Co-exposure of phthalates, bisphenols, parabens, and polycyclic aromatic hydrocarbons in follicular fluid of women undergoing assisted reproductive technologies and the associations with hormone levels
Abstract
Endocrine-disrupting chemicals (EDCs), particularly phthalates (PAEs), bisphenols, parabens, and polycyclic aromatic hydrocarbons (PAHs), constitute pervasive environmental contaminants with demonstrated potential to adversely affect female reproductive health. Although these compounds are known to exert adverse effects, critical knowledge gaps persist concerning their specific associations with reproductive outcomes. The present study analyzed 144 follicular fluid samples from women undergoing assisted reproductive technology procedures, quantifying concentrations of PAE metabolites (mPAEs), bisphenols, parabens, and hydroxylated PAHs (OH-PAHs). Analytical results demonstrated a descending concentration gradient: mPAEs exhibited the highest median concentration (6.14 ng/mL), followed by parabens (2.17 ng/mL), bisphenols (1.33 ng/mL), and OH-PAHs
Keywords
INTRODUCTION
Endocrine-disrupting chemicals (EDCs) are exogenous compounds that interfere with hormonal signaling, thereby disrupting the development and function of the female reproductive system[1]. The most prevalent EDCs in daily life include phthalates (PAEs), bisphenols, parabens, and polycyclic aromatic hydrocarbons (PAHs). As essential industrial chemicals, PAEs, bisphenols, and parabens are widely incorporated into personal care products, food preservatives, and plastic consumer goods[2,3]. As products of the incomplete combustion of organic substances, human activities are the most important source of PAHs[4]. Women are routinely exposed to these EDCs via multiple pathways including dietary ingestion, respiratory inhalation, and dermal absorption[5]. These compounds have been consistently detected across various biological matrices, including urine, serum, and breast milk samples[6-8].
Many studies have demonstrated the adverse effects of EDCs on female reproductive health[9-12]. Specifically, Peng et al. identified a potential association between PAE exposure and unexplained recurrent spontaneous abortion (URSA) in reproductive-aged women[13], and significantly elevated concentrations of di(2-ethylhexyl) phthalate (DEHP) in 594 URSA cases compared to 569 healthy controls were also observed by our previous study[14]. Furthermore, epidemiological evidence links bisphenol compounds including bisphenol A (BPA), bisphenol S (BPS), and bisphenol F (BPF) to increased risks of polycystic ovary syndrome (PCOS) and endometriosis[15,16]. Similarly, several studies have established correlations between paraben exposure and both ovarian volume abnormalities and endometriosis incidence[17,18]. In addition, PAH exposure has been significantly associated with elevated premature ovarian failure (POF) risk[19].
Estrogen and progesterone, as critical reproductive hormones, play indispensable roles in female reproductive health[15,20,21], with well-established associations to PCOS development, endometriosis progression, and clinical pregnancy outcomes[21-23]. Numerous studies demonstrate significant correlations between circulating hormone levels and urinary EDC concentrations. Sathyanarayana et al. identified positive associations between serum estradiol (E2) and urinary concentrations of monoisobutyl phthalate (MiBP), monobenzyl phthalate (MBzP), and di(2-ethylhexyl) phthalate (DEHP) metabolites[24]. Similarly, Yuan et al. reported comparable findings for urinary 1-OH-PHE and E2 levels[25]. However, an inverse relationship between urinary BPA concentrations and peak serum E2 levels was reported by MokLin et al.[26]. Moreover, elevated urinary methylparaben (MeP) concentrations have been consistently associated with lower serum luteinizing hormone (LH) and follicle-stimulating hormone (FSH) levels[27,28]. These results indicate that EDC exposure may disrupt reproductive endocrine homeostasis, potentially contributing to the pathogenesis of various reproductive disorders.
The ovary represents the female-specific reproductive organ whose functional status serves as a direct indicator of the reproductive health of women. Within ovarian physiology, follicles constitute the fundamental endocrine and reproductive functional units[29]. Follicular fluid contaminant concentrations can reflect the actual exposure doses reaching the female reproductive system, thereby offering critical insights into potential reproductive health impacts. A study in South American women undergoing assisted reproduction found that specific urinary and follicular fluid phthalate metabolites were associated with altered ovarian response and embryo development parameters, revealing both detrimental and unexpected beneficial correlations, warranting further investigation into the complex role in assisted reproductive technology (ART) outcomes by phthalate exposure[10]. Despite this significance, current research on EDCs in follicular fluid remains very limited[10,30-35]. Most existing studies have predominantly investigated contaminant effects on female reproductive health using other biological matrices such as urine and serum[6,8,36].
Given the unclear effects of EDC exposure on female infertility and the crucial role of reproductive hormones in female reproduction, we hypothesized that EDCs may promote infertility development through hormonal disruption. Therefore, the present study aimed to: (1) quantify EDC concentrations in follicular fluid; (2) investigate their associations with reproductive hormones and infertility subtypes; and (3) elucidate their specific effects on estradiol and progesterone levels. By analyzing the concentrations of EDCs or their metabolites in follicular fluid, the present findings provide novel insights into mixture effects on hormonal secretion and infertility pathogenesis, establishing a valuable foundation for female reproductive health risk assessment.
MATERIALS AND METHODS
Reagents and materials
Four kinds of standards containing parabens including MeP, propylparaben (PRP), butylparaben (BUP), ethylparaben (ETP), heptylparaben (HEP), and benzylparaben (BzP), bisphenols including BPA, BPS, BPF, bisphenol B (BPB), bisphenol AF (BPAF), bisphenol Z (BPZ), bisphenol P (BPP), and bisphenol AP (BPAP), hydroxylated polycyclic aromatic hydrocarbons (OH-PAHs) including 1-hydroxynathalene
β-glucuronidase was obtained from Sigma Aldrich Corp (St. Louis, MO, USA). Methanol, acetonitrile, and MAX solid-phase extraction (SPE) cartridges (10 mg/1 mL) were purchased from CNW Technologies (Shanghai, China).
Sample collection and preparation
This study enrolled 144 women aged ≤ 40 years with anti-Müllerian hormone (AMH) levels > 1.1 ng/mL who were undergoing ART treatment at Shunde Hospital of Southern Medical University (2021-2022). From each participant, follicular fluid (> 2 mL) and corresponding serum samples for hormonal analysis were collected. Participants completed brief lifestyle questionnaires. All samples were stored at -80 °C prior to laboratory analysis. All the volunteers signed the informed consent form and to participate in the present study. The experiments were approved by the Ethics Committee of Shunde Hospital of Southern Medical University (20210301).
Sample pretreatment and instrumental analysis
In the present study, mPAEs, bisphenols, parabens, and OH-PAHs in the follicular fluid were detected, and the pretreatment was similar to a previous study[31]. In brief, the internal standards of mPAEs, bisphenols, parabens, and OH-PAHs were added to the follicular fluid samples (200 μL), which were hydrolyzed by β-glucuronidase at 37 °C for 180 min. Then, the mixture was subsequently loaded onto MAX SPE cartridges, with target compounds eluted using 1 mL of 2% formic acid in methanol. All target compounds were separated by a Poroshell 120 EC-C18 column (100 mm × 4.6 mm, 2.7 μm particle diameter, Agilent) and determined by ultra-performance liquid chromatography-tandem mass spectrometry. Parabens and mPAEs were analyzed by applying a gradient of Milli-Q water and acetonitrile, while bisphenols and OH-PAHs were analyzed by applying a gradient of 2 mM ammonium acetate and methanol. Complete methodological details are provided in the Supplementary Text 1 and 2, with instrumental parameters summarized in Supplementary Table 1.
Quality assurance and quality control
To ensure analytical accuracy, each sample batch included one procedural blank, one reagent blank, and one matrix-spiked sample for recovery assessment and background contamination evaluation. The recoveries were 77%-109% for mPAEs, 74%-97% for parabens, 63%-108% for bisphenols, and 64%-105% for OH-PAHs. The background contamination concentrations were 4.14-8.91 ng/mL (mPAEs), 12.19-
Statistical analysis
Statistical analysis was carried out using an SPSS software version 27.0 (IBM, USA) with concentrations below the limit of detection (LOD) assigned zero values. Values between LOD and limit of quantitation (LOQ) were calculated as one-fourth of the LOQ when detection frequencies were below 50%, or as one-half of the LOQ when detection frequencies exceeded 50%[37]. The LOQs and LODs are presented in Supplementary Table 2. Nonparametric analyses included the Kruskal-Wallis H test and Mann-Whitney U test for variable comparisons, while Spearman’s correlation analysis evaluated both inter-compound relationships and compound-hormone associations, with all tests being two-tailed at a significance level of
To comprehensively investigate the relationships between target compounds and hormone levels, Python 13.0 (Guido van Rossum, Netherlands) for advanced statistical modeling was used. Initial multiple nonlinear regression analysis revealed complex nonlinear associations and dependencies among variables. Subsequent multivariate analyses incorporated logistic regression, support vector classification (SVC), classification trees, and neural networks to rigorously examine and verify the correlations from different perspectives and with different modeling approaches. This multi-methodological approach enabled robust characterization of subtle compound-hormone interactions while ensuring the reliability and validity of findings.
Bayesian kernel machine regression (BKMR), a robust method for assessing mixture health effects[38], was also used to explore the influence of chemical mixtures on hormone regulation. The BKMR model generated posterior inclusion probabilities (PIPs) to quantify individual chemical contributions, with a PIP threshold of 0.50 considered statistically significant[39]. Model adjustments incorporated age, body mass index (BMI), and therapeutic regimen as covariates. Complementary analysis using the Quantile g-computation (Qgcomp) model determined chemical-specific weight contributions. All analyses were conducted in R 4.1 (R Foundation for Statistical Computing, Vienna, Austria).
Sensitivity analysis
Sensitivity analyses confirmed the robustness of the results to methodological choices. Correlation-based screening retained only chemicals significantly associated with hormones, excluding weakly correlated ones, to reduce potential confounding. In the BKMR model, varying the kernel bandwidth and iteration parameters yielded stable exposure importance rankings, despite minor fluctuations in PIPs. The exposure-response curves also exhibited consistent trends, supporting the robustness of variable selection. For the Qgcomp model, sensitivity to quantile grouping parameters was evaluated by testing different discretization levels. Although slight variations in magnitude were observed, the weight trends and directional consistency of mixture effects remained stable across scenarios.
RESULTS AND DISCUSSION
Demographic characteristics of the study population
The demographic characteristics and serum hormone levels of the subjects are shown in Table 1. The cohort comprised women aged 22-40 years (mean age = 32 years) with an average infertility duration of approximately 3 years. All participants reported being non-smokers and abstaining from alcohol consumption.
Characteristics of the study population
Items | Subject (n = 144) | Items | Subject (n = 144) |
Age | Infertile years | ||
Mean (SD) | 31.9 (4.04) | Mean (SD) | 3.13 (2.54) |
BMI | Endometrial thickness (mm) | ||
Mean (SD) | 21.37 (3.26) | Mean (SD) | 10.04 (2.57) |
AMH (ng/mL) | Basal AFC | ||
Mean (SD) | 3.71 (3.62) | Mean (SD) | 16.31 (8.88) |
Basal_T (nmol/L) | Basal_P (nmol/L) | ||
Mean (SD) | 3.04 (17.36) | Mean (SD) | 0.47 (14.8) |
HCG_E2 (pg/mL) | HCG_number | ||
Mean (SD) | 3,163.9 (2,683.1) | Mean (SD) | 9.94 (5.67) |
Alcohol, n (%) | 0 | Smoking, n (%) | 0 |
Infertility form | n (%) | Therapeutic regimen | n (%) |
Primary infertility | 77 (53.5%) | ICSI | 1 (0.7%) |
Secondary infertility | 67 (46.5%) | IVF | 143 (99.3%) |
Moreover, the present study analyzed hormonal parameters during days 2-3 of the menstrual cycle, measuring basal testosterone (Basal_T), basal progesterone (Basal_P), and basal antral follicle count (Basal_AFC). On human chorionic gonadotropin (HCG) trigger day, estradiol levels (HCG_E2) and follicles ≥ 14 mm in diameter (HCG_number) were also evaluated. Furthermore, variations were observed in the forms of infertility among the subjects, specifically including primary infertility and secondary infertility. It was also found that most of them received in vitro fertilization (IVF) as a therapeutic regimen.
Detection frequencies of the target chemicals in the follicular fluid
The detection frequencies of mPAEs, bisphenols, parabens, and OH-PAHs in follicular fluid are shown in Table 2. The majority of target compounds were reliably detected, demonstrating that EDCs including PAEs, bisphenols, parabens, and PAHs can enter the female reproductive organs with potentially adverse effects on female reproductive health.
Distributions and levels of quantification of chemicals evaluated in primary analyses (ng/mL)
Compounds | Mean (SD) | Median [min, max] | Quantified, n (%) |
mPAEs | |||
MMP | 2.86 (4.16) | 1.52 [0.07, 31.1] | 118 (82%) |
MeP | 2.67 (4.00) | 1.29 [0.07, 25.0] | 133 (92%) |
MBP | 2.91 (4.39) | 1.43 [0.09, 29.9] | 121 (84%) |
MiBP | 1.29 (1.32) | 1.03 [0.13, 11.0] | 120 (83%) |
MEHP | 0.99 (3.67) | 0.25 [0.01, 33.0] | 85 (59%) |
MEHHP | 0.71 (1.99) | 0.39 [0.06, 19.5] | 98 (68%) |
MBzP | 0 | 0 | 0 |
MOP | 0.93 (2.88) | 0.25 [0.25, 28.8] | 102 (71%) |
ΣmPAEs | 10.1 (11.7) | 6.14 [0.37, 87.5] | |
Bisphenols | |||
BPAF | 0.08 (0.01) | 0.08 [0.06, 0.10] | 13 (9%) |
BPA | 4.43 (5.86) | 2.37 [0.46, 36.0] | 81 (56%) |
BPS | 0.69 (1.04) | 0.22 [0.04, 4.72] | 48 (33%) |
BPB | 0 | 0 | 0 |
BPF | 0.97 (0.33) | 0.85 [0.68, 1.55] | 9 (6%) |
BPAP | 0.04 (0.01) | 0.04 [0.03, 0.08] | 26 (18%) |
BPZ | 0.09 (0.06) | 0.08 [0.04, 0.37] | 37 (26%) |
BPP | 0.07(0.05) | 0.05 [0.01, 0.24] | 52 (36%) |
ΣBisphenols | 2.84 (4.93) | 1.33 [0, 36.14] | |
Parabens | |||
MeP | 1.76 (1.31) | 1.49 [0.16, 4.40] | 34 (24%) |
ETP | 0.67 (1.57) | 0.21 [0.07, 8.00] | 28 (19%) |
PRP | 2.40 (2.03) | 1.90 [0.27, 10.2] | 143 (99%) |
BUP | 0.04 (0.04) | 0.03 [0.01, 0.13] | 11 (8%) |
BzP | 0.13 (0.08) | 0.10 [0.07, 0.22] | 3 (2%) |
HEP | 0 | 0 | 0 |
ΣParabens | 2.94 (2.72) | 2.17 [0, 16.1] | |
OH-PAHs | |||
1-OH-NAP | 0.76 (0.69) | 0.52 [0.03, 3.55] | 80 (56%) |
2-OH-NAP | 0.77 (0.72) | 0.54 [0, 2.84] | 63 (44%) |
2-OH-FLU | 0.10 (0.11) | 0.08 [0, 0.50] | 39 (27%) |
3-OH-FLU | 0.02 (0.06) | 0.00 [0, 0.24] | 4 (3%) |
1/9-OH-PHE | 0 | 0 | 0 |
3-OH-PHE | 0 | 0 | 0 |
4-OH-PHE | 0 | 0 | 0 |
ΣOH-PAHs | 0.84 (1.24) | 0.26 [0, 5.75] |
Among the four classes of target compounds, mPAEs exhibited the highest detection frequencies, demonstrating widespread PAE exposure among participants. With the exception of non-detectable MBzP, all the other mPAEs showed detection frequencies exceeding 50%. The detection frequencies followed a descending order of MeP (92%), MBP (84%), MiBP (83%), MMP (82%), MOP (71%), MEHHP (68%), and MEHHP (59%). Li et al. also reported detection frequencies above 60% for mPAEs in follicular fluid from women undergoing in-vitro fertilization/intracytoplasmic sperm injection and embryo transfer procedures[40]. The consistent detection of these compounds provides compelling evidence for PAE accumulation in female reproductive tissues.
Bisphenols, parabens, and OH-PAHs showed significantly lower detection frequencies compared to mPAEs. Among bisphenols, BPA exhibited the highest detection frequency (56%), while other analogs were detected in < 50% of samples: BPP (36%), BPS (33%), and BPB (non-detectable). For parabens, PRP demonstrated exceptionally high detection (99%), whereas others showed lower frequencies in descending order of MeP (24%), ETP (19%), BUP (8%), BzP (2%), and HEP (non-detectable). Bellavia et al. reported that both bisphenols and parabens showed certain non-detection rates, with bisphenols were significantly higher, exceeding 80% (such as 95.5% for BPA and 80.8% for BPS) in the follicular fluid of Sweden and Estonia women undergoing ART treatment, while those of most parabens were less than 50% (such as 33.6% for PRP and 45.9% for ETP)[31]. The variable detection patterns suggest regional differences in exposure profiles, potentially reflecting lifestyle variations affecting chemical accumulation in reproductive tissues.
Among OH-PAHs, 1-OH-NAP showed the highest follicular fluid detection frequency (56%), followed by 2-OH-NAP (44%), 2-OH-FLU (27%), and 3-OH-FLU (3%), while monohydroxy PHE metabolites (1/3/4/9-OH-PHE) were non-detectable. This contrasts with our previous urinary biomonitoring data, which reported substantially higher detection rates: 2-OH-NAP (91.3%), 1-OH-NAP (82.5%), 3-OH-PHE (53.3%), 4-OH-PHE (36.3%), 2-OH-FLU (16.7%), and 3-OH-FLU (7.1%)[41]. The marked discrepancy between follicular fluid and urinary profiles supports the established metabolic pathway wherein PAHs are preferentially metabolized to monohydroxy compounds and excreted renally[42], resulting in lower accumulation in reproductive tissues.
Levels of mPAEs, bisphenols, parabens, and OH-PAHs in the follicular fluid
As shown in Supplementary Figure 1 and Table 2, ΣmPAEs exhibited the highest median concentration (6.14 ng/mL, range: 0.37-87.53 ng/mL) among the measured contaminants. For individual mPAE compounds, MMP demonstrated the highest median concentration (1.52 ng/mL), followed by MBP
For bisphenols, the analysis revealed the concentrations of Σbisphenols ranging from 0 to 36.1 ng/mL (median: 1.33 ng/mL) in follicular fluid [Supplementary Figure 1 and Table 2]. BPA demonstrated the highest median concentration (2.37 ng/mL), followed by its alternatives BPF (0.85 ng/mL) and BPS
Paraben analysis revealed Σparabens ranging from 0 to 16.1 ng/mL (median: 2.17 ng/mL) in follicular fluid
Compared with the above EDCs, OH-PAHs exhibited significantly lower exposure levels in follicular fluid with ΣOH-PAHs ranging from 0 to 5.75 ng/mL (median: 0.26 ng/mL) [Supplementary Figure 1 and Table 2]. Among these compounds, monohydroxy Nap metabolites showed the highest median concentrations (1-OH-NAP: 0.52 ng/mL; 2-OH-NAP: 0.54 ng/mL), while Flu metabolites demonstrated substantially lower levels (2-OH-FLU: 0.08 ng/mL; 3-OH-FLU: non-detectable). Similar compositions were found in human urine, with OH-NAP being the predominant OH-PAHs[4,41,47]. Inhalation represents a probable major exposure route for PAHs in this non-smoking cohort, with likely environmental sources including petrochemical combustion, vehicular emissions, and secondhand smoke exposure. Inhalation may represent a significant route of human exposure to PAHs. Since none of the study participants were habitual smokers, potential sources of PAHs likely include incomplete petrochemical combustion, vehicle emissions, and secondhand smoke[48,49].
Association of various chemicals with population hormones and infertility form
As shown in Figure 1, the study assessed correlations between demographic characteristics (age and BMI), hormone levels, infertility form, and follicular fluid concentrations of chemicals, including mPAEs, bisphenols, parabens, and OH-PAHs. Based on literature defining advanced maternal age as ≥ 35 years, participants were categorized into advanced (≥ 35 years) and non-advanced (< 35 years) groups for analysis[50]. No significant differences were observed in EDC exposure relative to age or BMI [Figure 1A and B]. However, variations in EDC metabolite concentrations were detected in follicular fluid across different Basal_T levels, with Basal_T levels exhibiting an increasing trend alongside higher EDC exposure, particularly for bisphenols (P = 0.030) [Figure 1C]. EDCs, such as bisphenols, modulate testosterone levels by inhibiting steroidogenic enzymes and disrupting negative feedback in the hypothalamic-pituitary-testicular (HPT) axis. Studies showed that BPA directly impairs Leydig cells, hindering cholesterol conversion to testosterone, while chronic low-dose exposure suppresses LH and FSH secretion, perpetuating testosterone decline[51,52]. Conversely, short-term high-dose exposure may transiently elevate testosterone, as demonstrated by positive correlations between urinary BPA levels and total testosterone in Italian men, and between serum BPA and total/free testosterone in women with PCOS[53,54]. Additionally, bisphenols may dysregulate genes involved in ovarian steroidogenesis, indirectly increasing testosterone[55]. Furthermore, higher testosterone levels (in PCOS) may contribute to impaired folliculogenesis[56] and adverse cognitive effects[57]. Hence, bisphenol exposure may pose a health risk to the female reproductive system by causing high levels of testosterone.
Figure 1. Differences in the levels of bisphenols, OH-PAHs, parabens, and mPAEs in follicular fluid based on demographic characteristics. (A) Age; (B) BMI; (C) Basal_T; (D) Infertility form. The lower and upper limits of the box denote the 25th and 75th quartiles, respectively. The horizontal line within the box represents the median value. The length of the whiskers is 1.5 times the interquartile range. The rhombus symbol denotes the 99th percentile. The small rectangular boxes represent average values, and the star symbol indicates that there are significant differences between groups [Kruskal–Wallis H and Mann–Whitney U tests were used. *P < 0.05 (2-tailed)]. OH-PAHs: Hydroxyl polycyclic aromatic hydrocarbon; mPAEs: phthalate metabolites; BMI: body mass index; Basal_T: basal testosterone.
Epidemiological studies on infertility covered primary infertility (defined as the inability to conceive in nulligravid women) and secondary infertility (characterized by parous women’s failure to conceive or carry a pregnancy to term, irrespective of previous outcomes)[58]. As shown in Figure 1D, the investigation of EDC metabolite concentrations in follicular fluid revealed a potential association between PAE exposure and secondary infertility development (P = 0.037). Follicles are the functional units of the ovary. The process of folliculogenesis enables the maintenance, development, and maturation of oocytes[59]. Epidemiological evidence demonstrates that PAE exposure directly impairs ovarian function by restricting sinus follicle growth and development, thereby contributing to diminished ovarian reserve (DOR) and infertility[44]. An in vitro study has shown that DEHP exposure significantly suppresses primordial follicle aggregation, leading to reduced primordial follicle counts and increased oocyte apoptosis[60]. Furthermore, PAE exposure has been shown to disrupt ovulation by modifying oocyte biochemical markers[61]. Therefore, these findings collectively indicate that PAEs can compromise fertility through ovarian dysfunction and may particularly contribute to secondary infertility development.
The correlations between chemical mixtures and hormones
To investigate potential associations between chemical mixtures and hormones, a series of systematic correlation analyses were conducted. As shown in Table 3, EDC metabolites showing statistically significant associations with hormone levels (HCG_E2 and Basal_P) were initially identified (P < 0.05). While significant correlations between EDC metabolites and these two hormones were observed, the correlation coefficients remained relatively low. The correlation heatmap revealed not only strong intra-category chemical correlations, such as between MBP and MeP (r = 0.91) and between MOP and MEHHP (r = 0.55)
The correlations between the chemicals and hormones [*P < 0.05; **P < 0.01 (2-tailed)]
Population hormone | Chemical a1 | Chemical a2 | Chemical a3 | Chemical a4 | Chemical a5 | Chemical group | Related group | |
HCG_E2a,b | BPS | BPAP | BPZ | MeP | MBP | A | I | |
Rc | -0.169* | 0.175* | -0.168* | 0.242** | 0.226** | |||
Basal_Pa,d | BPS | BPP | BUP | B | II | |||
R | 0.249** | -0.231** | 0.270** |
Therefore, EDC metabolites demonstrating significant associations with either HCG_E2 or Basal_P (P < 0.05) were selected for subsequent analysis of potential relationships between EDC mixture concentrations in follicular fluid and hormone levels. Consequently, two distinct correlation groups were established for further investigation: Group I comprising HCG_E2 with chemical group A (BPS, BPAP, BPZ, MeP, and MBP), and Group II consisting of Basal_P with chemical group B (BPS, BPP, and BUP).
To more comprehensively and accurately assess the correlation between chemical mixtures and hormone levels (the aforementioned groups), a multivariate nonlinear regression model was employed. However, the model’s performance proved suboptimal. Consequently, hormone levels were stratified into quartiles, and classification models were subsequently implemented for simulation analysis [Supplementary Table 3]. Model accuracy metrics indicated correlation values exceeding 0.4 - substantially higher than the coefficients observed for individual chemical-hormone correlations. These findings demonstrate that chemical mixtures exhibit significantly stronger associations with hormone levels compared to single compounds. Collectively, these results warrant further investigation into the effects of chemical mixtures on HCG_E2 and Basal_P levels.
The effect of chemical mixtures on hormones
To elucidate the effects of chemical mixtures on hormone levels, both BKMR and Qgcomp models for comprehensive analysis were employed for in-depth analysis, with results presented in Figure 2. The analysis revealed a significant downward trend in HCG_E2 and Basal_P levels when chemical mixture concentrations were at a higher quartile (> 55%-65%) [Figure 2A]. The PIP of each compound in the model was presented in Table 4. Regarding HCG_E2, BPS demonstrated the highest PIP value (0.720), followed by MeP (0.637) and MBP (0.428), indicating their predominant influence relative to other compounds. For Basal_P, BPP showed the strongest association (PIP = 0.587), with BUP (0.401) and BPS (0.292) following, suggesting BPP and BUP as primary contributors. Exposure-response relationships exhibited nonlinear decreasing trends between HCG_E2 and specific chemicals (BPS, BPAP, and BPB; Figure 2B), with analogous patterns observed for Basal_P in relation to BPS, BPP, and BUP [Figure 2C]. Notably, no significant associations emerged between individual chemical concentrations and either HCG_E2 or Basal_P levels [Figure 2B and C], suggesting that the observed negative trends predominantly stem from mixture effects rather than single compounds.
Figure 2. Joint association (95%CI) of the mixture on hormone levels. (A) Observe the combined effect (95%CI) of the selected chemical group and HCG_E2/Basal_P through the BKMR model; (B and C) The correlation between a single chemical and HCG_E2 and Basal_P when all other exposed compounds are fixed at the 25th, 50th, or 75th percentile of exposure. The univariate exposure-response function (95%CI) of the selected chemical concentration on HCG_E2 and Basal_P. h(Z) is interpreted as the relationship between the chemical and the potential continuous outcome; (D) The Qgcomp model is used to reveal a bar chart of the relative weight of each chemical and its influence on hormone levels. HCG_E2: Estradiol on HCG trigger day; Basal_P: basal progesterone; BKMR: Bayesian kernel machine regression.
PIPs for the chemical-related group incorporated into the HCG_E2 and Basal_P models, utilizing the BKMR model
PIP | |||
Chemical | HCG_E2 | Chemical | Basal_P |
BPS | 0.720 | BPS | 0.292 |
MBP | 0.428 | BPP | 0.587 |
MeP | 0.637 | BUP | 0.401 |
BPZ | 0.359 | ||
BPAP | 0.313 |
Consequently, the Qgcomp model was implemented for additional analysis, with age, BMI, and therapeutic regimen adjusted as covariates. The analysis demonstrated negative weight values for chemical mixtures in the model [Figure 2D]. Regarding HCG_E2, BPS exhibited the strongest negative association (weight = 0.651), followed by BPZ (0.213), MeP (0.069), and MBP (0.068). For Basal_P, BPP showed the most pronounced effect (weight = 0.593), with BUP (0.280) and BPS (0.127) displaying weaker associations. Collectively, these results indicate an inverse relationship between follicular fluid chemical mixture concentrations and hormone levels, with BPS and BPP emerging as the primary contributing factors for HCG_E2 and Basal_P, respectively.
The concordance between BKMR and Qgcomp model results mutually reinforces the reliability of the conclusions, demonstrating consistent negative associations between chemical mixtures and hormone levels. These findings suggest that elevated follicular fluid concentrations of chemical mixtures may substantially decrease estradiol and progesterone levels. Similar findings have been reported in the previous study, including Shi et al., who similarly reported model consistency in demonstrating positive associations between various mPAEs and abdominal adiposity in women[39]. Regarding female reproductive physiology, estradiol and progesterone serve critical functions. As Panagopoulos et al. documented, both hormones, i.e., estradiol and progesterone, are indispensable for uterine development[62]. Specifically, estradiol serves as a predictive biomarker for mature oocyte yield in ART cycles[20], while progesterone is fundamental for terminal follicular maturation and ovulation[63]. Clinically, depressed levels of these hormones may promote failure of mature follicle development, irregular menstruation, and elevated miscarriage risk[21,63].
Previous studies established that PAEs exhibit reproductive toxicity by interfering with ovarian estradiol production through inhibition of follicular estradiol synthesis[63-65]. BPA, structurally analogous to 17-β estradiol, functions as both an estrogen receptor agonist and antagonist, thereby disrupting endocrine and reproductive system function[66]. Although BPS was introduced as a presumably safer industrial alternative to BPA, emerging evidence indicates comparable adverse effects on human reproductive health[62]. For example, in vitro studies demonstrate BPS-induced suppression of progesterone and estradiol secretion in sheep granulosa cells[67]. These findings suggested that both PAEs and bisphenols may negatively affect the secretion of estradiol and progesterone, thereby causing adverse effects on female reproductive health. However, current literature primarily examines EDC effects on hormonal profiles through urinary biomarkers, revealing positive correlations between serum estradiol and mPAEs/1-OH-PHE, an inverse relationship between BPA and peak estradiol, and negative associations between MeP and LH/FSH ratios[24-28]. These findings underscore the need for mechanistic studies to elucidate how chemical mixtures influence female endocrine physiology.
Limitations of the study
First, due to the cross-sectional design of our study, the observed associations cannot definitively establish causality. Future longitudinal studies, particularly those including clinical populations such as women with PCOS or DOR, are warranted to further investigate the effects of chemical mixtures on hormone levels. Second, the EDCs or their metabolites (mPAEs, bisphenols, parabens, and OH-PAHs) analyzed in the present study have relatively short half-lives[4,10,68]. Additionally, follicular fluid samples were collected only on the day of oocyte retrieval during ART procedures, which limits their temporal scope. Consequently, these measurements better reflect short-term rather than long-term exposure. Third, while our study emphasizes the combined effects of chemical mixtures, the inherent complexity of these mixtures, including potential interactions among different EDCs, merits further investigation. More studies are needed to elucidate the underlying mechanisms by which EDCs influence hormonal regulation.
CONCLUSIONS
Analysis of 144 follicular fluid samples from women undergoing ART treatment detected measurable concentrations of mPAEs, bisphenols, parabens, and OH-PAHs, with mPAEs exhibiting the highest median concentration followed by parabens, bisphenols, and OH-PAHs. The study revealed a potential association between PAE exposure and secondary infertility, along with a positive correlation between follicular fluid bisphenol levels and testosterone concentrations. BKMR analysis indicated that EDC mixtures, rather than individual compounds, primarily influenced estradiol levels on HCG trigger day and basal progesterone concentrations, demonstrating an inverse dose-response relationship where higher mixture concentrations corresponded to lower hormone levels. The Qgcomp model further identified BPS as having the most pronounced negative effect on estradiol levels on HCG trigger day, while BPP showed the strongest impact on basal progesterone. These findings collectively suggest that EDCs may adversely affect female reproductive health through hormonal disruption. Given the complex mechanistic pathways underlying EDC effects on endocrine function and reproductive outcomes, additional research is clearly warranted to fully elucidate these relationships.
DECLARATIONS
Authors’ contributions
Methodology, data analysis and draft preparation: Dou, X.
Sampling, methodology and data analysis: Li, X.
Data analysis: Huang, S.; Long, C.; Chen, C.
Design, reviewing and editing: Chen, X.; Yu, Y.
Availability of data and materials
Information related to this article can be found in Supplementary Materials. Further data are available from the corresponding authors upon reasonable request.
Financial support and sponsorship
The study was supported by open research funds from the Affiliated Qingyuan Hospital (Qingyuan People’s Hospital), Guangzhou Medical University (202301-104), Talent Project of Center for Disease Prevention and Control of Guangdong Province (2024D344), Guangdong Province Outstanding Youth Medical Talent Training Program (SRSP2022022), and Guangdong-Hong Kong-Macao Joint Laboratory for Contaminants Exposure and Health (2020B1212030008).
Conflicts of interest
Yu, Y. is the Guest Editor of the Special Issue of “The Impact of Bisphenol Exposure on Human Health”. Yu, Y. is not involved in any steps of editorial processing, notably including reviewer selection, manuscript handling, and decision making. The other authors declared that there are no conflicts of interest.
Ethical approval and consent to participate
The study was approved by the Ethics Committee of Shunde Hospital of Southern Medical University (20210301). All donors involved in this study signed an informed consent form before the sample collection.
Consent for publication
Not applicable.
Copyright
© The Author(s) 2025.
Supplementary Materials
REFERENCES
1. Basso, C. G.; de Araújo-Ramos, A. T.; Martino-Andrade, A. J. Exposure to phthalates and female reproductive health: a literature review. Reprod. Toxicol. 2022, 109, 61-79.
2. Carwile, J. L.; Luu, H. T.; Bassett, L. S.; et al. Polycarbonate bottle use and urinary bisphenol A concentrations. Environ. Health. Perspect. 2009, 117, 1368-72.
3. Husøy, T.; Andreassen, M.; Hjertholm, H.; et al. The Norwegian biomonitoring study from the EU project EuroMix: levels of phenols and phthalates in 24-hour urine samples and exposure sources from food and personal care products. Environ. Int. 2019, 132, 105103.
4. Huang, S.; Li, Q.; Liu, H.; et al. Urinary monohydroxylated polycyclic aromatic hydrocarbons in the general population from 26 provincial capital cities in China: levels, influencing factors, and health risks. Environ. Int. 2022, 160, 107074.
5. Wittassek, M.; Koch, H. M.; Angerer, J.; Brüning, T. Assessing exposure to phthalates - the human biomonitoring approach. Mol. Nutr. Food. Res. 2011, 55, 7-31.
6. Aker, A. M.; Johns, L.; McElrath, T. F.; Cantonwine, D. E.; Mukherjee, B.; Meeker, J. D. Associations between maternal phenol and paraben urinary biomarkers and maternal hormones during pregnancy: a repeated measures study. Environ. Int. 2018, 113, 341-9.
7. Fisher, M.; MacPherson, S.; Braun, J. M.; et al. Paraben concentrations in maternal urine and breast milk and its association with personal care product use. Environ. Sci. Technol. 2017, 51, 4009-17.
8. Sathyanarayana, S.; Barrett, E.; Butts, S.; Wang, C.; Swan, S. H. Phthalate exposure and reproductive hormone concentrations in pregnancy. Reproduction 2014, 147, 401-9.
9. Bolden, A. L.; Rochester, J. R.; Schultz, K.; Kwiatkowski, C. F. Polycyclic aromatic hydrocarbons and female reproductive health: a scoping review. Reprod. Toxicol. 2017, 73, 61-74.
10. Hallberg, I.; Björvang, R. D.; Hadziosmanovic, N.; et al. Associations between lifestyle factors and levels of per- and polyfluoroalkyl substances (PFASs), phthalates and parabens in follicular fluid in women undergoing fertility treatment. J. Expo. Sci. Environ. Epidemiol. 2023, 33, 699-709.
11. Wei, M.; Chen, X.; Zhao, Y.; Cao, B.; Zhao, W. Effects of prenatal environmental exposures on the development of endometriosis in female offspring. Reprod. Sci. 2016, 23, 1129-38.
12. Zhou, B.; Yang, P.; Deng, Y. L.; Zeng, Q.; Lu, W. Q.; Mei, S. R. Prenatal exposure to bisphenol a and its analogues (bisphenol F and S) and ultrasound parameters of fetal growth. Chemosphere 2020, 246, 125805.
13. Peng, F.; Ji, W.; Zhu, F.; et al. A study on phthalate metabolites, bisphenol A and nonylphenol in the urine of Chinese women with unexplained recurrent spontaneous abortion. Environ. Res. 2016, 150, 622-8.
14. Aimuzi, R.; Huang, S.; Luo, K.; et al. Levels and health risks of urinary phthalate metabolites and the association between phthalate exposure and unexplained recurrent spontaneous abortion: a large case-control study from China. Environ. Res. 2022, 212, 113393.
15. Kandaraki, E.; Chatzigeorgiou, A.; Livadas, S.; et al. Endocrine disruptors and polycystic ovary syndrome (PCOS): elevated serum levels of bisphenol A in women with PCOS. J. Clin. Endocrinol. Metab. 2011, 96, E480-4.
16. Peinado, F. M.; Lendínez, I.; Sotelo, R.; et al. Association of urinary levels of bisphenols A, F, and S with endometriosis risk: preliminary results of the EndEA study. Int. J. Environ. Res. Public. Health. 2020, 17, 1194.
17. Pacyga, D. C.; Chiang, C.; Li, Z.; Strakovsky, R. S.; Ziv-Gal, A. Parabens and menopause-related health outcomes in midlife women: a pilot study. J. Womens. Health. 2022, 31, 1645-54.
18. Wieczorek, K.; Szczęsna, D.; Jurewicz, J. Environmental exposure to non-persistent endocrine disrupting chemicals and endometriosis: a systematic review. Int. J. Environ. Res. Public. Health. 2022, 19, 5608.
19. Ye, X.; Pan, W.; Li, C.; et al. Exposure to polycyclic aromatic hydrocarbons and risk for premature ovarian failure and reproductive hormones imbalance. J. Environ. Sci. 2020, 91, 1-9.
20. Malathi, A.; Balakrishnan, S.; Lakshimi, B. S. Correlation between estradiol levels on day of HCG trigger and the number of mature follicles, number of oocytes retrieved, and the number of mature oocytes (M2) after oocyte aspiration in ICSI cycles. Middle. East. Fertil. Soc. J. 2021, 26, 34.
21. Nagy, B.; Szekeres-Barthó, J.; Kovács, G. L.; et al. Key to life: physiological role and clinical implications of progesterone. Int. J. Mol. Sci. 2021, 22, 11039.
22. Chantalat, E.; Valera, M. C.; Vaysse, C.; et al. Estrogen receptors and endometriosis. Int. J. Mol. Sci. 2020, 21, 2815.
23. Yang, Y.; Liu, B.; Wu, G.; Yang, J. Exploration of the value of progesterone and progesterone/estradiol ratio on the hCG trigger day in predicting pregnancy outcomes of PCOS patients undergoing IVF/ICSI: a retrospective cohort study. Reprod. Biol. Endocrinol. 2021, 19, 184.
24. Sathyanarayana, S.; Butts, S.; Wang, C.; et al; TIDES Team. Early prenatal phthalate exposure, sex steroid hormones, and birth outcomes. J. Clin. Endocrinol. Metab. 2017, 102, 1870-8.
25. Yuan, Q.; Jin, K.; Zhou, X.; et al. Urinary polycyclic aromatic hydrocarbon metabolites are positively related to serum testosterone levels of males and serum estradiol levels of females among U.S. adults. Front. Endocrinol. 2022, 13, 1037098.
26. Mok-Lin, E.; Ehrlich, S.; Williams, P. L.; et al. Urinary bisphenol A concentrations and ovarian response among women undergoing IVF. Int. J. Androl. 2010, 33, 385-93.
27. Malakootian, M.; Chavoshani, A.; Hashemi, M.; et al. Concentrations of urinary parabens and reproductive hormones in Iranian women: exposure and risk assessment. Toxicol. Rep. 2022, 9, 1894-900.
28. Guth, M.; Pollock, T.; Fisher, M.; Arbuckle, T. E.; Bouchard, M. F. Concentrations of urinary parabens and reproductive hormones in girls 6-17 years living in Canada. Int. J. Hyg. Environ. Health. 2021, 231, 113633.
29. Panagiotou, E. M.; Ojasalo, V.; Damdimopoulou, P. Phthalates, ovarian function and fertility in adulthood. Best. Pract. Res. Clin. Endocrinol. Metab. 2021, 35, 101552.
30. Beck, A. L.; Rehfeld, A.; Mortensen, L. J.; et al. Ovarian follicular fluid levels of phthalates and benzophenones in relation to fertility outcomes. Environ. Int. 2024, 183, 108383.
31. Bellavia, A.; Zou, R.; Björvang, R. D.; et al. Association between chemical mixtures and female fertility in women undergoing assisted reproduction in Sweden and Estonia. Environ. Res. 2023, 216, 114447.
32. Bullach, A.; Trapphoff, T.; Zühlke, S.; Spiteller, M.; Dieterle, S. Impact of nonylphenols and polyhalogenated compounds in follicular fluid on the outcome of intracytoplasmic sperm injection. Reprod. Sci. 2021, 28, 2118-28.
33. Hoffmann-Dishon, N.; Barnett-Itzhaki, Z.; Zalko, D.; et al. Correction to: Endocrine-disrupting chemical concentrations in follicular fluid and follicular reproductive hormone levels. J. Assist. Reprod. Genet. 2024, 41, 1643.
34. Tian, T.; Hao, Y.; Wang, Y.; et al. Mixed and single effects of endocrine disrupting chemicals in follicular fluid on likelihood of diminished ovarian reserve: a case-control study. Chemosphere 2023, 330, 138727.
35. Zhang, S.; Gao, F.; Fu, M.; Zhang, Q.; Guan, J.; Shen, H. Reproductive toxicology of environmental endocrine-disrupting chemicals in women: a cohort study protocol. Front. Cell. Dev. Biol. 2024, 12, 1335028.
36. Petro, E. M.; Leroy, J. L.; Covaci, A.; et al. Endocrine-disrupting chemicals in human follicular fluid impair in vitro oocyte developmental competence. Hum. Reprod. 2012, 27, 1025-33.
37. GB 17378.2-2007. State standard of the People’s Republic of China. The specification for marine monitoring - Part 2: Data processing and quality control of analysis. https://openstd.samr.gov.cn/bzgk/gb/newGbInfo?hcno=2A832569DF947259C8793864F584618F&refer=outter. (accessed 7 May 2025).
38. Bobb, J. F.; Valeri, L.; Claus Henn, B.; et al. Bayesian kernel machine regression for estimating the health effects of multi-pollutant mixtures. Biostatistics 2015, 16, 493-508.
39. Shi, X.; Wang, W.; Feng, J.; Ma, X.; Xu, M.; Wang, C. Gender-specific abdominal fat distribution and insulin resistance associated with organophosphate esters and phthalate metabolites exposure. Environ. Pollut. 2024, 349, 123959.
40. Li, Y.; Xiao, N.; Liu, M.; et al. Dysregulation of steroid metabolome in follicular fluid links phthalate exposure to diminished ovarian reserve of childbearing-age women. Environ. Pollut. 2023, 330, 121730.
41. Li, X.; Zhong, Y.; He, W.; et al. Co-exposure and health risks of parabens, bisphenols, triclosan, phthalate metabolites and hydroxyl polycyclic aromatic hydrocarbons based on simultaneous detection in urine samples from guangzhou, south China. Environ. Pollut. 2021, 272, 115990.
42. Santos, P. M.; del Nogal Sánchez, M.; Pavón, J. L. P.; Cordero, B. M. Determination of polycyclic aromatic hydrocarbons in human biological samples: a critical review. TrAC. Trend. Anal. Chem. 2019, 113, 194-209.
43. Katsikantami, I.; Sifakis, S.; Tzatzarakis, M. N.; et al. A global assessment of phthalates burden and related links to health effects. Environ. Int. 2016, 97, 212-36.
44. Land, K. L.; Miller, F. G.; Fugate, A. C.; Hannon, P. R. The effects of endocrine-disrupting chemicals on ovarian- and ovulation-related fertility outcomes. Mol. Reprod. Dev. 2022, 89, 608-31.
45. Sanchis, Y.; Coscollà, C.; Yusà, V. Analysis of four parabens and bisphenols A, F, S in urine, using dilute and shoot and liquid chromatography coupled to mass spectrometry. Talanta 2019, 202, 42-50.
46. Kolatorova, L.; Vitku, J.; Hampl, R.; et al. Exposure to bisphenols and parabens during pregnancy and relations to steroid changes. Environ. Res. 2018, 163, 115-22.
47. Lin, M.; Tang, J.; Ma, S.; et al. Insights into biomonitoring of human exposure to polycyclic aromatic hydrocarbons with hair analysis: a case study in e-waste recycling area. Environ. Int. 2020, 136, 105432.
48. Sun, H.; Hou, J.; Zhou, Y.; et al. Dose-response relationship between urinary polycyclic aromatic hydrocarbons metabolites and urinary 8-hydroxy-2’-deoxyguanosine in a Chinese general population. Chemosphere 2017, 174, 506-14.
49. Teixeira, E. C.; Agudelo-Castañeda, D. M.; Mattiuzi, C. D. Contribution of polycyclic aromatic hydrocarbon (PAH) sources to the urban environment: a comparison of receptor models. Sci. Total. Environ. 2015, 538, 212-9.
50. Correa-de-Araujo, R.; Yoon, S. S. S. Clinical outcomes in high-risk pregnancies due to advanced maternal age. J. Womens. Health. 2021, 30, 160-7.
51. Nakamura, D.; Yanagiba, Y.; Duan, Z.; et al. Bisphenol A may cause testosterone reduction by adversely affecting both testis and pituitary systems similar to estradiol. Toxicol. Lett. 2010, 194, 16-25.
52. Yadav, S. K.; Bijalwan, V.; Yadav, S.; Sarkar, K.; Das, S.; Singh, D. P. Susceptibility of male reproductive system to bisphenol A, an endocrine disruptor: updates from epidemiological and experimental evidence. J. Biochem. Mol. Toxicol. 2023, 37, e23292.
53. Galloway, T.; Cipelli, R.; Guralnik, J.; et al. Daily bisphenol A excretion and associations with sex hormone concentrations: results from the InCHIANTI adult population study. Environ. Health. Perspect. 2010, 118, 1603-8.
54. Takeuchi, T.; Tsutsumi, O.; Ikezuki, Y.; Takai, Y.; Taketani, Y. Positive relationship between androgen and the endocrine disruptor, bisphenol A, in normal women and women with ovarian dysfunction. Endocr. J. 2004, 51, 165-9.
55. Shoorei, H.; Seify, M.; Talebi, S. F.; Majidpoor, J.; Dehaghi, Y. K.; Shokoohi, M. Different types of bisphenols alter ovarian steroidogenesis: special attention to BPA. Heliyon 2023, 9, e16848.
56. Cipriani, S.; Maseroli, E.; Vignozzi, L. Testosterone in females. In: Mulhall JP, Maggi M, Trost L, editors. Controversies in testosterone deficiency. Springer, Cham: 2021. pp. 81-105.
57. Perović, M.; Wugalter, K.; Einstein, G. Review of the effects of polycystic ovary syndrome on Cognition: looking beyond the androgen hypothesis. Front. Neuroendocrinol. 2022, 67, 101038.
58. Buck, G. M.; Sever, L. E.; Batt, R. E.; Mendola, P. Life-style factors and female infertility. Epidemiology 1997, 8, 435-41.
59. Hannon, P. R.; Flaws, J. A. The effects of phthalates on the ovary. Front. Endocrinol. 2015, 6, 8.
60. Zhang, T.; Li, L.; Qin, X. S.; et al. Di-(2-ethylhexyl) phthalate and bisphenol A exposure impairs mouse primordial follicle assembly in vitro. Environ. Mol. Mutagen. 2014, 55, 343-53.
61. Land, K. L.; Lane, M. E.; Fugate, A. C.; Hannon, P. R. Ovulation is inhibited by an environmentally relevant phthalate mixture in mouse antral follicles in vitro. Toxicol. Sci. 2021, 179, 195-205.
62. Panagopoulos, P.; Mavrogianni, D.; Christodoulaki, C.; et al. Effects of endocrine disrupting compounds on female fertility. Best. Pract. Res. Clin. Obstet. Gynaecol. 2023, 88, 102347.
63. Emori, M. M.; Drapkin, R. The hormonal composition of follicular fluid and its implications for ovarian cancer pathogenesis. Reprod. Biol. Endocrinol. 2014, 12, 60.
64. Ara, C.; Asmatullah,
65. Lovekamp-Swan, T.; Davis, B. J. Mechanisms of phthalate ester toxicity in the female reproductive system. Environ. Health. Perspect. 2003, 111, 139-45.
66. Basak, S.; Varma, S.; Duttaroy, A. K. Modulation of fetoplacental growth, development and reproductive function by endocrine disrupters. Front. Endocrinol. 2023, 14, 1215353.
67. Téteau, O.; Vitorino Carvalho, A.; Papillier, P.; et al. Bisphenol A and bisphenol S both disrupt ovine granulosa cell steroidogenesis but through different molecular pathways. J. Ovarian. Res. 2023, 16, 30.
Cite This Article

How to Cite
Download Citation
Export Citation File:
Type of Import
Tips on Downloading Citation
Citation Manager File Format
Type of Import
Direct Import: When the Direct Import option is selected (the default state), a dialogue box will give you the option to Save or Open the downloaded citation data. Choosing Open will either launch your citation manager or give you a choice of applications with which to use the metadata. The Save option saves the file locally for later use.
Indirect Import: When the Indirect Import option is selected, the metadata is displayed and may be copied and pasted as needed.
About This Article
Special Issue
Copyright
Data & Comments
Data
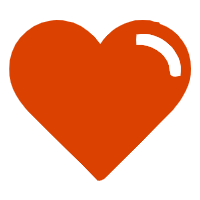
Comments
Comments must be written in English. Spam, offensive content, impersonation, and private information will not be permitted. If any comment is reported and identified as inappropriate content by OAE staff, the comment will be removed without notice. If you have any queries or need any help, please contact us at [email protected].