Which casein micelle removal method is suitable for studies of human milk extracellular vesicles? A systematic comparison of four different treatments for casein depletion before extracellular vesicle isolation from human milk
Abstract
Aim: This study aimed to systematically compare four casein micelle removal methods on the particle and protein characteristics of the isolated human milk EVs.
Methods: The defatted milk was treated with 1% sodium citrate, 20 mM ethylenediaminetetraacetic acid (EDTA), 1% acetic acid, or 1% chymosin/calcium chloride for 30 min at 4 °C to remove casein micelles. EV isolation was performed using qEV size exclusion chromatography. Milk turbidity at the optical density 350 nm and dot immunoblot with casein antibody were applied to monitor the qEV fractions. Particle analyses were performed using transmission electron microscopy (TEM) and nanoparticle tracking analysis (NTA). The enrichment of human milk EV markers, i.e., tetraspanins, Alix, lactadherin, butyrophilin, and xanthine dehydrogenase, and casein depletion capabilities were evaluated by proteomics and immunoblotting.
Results: Compared to the untreated condition, sodium citrate and EDTA decreased milk turbidity by disrupting casein micelles, while acetic acid and chymosin removed them by inducing precipitation/coagulation. All treatments shifted casein immunoreactivity in the qEV fractions from large micelles (the exclusion volume) to small molecular sizes (gel-infiltrated fractions). Acidification affected human milk EV morphology, while EDTA, acetic acid, and chymosin methods slightly altered EV particle numbers. Different casein micelle removal methods confer different degrees of human milk EV marker enrichment and casein depletion. The method performances could be ranked as follows: chymosin > EDTA > acetic acid > sodium citrate.
Conclusion: Our findings suggest that chymosin and EDTA should be considered as the method of choice for casein micelle removal in future studies involving human milk EV isolation and characterization.
Keywords
INTRODUCTION
Extracellular vesicles (EVs) in human milk primarily originate from mammary epithelial cells. Human
Caseins, comprising alpha-, beta- and kappa-isoforms, are a major protein component (3.6 g/L; about 40% of total protein) in human milk[9,10]. Caseins interact with colloidal calcium phosphate to form the supramolecular structure of casein micelles[11]. The biophysical size of casein micelles (100-600 nm)[12] overlaps with EVs (small EVs < 200 nm; large EVs 200-1,000 nm)[10,11]. Moreover, casein micelles have a density of 1.06 g/mL[10], while EVs exhibit a density of 1.08-1.19 g/mL[10,13]. As a result, casein micelles and HMEVs (especially small EV subpopulations) are almost always co-isolated by most isolation techniques. Removing or disaggregating casein micelles in human milk prior to HMEV isolation is therefore a critical strategy for improving the purity of HMEV.
Several methods have been applied to disaggregate and remove casein micelles from human milk. These include acidification[14,15], calcium chelators by sodium citrate[16], ethylenediaminetetraacetic acid (EDTA)[17], and chymosin[18,19]. The mechanisms by which casein micelles are removed vary across these methods. For example, sodium citrate and EDTA chelate calcium to disrupt the supramolecular structure of casein micelles down to casein sub-micellar monomers (< 40 nm)[16,17]. Acidification of milk disaggregates casein micelles by dissolving calcium phosphate and induces isoelectric precipitation of caseins[14,15,20]. Chymosin is a protease that hydrolyses the negatively charged end of kappa-caseins of the micelles, causing destabilization and coagulation of casein micelles[18,19]. Note that the substrate specificity of chymosin differs slightly between bovine and human kappa caseins; bovine kappa casein is cleaved at the peptide bond between amino acid residue 126-127 (phenylalanine-methionine; P02668, uniprot.org) while human kappa casein is cleaved at amino acid residue 117-118 (phenylalanine-isoleucine; P07498, uniprot.org).
Human milk differs from bovine milk regarding physicochemical characteristics, including the composition and amounts of casein micelles[9,21-23]. Bovine milk composition is high in alpha-casein (greater than 50% of whole casein)[24], while human milk composition is high in beta-casein (approximately alpha 15%, beta 55%, kappa 30% at 2-week lactation)[22]. Furthermore, alpha- and beta-casein have different biochemical properties; alpha-casein is more hydrophilic, while beta-casein is more hydrophobic[25]. These casein differences may affect the performance of casein removal methods that are applied to bovine milk and human milk.
Previous studies have compared various methods for removing casein micelles, particularly for isolating EVs from bovine milk[15,17,26]. However, research on casein micelle removal methods specifically for EV isolation from human milk is lacking. The physicochemical differences between human and bovine milk suggest that methods effective for bovine milk may or may not be directly applicable to human milk. Therefore, this study aimed to systematically compare and optimize methods previously used on bovine milk, including sodium citrate, EDTA, acetic acid, and chymosin treatments for casein removal, adapting these methodologies to the distinct isolation of HMEVs. This paper represents more than incremental progress by establishing an optimized, human milk-specific method for EV isolation from clinical samples.
MATERIALS AND METHODS
Human milk sample preparation
Whole milk samples from four donors were retrieved from the MOM2CHild Study (IRB protocol ID:
Casein micelle removal
The defatted milk (1 mL) was treated with sodium citrate (1% final; S4641, Sigma, USA), EDTA (20 mM final; E6758, Sigma, USA), acetic acid (1% final; A6283, Sigma, USA), and chymosin/ calcium chloride (CaCl2) (1% final; # 50-111-8061, Thermo Fisher Scientific, USA) and incubated at 4 °C for 30 min. After incubation, the samples with sodium citrate and EDTA were ready for EV isolation. In contrast, the samples treated with acetic acid and chymosin required an additional step of centrifugation at 12,000 × g, 4 °C for
To measure the kinetic of milk turbidity changes, the defatted milk was treated with sodium citrate (1% final), EDTA (20 mM final), acetic acid (1% final), and chymosin/CaCl2
HMEV isolation
For EV isolation, the samples were concentrated for large molecules while removing low molecular weight contamination using 100-kDa cutoff centrifugal filtration (Merck Millipore). The concentrated samples
Detection of caseins in EV fractions using dot immunoblot
Two microliters of each fraction (1 to 20) were blotted onto the nitrocellulose membrane. After air drying for 30 min, the membrane was then blocked with Everyblot blocking buffer (Bio-Rad, USA) at room temperature for 5 min and stained with the primary antibody of β-casein antibody (sc-53189; Santa Cruz Biotechnology, Inc., Dallas, TX) at 1:500 dilution in Everyblot blocking buffer at 4 °C overnight. After washing, the membranes were incubated with goat anti-mouse IgG secondary antibody-HRP (Invitrogen) at room temperature for 1 h. The dot blot was developed by SuperSignal West Pico PLUS Chemiluminescent (ThermoFisher) and imaged on a ChemiDoc Touch Imaging system (Bio-Rad, USA).
Nanoparticle tracking analysis
NanoSight NS300 was used with an integrated sample pump (Malvern Instruments Ltd., Malvern, Worcestershire, UK). The EV isolates were diluted at 1:100 in 0.22 µm filtered phosphate buffer saline (PBS) to 1 mL. The sample was then injected into the chamber with a syringe pump. Five 1-min videos were captured for each sample with the following parameters: camera: sCMOS; cell temperature: 25 °C. After capture, the videos were analyzed by NanoSight Software NTA 3.4 Build 3.4.003 with this setting: detection threshold: 5; blur size and max jump distance: auto. Ideal concentrations were measured at 20-100
Transmission electron microscopy
Five microliters of the EV isolates were applied onto a 200-mesh grid (FCF200-CU; Electron Microscopy Sciences) and negatively stained by using 2% uranyl acetate (#22400; Electron Microscopy Sciences) for 10 seconds. The grid was then washed 2 times with deionized water before air drying for 1 minute at room temperature. Imaging was performed using a transmission electron microscope (Thermo Talos L120C Transmission Electron Microscope) with an acceleration voltage of 100 kV.
Western blot analysis
The EV sample (5 μg protein) was lysed with RIPA buffer and mixed with 4x Leammli buffer (Biorad, USA). The samples were heated at 95 °C for 5 min and then run into the NuPAGE™ 12%, Bis-Tris, 1.0 mm, mini protein gels for 50 min (Invitrogen, USA) before being transferred onto a PVDF membrane using a transblot (Bio-Rad, USA). The membrane was blocked with Everyblot blocking buffer (Bio-Rad, USA) and then probed with 1:1,000 rabbit anti-CD9 (ab223052) or β-casein antibody (sc-53189; Santa Cruz Biotechnology, Inc.) at 1:500 at 4 °C overnight. After washing, the membranes were incubated with 1:3,000 goat anti-rabbit IgG secondary antibody HRP (Abcam) (for CD9) and goat anti-mouse IgG secondary antibody HRP (Invitrogen) (for β-casein) at room temperature for 1 h. The immunoblot was developed by SuperSignal West Pico PLUS Chemiluminescent (ThermoFisher) and imaged on a ChemiDoc Touch Imaging system (Bio-Rad, USA).
Mass spectrometric proteomics
Proteomic analysis was performed as described previously[5]. Briefly, two micrograms of EV proteins were reduced, alkalinized, and digested by trypsin (1:50 w/w; MS-grade, Thermo) at 37 °C overnight, and cleaned up by C18 solid phase extraction. Data were collected on an Orbitrap Eclipse mass spectrometer coupled to Dionex Ultimate 3000 RSLCnano (Thermo) using an EASY-Spray column PepMap RSLC C18 with a
RESULTS
Casein micelles share a comparable particle diameter with EVs[10-12], have a very high abundance in milk matrix, and are the significant contamination during HMEV isolation. This study, therefore, compared four biochemical methods commonly applied to remove caseins from bovine and human milk in literature and measured particle and protein evidence following HMEV isolation.
Chymosin treatment increased milk turbidity by agglutinating casein micelles, while acetic acid, EDTA, and sodium citrate decreased turbidity by disrupting the micelles
To assess the impact of different casein micelle disaggregation/removal methods, we measured the kinetic of milk turbidity changes following treatments with 1% sodium citrate, 20 mM EDTA, 1% acetic acid, and 1% chymosin/CaCl2 at 0, 0.5, 1, 2, 5, 10, 15, and 30 min. The optical density at 350 nm (OD350) was applied as this wavelength is sensitive to monitor the eluted EVs from size exclusion chromatography. Figure 1 shows that chymosin treatment gradually increased the OD350 values over time compared to the untreated condition, suggesting increased turbidity, which is consistent with its mechanism to agglutinate casein micelles. In contrast, calcium chelators, including EDTA and sodium citrate, markedly decreased the OD350 values by disrupting the casein micelle complexes, reaching the plateau within 10 min of incubation. Acetic acid treatment gradually reduced the OD350 values over time by changing the solution pH, resulting in casein precipitation out of the solution. Note that both chymosin and acetic acid methods required an incubation time of up to 30 min before the OD350 reached the plateau and needed an additional step of centrifugation to remove the casein aggregates before submitting the supernatant for HMEV isolation, thus increasing the workload and turnaround time compared to EDTA and sodium citrate treatments.
Figure 1. The kinetics of milk turbidity changes upon different casein micelle removal treatments. The defatted milk was treated with sodium citrate (NaCit), EDTA, acetic acid, and chymosin/CaCl2. The mixture was measured with the OD350 at 0, 0.5, 1, 2, 5, 10, 15, and 30 min following treatments (n = 3 independent replicates). The results were reported as mean ± standard error. EDTA: ethylenediaminetetraacetic acid.
All casein micelle removal treatments shift casein immunoreactivity from large micelles to lower molecular sizes, as detected by a dot blot of qEV fractions
To demonstrate the capability of casein micelle removal based on the biophysical property, this study performed qEV size exclusion chromatography, which allowed us to separate the supramolecular structure of casein micelles (eluted at the exclusion volume of the qEV column similar to HMEVs; fractions 5 and 6) from sub-micellar caseins and their monomers that have smaller biophysical sizes (eluted at fractions 7-20). Following the qEV fractionation, casein antigenicity in every fraction (from 1 to 20) was detected by dot immunoblot with casein-specific antibody [Figure 2A]. The results showed that: (i) in the untreated condition, high casein immunoreactive signals were observed in fractions 5 and 6 (representing casein micelles) with tails in fractions 7-10 (representing sub-micellar caseins) and fractions 11-15 (indicating casein monomers); (ii) all casein micelle removal methods could shift the firstly detected fraction of casein immunoreactivity from the qEV exclusion volume, suggesting depletion of casein micelle contaminants in the collected HMEVs (fractions 5 and 6); (iii) by visual recognition, the method efficacy in shifting the supramolecular structure of casein micelles to smaller casein monomers which could infiltrate into the qEV gel beads were as follows: chymosin > EDTA > sodium citrate > acetic acid. Consistently, the protein content of all the collected fractions recapitulated the shift of casein micelles to the smaller forms in the later fractions [Figure 2B]. This finding strongly suggested that all treatments can reduce casein micelles from the EV fractions. Of note, dot immunoblot can only detect caseins in the solution, not caseins inside the lipid bilayer-enclosed HMEVs.
Figure 2. Dot blot analysis of qEV fractions using anti-casein antibody. (A) The representative dot blot images display the profiles of milk EV-isolated fractions after treatment with casein micelle disaggregation/removal reagents. Two microliters of each of the 20 fractions were blotted onto a nitrocellulose membrane. The membranes were then probed with antibodies specific for β-casein and developed using anti-mouse-HRP for signal detection; (B) Protein concentrations in all collected fractions measured by Pierce 660 protein assay. EDTA: ethylenediaminetetraacetic acid.
Casein micelle removal methods affect the intact HMEV morphology and particle size distribution differently
To determine whether casein micelle removal methods altered EV particle characteristics, TEM was used to visualize the single vesicle morphology, and NTA was applied to determine particle size distribution. As a result, TEM exhibited HMEVs as cup-shaped membrane-enclosed nanoscale vesicles. Their shape and morphology were not affected by most methods except acidification which may induce the rough surface morphology [Figure 3A]. NTA showed that EDTA and acetic acid methods slightly increased the number of particles and chymosin slightly decreased the EV numbers compared to the untreated condition [Figure 3B]. One possible explanation is that EDTA and acetic acid methods may release a number of EVs from casein micelle complexes while chymosin, because of its mechanism to induce casein micelle coagulation, may trap and remove some of EVs during aggregation removal by centrifugation. These findings suggested that different casein removal methods may affect HMEV morphology and particle numbers.
Figure 3. HMEV particle evidence after casein micelle removal treatment. (A) Transmission electron microscopy (TEM) images show the cup-shaped morphology of isolated EVs following casein micelle disaggregation/removal; (B) Nanoparticle tracking analysis (NTA) presents the size distribution, average diameter, and concentration (particles/mL) of the HMEVs following each treatment. EDTA: ethylenediaminetetraacetic acid.
Different casein micelle removal methods confer different degrees of HMEV protein marker enrichment and casein depletion
It was expected that, after casein micelle removal, the HMEVs and their associated protein markers would be enriched. In this direction, we conducted proteomic analyses to characterize common EV proteins[8] and specific HMEV markers[28,29] and assess the amounts of casein contamination in the HMEV isolates. As a result, proteomic analysis detected 393 proteins across all treatment groups
Figure 4A demonstrates the fold changes of 87 HMEV proteins following casein micelle removal methods compared to the untreated condition. In contrast, Figure 4B illustrates the relative protein intensities of selected EV markers and caseins. Overall, the result showed that: (i) the common EV proteins and
Figure 4. HMEV protein evidence after casein micelle removal treatment. Proteomic analysis of HMEVs isolated after casein micelle disaggregation/removal by sodium citrate, EDTA, acetic acid, and chymosin treatments [full results in Supplementary Table 1]. (A) Fold-changes of 87 HMEV proteins as compared to the untreated condition (details in Supplementary Table 2). Color codes: blue, common and specific HMEV markers; green, highly abundant soluble proteins in human milk; black, caseins; (B) The average protein intensities of selected HMEV markers, total caseins (alpha-, beta-, kappa-caseins), and the ratio of caseins per CD9; (C) Western immunoblot demonstrated that different casein micelle removal methods exhibited different capabilities of casein depletion and HMEV enrichment (full-length blots of the cropped images were provided in Supplementary Figure 2). BTN1A1: butyrophilin 1A1; BSSL: bile salt-stimulated lipase; LALBA: alpha-lactalbumin; LF: lactoferrin; MFGE8: milk fat globule-epidermal growth factor 8 (also known as lactadherin); MUC1: mucin 1; NaCit: sodium citrate; TNC: tenascin; XDH: xanthine dehydrogenase. EDTA: ethylenediaminetetraacetic acid.
DISCUSSION
This study systematically compared four biochemical methods and their performance in reducing casein contamination while enriching HMEVs isolated from human milk. Comparisons included sodium citrate and EDTA, which disaggregate casein micelles by calcium chelation, and acetic acid and chymosin/CaCl2, which precipitate caseins out of the solution. The main findings are that all methods could remove or disaggregate large casein micelles out of the qEV exclusion volume [Figure 2]; different methods had different effects on HMEV particle analysis and yields [Figure 3]; and that different methods had different efficacy on casein depletion and HMEV enrichment [Figure 4].
The strength of calcium chelation-based methods is their simplicity and turnaround time. Both sodium citrate and EDTA treatments need only one step of the procedure to mix the chemicals with the human milk matrix, and casein micelle disaggregation reaches a plateau within 10 min [Figure 1]. After ten min, the mixture is ready for HMEV isolation. In contrast, casein precipitation/coagulation methods require a two-step procedure: first, sample treatment and second, centrifugation. In our experience, the optimal incubation time for acetic acid and chymosin treatments can be up to 30 min [Figure 1]. The workload and turnaround time difference between calcium chelation-based and casein precipitation methods may be insignificant in small projects, but the difference in efficiency is an important consideration in larger studies.
In our study, HMEV morphology was not affected by most casein removal treatments, which was consistent with the findings of Tong et al. in their comparison of chymosin and HCl treatment[26]. On the other hand, two studies reported a rough surface of bovine milk EVs treated with acidification despite the vesicle morphology remaining spherical[15,30]. In our experiments, we used human milk samples rather than bovine milk. Our TEM results showed that the acetic acid method may also induce the rough surface of HMEVs [Figure 3A], even though that change was not as obvious as reports from previous studies of bovine EVs[15,30]. Human milk features lower concentrations of casein, lower levels of alpha- and higher beta-casein isoforms, smaller casein micelles, and exhibits less calcium interaction than bovine milk[9,21-23]. While the effects of different casein micelle removal methods on particle characteristics of bovine milk EVs have yet to be determined, our study did not observe major issues for HMEVs in this regard.
Among different methods, chymosin and EDTA obviously shifted the supramolecular structure of casein micelles from the HMEV fractions, where the casein contents were moving to latter fractions, as shown in the dot blot [Figure 2], suggesting the effectiveness of both methods in reducing the size of caseins. Interestingly, proteomic analysis revealed that caseins were still detectable in substantial amounts in the HMEV isolates [Figure 4A]. A possible explanation was that caseins detected in the HMEV isolates were the remnants of sub-micellar caseins, intravesicular proteins, or the surface corona of HMEVs. The surface corona is a set of molecules, mainly proteins, that are adsorbed around EVs in the aqueous phase to constitute the external layer of EVs. The surface corona is thought to affect EV physicochemical properties, cellular uptake, and tissue biodistribution[31]. This hypothesis that detected caseins in the HMEV isolates were remnants of the surface corona of EVs was supported by the ratio of caseins to CD9 [Figure 4B and C] in which, per one unit of CD9, caseins were markedly depleted by EDTA, acetic acid, and chymosin treatments (and slightly reduced by sodium citrate method). Since intravesicular caseins should be considerably stable compared to one unit of CD9, our findings did not support this possibility. To distinguish between sub-micellar caseins and caseins of the HMEV corona, a more rigorous strategy to completely remove sub-micellar caseins would be required, for example, a combination of two or three methods with different mechanisms of casein disaggregation/removal. Thereafter, surfaceomics (proteomics of surface proteins) could be applied to obtain the evidence of caseins presented in the HMEV corona. Proper optimizations are needed to establish a successful combination strategy, including the method type, number, and sequence of action, while the cost, time, and workload per sample should be considered a reasonable trade-off. Given the significant roles of the EV corona, HMEV surfaceomics should also be pursued in future studies.
This study had limitations. First, this study compared casein micelle removal methods and applied qEV size exclusion chromatography as the sole EV isolation technique. The methods performed in this study may slightly differ when applied to other EV isolation methodologies. When applying an isolation method other than qEV size exclusion chromatography, some may use our findings to choose a preferred casein micelle removal method and then confirm its performance in the context of a different EV isolation method. Second, this study used frozen biobanked human milk. Due to the limited volume of individual samples, we generated a master pool from four individual milk samples before aliquoting for each arm of the casein removal methods to ensure that the amount of EVs is sufficient and consistent for all comparison conditions. Concerns about the freezing effects of milk samples on EV integrity and yield were addressed by conducting NTA on EVs from both fresh and frozen samples, stored at -80 °C, from the same donor. The results confirmed that a single freeze-thaw cycle did not adversely affect the EVs [Supplementary Figure 3]. Lastly, this study did not include HMEV functionalities in the comparison. Different chemicals that remove caseins may induce changes in HMEV molecular cargos and their functions. Contamination, even at the minute amount, of sodium citrate, EDTA, acetic acid, and chymosin may interfere with HMEV functional studies. Different degrees of casein depletion and HMEV marker enrichment of four casein micelle removal methods may largely contribute to the HMEV potency. Since the findings would be crucial to breastfeeding medicine and HMEV therapeutics, future studies should be pursued to address those issues.
Table 1 summarizes the performance of four casein micelle removal methods based on particle analysis, casein depletion, HMEV enrichment, workload, and turnaround time. This information can guide the preferred method to handle casein contamination in future studies involving HMEV isolation and characterization. From our perspective, chymosin or EDTA treatments are two methods of choice, and their selection may depend on the number of samples and the specific needs of each project.
The performance of four casein micelle removal methods in this HMEV study
Casein micelle removal methoda | Particle size and yield | Casein depletion | HMEV marker enrichment | Step of procedure | Turnaround time |
1% Sodium citrate | ++ | + | +/- | 1 | < 10 min |
20 mM EDTA | +++ | ++ | +++ | 1 | < 10 min |
1% Acetic acid | +++ | ++ | ++ | 2 | > 30 min |
1% Chymosin/CaCl2 | ++ | +++ | ++++ | 2 | > 30 min |
DECLARATIONS
Acknowledgment
We thank Drs. Kenneth D. Greis, Wendy Haffey, Desiree Benefield, and Michael Wyder for their technical assistance on proteomics and transmission electron microscopy. The graphical abstract was created with Biorender.com.
Authors’ contributions
Conceptualization: Chutipongtanate S
Methodology, investigation, and formal analysis: Cetinkaya H, Kongsomros S, Chutipongtanate S
Validation, resource, and writing-review & Editing: Nommsen-Rivers L, Morrow AL, Chutipongtanate S
Funding acquisition: Morrow AL
Supervision: Morrow AL, Chutipongtanate S
Writing-original draft preparation: Cetinkaya H, Kongsomros S
Read and agreed to the published version of the manuscript: Cetinkaya H, Kongsomros S, Nommsen-Rivers L, Morrow AL, Chutipongtanate S
Availability of data and materials
The data that support the findings of this study are available in the manuscript and Supplementary Materials.
Financial support and sponsorship
This study was supported by the NIH/NICHD grant with the reference number R01HD109915; The mass spectrometry data were collected on an Orbitrap system purchased in part through support from a National Institutes of Health Shared Instrumentation Grant Program (S10OD02671).
Conflicts of interest
All authors declared that there are no conflicts of interest.
Ethical approval and consent to participate
Whole milk samples from four donors were retrieved from the MOM2CHild Study (IRB protocol ID: 2022-0653). Written informed consent to participate was obtained.
Consent for publication
Not applicable.
Supplementary Materials
Copyright
© The Author(s) 2024.
REFERENCES
1. Chutipongtanate S, Morrow AL, Newburg DS. Human milk extracellular vesicles: a biological system with clinical implications. Cells 2022;11:2345.
2. Ngu A, Munir J, Zempleni J. Milk-borne small extracellular vesicles: kinetics and mechanisms of transport, distribution, and elimination. Extracell Vesicles Circ Nucl Acids 2023;4:339-46.
3. Freiría-Martínez L, Iglesias-Martínez-Almeida M, Rodríguez-Jamardo C, et al. Proteomic analysis of exosomes derived from human mature milk and colostrum of mothers with term, late preterm, or very preterm delivery. Anal Methods 2023;15:4905-17.
4. Meng Z, Zhou D, Lv D, et al. Human milk extracellular vesicles enhance muscle growth and physical performance of immature mice associating with Akt/mTOR/p70s6k signaling pathway. J Nanobiotechnology 2023;21:304.
5. Chutipongtanate S, Cetinkaya H, Zhang X, et al. Prenatal SARS-CoV-2 infection alters postpartum human milk-derived extracellular vesicles. bioRxiv 2023;Online ahead of print:2023.06.01.543234.
6. Peng W, Han J, Li S, et al. The association of human milk feeding with short-term health outcomes among chinese very/extremely low birth weight infants. J Hum Lact 2022;38:670-7.
7. Royo F, Théry C, Falcón-Pérez JM, Nieuwland R, Witwer KW. Methods for separation and characterization of extracellular vesicles: results of a worldwide survey performed by the ISEV rigor and standardization subcommittee. Cells 2020;9:1955.
8. Welsh JA, Goberdhan DCI, O'Driscoll L, et al. MISEV Consortium. Minimal information for studies of extracellular vesicles (MISEV2023): from basic to advanced approaches. J Extracell Vesicles 2024;13:e12404.
9. Hernell O. Human Milk vs. Cow’s milk and the evolution of infant formulas. In: Clemens R, Hernell O, Michaelsen K, editors. Milk and Milk Products in Human Nutrition. S. Karger AG; 2011. pp. 17-28.
10. Blans K, Hansen MS, Sørensen LV, et al. Pellet-free isolation of human and bovine milk extracellular vesicles by size-exclusion chromatography. J Extracell Vesicles 2017;6:1294340.
11. McMahon DJ, Oommen BS. Supramolecular structure of the casein micelle. J Dairy Sci 2008;91:1709-21.
12. Trejo R, Dokland T, Jurat-Fuentes J, Harte F. Cryo-transmission electron tomography of native casein micelles from bovine milk. J Dairy Sci 2011;94:5770-5.
13. Brennan K, Martin K, FitzGerald SP, et al. A comparison of methods for the isolation and separation of extracellular vesicles from protein and lipid particles in human serum. Sci Rep 2020;10:1039.
14. Somiya M, Yoshioka Y, Ochiya T. Biocompatibility of highly purified bovine milk-derived extracellular vesicles. J Extracell Vesicles 2018;7:1440132.
15. Rahman MM, Shimizu K, Yamauchi M, et al. Acidification effects on isolation of extracellular vesicles from bovine milk. PLoS One 2019;14:e0222613.
16. Benmoussa A, Michel S, Gilbert C, Provost P. Isolating multiple extracellular vesicles subsets, including exosomes and membrane vesicles, from bovine milk using sodium citrate and differential ultracentrifugation. Bio Protoc 2020;10:e3636.
17. Morozumi M, Izumi H, Shimizu T, Takeda Y. Comparison of isolation methods using commercially available kits for obtaining extracellular vesicles from cow milk. J Dairy Sci 2021;104:6463-71.
18. Liu Q, Hao H, Li J, et al. Oral administration of bovine milk-derived extracellular vesicles attenuates cartilage degeneration via modulating gut microbiota in DMM-induced mice. Nutrients 2023;15:747.
19. Chen CC, Chen LY, Li WT, et al. Influence of chymosin on physicochemical and hydrolysis characteristics of casein micelles and individual caseins. Nanomaterials 2021;11:2594.
20. Mukhopadhya A, Santoro J, O'Driscoll L. Extracellular vesicle separation from milk and infant milk formula using acid precipitation and ultracentrifugation. STAR Protoc 2021;2:100821.
21. Pietrzak-Fiećko R, Kamelska-Sadowska AM. The comparison of nutritional value of human milk with other mammals' milk. Nutrients 2020;12:1404.
22. Liao Y, Weber D, Xu W, Durbin-Johnson BP, Phinney BS, Lönnerdal B. Absolute quantification of human milk caseins and the whey/casein ratio during the first year of lactation. J Proteome Res 2017;16:4113-21.
23. Βasdeki AM, Fatouros DG, Βiliaderis CG, Moschakis T. Physicochemical properties of human breast milk during the second year of lactation. Curr Res Food Sci 2021;4:565-76.
24. Huppertz T, Fox P, Kelly A, Yada R. Proteins in food processing. In: Woodhead Publishing Cambridge, UK; 2018. pp. 1-654.
25. Hassanin AA, Osman A, Atallah OO, et al. Phylogenetic comparative analysis: chemical and biological features of caseins (alpha-S-1, alpha-S-2, beta- and kappa-) in domestic dairy animals. Front Vet Sci 2022;9:952319.
26. Tong L, Hao H, Zhang X, et al. Oral administration of bovine milk-derived extracellular vesicles alters the gut microbiota and enhances intestinal immunity in mice. Mol Nutr Food Res 2020;64:e1901251.
27. Chutipongtanate S, Greis KD. Multiplex biomarker screening assay for urinary extracellular vesicles study: a targeted label-free proteomic approach. Sci Rep 2018;8:15039.
28. Admyre C, Johansson SM, Qazi KR, et al. Exosomes with immune modulatory features are present in human breast milk. J Immunol 2007;179:1969-78.
29. van Herwijnen MJ, Zonneveld MI, Goerdayal S, et al. Comprehensive proteomic analysis of human milk-derived extracellular vesicles unveils a novel functional proteome distinct from other milk components. Mol Cell Proteomics 2016;15:3412-23.
30. Yamauchi M, Shimizu K, Rahman M, et al. Efficient method for isolation of exosomes from raw bovine milk. Drug Dev Ind Pharm 2019;45:359-64.
Cite This Article
Export citation file: BibTeX | EndNote | RIS
OAE Style
Cetinkaya H, Kongsomros S, Nommsen-Rivers L, Morrow AL, Chutipongtanate S. Which casein micelle removal method is suitable for studies of human milk extracellular vesicles? A systematic comparison of four different treatments for casein depletion before extracellular vesicle isolation from human milk. Extracell Vesicles Circ Nucleic Acids 2024;5:221-32. http://dx.doi.org/10.20517/evcna.2024.02
AMA Style
Cetinkaya H, Kongsomros S, Nommsen-Rivers L, Morrow AL, Chutipongtanate S. Which casein micelle removal method is suitable for studies of human milk extracellular vesicles? A systematic comparison of four different treatments for casein depletion before extracellular vesicle isolation from human milk. Extracellular Vesicles and Circulating Nucleic Acids. 2024; 5(2): 221-32. http://dx.doi.org/10.20517/evcna.2024.02
Chicago/Turabian Style
Hatice Cetinkaya, Supasek Kongsomros, Laurie Nommsen-Rivers, Ardythe L. Morrow, Somchai Chutipongtanate. 2024. "Which casein micelle removal method is suitable for studies of human milk extracellular vesicles? A systematic comparison of four different treatments for casein depletion before extracellular vesicle isolation from human milk" Extracellular Vesicles and Circulating Nucleic Acids. 5, no.2: 221-32. http://dx.doi.org/10.20517/evcna.2024.02
ACS Style
Cetinkaya, H.; Kongsomros S.; Nommsen-Rivers L.; Morrow AL.; Chutipongtanate S. Which casein micelle removal method is suitable for studies of human milk extracellular vesicles? A systematic comparison of four different treatments for casein depletion before extracellular vesicle isolation from human milk. Extracell. Vesicles. Circ. Nucleic. Acids. 2024, 5, 221-32. http://dx.doi.org/10.20517/evcna.2024.02
About This Article
Copyright
Data & Comments
Data
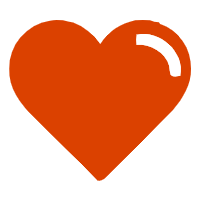
Comments
Comments must be written in English. Spam, offensive content, impersonation, and private information will not be permitted. If any comment is reported and identified as inappropriate content by OAE staff, the comment will be removed without notice. If you have any queries or need any help, please contact us at support@oaepublish.com.