Chemical ingredients in personal care products and their hormetic effects on freshwater photobacteria
Abstract
Personal care products (PCPs), including skin lotions, toners, and make-up waters, are ubiquitous in daily life. Prior research has demonstrated hormesis, a biphasic dose-response phenomenon, in these PCPs on the freshwater photobacterium Vibrio qinghaiensis sp. -Q67 (Q67). Given that PCPs contain various active chemical components, their hormetic effects are intricately linked to these ingredients. However, studies focusing on the concentration-response relationships of individual PCP chemical ingredients are scarce. Building upon our previous work, we analyzed the ingredients of 6 PCPs and employed Q67 as a model organism to investigate the concentration-response relationships of these ingredients. Our analysis of 6 representative PCPs revealed 49 chemical ingredients, categorized into 10 moisturizers, 5 preservatives, 5 emulsifiers, 5 emollients, 20 ingredients with known efficacy, and 4 with unknown efficacy. Among these 49 chemical ingredients, 30 water-soluble ones were suitable for toxicity testing. At 0.25 h, 23 chemical ingredients exhibited S-shaped concentration-response curves (CRCs) with EC50 values spanning from 6.943E-5 g/mL [lactic acid (LAA)] to 1.032 g/mL [sorbitol (SBO)], while 2 [PEG-7 glyceryl cocoate (PGC) and Tween 80 (TW80)] showed hormesis with J-shaped CRCs, and 5 [adenosine (ADE), dexpanthenol (DEX), E4C, E6C, serine (SER)] lacked discernible CRCs. At 12 h, 13 chemical ingredients displayed S-shaped CRCs with EC50 values ranging from 4.402E-5 g/mL (E6C) to 1.961E-1 g/mL [dicaprylyl carbonate (DIC)], while 16 showed J-shaped CRCs, with EC50, zero effective concentration point (ZEP), ECmin, and Emin values indicating a consistent variation range spanning 4 orders of magnitude. These findings underscore the importance of considering individual chemical ingredients in assessing the risks associated with PCPs.
Keywords
INTRODUCTION
Personal care products (PCPs) are a significant subset of pharmaceuticals and personal care products (PPCPs), yet there is no clear classification, leading to confusion with drugs and cosmetics. PCPs refer to household items for health, beauty, and hygiene, such as disinfectants, soaps, cosmetics, perfumes, insect repellents, preservatives, and sunscreens[1]. The U.S. FDA classifies some PCPs, such as skin moisturizers and shampoos, as cosmetics, while others with therapeutic effects, such as acne treatments or mouthwashes, are classified as drugs. According to the Federal Food, Drug, and Cosmetic Act, cosmetics are for beautifying or cleansing (e.g., shampoo, lipstick), while drugs treat or prevent diseases or affect body functions (e.g., sunscreens, acne creams) (FDA). Some products, like moisturizing sunscreens, may fall under both categories. The term “cosmetic” is sometimes used broadly for PCPs[2]. Daily-use PCPs, including shampoos, facial cleansers, toners, lipsticks, sunscreens, and body lotions, are applied to the skin or mucosal surfaces, leading to systemic exposure. Surveys show people use an average of 9 different PCPs daily, with 1/4 of women using 15 or more[3].
PCPs are complex mixtures of various chemicals and plant extracts. Consumers are primarily exposed to PCPs through application methods such as smearing, wiping, and spraying, which increase direct contact with active ingredients. Activities like massaging further enhance exposure by increasing the frequency and likelihood of absorption through multiple pathways. Components of PCPs can penetrate through epidermal cells and have been detected in human urine, serum, and other body fluids. For instance, Li et al. detected 11 common PCP components in urine samples from 941 pregnant women, with 66% showing detectable levels of parabens, benzophenones, and triclosan[4]. Seasonal differences, such as higher benzophenone levels in summer, reflect increased sunscreen use[4]. Parabens act as preservatives, benzophenones as sunscreens, and triclosan as an antibacterial agent. Several studies have shown that the detected concentration of PCP chemical ingredients in urine and serum correlates with the frequency of PCP use, ranging from ng/L to µg/L[5-7]. PCP components have been detected in environmental matrices, such as river water, surface water, soil, and groundwater, mainly through daily washing and discharge from swimming pools or wastewater treatment plants[8-12]. They have even been found in remote locations such as Antarctica’s natural water bodies[13]. Montes-Grajales et al. analyzed the presence of 72 PCP ingredients in aquatic substrates from 30 countries, finding that fragrances, preservatives, and sunscreens were most frequently detected[14]. High detection concentrations included musk, triclosan, and acetophenone in surface water, groundwater, and wastewater, respectively.
The increasing detection of PCP ingredients in environmental matrices highlights their toxic effects on humans, animals, and aquatic organisms. For example, triclosan is known for its endocrine-disrupting effects on reproductive and thyroid systems and causes acute toxicity in aquatic life, particularly algae[15-17]. Benzophenone UV filters are commonly added to PCPs at concentrations of 5%-10% w/w due to their effective sun protection and relatively low production costs[18]. However, their lipophilic nature leads to strong bioaccumulation in organisms and various endocrine-disrupting effects[19,20]. Moreover, these compounds can increase the rate of malignant cell transformation, posing significant health risks[21,22]. In addition to everyday applications, individuals are commonly exposed to PCP chemical ingredients through inhalation and dietary consumption. Zhu et al. conducted research on parabens, triclosan, triclocarban, bisphenol A, and its analogs in 289 indoor dust samples collected across various geographical locations in China[23]. The study assessed human exposure to these chemicals via ingestion of indoor dust and dermal absorption. The findings revealed that the median and 95th percentile estimated daily intakes (EDI) of these chemicals ranged from 0.439 ng/kg bw/day for adults to 4.57 ng/kg bw/day for infants, and from 6.26 ng/kg bw/day for adults to 62.1 ng/kg bw/day for infants, respectively, generally decreasing with age. Another study demonstrated that the EDIs of antioxidants for preschoolers, school-aged children, adults, and the elderly, through dietary intake, were 22,200, 9,970, 7,540, and 7,700 ng/kg bw/day, respectively[24]. The EDIs indicated that the exposure risks associated with antioxidants decreased with age, with preschoolers facing the highest exposure risk. Notably, fetuses can also be exposed to these PCP chemicals through the placental transfer of nutrients from their mothers[25].
Our initial analysis of 24 PCPs showed that 18 products exhibited significant hormesis effects when tested with the freshwater photobacteria Vibrio qinghaiensis sp. -Q67 (Q67), characterized by low-concentration stimulation and high-concentration inhibition after long-term exposure, while 6 did not show this pattern. These findings suggest the need for systematic analysis of PCP ingredient information and dose-response relationships. This study will focus on 6 representative PCPs that exhibit hormesis effects, analyzing their components and toxicity using Q67. The outcomes aim to provide scientific guidance for the risk assessment and regulatory control of PCPs and their chemical ingredients.
MATERIALS AND METHODS
Chemical ingredients analysis of six representative PCPs
The selection of the six typical PCPs was based on their performance in the 12-hour maximum stimulus effect indicators, as described in our earlier study[26]. These six products were chosen to represent a broad range of effects observed in the study. Specifically, the top three products - skin water S6, S5, and toner T5 - were selected for their high stimulus effects. Additionally, skin water S2 was chosen as it ranked lowest in the 12-hour maximum stimulus effect indicators. We also included make-up water M3, which was the only make-up water to show a stimulus effect within 12 h, and make-up water M2, which exhibited an inhibitory effect at 12 h but a slight stimulus effect at 0.25 h. These selections allow us to capture a range of PCPs with varying hormetic effects, from short-term to long-term responses, and with maximum stimulus effects that vary from low to high under prolonged exposure. Shanghai Microspectral Chemical Co., Ltd. conducted the component detection and analysis for these 6 products. It is notable that the data for S5 were sourced from our previous study[27]. The detection of PCP ingredients was carried out using several advanced instruments under specific conditions. The Fourier transform infrared spectrometer (FTIR) used was a Thermo Nicolet iS10, with samples prepared by application and tested at 25 °C. The instrument conducted 16 scans within a spectral range of 4,000-350 cm-1. The BRUKER nuclear magnetic resonance (NMR) instrument operated at 400 MHz with an automatic 60-position sampler, utilizing D2O as a co-solvent; samples were diluted and directly injected for testing. Gas chromatography-mass spectrometry (GC-MS) was performed using an Agilent 7890B-5977A, featuring a mass range of 1.6-1,050 amu and a sensitivity of 1 pg Octafluoronaphthalene. Under electron ionization (EI) conditions, the signal-to-noise ratio (S/N) was greater than 200:1. Samples were extracted with methanol and directly injected. Liquid chromatography-mass spectrometry (LC-MS) utilized a WATERS SQD2 with a detection limit of 50 ppb and a molecular weight scanning range of 50-2,000, suitable for qualitative and quantitative analysis of organic compounds and separation of complex components; samples were diluted and directly injected. The inductively coupled plasma emission spectrometer (ICP) used was an Optima 8000, and the testing method involved wet digestion of the sample with 6 mL of nitric acid and 2 mL of hydrogen peroxide. Lastly, the X-ray fluorescence spectrometer (XRF) was a Panaco AxiosMAX, capable of detecting elements from carbon to uranium (C-U), operating with a light tube power of 4,000 W and a single-channel scanning detector.
Reagents
With the assistance of Shanghai Microspectral Chemical Co., Ltd., the chemical ingredients in the PCPs were analyzed. The analysis conducted was primarily non-targeted, and the concentrations of the chemical ingredients in the PCPs were not quantified. The identified chemicals were subsequently purchased and utilized in the toxicity testing for this study. Ascorbic acid 2-glucoside (AAG), ascorbic acid (ASA), 1,3-butanediol (BUT), citric acid (CIA), dexpanthenol (DEX), lactic acid (LAA), linalool (LIO), 1,2-propanediol (PDO), polyethylene glycol 400 (PEG400), proline (PRO), sorbitol (SBO), and Tween 80 (TW80) were sourced from Tokyo Chemical Industry. Adenosine (ADE), betaine (BET), 1,2-octanediol (ODO), 2-phenoxyethanol (POE), serine (SER), sodium citrate (SOC), and triethanolamine (TEA) were procured from Swiss Adamas Reagent Company. Benzoic acid C12-15-alkyl esters (BEN) originated from Guangzhou Donglin Chemical Co., Ltd. Coco-glucoside (COG) and decyl glucoside (DEG) were acquired from Shanghai Fakai Chemical Co., Ltd. Dicaprylyl carbonate (DIC) was purchased from Guangzhou Keyou Chemical Co., Ltd. Ethoxylated hydrogenated castor oil (E4C) and PEG-7 glyceryl cocoate (PGC) were obtained from BASF SE. Glycerol (GLO) was sourced from Sinopharm Chemical Reagent Co., Ltd. 2-Propanol (IPO) was purchased from Merck Sigma-Aldrich. Isothiazolinone (ITZ) was acquired from Shanghai McLean Biochemical Technology Co., Ltd. Lastly, α-iso-methylionone (MET) was procured from Shanghai Huake Experimental Equipment Co., Ltd. A comprehensive summary of these 30 components, including abbreviations, names, structural formulas, CAS numbers, purity specifications, molecular weights, and the respective purchase channels is presented in Table 1 and Supplementary Figure 1.
Selected physicochemical properties of 30 chemical ingredients in six PCPs
Abbr. | Chemical ingredients | CAS | Purity | M.W. (g/mol) | Water solubility (g/L)a |
AAG | Ascorbic acid 2-glucoside | 129499-78-1 | 97% | 338.26 | 879 (25 °C) |
ADE | Adenosine | 58-61-7 | 98% | 154.25 | Soluble |
ASA | Ascorbic acid | 50-81-7 | 99% | 176.12 | 50 (20 °C) |
BEN | Benzoic acid C12-15-alkyl esters | 68411-27-8 | 99% | 290.45 | 2.47-174,000 μg/L (20-30 °C) |
BET | Betaine | 107-43-7 | 98% | 117.15 | 1,600 |
BUT | 1,3-Butanediol | 107-88-0 | 99% | 90.12 | > 500 |
CIA | Citric acid | 77-92-9 | 98% | 192.14 | 1,809 (30 °C) |
COG | Coco-glucoside | NA | 99% | NA | NA |
DEG | Decyl glucoside | 141464-42-8 | 50% | 320.42 | NA |
DEX | Dexpanthenol | 81-13-0 | 98% | 205.25 | Soluble |
DIC | Dicaprylyl carbonate | 1680-31-5 | 50% | 286.45 | NA |
E4C | Ethoxylated hydrogenated castor oil | 61788-85-0 | 99% | NA | 0.0005 (20 °C) |
E6C | Ethoxylated hydrogenated castor oil | 61788-85-0 | CP | NA | 0.0005 (20 °C) |
GLO | Glycerol | 56-81-5 | 99% | 92.09 | > 500 (20 °C) |
IPO | 2-Propanol | 67-63-0 | 99.5% | 60.1 | Miscible |
ITZ | Isothiazolinone | 55965-84-9 | 14% in H2O | 264.742 | NA |
LAA | Lactic acid | 50-21-5 | 85% | 90.08 | Soluble |
LIO | Linalool | 78-70-6 | 98% | 154.25 | 1.45 (25 °C) |
MET | α-iso-Methylionone | 127-51-5 | 60%-70% | 206.32 | 0.028 (25°C) |
ODO | 1,2-Octanediol | 1117-86-8 | 98% | 146.23 | 3 (20 °C) |
PDO | 1,2-Propanediol | 57-55-6 | 99% | 76.09 | Miscible |
PEG400 | Polyethylene glycol 400 | 25322-68-3 | CP | 380-420 | 50 |
PGC | PEG-7 glyceryl cocoate | 68201-46-7 | 99% | NA | NA |
POE | 2-Phenoxyethanol | 122-99-6 | 98% | 138.16 | 30 (20 °C) |
PRO | Proline | 29795-82-2 | 98% | 115.13 | > 1,000 (20 °C) |
SBO | Sorbitol | 50-70-4 | 97% | 182.17 | Soluble |
SER | Serine | 302-84-1 | 98% | 105.09 | 50.23 (25 °C) |
SOC | Sodium citrate | 6132-04-3 | 99% | 294.10 | 720 (25 °C) |
TEA | Triethanolamine | 102-71-6 | 99% | 149.19 | Soluble |
TW80 | Tween 80 | 9005-65-6 | CP | NA | 50-100 (23 °C) |
Q67 toxicity test
The detail of Q67 medium is presented in the Supplementary Materials (Q67 medium). The short-term/long-term microplate toxicity analysis (S/L-MTA) method was employed to assess luminescence inhibition rates of pollutants on Q67 using a 96-well opaque white microplate. This approach, based on improvements by Zhu et al., measured acute (0.25 h) and long-term (12 h) luminescence inhibition rates[28]. To minimize edge effects, 200 μL of ultrapure water was added to the surrounding 36 wells. The distribution of blanks and the 12 concentration gradients on the 96-well microplate is shown in Supplementary Figure 2. A total of 24 wells in columns 2, 6, 7, and 11 were selected as blank controls, while the remaining 36 wells were designed with 12 concentration gradients based on a specific dilution factor[29]. Each concentration gradient included 3 replicates, and 100 μL of bacterial solution was added to each well, resulting in a total volume of 200 μL per well. The experiment was repeated on three plates. The relative luminescence units (RLU) were measured at 0.25 and 12 h using the Power-Wave microplate spectrophotometer (American BIOTEK company) at 22 ± 1 °C.
Concentration-response curve fitting
Calculate the relative luminescence inhibition rate E% for the 12 concentration points across the 3 boards at 0.25 h and 12 h using the formula [Equation (1)]:
where RLU0 represents the average RLU of the control group, while RLU denotes the RLU of the experimental group.
For the monotonic S-shaped nonlinear concentration-effect relationship, the nonlinear Hill function [Equation (2)] is selected for optimal nonlinear regression. In experimental observations, lower toxicity effects are associated with higher measurement uncertainty. Consequently, the 95% observed confidence interval (OCI) is chosen to reasonably characterize the fitting and observational uncertainties.
where Hup is the Hill index, Em represents the effect value at a compound concentration of zero, Emax indicates the effect value corresponding to a sufficiently high compound concentration, and ECup denotes the compound concentration associated with the effect calculated using Equation (3).
For compounds exhibiting a hormetic effect, their concentration-response curve (CRC) is J-shaped. In JSFit, a seven-parameter model [Equation (6)] is employed to fit this J-shaped CRC by combining two Hill functions [Equations (4 and 5)], and the 95%OCI is calculated.
where E denotes the inhibitory effect, C represents the concentration, E0 is the maximum effect value of the Hdown function [Equation (6)], Em is the minimum effect value in both Equations (4 and 5), Emax signifies the maximum effect value of the Hup function, and ECdown corresponds to the concentration at which the effect is (E0 + Em)/2 in the Hdown function. Hdown is the Hill index for the descending segment (or stimulation interval), Hup is the Hill index for the ascending segment (or inhibition interval), and ECup is the concentration at which the effect is (Em + Emax)/2 in the Hup function.
For non-monotonic J-shaped CRCs that include both stimulation and inhibition phases, this study identifies EC50, zero effective concentration point (ZEP), ECmin, and Emin as key characteristic parameters of hormesis. Here, EC50 represents the concentration causing half of the maximum effect, ZEP is the zero-effect concentration point, ECmin indicates the concentration at which the maximum stimulation effect occurs, and Emin refers to the minimum inhibitory effect or the maximum stimulation effect on the dose-effect curve[26,27,30].
RESULTS AND DISCUSSION
Summary of PCP ingredients detection
The analysis of 6 PCP products revealed a total of 49 components, encompassing moisturizers, preservatives, emulsifiers, and softeners. Specifically, the products include 10 moisturizers (such as GLO, BET, BUT, PRO, SER, SBO, DEX, LAA, trehalose, and propanediol), 5 preservatives (including POE, ITZ, chlorpheniramine, hydroxybenzyl ester, and hydroxybenzyl ethyl ester), 5 emulsifiers (like PEG-7 glycerol cocoate, vitamin E acetate, oil alcohol polyether-20, ethoxylated hydrogenated castor oil, and oil alcohol polyether), and 5 softeners (such as ODO, polyethylene glycol-8, BEN, DIC, propionine cysteine). Additionally, there are 3 pH regulators (SOC, TEA, and CIA), 3 odor regulators (LIO, essence, and iso-methylionone), 2 anti-inflammatory agents (salicylic acid and allantoin), 2 cleaning agents (DEG and COG), 2 thickening agents (dextrin and carbomer), 2 whitening agents (octyl salicylic acid and AAG), 2 solubilizers (ethoxylated hydrogenated castor oil and IPO), a sunscreen agent (benzyl salicylate), an antioxidant (ASA), a chelating agent (disodium ethylenediaminetetraacetic acid), a conditioner (gold), and 4 other unidentified components (silicon dioxide, magnesium silicate, polysorbate 80, and ADE). The concentrations of these chemicals were not quantified.
PCPs, including make-up waters, skin waters, and toners, typically contain humectants, detergents, emulsifiers, and preservatives. Preservatives, such as hydroxybenzyl methyl ester and hydroxybenzyl ethyl ester, inhibit microbial growth and extend product shelf life by disrupting cell membranes, denaturing proteins, and suppressing enzyme activity[31,32]. ITZ, another preservative, can cause allergic reactions[33], while methylparaben, an endocrine disruptor, has potential reproductive toxicity, including risks of breast cancer and premature development[34,35]. Humectants, substances that retain moisture on the skin, encompass a diverse range of alcohols, amino acids, and polysaccharides, and are frequently employed in cosmetic formulations. Emulsifiers, vital for stabilizing blends of incompatible substances, are ubiquitous in cleaning and cosmetic products, existing in both natural and synthetic forms. Surfactants, categorized into anionic, cationic, and zwitterionic types, have the potential to influence drug accumulation in invertebrates and modify protein structures, with their effects intimately tied to their concentration levels[36,37].
The ingredients listed on the packaging of PCPs and those detected through analysis are summarized in Supplementary Table 1. There is a notable discrepancy between the listed ingredients and those actually detected. This difference may be due to limitations in current analytical technology or because some compounds are below the detection threshold. A comparative analysis of the 6 PCPs shows the following: M3: The packaging lists 9 ingredients, 5 of which were detected, resulting in a dissimilarity of 44%, with 6 additional substances identified. M2: The packaging lists 22 ingredients, 19 of which were detected, leading to a dissimilarity of 14%, with no new substances identified. S2: The packaging lists 11 ingredients, 5 of which were detected, showing a dissimilarity of 54%, with 8 new substances detected. S5: The packaging lists 18 ingredients, 9 of which were detected, resulting in a dissimilarity of 50%, with 3 new substances detected. S6: The packaging lists 6 ingredients, with only phenoxyethanol detected, resulting in a dissimilarity of 83%, and 3 new substances identified. T5: The packaging lists 16 ingredients, 4 of which were detected, leading to a dissimilarity of 75%, with 4 new substances detected.
The degree of difference (DD) between the listed ingredients and those detected is calculated as follows:
where the packaging displays an ingredient quantity of k, and the detected ingredient with the same ingredient quantity as the packaging display is j.
In our daily lives, PCPs frequently make direct contact with the human body, and their active chemical constituents have the potential to undergo alterations over extended periods of usage. The outcomes of testing have unveiled certain uncertainties pertaining to these chemical ingredients. For instance, in S2, ITZ was detected but not listed on the packaging. ITZ and its derivatives are known for their strong antibacterial properties even at low concentrations, making them effective fungicides in various products such as household cleaners, industrial fungicides, and cosmetics. Despite their low effective use concentration, ITZ has been associated with potential health hazards for both consumers and workers[38-40]. Consequently, EU legislation restricts the use of ITZ in biocidal products, which require authorization before market release, with active substances needing prior approval (The Biocidal Products Regulation, EU 528/2012). Although ITZ has a short half-life, it has been detected in sewage and rivers at concentrations as low as ng/L[41-44]. The potential ecological risks associated with certain chemicals, exemplified by methyl ITZ, are considerable, as evidenced by its detrimental effects on zebrafish embryonic development and the manifestation of neurotoxicity[45,46].
Short-term concentration-response relationship of PCP chemical ingredients
Out of the 49 chemical ingredients analyzed, 12 are insoluble in water (including silicon dioxide, magnesium silicate, benzyl salicylate, octylsalicylic acid, vitamin E acetate, disodium ethylenediaminetetraacetate, dextrin, gold, chlorphenylene glycol, methyl hydroxyphenyl ester, ethyl hydroxyphenyl ester, and allantoin). Additionally, the type of one ingredient (essence) remains unclear, and stable experimental data for 6 chemical ingredients (trehalose, oil alcohol polyether-20, oleyl polyether, carbomer, alanine, and salicylic acid) could not be obtained in subsequent experiments. Consequently, the concentration-response relationships of 30 chemical ingredients were acquired. It is notable that the data for ASA, BET, GLO, ODO, PEG400, and POE were sourced from our previous study[27].
At 0.25 h, 23 of the 30 chemical ingredients displayed an S-shaped CRC. Hormesis was observed in 2 chemical ingredients (PGC and TW80), which exhibited a J-shaped CRC. Five components (ADE, DEX, E4C, E6C, and SER) did not show a CRC. The CRCs of these chemical ingredients on Q67 at 0.25 h are illustrated in Figures 1 and 2. The fitting model parameters (Em, Emax, ECup, and Hup), statistical parameters [coefficient of determination (R2) and root mean square error (RMSE)], EC50, and its 95%OCI are listed in Supplementary Table 2. The R2 and RMSE values suggest that the Hill function effectively describes CRC. At 0.25 h, of the 30 PCP chemical ingredients tested, 25 displayed concentration-response relationships, with their EC50 values ranging from 6.943E-5 g/mL (LAA) to 1.032 g/mL (SBO). With the inclusion of 95%OCI, it is evident that LAA exhibits the highest luminescence inhibition toxicity on Q67 in short-term exposure, while SBO has the lowest, showing a difference of 5 orders of magnitude between the two.
Figure 1. The concentration-response relationship of 11 PCP chemical ingredients at 0.25 h (○: 0.25 h experimental value; -: the fitted CRC; ---: 95%OCI). Data for ASA, GLO, and PEG400 were sourced from our previous study[27]. PCP: Personal care product; CRC: concentration-response curve; OCI: observed confidence interval; ASA: ascorbic acid; GLO: glycerol; PEG400: polyethylene glycol 400.
Figure 2. The concentration-response relationship of 14 PCP chemical ingredients at 0.25 h (○: 0.25 h experimental value; -: the fitted CRC; ---: 95%OCI). Data for BET, ODO, and POE were sourced from our previous study[27]. PCP: Personal care product; CRC: concentration-response curve; OCI: observed confidence interval; BET: betaine; ODO: 1,2-octanediol; POE: 2-phenoxyethanol.
Only limited data on the safety of these chemical ingredients were available. Dermal application of PGC at a concentration of 50% did not cause irritation in mice and guinea pigs, but it did result in slight irritation in rabbits[47]. The GLO analogs induced morphological changes in Trypanosoma brucei, including detached flagella, cytokinesis defects, and the “big-eye” phenotype. Additionally, the compounds demonstrated primarily an antagonistic interaction with salicylhydroxamic acid, rather than a synergistic effect[48]. Yi et al. suggested that PDO protects against total body irradiation (TBI)-induced hematopoietic injury by enhancing the activities of antioxidant enzymes and inhibiting apoptosis in HSCs[49]. Additionally, PDO increased serum levels of granulocyte-colony stimulating factor and interleukin-6, regardless of TBI. Overall, the study concluded that PDO serves as a radioprotector, providing protection against radiation-induced hematopoietic injury[49].
Long-term concentration-response relationship of PCP chemical ingredients
It is notable that the data for ASA, BET, E4C, GLO, ODO, PEG400, and POE were sourced from our previous study[27]. At 12 h, 13 PCP chemical ingredients displayed S-shaped CRC [Figure 3], 16 chemical ingredients showed J-shaped CRC [Figure 4], and 1 chemical ingredient (TW80) had no CRC. The fitting parameters (Em, Emax, ECup, Hup), statistics (R2, RMSE), EC50, and 95%OCI for the 13 chemical ingredients with S-shaped CRC are detailed in Table 2. For the 16 chemical ingredients with J-shaped CRC, the fitting parameters (Em, ECdown, Hdown, Emax, ECup, Hup) and statistics are summarized in Supplementary Table 3. The EC50 of the 13 components ranges from 4.402E-5 g/mL (E6C) to 1.961E-1 g/mL (DIC), indicating that E6C has the highest toxicity on Q67 luminescence inhibition at 12 h, while DIC has the lowest, differing by 4 orders of magnitude. For the 16 chemical ingredients with hormesis, the parameters [Table 3] range from an EC50 of 8.070E-5 g/mL (LAA) to 7.594E-1 g/mL (SBO), ZEP of 4.189E-5 g/mL (PGC) to 5.700E-1 g/mL (SBO), ECmin of 1.679E-5 g/mL (E4C) to 2.042E-1 g/mL (SBO), and Emin of -804.4% (BET) to -24.0% (BEN), all spanning 4 orders of magnitude. The fitting parameters for the J-shaped CRC models of PGC and TW80 at 0.25 h are presented in Table 3. For the hormesis characteristic parameters, PGC has an EC50 of 2.696E-3 g/mL, ZEP of 2.008E-4 g/mL, ECmin of 1.718E-5 g/mL, and Emin of -38.5%. TW80 has an EC50 of 1.895E-1 g/mL, ZEP of 1.408E-1 g/mL, ECmin of 2.723E-2 g/mL, and Emin of -75.1%. LAA consistently shows the highest luminescence inhibition toxicity to Q67 under both short-term (0.25 h) and long-term (12 h) exposure, while SBO shows the least toxicity across both timeframes.
Figure 3. The concentration-response relationship of 13 PCP chemical ingredients at 12 h (•: 12 h experimental value; -: the fitted CRC;
Figure 4. The concentration-response relationship of 16 PCP chemical ingredients at 12 h (•: 12 h experimental value; -: the fitted CRC;
Thirteen chemical ingredients were fitted with S-shaped CRC parameters (Em, Emax, ECup, and Hup) and statistical measures
Chemical ingredient | Em | Emax | ECup | Hup | R2 | RMSE | EC50 (g/mL) | OCIleft (g/mL) | OCIright (g/mL) |
ASA | 0.121 | 1.013 | 1.720E-3 | 7.423 | 0.9928 | 0.03719 | 1.648E-3 | 1.490E-3 | 1.800E-3 |
BUT | -0.059 | 1.056 | 1.813E-2 | 1.957 | 0.9971 | 0.02289 | 1.066E-2 | 1.550E-2 | 2.145E-2 |
CIA | -0.047 | 1.007 | 1.400E-4 | 1.578 | 0.9906 | 0.03409 | 1.411E-4 | 1.024E-4 | 1.931E-4 |
DEX | 0.216 | 0.985 | 3.820E-3 | 5.296 | 0.9909 | 0.04097 | 3.458E-3 | 2.756E-3 | 4.130E-3 |
DIC | 0.102 | 1.178 | 2.452E-1 | 2.385 | 0.9913 | 0.03564 | 1.961E-1 | 1.522E-1 | 2.464E-1 |
E6C | 0.008 | 0.937 | 4.000E-5 | 1.283 | 0.996 | 0.02593 | 4.402E-5 | 3.155E-5 | 6.167E-5 |
GLO | -0.265 | 1.026 | 6.470E-3 | 1.116 | 0.9938 | 0.0297 | 9.043E-3 | 6.540E-3 | 1.257E-2 |
MET | 0.119 | 1.088 | 3.712E-2 | 2.905 | 0.9909 | 0.0393 | 3.197E-2 | 2.456E-2 | 4.023E-2 |
PDO | 0.000 | 1.025 | 1.700E-1 | 1.765 | 0.9983 | 0.01561 | 1.653E-1 | 1.456E-1 | 1.882E-1 |
PEG400 | 0.016 | 1.104 | 3.552E-2 | 2.418 | 0.9918 | 0.04145 | 3.243E-2 | 2.474E-2 | 4.214E2 |
PRO | 0.017 | 0.952 | 8.500E-4 | 1.948 | 0.9977 | 0.00815 | 8.810E-4 | - | 9.506E-4 |
SOC | -3.069 | 2.572 | 2.000E-5 | 0.106 | 0.9933 | 0.02137 | 3.099E-3 | 1.804E-3 | 5.350E-3 |
TEA | 0.023 | 1.005 | 6.580E-3 | 3.581 | 0.9985 | 0.01529 | 6.476E-3 | 6.013E-3 | 6.952E-3 |
Four hormesis characteristic parameters (EC50, ZEP, ECmin, and Emin) and the corresponding 95%OCI of chemical ingredients for Q67a
Chemical ingredient | Time (h) | EC50 (g/mL) | OCIleft (g/mL) | OCIright (g/mL) | ZEP (g/mL) | OCIleft (g/mL) | OCIright (g/mL) | ECmin (g/mL) | OCIleft (g/mL) | OCIright (g/mL) | Emin | OCIup | OCIdown |
AAG | 12 | 4.582E-3 | 4.397E-3 | 4.902E-3 | 4.289E-3 | 4.145E-3 | 4.456E-3 | 3.162E-3 | 2.131E-3 | 3.805E-3 | -124.5% | -148.8% | -100.1% |
ADE | 12 | 2.134E-2 | 1.546E-2 | 9.999E+00 | 1.475E-2 | 1.050E-2 | - | 1.096E-3 | 5.658E-4 | - | -243.0% | -287.2% | -198.8% |
BEN | 12 | 1.009E-1 | 7.842E-2 | 1.340E-1 | 3.836E-2 | 3.208E-2 | 4.600E-2 | 2.265E-2 | 1.577E-2 | 3.106E-2 | -24.0% | -35.0% | -12.9% |
BET | 12 | 1.657E-1 | 1.350E-1 | 2.385E-1 | 1.285E-1 | 1.124E-1 | 1.528E-1 | 2.818E-2 | 2.252E-2 | 3.496E-2 | -804.4% | -847.7% | -761.1% |
COG | 12 | 2.527E-4 | 2.178E-4 | 3.174E-4 | 1.796E-4 | 1.668E-4 | 1.966E-4 | 1.047E-4 | 1.021E-4 | 1.104E-4 | -203.7% | -220.9% | -186.6% |
DEG | 12 | 4.264E-4 | 3.285E-4 | 5.852E-4 | 2.161E-4 | 1.820E-4 | 2.622E-4 | 1.303E-4 | 1.217E-4 | 1.481E-4 | -52.1% | -68.7% | -35.5% |
E4C | 12 | 5.331E-4 | 4.201E-4 | 7.175E-4 | 1.792E-4 | 1.399E-4 | 2.212E-4 | 1.679E-5 | 1.299E-5 | 7.710E-5 | -32.9% | -42.8% | -23.0% |
IPO | 12 | 9.081E-3 | 6.275E-3 | 1.462E-2 | 4.336E-3 | 2.843E-3 | 6.186E-3 | 3.055E-4 | 2.372E-4 | 2.019E-3 | -63.6% | -89.8% | -37.3% |
ITZ | 12 | 1.152E-4 | 1.061E-4 | 1.284E-4 | 9.811E-5 | 9.346E-5 | 1.039E-4 | 7.674E-5 | 6.394E-5 | 8.670E-5 | -71.1% | -91.7% | -50.4% |
LAA | 12 | 8.070E-5 | 7.509E-5 | 8.851E-5 | 7.078E-5 | 6.589E-5 | 7.562E-5 | 5.188E-5 | 4.320E-5 | 6.169E-5 | -74.8% | -102.7% | -46.8% |
LIO | 12 | 1.634E-3 | 1.467E-3 | 1.862E-3 | 1.272E-3 | 1.173E-3 | 1.385E-3 | 6.166E-4 | 3.664E-4 | 8.689E-4 | -93.7% | -110.5% | -77.0% |
ODO | 12 | 3.990E-4 | 3.374E-4 | 4.836E-4 | 2.164E-4 | 1.833E-4 | 2.532E-4 | 7.079E-5 | 4.183E-5 | 1.175E-4 | -56.4% | -70.0% | -42.8% |
PGC | 0.25 | 2.696E-3 | 1.504E-3 | 4.729E-3 | 2.008E-4 | 9.682E-5 | 3.909E-4 | 1.718E-5 | 1.640E-5 | 1.672E-5 | -38.5% | -51.5% | -25.6% |
PGC | 12 | 1.269E-4 | 8.746E-5 | 2.038E-4 | 4.189E-5 | 3.244E-5 | 5.467E-5 | 1.995E-5 | 1.699E-5 | 1.861E-5 | -42.0% | -57.2% | -26.9% |
POE | 12 | 9.418E-4 | 7.948E-4 | 1.133E-3 | 4.383E-4 | 3.715E-4 | 5.141E-4 | 1.288E-4 | 2.746E-5 | 2.332E-4 | -45.6% | -56.9% | -34.3% |
SBO | 12 | 7.594E-1 | 7.033E-1 | 8.272E-1 | 5.700E-1 | 5.451E-1 | 5.990E-1 | 2.042E-1 | 1.818E-1 | 2.314E-1 | -374.0% | -385.8% | -362.1% |
SER | 12 | 2.571E-1 | 2.357E-1 | 2.920E-1 | 2.090E-1 | 2.018E-1 | 2.184E-1 | 1.380E-1 | 1.132E-1 | 1.618E-1 | -121.1% | -134.2% | -108.1% |
TW80 | 0.25 | 1.895E-1 | 1.728E-1 | 2.308E-1 | 1.408E-1 | 1.262E-1 | 1.556E-1 | 2.723E-2 | 8.237E-3 | 6.301E-2 | -75.1% | -87.7% | -62.5% |
While LAA demonstrates the highest luminescence inhibition toxicity in short-term exposure, it exhibits significant hormesis in long-term exposure. For instance, at the concentration with the maximum observed effect, LAA inhibits luminescence by 25.2% at 0.25 h but stimulates it by -74.2% at 12 h. The underlying reasons and mechanisms behind this phenomenon warrant further investigation. However, we hypothesize that it may be related to the growth characteristics of Q67. At low concentrations, short-term environmental adaptation appears to induce inhibitory effects on the luminescence of Q67. Following this adaptation, low concentrations of LAA may be perceived as more beneficial by Q67, leading to the stimulation of luminescence during the growth phase. In terms of environmental risks, this transition suggests that low concentrations of LAA could have detrimental effects, such as suppressing microbial luminescence, which serves as a marker for cellular activity. However, as the exposure level increases or as organisms adapt, these same low concentrations may stimulate cellular processes, potentially indicating an adaptive response or altered metabolic pathways in the exposed organisms. In line with previous findings, BET and POE also exhibited time-dependent hormesis, with characteristic parameters indicating that the absolute value of the maximum stimulatory effect generally increased with time[50]. Additionally, in a prior study, eleven PCP compounds that showed stimulatory effects on Vibrio fischeri at low concentration doses (ranging from 0% to -20%) followed a pattern similar to U-shaped or J-shaped curves, or at least a segment of these curves[51]. This phenomenon is highly relevant to environmental toxicology, as it highlights that low-dose exposures, which are often considered benign, could still pose risks under certain conditions. Furthermore, it emphasizes the need to evaluate the full spectrum of concentrations and exposure durations in environmental risk assessments, as biological responses to pollutants like BET can be complex and nonlinear.
A comparison of short-term (0.25 h) and long-term (12 h) exposure results reveals distinct dose-response relationships among the tested chemical ingredients. Eight chemical ingredients (ASA, BUT, CIA, DIC, GLO, MET, PDO, PEG400, and PRO) consistently showed inhibitory effects on Q67 luminescence at both time points, with CRCs exhibiting a monotonic S-shape. Fourteen chemical ingredients (AAG, ADE, BEN, BET, COG, E4C, IPO, ITZ, LAA, LIO, ODO, POE, SBO, and SER) displayed S-shaped CRCs at 0.25 h but transitioned to J-shaped CRCs at 12 h, indicating significant hormesis. Two chemical ingredients (SOC and TEA) showed S-shaped CRCs at 0.25 h, indicating low-concentration stimulation and high-concentration inhibition of hormesis, while maintaining an S-shaped CRC at 12 h. DEG exhibited S-shaped CRC at 0.25 h but showed hormesis at both time points. PGC demonstrated hormesis with a J-shaped CRC at both 0.25 and 12 h, whereas TW80 exhibited no CRC at 12 h but displayed hormesis with a J-shaped CRC at 0.25 h. The reason why PGC exhibited hormesis at both time points may be linked to findings from a previous study, which reported that PGC did not show reproductive toxicity in oral toxicity tests. This lack of reproductive toxicity is likely due to the chemical structure of PGC, which suggests that it is unlikely to cause reproductive or developmental effects[47]. Two chemical ingredients (DEX and E6C) did not exhibit CRC at 0.25 h but showed S-shaped CRCs at 12 h. E4C, which showed no CRC at 0.25 h, exhibited hormesis with a J-shaped CRC at 12 h. Based on 95%OCI, the long-term toxicity of the 8 PCP chemical ingredients (BUT, CIA, DIC, GLO, PDO, PRO, SOC, and TEA) was significantly greater than their short-term toxicity, while MET had only slightly higher long-term toxicity. The toxicity of ASA and PEG400 remained unchanged between short-term and long-term exposures, with overlapping CRCs across the entire concentration range, indicating consistent inhibitory effects.
Despite being single-chain alcohols, ASA and PEG400 exhibited distinct concentration-response relationships on Q67. PDO, BUT, and GLO maintained monotonic S-shaped CRCs under both short-term and long-term exposure, consistently inhibiting Q67 luminescence. In contrast, IPO (Emin = -63.6%), ODO (Emin = -56.4%), and SBO (Emin = -374.0%) displayed significant hormesis on Q67 after 12 h, with SBO showing the second highest maximum stimulation effect. However, a study found that isopropanol (IPO) exhibited a stimulating effect on Q67 after 0.25 h exposure with an Emin of -21.6%[52]. Possible explanations for these differences include: (1) Hormesis is time-dependent and may vary with exposure duration; (2) Variability in individual bacterial cells can influence responses; (3) Zheng et al.’s study only conducted acute toxicity testing, without long-term analysis[52]; (4) The maximum stimulatory effect may be subtle, rendering hormesis less noticeable; (5) Different J-shaped CRC models can yield varied results depending on the fitting approach used. These findings highlight the complex nature of chemical ingredient effects on Q67, emphasizing the need for comprehensive evaluation across different time points and models.
The current study has several limitations that should be addressed in future research. First, we only tested two time points, which limits our understanding of how hormesis may vary over a longer duration, particularly beyond the 12-hour period. Second, the mechanisms behind both the stimulatory and inhibitory effects of hormesis were not fully explored in this study. Third, we used Q67 as the sole model organism, and it remains to be determined whether the observed hormesis effects are applicable to other aquatic organisms. Lastly, a more comprehensive risk assessment of hormesis, particularly in the context of environmental exposure, is needed in future studies to better understand the potential implications.
CONCLUSIONS
Analysis of 6 representative PCPs revealed a total of 49 components, including moisturizers, preservatives, emulsifiers, and softeners. Notably, ITZ, an endocrine disruptor and preservative, was detected despite not being listed on the packaging ingredient list, highlighting the need for further scrutiny. Among these 49 chemical ingredients, 30 water-soluble ones were deemed suitable for toxicity testing. At 0.25 h, 23 chemical ingredients showed no hormesis, 2 exhibited hormesis, and 5 did not display a dose-response curve. At 12 h, 13 chemical ingredients showed no hormesis, 16 exhibited hormesis, and 1 lacked a dose-response curve. The analysis highlights the presence of 49 PCP chemical ingredients, including unlisted endocrine disruptors like ITZ, underscoring the need for more comprehensive ingredient transparency and safety evaluations. Future research should focus on further investigating the effects of these chemical ingredients, particularly those exhibiting hormesis, and improving toxicity testing methods for better risk assessment.
DECLARATIONS
Authors’ contributions
Methodology, sample analysis, data curation, and writing - original draft: Li K
Writing - review and editing: Li XW
Conceptualization, writing - review and editing, supervision, and funding acquisition: Xu YQ
Availability of data and materials
The data supporting the findings of this study are available within this article and its Supplementary Materials. Further data are available from the corresponding authors upon reasonable request.
Financial support and sponsorship
This work was supported by the Foundation of Key Laboratory of Yangtze River Water Environment, Ministry of Education (Tongji University), China (NO. YRWEF202201).
Conflicts of interest
All authors declared that there are no conflicts of interest.
Ethical approval and consent to participate
Not applicable.
Consent for publication
Not applicable.
Copyright
© The Author(s) 2024.
Supplementary Materials
REFERENCES
1. Daughton CG, Ternes TA. Pharmaceuticals and personal care products in the environment: agents of subtle change? Environ Health Perspect 1999;107:907-38.
2. Nicolopoulou-Stamati P, Hens L, Sasco AJ. Cosmetics as endocrine disruptors: are they a health risk? Rev Endocr Metab Disord 2015;16:373-83.
3. Kessler R. More than cosmetic changes: taking stock of personal care product safety. Environ Health Perspect 2015;123:A120-7.
4. Li J, Liu W, Xia W, et al. Variations, determinants, and coexposure patterns of personal care product chemicals among Chinese pregnant women: a longitudinal study. Environ Sci Technol 2019;53:6546-55.
5. Fandiño-Del-Rio M, Matsui EC, Calafat AM, et al. Recent use of consumer and personal care products and exposures to select endocrine disrupting chemicals among urban children with asthma. J Expo Sci Environ Epidemiol 2024;34:637-46.
6. Goldberg M, Adgent MA, Stevens DR, et al. Environmental phenol exposures in 6- to 12-week-old infants: the infant feeding and early development (IFED) study. Environ Res 2024;252:119075.
7. Sdougkou K, Papazian S, Bonnefille B, et al. Longitudinal exposomics in a multiomic wellness cohort reveals distinctive and dynamic environmental chemical mixtures in blood. Environ Sci Technol 2024;58:16302-15.
8. García-Pimentel M, Campillo JA, Castaño-Ortiz JM, Llorca M, León VM. Occurrence and distribution of contaminants of legacy and emerging concern in surface waters of two Western Mediterranean coastal areas: Mar Menor Lagoon and Ebro Delta. Mar Pollut Bull 2023;187:114542.
9. Lu S, Wang J, Wang B, et al. Comprehensive profiling of the distribution, risks and priority of pharmaceuticals and personal care products: a large-scale study from rivers to coastal seas. Water Res 2023;230:119591.
10. Ghosh R, Parde D, Bhaduri S, Rajpurohit P, Behera M. Occurrence, fate, transport, and removal technologies of emerging contaminants: a review on recent advances and future perspectives. CLEAN Soil Air Water 2024;52:2300259.
11. K’oreje K, Okoth M, Van Langenhove H, Demeestere K. Occurrence and point-of-use treatment of contaminants of emerging concern in groundwater of the Nzoia River basin, Kenya. Environ Pollut 2022;297:118725.
12. Lu J, Mao H, Li H, Wang Q, Yang Z. Occurrence of and human exposure to parabens, benzophenones, benzotriazoles, triclosan and triclocarban in outdoor swimming pool water in Changsha, China. Sci Total Environ 2017;605-6:1064-9.
13. Vecchiato M, Barbaro E, Spolaor A, et al. Fragrances and PAHs in snow and seawater of Ny-Ålesund (Svalbard): local and long-range contamination. Environ Pollut 2018;242:1740-7.
14. Montes-Grajales D, Fennix-Agudelo M, Miranda-Castro W. Occurrence of personal care products as emerging chemicals of concern in water resources: a review. Sci Total Environ 2017;595:601-14.
15. Kek T, Geršak K, Virant-Klun I. Exposure to endocrine disrupting chemicals (bisphenols, parabens, and triclosan) and their associations with preterm birth in humans. Reprod Toxicol 2024;125:108580.
16. Veettil P, Nikarthil Sidhick J, Kavungal Abdulkhader S, Ms SP, Kumari Chidambaran C. Triclosan, an antimicrobial drug, induced reproductive impairment in the freshwater fish, Anabas testudineus (Bloch, 1792). Toxicol Ind Health 2024;40:254-71.
17. Wimmerova L, Solcova O, Spacilova M, Cehajic N, Krejcikova S, Marsik P. Toxicity assessment and treatment options of diclofenac and triclosan dissolved in water. Toxics 2022;10:422.
18. Fent K, Zenker A, Rapp M. Widespread occurrence of estrogenic UV-filters in aquatic ecosystems in Switzerland. Environ Pollut 2010;158:1817-24.
19. Carstensen L, Beil S, Börnick H, Stolte S. Structure-related endocrine-disrupting potential of environmental transformation products of benzophenone-type UV filters: a review. J Hazard Mater 2022;430:128495.
20. Mao JF, Li W, Ong CN, He Y, Jong MC, Gin KY. Assessment of human exposure to benzophenone-type UV filters: a review. Environ Int 2022;167:107405.
21. Ghazipura M, McGowan R, Arslan A, Hossain T. Exposure to benzophenone-3 and reproductive toxicity: a systematic review of human and animal studies. Reprod Toxicol 2017;73:175-83.
22. Zheng X, Ren X, Zhao L, Guo L. Binding and activation of estrogen related receptor γ as possible molecular initiating events of hydroxylated benzophenones endocrine disruption toxicity. Environ Pollut 2020;263:114656.
23. Zhu Q, Wang M, Jia J, et al. Occurrence, distribution, and human exposure of several endocrine-disrupting chemicals in indoor dust: a nationwide study. Environ Sci Technol 2020;54:11333-43.
24. Wang W, Wang X, Zhu Q, et al. Occurrence of synthetic phenolic antioxidants in foodstuffs from ten provinces in China and its implications for human dietary exposure. Food Chem Toxicol 2022;165:113134.
25. Tang S, Sun X, Qiao X, et al. Prenatal exposure to emerging plasticizers and synthetic antioxidants and their potency to cross human placenta. Environ Sci Technol 2022;56:8507-17.
26. Xu YQ, Liu SS, Wang ZJ, Li K, Qu R. Commercial personal care product mixtures exhibit hormetic concentration-responses to Vibrio qinghaiensis sp.-Q67. Ecotoxicol Environ Saf 2018;162:304-11.
27. Xu YQ, Li K, Wang ZJ, Huang P, Liu SS. Transfer pattern of hormesis into personal care product mixtures from typical hormesis-inducing compounds. Sci Total Environ 2023;855:158981.
28. Zhu XW, Liu SS, Ge HL, Liu Y. Comparison between the short-term and the long-term toxicity of six triazine herbicides on photobacteria Q67. Water Res 2009;43:1731-9.
29. Liu S, Zhang J, Zhang Y, Qin L. APTox: assessment and prediction on toxicity of chemical mixtures. Acta Chim Sinica 2012;70:1511.
30. Wang ZJ, Liu SS, Qu R. JSFit: a method for the fitting and prediction of J- and S-shaped concentration-response curves. RSC Adv 2018;8:6572-80.
31. Stroppel L, Schultz-Fademrecht T, Cebulla M, et al. Antimicrobial preservatives for protein and peptide formulations: an overview. Pharmaceutics 2023;15:563.
32. Esimbekova EN, Asanova AA, Kratasyuk VA. Alternative enzyme inhibition assay for safety evaluation of food preservatives. Life 2023;13:1243.
33. Reeder MJ, Zhang D, Aravamuthan SR, et al. More than just methylisothiazolinone: Retrospective analysis of patients with isothiazolinone allergy in North America, 2017-2020. J Am Acad Dermatol 2024;90:319-27.
34. Molin EAD, Leite GAA, Lazzari VM. A systematic review focused on lubricant use and sperm quality: improving human reproductive success by informing lubricants toxicity. J Appl Toxicol 2024;44:1470-7.
35. Sala-Hamrick KE, Tapaswi A, Polemi KM, Nguyen VK, Colacino JA. High-throughput transcriptomics of nontumorigenic breast cells exposed to environmentally relevant chemicals. Environ Health Perspect 2024;132:47002.
36. Jun T, Shin SH, Won YY. Engineered polymeric excipients for enhancing the stability of protein biologics: poly(N-isopropylacrylamide)-poly(ethylene glycol) (PNIPAM-PEG) block copolymers. Int J Pharm 2024;664:124636.
37. Lou H, Wu Y, Kuczera K, Schöneich C. Coarse-grained molecular dynamics simulation of heterogeneous polysorbate 80 surfactants and their interactions with small molecules and proteins. Mol Pharm 2024;21:5041-52.
38. Friis UF, Menné T, Thyssen JP, Johansen JD. A patient’s drawing helped the physician to make the correct diagnosis: occupational contact allergy to isothiazolinone. Contact Dermatitis 2012;67:174-6.
39. Tang L, Liu M, Li J, et al. Isothiazolinone disrupts reproductive endocrinology by targeting the G-protein-coupled receptor signaling. Environ Sci Technol 2024;58:1076-87.
40. Silva V, Silva C, Soares P, Garrido EM, Borges F, Garrido J. Isothiazolinone biocides: chemistry, biological, and toxicity profiles. Molecules 2020;25:991.
41. Schwensen JF, Lundov MD, Bossi R, et al. Methylisothiazolinone and benzisothiazolinone are widely used in paint: a multicentre study of paints from five European countries. Contact Dermatitis 2015;72:127-38.
42. Amat AM, Arques A, López-Pérez M, Nacher M, Palacios S. Effect of methylisothiazolinone on biological treatment: efficiency of SBRs and bioindicative studies. Environ Eng Sci 2015;32:479-85.
43. Paijens C, Frère B, Caupos E, Moilleron R, Bressy A. Determination of 18 biocides in both the dissolved and particulate fractions of urban and surface waters by HPLC-MS/MS. Water Air Soil Pollut 2020;231:4546.
44. Paun I, Pirvu F, Iancu VI, Chiriac FL. Occurrence and transport of isothiazolinone-type biocides from commercial products to aquatic environment and environmental risk assessment. Int J Environ Res Public Health 2022;19:7777.
45. Lee S, Ji K. Toxicological signature for thyroid endocrine disruption of dichlorooctylisothiazolinone in zebrafish larvae. Ecotoxicology 2023;32:38-45.
46. Lee S, Lee JS, Kho Y, Ji K. Effects of methylisothiazolinone and octylisothiazolinone on development and thyroid endocrine system in zebrafish larvae. J Hazard Mater 2022;425:127994.
47. Lanigan RS. Final report on the safety assessment of PEG-7, -30, -40, -78, and -80 glyceryl cocoate. Int J Toxicol 1999;18:33-42. Available from: https://www.semanticscholar.org/paper/Final. [Last accessed on 19 Dec 2024]
48. Humann RA, Smith TK. Potential trypanocidal activity of glycerol analogues. ChemistryOpen 2024;13:e202400094.
49. Yi L, Tian M, Piao C, et al. The protective effects of 1,2-propanediol against radiation-induced hematopoietic injury in mice. Biomed Pharmacother 2019;114:108806.
50. Cheng R, Huang P, Ding TT, Gu ZW, Tao MT, Liu SS. Time-dependent hormesis transfer from five high-frequency personal care product components to mixtures. Environ Res 2024;248:118418.
51. Ortiz de García S, García-Encina PA, Irusta-Mata R. Dose-response behavior of the bacterium Vibrio fischeri exposed to pharmaceuticals and personal care products. Ecotoxicology 2016;25:141-62.
Cite This Article

How to Cite
Li, K.; Li, X. W.; Xu, Y. Q. Chemical ingredients in personal care products and their hormetic effects on freshwater photobacteria. J. Environ. Expo. Assess. 2024, 3, 30. http://dx.doi.org/10.20517/jeea.2024.36
Download Citation
Export Citation File:
Type of Import
Tips on Downloading Citation
Citation Manager File Format
Type of Import
Direct Import: When the Direct Import option is selected (the default state), a dialogue box will give you the option to Save or Open the downloaded citation data. Choosing Open will either launch your citation manager or give you a choice of applications with which to use the metadata. The Save option saves the file locally for later use.
Indirect Import: When the Indirect Import option is selected, the metadata is displayed and may be copied and pasted as needed.
About This Article
Special Issue
Copyright
Data & Comments
Data
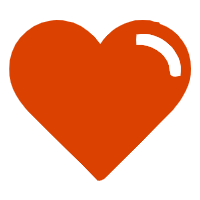
Comments
Comments must be written in English. Spam, offensive content, impersonation, and private information will not be permitted. If any comment is reported and identified as inappropriate content by OAE staff, the comment will be removed without notice. If you have any queries or need any help, please contact us at support@oaepublish.com.