nutritional therapies. As described in the highlighted research in this review, the anti-stress properties of A. muciniphila have only been confirmed in experimental animal studies, and could depend on the Amuc_1100 protein, whose beneficial metabolic effects are well established. The beneficial role of A. muciniphila in stress management is promising, but further experimental and clinical studies are required to confirm its anti-stress effects.
DECLARATIONS
Authors’ contributions
Substantial contributions to the conception and design of the study and data analysis and interpretation: Skonieczna-Żydecka K, Misera A, Łoniewski I, Marlicz W
Data acquisition, providing administrative, technical, and material support: Podkówka A, Łoniewski I, Marlicz W
Availability of data and materials
Not applicable.
Financial support and sponsorship
None.
Conflicts of interest
Łoniewski I and Marlicz W are probiotic company shareholders; Skonieczna-Żydecka K and Misera A receive remuneration from probiotic company. These sources had no role in concept design, selection of articles, the decision to publish, or the preparation of the manuscript. While the other authors have declared that they have no conflicts of interest.
Ethical approval and consent to participate
Not applicable.
Consent for publication
Not applicable.
Copyright
© The Author(s) 2024.
REFERENCES
1. Abbasi A, Bazzaz S, Da Cruz AG, et al. A critical review on Akkermansia muciniphila: functional mechanisms, technological challenges, and safety issues. Probiotics Antimicrob Proteins 2024;16:1376-98.
2. Zhao Q, Yu J, Hao Y, et al. Akkermansia muciniphila plays critical roles in host health. Crit Rev Microbiol 2023;49:82-100.
3. Lei W, Cheng Y, Gao J, et al. Akkermansia muciniphila in neuropsychiatric disorders: friend or foe? Front Cell Infect Microbiol 2023;13:1224155.
4. Zhang F, Wang D. Potential of Akkermansia muciniphila and its outer membrane proteins as therapeutic targets for neuropsychological diseases. Front Microbiol 2023;14:1191445.
5. Yan J, Sheng L, Li H. Akkermansia muciniphila: is it the Holy Grail for ameliorating metabolic diseases? Gut Microbes 2021;13:1984104.
6. Wang J, Xu W, Wang R, Cheng R, Tang Z, Zhang M. The outer membrane protein Amuc_1100 of Akkermansia muciniphila promotes intestinal 5-HT biosynthesis and extracellular availability through TLR2 signalling. Food Funct 2021;12:3597-610.
7. Jones LA, Sun EW, Martin AM, Keating DJ. The ever-changing roles of serotonin. Int J Biochem Cell Biol 2020;125:105776.
8. Sun Y, Zhu H, Cheng R, Tang Z, Zhang M. Outer membrane protein Amuc_1100 of Akkermansia muciniphila alleviates antibiotic-induced anxiety and depression-like behavior in mice. Physiol Behav 2023;258:114023.
9. Segers A, de Vos WM. Mode of action of Akkermansia muciniphila in the intestinal dialogue: role of extracellular proteins, metabolites and cell envelope components. Microbiome Res Rep 2023;2:6.
10. Bleibel L, Dziomba S, Waleron KF, Kowalczyk E, Karbownik MS. Deciphering psychobiotics’ mechanism of action: bacterial extracellular vesicles in the spotlight. Front Microbiol 2023;14:1211447.
11. Yaghoubfar R, Behrouzi A, Ashrafian F, et al. Modulation of serotonin signaling/metabolism by Akkermansia muciniphila and its extracellular vesicles through the gut-brain axis in mice. Sci Rep 2020;10:22119.
12. Labellarte MJ, Walkup JT, Riddle MA. The new antidepressants. Selective serotonin reuptake inhibitors. Pediatr Clin North Am 1998;45:1137-55, ix.
13. Song M, Cui M, Fang Z, Liu K. Advanced research on extracellular vesicles based oral drug delivery systems. J Control Release 2022;351:560-72.
14. Hallal S, Tűzesi Á, Grau GE, Buckland ME, Alexander KL. Understanding the extracellular vesicle surface for clinical molecular biology. J Extracell Vesicles 2022;11:e12260.
15. EFSA. Safety of pasteurised Akkermansia muciniphila as a novel food pursuant to regulation (EU) 2015/2283. Available from: https://www.efsa.europa.eu/en/efsajournal/pub/6780. [Last accessed on 4 Sep 2024].
16. Paoletti P, Pellegrino M, Ben-Soussan TD. A three-fold integrated perspective on healthy development: an opinion paper. Brain Sci 2023;13:857.
17. Lazarus R, Folkman S. Stress, appraisal, and coping. Springer Publishing Company; 1984. Available from: https://books.google.com/books/about/Stress_Appraisal_and_Coping.html?id=i-ySQQuUpr8C. [Last accessed on 4 Sep 2024].
18. Scott SB, Graham-Engeland JE, Engeland CG, et al. The Effects of Stress on Cognitive Aging, Physiology and Emotion (ESCAPE) Project. BMC Psychiatry 2015;15:146.
19. Rademacher L, Kraft D, Eckart C, Fiebach CJ. Individual differences in resilience to stress are associated with affective flexibility. Psychol Res 2023;87:1862-79.
20. Palomero-Gallagher N, Amunts K. A short review on emotion processing: a lateralized network of neuronal networks. Brain Struct Funct 2022;227:673-84.
21. Skonieczna-Żydecka K, Łoniewski I, Marlicz W, Karakiewicz B. Gut microbiota and its potential contribution to human emotional disorders. Available online: http://www.medmikro.org/mikrobiota-jelitowa-jako-potencjalna-przyczyna-zaburzen-funkcjonowania-emocjonalnego-czlowieka?lang=pl. [Last accessed on 4 Sep 2024].
22. Landowski J. Neurobiology of stress. Neuropsych Neuro Pol 2007;2:26-36. Available from: https://www.termedia.pl/Artykul-pogladowy-Neurobiologia-reakcji-stresowej,46,8682,0,0.html. [Last accessed on 4 Sep 2024]
23. Chu B, Marwaha K, Sanvictores T, Awosika AO, Ayers D. Physiology, stress reaction. In: StatPearls; StatPearls Publishing: Treasure Island (FL), 2023.
25. Marlicz W, Yung DE, Skonieczna-Żydecka K, et al. From clinical uncertainties to precision medicine: the emerging role of the gut barrier and microbiome in small bowel functional diseases. Expert Rev Gastroenterol Hepatol 2017;11:961-78.
26. Silvernale C, Garcia-Fischer I, Staller K. Relationship between psychological trauma and irritable bowel syndrome and functional dyspepsia in a joint hypermobility syndrome/ehlers-danlos syndrome patient population. Dig Dis Sci 2024;69:870-5.
27. Santucci NR, Velasco-Benitez CA, Cunningham N, et al. Psychological distress and coping efficacy in children with disorders of gut-brain interaction. Neurogastroenterol Motil 2024;36:e14724.
28. Stachowska E, Maciejewska D, Ryterska K, et al. Abdominal pain and disturbed bowel movements are frequent among young people. A population based study in young participants of the Woodstock Rock Festival in Poland. J Gastrointestin Liver Dis 2018;27:379-83.
29. Skonieczna-Żydecka K, Stachowska E, Maciejewska D, et al. The digestive health among participants of the Woodstock Rock Festival in Poland - a cross-sectional survey. Int J Environ Res Public Health 2018;15:2256.
30. Nazarewska A, Lewandowski K, Kaniewska M, Rosołowski M, Marlicz W, Rydzewska G. Irritable bowel syndrome following COVID-19: an underestimated consequence of SARS-CoV-2 infection. Pol Arch Intern Med 2022;132:16323.
31. Nazarewska A, Lewandowski K, Kaniewska M, et al. Long-lasting dyspeptic symptoms - another consequence of the COVID-19 pandemic? Prz Gastroenterol 2023;18:175-82.
32. Person H, Keefer L. Psychological comorbidity in gastrointestinal diseases: update on the brain-gut-microbiome axis. Prog Neuropsychopharmacol Biol Psychiatry 2021;107:110209.
33. Davis MT, Holmes SE, Pietrzak RH, Esterlis I. Neurobiology of chronic stress-related psychiatric disorders: evidence from molecular imaging studies. Chronic Stress 2017;1:2470547017710916.
34. Zuckerman M. Diathesis-stress models. In: Vulnerability to psychopathology: a biosocial model. Washington, DC: American Psychological Association; 1999. pp. 3-23.
35. Misera A, Łoniewski I, Palma J, et al. Clinical significance of microbiota changes under the influence of psychotropic drugs. An updated narrative review. Front Microbiol 2023;14:1125022.
36. Ingram RE, Luxton DD. Vulnerability-stress models. In: Hankin BL, Abela JRZ, editors. Development of psychopathology: a vulnerability-stress perspective. SAGE Publications, Inc.; 2005. pp. 32-46.
37. Zubin J, Spring B. Vulnerability - a new view of schizophrenia. J Abnorm Psychol 1977;86:103-26.
38. de Vos WM, Tilg H, Van Hul M, Cani PD. Gut microbiome and health: mechanistic insights. Gut 2022;71:1020-32.
39. Shanahan F, Ghosh TS, O’Toole PW. The healthy microbiome - What is the definition of a healthy gut microbiome? Gastroenterology 2021;160:483-94.
41. Kundu P, Blacher E, Elinav E, Pettersson S. Our gut microbiome: the evolving inner self. Cell 2017;171:1481-93.
42. Skonieczna-Żydecka K, Marlicz W, Misera A, Koulaouzidis A, Łoniewski I. Microbiome - the missing link in the gut-brain axis: focus on its role in gastrointestinal and mental health. J Clin Med 2018;7:521.
43. Liang S, Jin F, Jia C. Editorial: the effect of gut microbiota on the brain structure and function. Front Integr Neurosci 2023;17:1226664.
44. Kim YK, Shin C. The microbiota-gut-brain axis in neuropsychiatric disorders: pathophysiological mechanisms and novel treatments. Curr Neuropharmacol 2018;16:559-73.
45. Marin IA, Goertz JE, Ren T, et al. Microbiota alteration is associated with the development of stress-induced despair behavior. Sci Rep 2017;7:43859.
46. Watanabe Y, Arase S, Nagaoka N, Kawai M, Matsumoto S. Chronic psychological stress disrupted the composition of the murine colonic microbiota and accelerated a murine model of inflammatory bowel disease. PLoS One 2016;11:e0150559.
47. Knowles SR, Nelson EA, Palombo EA. Investigating the role of perceived stress on bacterial flora activity and salivary cortisol secretion: a possible mechanism underlying susceptibility to illness. Biol Psychol 2008;77:132-7.
48. Kato-Kataoka A, Nishida K, Takada M, et al. Fermented milk containing Lactobacillus casei strain shirota preserves the diversity of the gut microbiota and relieves abdominal dysfunction in healthy medical students exposed to academic stress. Appl Environ Microbiol 2016;82:3649-58.
49. Gao F, Guo R, Ma Q, et al. Stressful events induce long-term gut microbiota dysbiosis and associated post-traumatic stress symptoms in healthcare workers fighting against COVID-19. J Affect Disord 2022;303:187-95.
50. Molina-Torres G, Rodriguez-Arrastia M, Roman P, Sanchez-Labraca N, Cardona D. Stress and the gut microbiota-brain axis. Behav Pharmacol 2019;30:187-200.
51. Naseribafrouei A, Hestad K, Avershina E, et al. Correlation between the human fecal microbiota and depression. Neurogastroenterol Motil 2014;26:1155-62.
52. Nguyen TT, Kosciolek T, Eyler LT, Knight R, Jeste DV. Overview and systematic review of studies of microbiome in schizophrenia and bipolar disorder. J Psychiatr Res 2018;99:50-61.
53. Nguyen TT, Kosciolek T, Maldonado Y, et al. Differences in gut microbiome composition between persons with chronic schizophrenia and healthy comparison subjects. Schizophr Res 2019;204:23-9.
54. Stasi C, Nisita C, Cortopassi S, et al. Subthreshold psychiatric psychopathology in functional gastrointestinal disorders: can it be the bridge between gastroenterology and psychiatry? Gastroenterol Res Pract 2017;2017:1953435.
55. Skonieczna-Żydecka K, Łoniewski I, Misera A, et al. Second-generation antipsychotics and metabolism alterations: a systematic review of the role of the gut microbiome. Psychopharmacology 2019;236:1491-512.
56. Söderholm JD, Yates DA, Gareau MG, Yang PC, MacQueen G, Perdue MH. Neonatal maternal separation predisposes adult rats to colonic barrier dysfunction in response to mild stress. Am J Physiol Gastrointest Liver Physiol 2002;283:G1257-63.
57. Lennon EM, Maharshak N, Elloumi H, Borst L, Plevy SE, Moeser AJ. Early life stress triggers persistent colonic barrier dysfunction and exacerbates colitis in adult IL-10-/- mice. Inflamm Bowel Dis 2013;19:712-9.
58. Smith F, Clark JE, Overman BL, et al. Early weaning stress impairs development of mucosal barrier function in the porcine intestine. Am J Physiol Gastrointest Liver Physiol 2010;298:G352-63.
59. Spadoni I, Zagato E, Bertocchi A, et al. A gut-vascular barrier controls the systemic dissemination of bacteria. Science 2015;350:830-4.
60. Shiels PG, Buchanan S, Selman C, Stenvinkel P. Allostatic load and ageing: linking the microbiome and nutrition with age-related health. Biochem Soc Trans 2019;47:1165-72.
61. Westfall S, Iqbal U, Sebastian M, Pasinetti GM. Gut microbiota mediated allostasis prevents stress-induced neuroinflammatory risk factors of Alzheimer’s disease. Prog Mol Biol Transl Sci 2019;168:147-81.
62. Liu Y, Wang J, Wu C. Modulation of gut microbiota and immune system by probiotics, pre-biotics, and post-biotics. Front Nutr 2021;8:634897.
63. Li N, Wang Q, Wang Y, et al. Oral probiotics ameliorate the behavioral deficits induced by chronic mild stress in mice via the gut microbiota-inflammation axis. Front Behav Neurosci 2018;12:266.
64. Ge X, Ding C, Zhao W, et al. Antibiotics-induced depletion of mice microbiota induces changes in host serotonin biosynthesis and intestinal motility. J Transl Med 2017;15:13.
65. Kennedy EA, King KY, Baldridge MT. Mouse microbiota models: comparing germ-free mice and antibiotics treatment as tools for modifying gut bacteria. Front Physiol 2018;9:1534.
66. Zareie M, Johnson-Henry K, Jury J, et al. Probiotics prevent bacterial translocation and improve intestinal barrier function in rats following chronic psychological stress. Gut 2006;55:1553-60.
67. Emge JR, Huynh K, Miller EN, et al. Modulation of the microbiota-gut-brain axis by probiotics in a murine model of inflammatory bowel disease. Am J Physiol Gastrointest Liver Physiol 2016;310:G989-98.
68. Messaoudi M, Lalonde R, Violle N, et al. Assessment of psychotropic-like properties of a probiotic formulation (Lactobacillus helveticus R0052 and Bifidobacterium longum R0175) in rats and human subjects. Br J Nutr 2011;105:755-64.
69. Messaoudi M, Violle N, Bisson JF, Desor D, Javelot H, Rougeot C. Beneficial psychological effects of a probiotic formulation
70. Government of Canada. Product Information. Available from: https://health-products.canada.ca/lnhpd-bdpsnh/info?licence=80021343. [Last accessed on 4 Sep 2024].
71. Arksey H, O’Malley L. Scoping studies: towards a methodological framework. Int J Soc Res Methodol 2005;8:19-32.
72. Pham MT, Rajić A, Greig JD, Sargeant JM, Papadopoulos A, McEwen SA. A scoping review of scoping reviews: advancing the approach and enhancing the consistency. Res Synth Methods 2014;5:371-85.
73. Mazzantini D, Calvigioni M, Celandroni F, Lupetti A, Ghelardi E. Spotlight on the compositional quality of probiotic formulations marketed worldwide. Front Microbiol 2021;12:693973.
74. Kolaček S, Hojsak I, Berni Canani R, et al; ESPGHAN Working Group for Probiotics and Prebiotics. Commercial probiotic products: a call for improved quality control. A position paper by the ESPGHAN Working Group for probiotics and prebiotics. J Pediatr Gastroenterol Nutr 2017;65:117-24.
76. Belzer C, de Vos WM. Microbes inside - from diversity to function: the case of Akkermansia. ISME J 2012;6:1449-58.
77. Cani PD, Depommier C, Derrien M, Everard A, de Vos WM. Akkermansia muciniphila: paradigm for next-generation beneficial microorganisms. Nat Rev Gastroenterol Hepatol 2022;19:625-37.
78. Cani PD, de Vos WM. Next-generation beneficial microbes: the case of Akkermansia muciniphila. Front Microbiol 2017;8:1765.
79. Wang L, Tang L, Feng Y, et al. A purified membrane protein from Akkermansia muciniphila or the pasteurised bacterium blunts colitis associated tumourigenesis by modulation of CD8+ T cells in mice. Gut 2020;69:1988-97.
80. Plovier H, Everard A, Druart C, et al. A purified membrane protein from Akkermansia muciniphila or the pasteurized bacterium improves metabolism in obese and diabetic mice. Nat Med 2017;23:107-13.
81. Ashrafian F, Keshavarz Azizi Raftar S, Shahryari A, et al. Comparative effects of alive and pasteurized Akkermansia muciniphila on normal diet-fed mice. Sci Rep 2021;11:17898.
82. Wang J, Xiang R, Wang R, et al. The variable oligomeric state of Amuc_1100 from Akkermansia muciniphila. J Struct Biol 2020;212:107593.
83. Shi M, Yue Y, Ma C, Dong L, Chen F. Pasteurized Akkermansia muciniphila ameliorate the LPS-induced intestinal barrier dysfunction via modulating AMPK and NF-κB through TLR2 in Caco-2 cells. Nutrients 2022;14:764.
84. Gu Z, Pei W, Shen Y, et al. Akkermansia muciniphila and its outer protein Amuc_1100 regulates tryptophan metabolism in colitis. Food Funct 2021;12:10184-95.
85. Myint AM, Halaris A. Imbalances in kynurenines as potential biomarkers in the diagnosis and treatment of psychiatric disorders. Front Psychiatry 2022;13:913303.
86. Ottman N, Reunanen J, Meijerink M, et al. Pili-like proteins of Akkermansia muciniphila modulate host immune responses and gut barrier function. PLoS One 2017;12:e0173004.
87. Muccioli GG, Naslain D, Bäckhed F, et al. The endocannabinoid system links gut microbiota to adipogenesis. Mol Syst Biol 2010;6:392.
88. Carabotti M, Scirocco A, Maselli MA, Severi C. The gut-brain axis: interactions between enteric microbiota, central and enteric nervous systems. Ann Gastroenterol 2015;28:203-9.
89. Nie X, Kitaoka S, Tanaka K, et al. The innate immune receptors TLR2/4 mediate repeated social defeat stress-induced social avoidance through prefrontal microglial activation. Neuron 2018;99:464-79.e7.
90. Quave CB, Nieto SJ, Haile CN, Kosten TA. Immune receptor toll-like receptor 4 contributes to stress-induced affective responses in a sex-specific manner. Brain Behav Immun Health 2021;14:100248.
91. Figueroa-Hall LK, Paulus MP, Savitz J. Toll-like receptor signaling in depression. Psychoneuroendocrinology 2020;121:104843.
92. Hung YY, Huang KW, Kang HY, Huang GY, Huang TL. Antidepressants normalize elevated Toll-like receptor profile in major depressive disorder. Psychopharmacology 2016;233:1707-14.
93. Hung YY, Kang HY, Huang KW, Huang TL. Association between toll-like receptors expression and major depressive disorder. Psychiatry Res 2014;220:283-6.
94. Chen T, Wang R, Duan Z, et al. Akkermansia muciniphila protects against psychological disorder-induced gut microbiota-mediated colonic mucosal barrier damage and aggravation of colitis. Front Cell Infect Microbiol 2021;11:723856.
95. Ding Y, Bu F, Chen T, et al. A next-generation probiotic: Akkermansia muciniphila ameliorates chronic stress-induced depressive-like behavior in mice by regulating gut microbiota and metabolites. Appl Microbiol Biotechnol 2021;105:8411-26.
96. Cheng R, Xu W, Wang J, Tang Z, Zhang M. The outer membrane protein Amuc_1100 of Akkermansia muciniphila alleviates the depression-like behavior of depressed mice induced by chronic stress. Biochem Biophys Res Commun 2021;566:170-6.
97. Guo H, Liu X, Chen T, Wang X, Zhang X. Akkermansia muciniphila improves depressive-like symptoms by modulating the level of 5-HT neurotransmitters in the gut and brain of mice. Mol Neurobiol 2024;61:821-34.
98. Cheng R, Zhu H, Sun Y, Hang T, Zhang M. The modified outer membrane protein Amuc_1100 of Akkermansia muciniphila improves chronic stress-induced anxiety and depression-like behavior in mice. Food Funct 2022;13:10748-58.
99. Pomeranian Medical University Szczecin The effects of the anti-inflammatory microbe - Pasteurized Akkermansia Muciniphila (PAM) on symptoms of somatic and mental stress in healthcare professionals. 2023. Available from: https://clinicaltrial.be/en/details/259148?per_page=20&only_recruiting=0&only_eligible=0. [Last accessed on 4 Sep 2024].
100. Marlicz W, Koulaouzidis A, Charisopoulou D, et al. Burnout in healthcare - the Emperor’s new clothes. Prz Gastroenterol 2023;18:274-80.
101. Fasanella NA, Custódio CG, Cabo JSD, Andrade GS, Almeida FA, Pavan MV. Use of prescribed psychotropic drugs among medical students and associated factors: a cross-sectional study. Sao Paulo Med J 2022;140:697-704.
102. Flowers SA, Evans SJ, Ward KM, McInnis MG, Ellingrod VL. Interaction between atypical antipsychotics and the gut microbiome in a bipolar disease cohort. Pharmacotherapy 2017;37:261-7.
103. Depommier C, Everard A, Druart C, et al. Supplementation with Akkermansia muciniphila in overweight and obese human volunteers: a proof-of-concept exploratory study. Nat Med 2019;25:1096-103.
Cite This Article
How to Cite
Misera, A.; Marlicz W.; Podkówka A.; Łoniewski I.; Skonieczna-Żydecka K. Possible application of Akkermansia muciniphila in stress management. Microbiome. Res. Rep. 2024, 3, 48. http://dx.doi.org/10.20517/mrr.2023.81
Download Citation
Export Citation File:
Type of Import
Tips on Downloading Citation
Citation Manager File Format
Type of Import
Direct Import: When the Direct Import option is selected (the default state), a dialogue box will give you the option to Save or Open the downloaded citation data. Choosing Open will either launch your citation manager or give you a choice of applications with which to use the metadata. The Save option saves the file locally for later use.
Indirect Import: When the Indirect Import option is selected, the metadata is displayed and may be copied and pasted as needed.
About This Article
Special Issue
Copyright
Data & Comments
Data
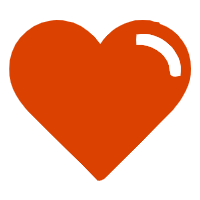
Comments
Comments must be written in English. Spam, offensive content, impersonation, and private information will not be permitted. If any comment is reported and identified as inappropriate content by OAE staff, the comment will be removed without notice. If you have any queries or need any help, please contact us at support@oaepublish.com.