Cyclodextrin-assisted supramolecular host-guest inclusion for durable and sustainable optoelectronics
Abstract
Metal halide perovskites and organic nonlinear optical materials have showcased enormous potential in many kinds of optoelectronic applications, such as solar cells, light-emitting diodes, and patterned displays. However, further enhancement of optoelectronic performances has been largely limited by the intractable issues of these materials including high defect densities, unstable crystallographic structure, harsh fabrication conditions, and unfavorable biocompatibility and environmental sustainability. Encouragingly, several recent works have demonstrated an effective supramolecular host-guest inclusion strategy could ideally address abovementioned concerns by nesting optoelectronic materials within the cavities of cyclodextrin molecules and their analogs. Specifically, the supramolecule hosts embedded with multiple functional groups and/or crosslinked networks could robustly interact with those optoelectronic materials, which play multifaceted roles in terms of chemical chelation, spatial confinement, structural stabilization, defect passivation, ion immobilization and compensation, thus resulting in comprehensive enhancement of optoelectronic performances and sustainability. The current challenging issues and potential solutions are also discussed to provide a roadmap for achieving more durable and sustainable optoelectronics toward practical applications and real commercialization.
Keywords
INTRODUCTION
Over the past decades, the metal halide perovskites (MHPs) have witnessed unprecedentedly fast development and have been regarded as the new generation photovoltaic and luminescent materials due to their fascinating optoelectronic properties such as tunable bandgaps, decent light absorption coefficient, long carrier diffusion length, high color purity and high photoluminescence quantum yield (PLQY). Despite fruitful breakthroughs in MHPs-based optoelectronics, the inherent crystallographic imperfections of MHPs and their structural instability under light/heat/solvent stimuli have been huge obstacles to limiting the further device performance enhancement and practical application[1]. The same situation is encountered for organic nonlinear optical (NLO) materials, which, beyond that, also suffer from harsh fabrication conditions.
It is well known that the solution-processed MHPs intrinsically contain multiple types of ionic defects, which inevitably alter the optoelectronic properties and performances of MHPs materials and devices. Because of low migration activation energy, several kinds of ions can easily migrate through the vacancy defects within the perovskite lattice, which would cause the decomposition of MHPs and result in the degradation of MHPs-based optoelectronics[2]. Various small organic molecules with moderate molecular size and several functional groups have been designed for passivating both the bulk and surface defects of MHPs. For example, uracil was introduced to manipulate the film growth by Wang et al.[3]. Though effective and promising, one can still expect the potential shortcomings such as limited chemical interaction sites, insufficient surface protection[4] and extra processing steps[5], not to mention their inferior capability of reducing lead leakage. Specifically, the limited chemical interaction sites would result in limited passivation efficacy of crystallographic imperfections and limited ion immobilization effect. Moreover, most of the reported organic molecules may have planar configurations and/or relatively short molecular chain length, which has negligible spatial confinement effect on MHPs. Superior to the conventionally employed molecules, several recent works have demonstrated the unique advantages of cyclodextrins (CDs) and their analogs, which possessed desirable inner/outer cavities embedded with ample functional groups that could form a supramolecular host-guest inclusion complex with MHPs and NLO materials. Encouragingly, such a supramolecular host-guest inclusion strategy can passivate the crystallographic imperfections, spatially confine the guest materials, inhibit the ion migration and capture the leaked toxic metal ions in a more effective manner, thus making MHPs and NLO materials-based optoelectronics more efficient, durable and sustainable. Interestingly, such a strategy has resulted in the fabrication of waterproof perovskite luminescent textiles (PLTs) that are durable in water for more than one year, light/thermal-stable Pb-based perovskite solar cells (PSCs) that are biocompatible and sustainable and flexible NLO membranes for realizing in-vivo noninvasive two-photon bioimaging.
This perspective summarizes the recent advancement of employing supramolecular host-guest inclusion strategy to improve the performance, durability and sustainability of MHPs or NLO materials-based optoelectronic devices, with particular emphasis on highlighting the multifaceted roles of host-guest inclusion strategy in defect passivation, spatial confinement, ion immobilization and compensation, and lead capture. An insightful perspective toward future optimization routes and research directions will be provided, aiming to popularize the host-guest inclusion strategy and facilitate the development of more efficient, durable and sustainable optoelectronics.
MULTIPLE CHEMICAL INTERACTIONS AND DEFECT PASSIVATION
MHPs, with a general chemical formula of ABX3, are composed of monovalent A cations and corner-sharing metal halide [BX6]4- octahedras, in which B and X are bivalent metals and monovalent halides, respectively. Due to the fragile chemical bonds between the cations and anions, ionic defects are easy to form and migrate within MHPs when subjected to external stress or stimuli, thus accelerating the perovskite degradation. In addition, severe ion migration can eventually lead to local stoichiometric variations of MHPs, which would change their defect chemistry and promote unwanted variations of optoelectronic properties. CDs are cyclic oligosaccharides linked by α- 1,4-glycosidic bonds[6]. Three major CD variants, namely, α-, β-, and γ-CDs, comprise six, seven, and eight D-glucose units, respectively. More interestingly, the CDs feature a distinct truncated cone structure with a hydrophilic exterior and hydrophobic interior. Multidentate hydroxyl groups embedded along the wall of internal and external cavities enable the formation of robust inclusion complexes with various guest molecules, such as perovskites, and simultaneously passivate the defects and enhance the optoelectronic performance of perovskites.
Yang et al. have innovatively introduced a self-crosslinking supramolecular complex consisting of
Figure 1. (A) Schematic diagram of multiple chemical interactions between crosslinked HPβCD-BTCA network and perovskites. (B) Schematic illustration of the HPβCD-BTCA-assisted stabilization of the whole fabrication process from precursor to film and to the final device. Space charge-limited current curves of the (C) control and (D) target HPβCD-BTCA-modified device. Reprinted with permission[7]. Copyright © 2023 Springer Nature Limited. (E) Different distributions of hydroxyl groups in OMβCD and G4, and (F) their interactions with different ions in perovskites. Reprinted with permission[8]. Copyright © 2023 Wiley-VCH GmbH.
SPATIAL CONFINEMENT
When the size of perovskite materials is reduced to the nanometer level comparable to their exciton Bohr radius, the quantum confinement effect is shown and the energy level is quantized, which would change the band gap of perovskites, thus producing some unique photoelectric properties. The abovementioned supramolecular host-guest inclusion strategy could also be employed to confine the sizes of perovskite nanocrystals, aiming to enhance the optical properties and durability of perovskite luminescent materials. Tian et al. have demonstrated that inclusion of CsPbBr3 by HPβCD and super-hydrophobic perfluorooctyltriethoxysilane (PFOS) could significantly improve the color purity of fibers with narrow-band photoluminescence (full-width at half-maximum < 17 nm), enhance the radiative recombination rate by more than 4-fold, and improve the PLQY by 25-fold to approximately 50%, relative to the control counterpart, which can be attributed to the quantum confinement effect, manifold defect passivation and reduced overlap of the absorption band edge and the PL emission peak [Figure 2A-C][9]. Specifically, unlike the irregular-shaped perovskite crystals disorderedly distributed and aggregated on the surface of the control perovskite-polymer composited fibers, the aggregation of CsPbBr3 nanocrystals could be efficiently suppressed in the CsPbBr3@HPβCD composites, in which the CsPbBr3 nanocrystals were uniformly encapsulated and spatially separated by HPβCD clusters, thus confining the evolved CsPbBr3 nanocrystals to much smaller sizes (i.e., 14.24 ± 6.02 nm) inside and/or at the surface of the polymer matrix
Figure 2. (A) Schematic illustration of CsPbBr3@HPβCD@PFOS composites with host-guest interaction and hydrogen bond formation. (B) UV-visible absorption and PL spectra of CsPbBr3, CsPbBr3@HPβCD and CsPbBr3@HPβCD@PFOS fibers. (C) Fluorescence image of large-area CsPbBr3@HPβCD@PFOS fibrous membrane excited by UV light. TEM images of the (D) control CsPbBr3 fibers and (E) CsPbBr3@HPβCD fibers. Reprinted with permission[9]. Copyright © 2023 Springer Nature Limited.
The spatial confinement effect induced by supramolecular host-guest inclusion could also be applied to organic NLO materials. Very recently, Tian et al. demonstrated the production of the large-area organic NLO membranes consisting of 4-N,N-dimethylamino-4′-N′-methylstilbazolium tosylate (DAST)@HPβCD host-guest supramolecular complexes with outstanding multiphoton absorption characteristics[10]. DAST is a kind of stilbazolium derivative, featuring a structure of donor-π-acceptor (D-π-A)-type. Without spatial confinement and geometric restriction, the DAST molecules are easily burdened with serious aggregation-caused quenching (ACQ), trans-cis isomerization and/or intramolecular charge transfer (ICT) process-induced nonradiative decay, which, more often than not, showcased inferior luminescent performance and even lost their NLO activity when DAST materials are exposed in ambient air and/or polar solvent environment[11]. With crosslinked HPβCD clusters incorporation, DAST molecules are inserted into the cavity of HPβCD hosts to form the DAST@HPβCD supramolecular host-guest inclusion complexes via robust interactions between these molecules, including hydrogen bonding interactions and electrostatic interaction [Figure 3A and B]. In this case, the HPβCD framework could spatially split the DAST molecules and successfully restrict the isomerization of DAST molecules, thus minimizing the ACQ effect, inhibiting the excited-state rotation from locally-excited (LE) state to twisted ICT (TICT) state [Figure 3C], and essentially restrain the TICT state-caused nonradiative decay [Figure 3D and E]. All these advantages contribute together to amplifying the PLQY of DAST@HPβCD fibers by ~78 fold, attaining a high value of 70.4%, which significantly outperformed that of the control counterpart (an extremely low PLQY of 0.9%). Further crosslink of DAST@HPβCD supramolecular framework boosted the PLQY to 73.5% [Figure 3F], which represented one of the highest reported values for the DAST materials and their derivatives. Beyond one-photon-excited fluorescence (1PEF), the DAST@HPβCD membranes showed outstanding NLO properties, which improved second-harmonic generation (SHG) signal, and could exhibit broadband two-photon-excited fluorescence (2PEF) (ca. 520-650 nm) when excited at a wavelength region from 770 nm to 1,000 nm and showcase three-photon-excited fluorescence (3PEF) when excited at 1,590 nm [Figure 3G]. The chemically and thermally stable crosslinked HPβCD matrix could achieve robust supramolecular encapsulation of DAST guests, in which the structural deformation of DAST molecules was successfully inhibited, even under high humidity or high temperature conditions, thus endowing the luminescent DAST@HPβCD membranes with outstanding ambient and thermal stability. Surprisingly, the
Figure 3. (A) Schematic showing the insertion of DAST guest molecules into the cavities of HPβCD hosts. (B) The correlation spectroscopy (COSY) NMR of DAST@HPβCD supramolecular host-guest inclusion complex. (C) Potential energy diagram and corresponding excited-state conversion of DAST@HPβCD fibers. The TA contour plots of (D) DAST and (E) DAST@HPβCD samples. (F) Fluorescence image of
ION IMMOBILIZATION AND COMPENSATION
Benefiting from the abovementioned multiple chemical interactions between host and guest molecules, the structural stability of MHPs is expected to be enhanced owing to the inhibited ion migration and retarded lattice deformation. Yang et al. have demonstrated the crosslinked HPβCD matrix with multiple positively charged hydrogen ions could interact intensely with negatively charged halide ions in perovskites via massive hydrogen bonding[7]. Modified by 3D supramolecular framework, the target PSCs exhibited enhanced stability under the stress of light and heat, which preserved nearly 99% of their initial PCE after 700 h of continuous 1 sun illumination and retained 80% of the initial efficiency after 300 h upon continuous heating at 85 °C, while the control device showed poor stability. The remarkably extended stability of PSCs could be attributed to the ion immobilization effect induced by anchoring of HPβCD-BTCA crosslinking supramolecular framework, especially at the grain boundaries and on the film surface of perovskite film[7]. It is anticipated that, relative to pristine HPβCD modification, the incorporation of cross-linking HPβCD-BTCA supramolecular network could further reinforce the ion immobilization effect towards the perovskites owing to more luxuriant interactions to stabilize the ions and more robust physical blocking effect to inhibit the ions migration. Learning from the supramolecular design of Yang et al.[7], Li et al. employed the β-cyclodextrin (βCD) as a typical I2 trapper, in which the I2 can be trapped into the hydrophobic cavity of bowl-like βCD, which beneficially increased the volatilization temperature of I2 and inhibited its escape from perovskites[12]. During device operation, the trapped I2 could react with Pb0 to eliminate related defects, and favorably interact with FAI to form photoactive perovskites [Figure 4A]. It is worth noting that under International Submit on Organic Photovoltaic Stability (ISOS) protocol, the βCD-modified PSCs exhibited both high efficiency (> 23%) and good thermal stability, retaining over 80% of the highest efficiency after tracking at maximum power output point (MPP) for 300 h at 85 °C, while the control PSCs only retained about 56% of highest efficiency after 150 h [Figure 4B][12].
Figure 4. (A) Schematic diagram of iodine immobilization and regeneration of photoactive perovskites via βCD incorporation and modification. (B) Stability test of control and βCD-modified PSCs under ISOS protocol. Reprinted with permission[12]. Copyright © 2023 Royal Society of Chemistry. (C) Molecular structure of Starch and Starch-I, along with proposed functions of the Starch- I buffer layer regarding halides diffusion inhibition and halide vacancies healing upon applied bias. (D) PCE tracking of the reference (Ref) and Starch-I devices upon day and night cycle operation. Reprinted with permission[13]. Copyright © 2023 Springer Nature Limited.
In addition to halide ion immobilization, supramolecular host-guest strategy can be employed to realize halide ion compensation, which is beneficial to reduce the halide deficiency, and mitigate the ion migration and redistribution. Very recently, Zhang et al. innovatively introduced a starch-polyiodide supermolecule (Starch-I) as a bifunctional buffer layer at the buried interface of perovskite film, which simultaneously suppressed ion migration and promoted defect self-healing via modulating the halide migration kinetics by reserving sufficient iodide species[13]. Starch is a polymeric carbohydrate with a molecular formula of
LEAD CAPTURE
Though promising, practical application of Pb-containing MHPs and long-term deployment of MHPs-based devices is confronted with the Pb toxicity matter, not to mention the case of unwanted Pb leakage from broken devices, which has become a serious problem with damaging impact on the environment and human health. Up till now, it is demonstrated that different approaches have been developed to reduce the Pb leakage through additional physical encapsulation or chemical absorption, but at the cost of introducing extra manufacturing processes. Yang et al. subtly minimized Pb leakage and lowered Pb toxicity via incorporating crosslinking HPβCD-BTCA supramolecular complex that enabled robust chemical coordination and multidentate chelation with Pb2+ ions [Figure 5A and B][7]. When the PSC devices were severely damaged, it was worth noting that Pb leakage rates significantly decreased from a high speed of
Figure 5. (A) Schematic showing the negligible Pb leakage and biocompatibility to living organisms of broken PSCs with supramolecular HPβCD-BTCA complex modification. and (B) schematic diagram of the lead capture mechanism of supramolecular HPβCD-BTCA complex. (C) Comparison of Pb sequestration for the damaged control and HPβCD-BTCA-modified PSCs. (D) Schematic illustration of Pb toxicity test procedures and (E) the corresponding results for the impacts of pristine perovskites and HPβCD-BTCA-chelated perovskites on the growth of E. coli. (F) Schematic illustration of Pb recycling and management in PSCs. Readapted with permission[7]. Copyright © 2023 Springer Nature Limited.
The conventional Pb-based photovoltaics poses challenges due to the presence of toxic, pollutional, and bio-accumulative Pb, which could hinder the widespread adoption of this fascinating photovoltaic technology. Obviously, inhibiting the Pb leakage is still not safe enough to ensure the sustainable deployment of perovskite photovoltaics. Note that the biocompatible CD supramolecules have been demonstrated to be capable of constructing bio-safe host-guest inclusion complex-based fluorescence probes for realizing in-vivo, non-invasive bioimaging[10]. Yang et al., for the first time, evaluated the biocompatibility of the different perovskite compositions by evaluating their impacts on the growth of E. coli[7] [Figure 5D]. The results indicated no difference in the E. coli growth between the target sample (with HPβCD-BTCA-modified perovskite) and the blank sample (no Pb contamination). In contrast, the control sample (with pristine perovskite contamination) revealed a remarkable inhibitory effect [Figure 5E], suggesting the reduced Pb toxicity for the former. It is worth noting that the biocompatibility of Pb-based perovskites with HPβCD-BTCA chelation is comparable to that of Pb-free perovskites, such as pure Sn-based compositions. In addition to preventing lead leakage and reducing lead toxicity, lead recycling and management is crucial for making perovskite photovoltaics safe and environmentally sustainable, especially for failing devices.
SUMMARY AND OUTLOOK
In summary, the aforementioned breakthrough studies have highlighted the unique advantages and beneficial roles of host-guest inclusion between supramolecules host and optoelectronic materials guests (i.e., perovskites and organic NLO materials). The outstanding merits are multifaceted, including effective defect passivation, uniform nanoconfinement and robust ion immobilization, which synergically boosted the optoelectronic performance of photovoltaics and luminescent devices. Encouragingly, the supramolecular host-guest inclusion strategy has enabled the realization of high-performance PSCs with champion efficiency of up to 24.22% with an extended lifespan of over 1,000 h. The perovskite-based luminescent films showed a champion PLQY of 49.7%, corresponding to an amplification of 25-fold. The NLO-based luminescent films showed a champion PLQY of 73.5%, corresponding to an amplification of
Summary of the key performances of cyclodextrin-modified optoelectronics
While fruitful results and advanced breakthroughs have been achieved, the relevant community was encouraged to seek future development. We thus provide an insightful perspective toward future research directions, aiming to take full advantage of MHPs and organic NLO materials and further extend their applications in various optoelectronics. First of all, the CDs and their derivatives have luxuriant structural constituents. As for the principles of selecting different CDs, one should consider their inner cavity size, types and numbers of functional groups, and their chemical interaction affinity with guest molecules, which is beneficial to achieve robust host-guest inclusion effect and improve the optoelectronic properties of resultant complexes. More advanced design of supramolecular host is desirable, for instance, exploring other functionalized CDs with different function groups or moieties that could play multifunctional roles for optimizing optoelectronic properties of guest materials. Secondly, the host-guest relationship and mutual interaction mechanisms between the supramolecular host and optoelectronic guest molecules should be further studied, aiming to excavate the fundamental understanding of complicated host-guest inclusion chemistry and guide the further advancement of the optoelectronic performance. Thirdly, one of the most challenging obstacles that the supramolecular inclusion strategy encounters in the process of commercializing perovskite-based optoelectronic devices is its compatibility to realize low-cost, high throughput fabrication of efficient, stable and reproducible optoelectronic devices. To tackle it, one should adapt the well-designed host-guest inclusion ingredients/compositions to those advanced fabrication techniques that are compatible with large-scale mass production (e.g., electrospinning, blade-coating, inkjet printing, roll-to-roll manufacturing, and slot-die coating). Note that the functional groups embedded in the CD could be positively engaged in the crystallization process of perovskites, which beneficially helped to improve the uniformity of large-area perovskite films. Last but not least, not just limited to PSCs and luminescent applications, such a promising supramolecular host-guest inclusion strategy should be extended to other optoelectronic applications, such as photodetectors, lasers, photocatalysis, X-ray imaging, etc.
DECLARATIONS
Authors’ contributions
Literature review and manuscript draft: Chen H
Figure layout: Chen H, Chang X
Picture production: Chen H, Yang G
Manuscript revision: Lei BX, Wu WQ
Project supervision: Wu WQ
Availability of data and materials
Not applicable.
Financial support and sponsorship
Financial support from the National Natural Science Foundation of China (22005355), Guangxi Key Laboratory of Advanced Structural Materials and Carbon Neutralization (GXAMCN23-2), and the NSF of Guangdong Province (2023B1515120008).
Conflicts of interest
All authors declared that there are no conflicts of interest.
Ethical approval and consent to participate
Not applicable.
Consent for publication
Not applicable.
Copyright
© The Author(s) 2024.
REFERENCES
1. Hou J, Chen P, Shukla A, et al. Liquid-phase sintering of lead halide perovskites and metal-organic framework glasses. Science 2021;374:621-5.
2. Bi E, Song Z, Li C, Wu Z, Yan Y. Mitigating ion migration in perovskite solar cells. Trends Chem 2021;3:575-88.
3. Wang M, Sun H, Wang M, Meng L, Li L. Uracil induced simultaneously strengthening grain boundaries and interfaces enables high-performance perovskite solar cells with superior operational stability. Adv Mater 2024;36:e2306415.
4. Feng W, Tan Y, Yang M, et al. Small amines bring big benefits to perovskite-based solar cells and light-emitting diodes. Chem 2022;8:351-83.
5. Li M, Zhu Z, Wang Z, et al. High-quality hybrid perovskite thin films by post-treatment technologies in photovoltaic applications. Adv Mater 2024;36:e2309428.
6. Hashidzume A, Takashima Y, Yamaguchi H, Harada A. 1.12 - Cyclodextrin. In: Comprehensive supramolecular chemistry II, Atwood JL, editor. Oxford: Elsevier; 2017; pp. 269-316.
7. Yang M, Tian T, Fang Y, et al. Reducing lead toxicity of perovskite solar cells with a built-in supramolecular complex. Nat Sustain 2023;6:1455-64.
8. Liu X, Jiang X, Zhang J, Li C, Guo X. Multiple-ion management of perovskites by regulating spatial distribution of hydroxyls in oligosaccharides. Small 2023;19:e2301437.
9. Tian T, Yang M, Fang Y, et al. Large-area waterproof and durable perovskite luminescent textiles. Nat Commun 2023;14:234.
10. Tian T, Fang Y, Wang W, et al. Durable organic nonlinear optical membranes for thermotolerant lightings and in vivo bioimaging. Nat Commun 2023;14:4429.
11. Tian T, Cai B, Sugihara O. DAST single-nanometer crystal preparation using a substrate-supported rapid evaporation crystallization method. Nanoscale 2016;8:18882-6.
12. Li X, Yang H, Liu A, et al. Iodine-trapping strategy for light-heat stable inverted perovskite solar cells under ISOS protocols. Energy Environ Sci 2023;16:6071-7.
Cite This Article
Export citation file: BibTeX | EndNote | RIS
OAE Style
Chen H, Chang X, Yang G, Lei BX, Wu WQ. Cyclodextrin-assisted supramolecular host-guest inclusion for durable and sustainable optoelectronics. Microstructures 2024;4:2024031. http://dx.doi.org/10.20517/microstructures.2023.83
AMA Style
Chen H, Chang X, Yang G, Lei BX, Wu WQ. Cyclodextrin-assisted supramolecular host-guest inclusion for durable and sustainable optoelectronics. Microstructures. 2024; 4(3): 2024031. http://dx.doi.org/10.20517/microstructures.2023.83
Chicago/Turabian Style
Huanyu Chen, Xueqing Chang, Guo Yang, Bing-Xin Lei, Wu-Qiang Wu. 2024. "Cyclodextrin-assisted supramolecular host-guest inclusion for durable and sustainable optoelectronics" Microstructures. 4, no.3: 2024031. http://dx.doi.org/10.20517/microstructures.2023.83
ACS Style
Chen, H.; Chang X.; Yang G.; Lei B.X.; Wu W.Q. Cyclodextrin-assisted supramolecular host-guest inclusion for durable and sustainable optoelectronics. Microstructures. 2024, 4, 2024031. http://dx.doi.org/10.20517/microstructures.2023.83
About This Article
Special Issue
Copyright
Data & Comments
Data
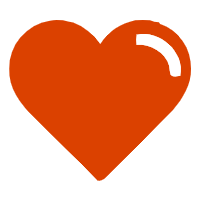
Comments
Comments must be written in English. Spam, offensive content, impersonation, and private information will not be permitted. If any comment is reported and identified as inappropriate content by OAE staff, the comment will be removed without notice. If you have any queries or need any help, please contact us at support@oaepublish.com.