Proton shuttle: a key for efficient ammonia electrosynthesis
Keywords
Ammonia (NH3) is one of the essential feedstocks for fertilizers, pharmaceuticals, polymers and other chemicals, as well as an ideal zero-carbon energy carrier, with a global market of ~350 million metric tons per year by 2050[1]. The industrial synthesis of NH3 by Haber-Bosch process under high pressure (i.e., 150 to 200 bar) at elevated temperatures (i.e., 350 to 450 oC) consumes a huge amount (i.e., ~1%) of global energy every year, leading to a 1.3% of global CO2 emission[2]. Alternatively, the electrochemical nitrogen reduction reaction using lithium as medium (Li-NRR) in electrolytes for NH3 synthesis has proven to be a zero-carbon emission process, with a highly desired perspective[3,4]. Generally, the Li-NRR for the NH3 synthesis contains three steps [Figure 1A]: (1) the Li+ in electrolyte is electrochemically reduced to Li0 on the cathode; (2) the Li0 dissociates N2 to generate lithium nitride (e.g., Li3N); (3) the lithium nitride is further protonated by proton source, offering NH3 product and leaving Li+ ions to repeat the catalytic cycle[5]. The required sacrificial solvent (e.g., ethanol) as the proton sources in nonaqueous electrolytes strongly hampers the industrialization of Li-NRR for continuous NH3 synthesis over the past three decades.
Figure 1. (A) Schematic proton-shuttling process for lithium-mediated ammonia synthesis in a continuous flow reactor; (B) Ammonia faradaic efficiencies for a variety of proton shuttles by passing a total charge of 700 C (over 2.5 h) with the constant potential cycling
A proton shuttle, a type of functional molecule that favors proton transportation and can be fully recycled, proposed for solving the problem of sacrificial proton sources is of significance as it allows the proton for continuous Li-NRR on the cathode coming from a sustainable hydrogen oxidation reaction (HOR) of external H2 gas on the anode of an electrocatalysis system. The first exploration of proton shuttles was performed by Krishnamurthy et al., who report that 1-butanol is identified as one of the most effective proton shuttles [faradaic efficiency (FE) of 15.6%][6]. However, they later clarified that the enhanced Li-NRR performance was mainly due to the change property of the solid-electrolyte interphase in the presence of proton shuttles. Recently, Suryanto et al. developed the phosphonium-based salts (e.g., [P6,6,6,14][eFAP]) as the proton shuttle for Li-NRR over three days of continuous operation, and confirmed the protonation reversibility of [P6,6,6,14]+, unfortunately, by an ex-situ simulated experiment reacting with acetic acid[7]. It leaves an unclarified proton shuttling mechanism in the real Li-NRR process for ammonia electrosynthesis, causing an unknown design strategy for effective and easily accessible proton shuttles.
The study by Fu et al. fills some of these gaps[8]. Specifically, by combining detailed Li-NRR process analysis and functional interpretations, the authors summarize the basic requirements and principles for designing effective proton shuttles: (1) containing functional groups (e.g., -OH, -COOH, and -CHO-) or specific moiety (e.g., α-hydrogen atom and -CH2-) that can donate/accept a proton; (2) a proper acid dissociation constant (pKa) to balance protonation and minimize side reaction in electrolytes; (3) enabling form a functional solid-electrolyte interphase on cathode to allow H+ and Li+ diffusion; (4) good electrochemical stability and high chemical stability; (5) an optimal diffusion rate in electrolytes that allows the control of the amount of available protons for reacting with lithium nitride on the cathode surface; (6) excellent compatibility with the catalysts and electrolytes in Li-NRR system; Following the essential elements proposed in principles, the authors carefully evaluate dozens of potential proton shuttles in a continuous-flow reactor; it shows that phenol offers the highest performance with 72% ± 3% FE, alcohols are good shuttles (27%~69% FE), amines and carboxylic acids are inefficient shuttles (4%~24% FE), and phosphonium salts remain in the mediate [Figure 1B], offering a conclusion: the dependence of the Li-NRR performance on the pKa and diffusion coefficient of proton shuttles. This comprehensive exploration and evaluation on proton shuttles solve one of the hardest but the most important tasks in Li-NRR process. It is of great value to the scientific study and the industrialization of Li-NRR for zero-carbon emission NH3 synthesis, which are strongly supported by the later significant breakthroughs in long-term continuous ammonia electrosynthesis from Li et al.[9,10].
A key finding of this work is the identification of the proton shuttling behavior in the real Li-NRR process by operando isotope-labeled mass spectrometry, coupled with utilizing the deprotonated proton shuttles (B-) to evaluate the proton transfer capability. Using deuterium (D2) oxidation reaction (DOR) as the proton (D+) source, the classically hypothesized proton shuttling mechanism was skillfully in-situ confirmed: D+ is shuttled by phenol (PhOH) during Li-NRR, and it offers the main ammonia product gradual changing from NH3, NH2D and NH1D2, finally to a sole ND3 owing to the protonation of PhO- to PhOD [Figure 1C]. The protonation and deprotonation behavior of proton shuttles (i.e., PhO-) was respectively clarified by using a PhOLi precursor, which converts to PhOH in real Li-NRR process. This work provides unambiguous evidence of the proton shuttling using PhOH as a proof-of-concept demonstration for the first time, setting an excellent example for future researchers on developing alternative novel proton shuttles.
While searching for the mechanisms enabling PhOH to be the efficient proton shuttle, the authors could unexpectedly identify that the pKa and the size-related diffusivity codetermine the Li-NRR performance: an optimal NH3 FE would require either acidic but transport-limited proton shuttles or basic but transport-fast shuttles [Figure 1D and E]. The pKa determines the protonation-deprotonation kinetic of proton shuttles on the anode and cathode surfaces, while the size of proton shuttles affects their diffusion mobility between the anode and cathode. These findings highlight the intense correlation between pKa and size-related diffusivity in proton shuttling, and they could serve as significant guidelines for developing novel proton shuttles.
This study provides a detailed analysis on sieving efficient proton shuttles and clarifies the proton shuttling behavior in the real Li-NRR process. The detailed mechanistic insights and the demonstration of two key factors [i.e., the pKa in tetrahydrofuran (THF) and the size-related diffusivity] mark a significant advancement in our understanding of proton shuttles. However, several questions remain open. A key area for further investigation is elucidating the universality of the proposed design principle in various solvents of electrolytes (such as ether-based solvents), other than THF. Additional catalytic kinetic exploration needs to be performed to clarify the way how the instinct property of solvent affects the H+ limited and N2 limited step during the Li-NRR process [Figure 1D]. Moreover, even though the theoretical reaction steps were clearly presented [Figure 1A], the practical reaction mechanism, in particular, how the protons obtained from the deprotonation of proton shuttle react with lithium nitride, remains enigmatic. It indicates that an urgent and significant breakthrough in the Li-NRR mechanism is highly desired, potentially through the use of advanced operando technologies (e.g., in-situ transient absorption spectroscopy, operando isotope-labeled mass spectrometry, etc.). Besides that, the large operation voltage of Li-NRR (i.e., ~4.3 V[8], much higher than the standard electrode potential of Li, 3.045 V) shows a low energy efficiency of the current Li-NRR process. Further development on promising electrolytes and electrode materials for the industrialization of Li-NRR with low energy consumption is important. All these questions are required to be addressed in future studies in order to set up the way for sustainable and environmentally friendly ammonia production.
DECLARATIONS
Authors’ contributions
Made substantial contributions to conception and design of the study and performed data analysis and interpretation: Wang, Z., Su, B.L.
Performed data acquisition and provided administrative, technical, and material support: Wang, Z., Guan, Z.L.
Availability of data and materials
Not applicable.
Financial support and sponsorship
Wang, Z. acknowledges the Hubei Provincial Department of Education for the “Chutian Scholar” program and the “Wuhan Yingcai” program. This work was supported by the National Key R&D Program of China (grant 2021YFE0115800), National Natural Science Foundation of China (Nos. 22293020, 22293022, 21902122), Natural Science Foundation of Hubei Province (No. 20221j0082) and Youth Innovation Research Foundation of the State Key Laboratory of Advanced Technology for Material Synthesis and Processing.
Conflicts of interest
Wang, Z., a Junior Editorial Member of Chemical Synthesis, was not involved in the editorial process of the work. Su, B.L., Editor-in-Chief of Chemical Synthesis, was not involved in the editorial process of the work. While the other author has declared that he has no conflicts of interest.
Ethical approval and consent to participate
Not applicable.
Consent for publication
Not applicable.
Copyright
© The Author(s) 2025.
REFERENCES
1. Brown, T. What drives new investments in low-carbon ammonia production? One million tons per day demand. 2018. Available from: https://ammoniaenergy.org/articles/what-drives-new-investments-in-low-carbon-ammonia-production-one-million-tons-per-day-demand/. [Last accessed on 2 Dec 2024].
2. Erisman, J. W.; Sutton, M. A.; Galloway, J.; Klimont, Z.; Winiwarter, W. How a century of ammonia synthesis changed the world. Nature. Geosci. 2008, 1, 636-9.
3. Fu, X.; Pedersen, J. B.; Zhou, Y.; et al. Continuous-flow electrosynthesis of ammonia by nitrogen reduction and hydrogen oxidation. Science 2023, 379, 707-12.
4. Cao, N.; Zhang, N.; Wang, K.; Yan, K.; Xie, P. High-throughput screening of B/N-doped graphene supported single-atom catalysts for nitrogen reduction reaction. Chem. Synth. 2023, 3, 23.
5. Li, K.; Andersen, S. Z.; Statt, M. J.; et al. Enhancement of lithium-mediated ammonia synthesis by addition of oxygen. Science 2021, 374, 1593-7.
6. Krishnamurthy, D.; Lazouski, N.; Gala, M. L.; Manthiram, K.; Viswanathan, V. Closed-loop electrolyte design for lithium-mediated ammonia synthesis. ACS. Cent. Sci. 2021, 7, 2073-82.
7. Suryanto, B. H. R.; Matuszek, K.; Choi, J.; et al. Nitrogen reduction to ammonia at high efficiency and rates based on a phosphonium proton shuttle. Science 2021, 372, 1187-91.
8. Fu, X.; Xu, A.; Pedersen, J. B.; et al. Phenol as proton shuttle and buffer for lithium-mediated ammonia electrosynthesis. Nat. Commun. 2024, 15, 2417.
9. Li, S.; Fu, X.; Nørskov, J. K.; Chorkendorff, I. Towards sustainable metal-mediated ammonia electrosynthesis. Nat. Energy. 2024, 9, 1344-9.
Cite This Article
How to Cite
Download Citation
Export Citation File:
Type of Import
Tips on Downloading Citation
Citation Manager File Format
Type of Import
Direct Import: When the Direct Import option is selected (the default state), a dialogue box will give you the option to Save or Open the downloaded citation data. Choosing Open will either launch your citation manager or give you a choice of applications with which to use the metadata. The Save option saves the file locally for later use.
Indirect Import: When the Indirect Import option is selected, the metadata is displayed and may be copied and pasted as needed.
About This Article
Copyright
Author Biographies
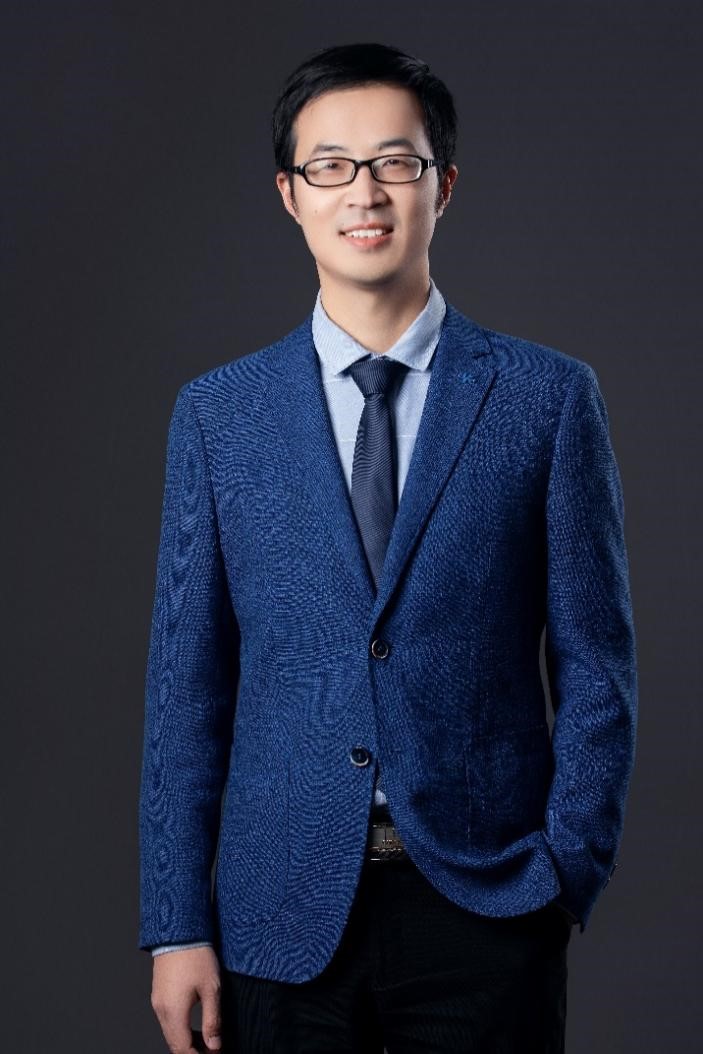
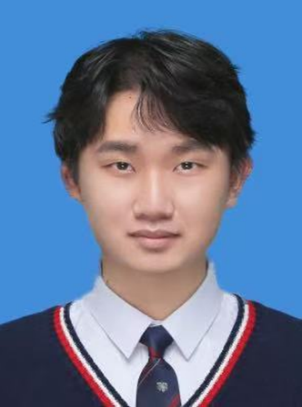
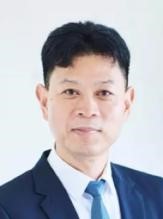
Comments
Comments must be written in English. Spam, offensive content, impersonation, and private information will not be permitted. If any comment is reported and identified as inappropriate content by OAE staff, the comment will be removed without notice. If you have any queries or need any help, please contact us at [email protected].