Achieving low carbon finance of the iron and steel industry in China
Abstract
As a key sector characterized by high energy consumption and carbon emissions, the low-carbon transition of the iron and steel industry is crucial for achieving national emission reduction targets. Although China's iron and steel industry has made some progress in reducing total energy demand through capacity control, it still faces challenges in terms of technological paths toward industrial decarbonization. Financing low-carbon technologies during the transition process will have to deal with significant funding gaps and a capital structure mismatched with technological development. We propose policies and methods to promote financing for the iron and steel industry’s low-carbon transition. We suggest strengthening public investment guidance, promoting green financial policies, and innovating carbon finance instruments. Emphasis should be placed on the role of policies such as green credit, carbon pricing, and R&D subsidies, while highlighting the importance of integrating financial policies with technology and flexibly adjusting them throughout the "financing lifecycle".
Keywords
EMISSION REDUCTION STRATEGIES FOR THE IRON AND STEEL INDUSTRY
The iron and steel industry is characterized by high energy consumption and carbon emissions, accounting for approximately 7% of global carbon dioxide emissions in 2019[1]. China produced over 1 billion tons of crude steel in 2023, accounting for more than half of the global total[2]. Steel production is primarily divided into the long process using blast furnace-basic oxygen furnace (BF-BOF) and the short process using scrap melting-electric arc furnace (Scrap-EAF). Compared to the former, the latter reduces energy consumption by 50%[3]. However, China's scrap utilization rate is currently low, and the long process still dominates the production structure, accounting for about 90% of production[4]. The iron and steel industry accounts for 14% of China's total energy consumption and contributes approximately 15% of the national emissions, second only to the power sector[5]. Therefore, strictly controlling carbon emissions in the iron and steel industry is crucial for achieving the nation's emission reduction targets[6]. The transition to sustainable development requires a fundamental shift in the flow of capital, moving away from unsustainable technologies toward sustainable ones[7-10].
Capacity control is considered a key measure for promoting the low-carbon development of China's iron and steel industry[11,12]. Many studies predict that China's crude steel demand will gradually decline to a stable level[13-16]. However, simply reducing production capacity and eliminating outdated capacities will not be sufficient to achieve the emission reductions needed to meet China's carbon neutrality goals. The iron and steel industry in China urgently needs to explore technological pathways for low-carbon transition[17-19].
First, adjusting the production structure is a long-term solution for reducing emissions in the iron and steel industry[6,20]. Currently, the capacity ratio of BF-BOF to Scrap-EAF in China's iron and steel industry is 9:1[21,22], with the proportion of EAF being lower than the global average[19]. Since it does not require coal and coke as reducing agents, producing crude steel by recycling scrap metal consumes less energy and significantly reduces carbon dioxide emissions[23,24].
Second, promoting the application of energy-saving technologies has become a significant measure for improving energy efficiency and reducing energy consumption in China’s iron and steel industry[25-27]. Technological improvements should be encouraged at all stages of steel production, such as pulverized coal injection into blast furnaces, coke dry quenching, and low-temperature sintering technologies[28-30]. Energy-saving technologies based on the BF-BOF process are among the most studied emission reduction solutions[31]. It is estimated that by adopting energy-saving technologies along the BF-BOF production pathway, energy consumption per ton of crude steel can be reduced by about 20%[1] and carbon dioxide emissions can be decreased by 22%[27].
Third, innovative technologies like carbon capture and storage (CCS) and direct reduced iron (DRI) are key to achieving carbon neutrality in China’s iron and steel industry[32]. Utilizing existing technologies can reduce emissions in China’s iron and steel industry by 54%, but it is insufficient to achieve carbon neutrality[17]. Combining traditional technologies with net-zero emission technologies, such as CCS and DRI, can fundamentally reduce carbon emissions and achieve an 80%-90% reduction, thereby fulfilling climate targets[17,33]. The developing direct reduced iron-electric arc furnace (DRI-EAF) technology, which bypasses the coke oven and sintering process, has the advantages of a shorter process, less pollution, and lower energy consumption. It is also not affected by the shortage of coking coal resources, making it highly promising for reducing energy consumption and emissions[34].
FINANCING CHALLENGES OF LOW-CARBON TRANSITION IN THE IRON AND STEEL SECTOR
The investment demand of low-carbon transition includes not only the initial costs of deploying mature technologies, as well as the costs of energy, raw materials, operations and maintenance, and financing, but also the research and development (R&D) expenses needed to develop new low-carbon technologies[31,35,36]. On one hand, the market share of mature low-carbon technologies such as Scrap-EAF is currently limited, and expanding their deployment requires substantial financial support[37]. On the other hand, new low-carbon technologies such as CCS are often capital-intensive and in an immature stage of development, thus requiring substantial investments in R&D[38].
The iron and steel industry is a typical capital-intensive sector facing long project cycles and high capital requirements in its green transition and upgrade. It often encounters challenges such as insufficient transformation momentum and financing constraints, with enterprises' own funds unable to meet the demands of green transition[39]. Due to a lack of sufficient financial support, some emission reduction solutions that are technically feasible and effective in addressing climate change risks have stalled, with financing difficulties seen as a key obstacle to their progress[40-42]. Additionally, different investments have varying risk-return characteristics, so investors may choose different investment methods based on differences in risk preferences, loss tolerance, and the tasks they undertake[43-45]. In the early stages of low-carbon transition, commercially immature technologies face significant uncertainty, making investors reluctant to allocate resources to these high-risk areas[10,46]. Therefore, there is a significant mismatch between the available sources of capital and the financial needs of the energy transition[40,47].
Compared to high-carbon assets, the low-carbon technologies required for the transformation of the iron and steel industry are typically considered to carry higher investment risks under current climate policies, leading to increased financing costs[8,38]. Moreover, low-carbon technologies are usually capital-intensive and require large investment amounts, reducing their competitiveness against fossil fuel technologies or existing high-energy-consuming technologies, thus facing a more challenging financing environment. It is estimated that nearly 3.5 trillion RMB will be needed for the iron and steel industry to reach peak carbon emissions, and achieving carbon neutrality will require an additional 19 trillion RMB[48]. Moreover, advanced steel production processes like Scrap-EAF, DRI-EAF, and decarbonization technologies like CCS have high costs due to expensive construction and the lack of economies of scale. In 2022, the unit cost of producing crude steel through Scrap-EAF in China was about $100/ton higher than that of BF-BOF[49]. Furthermore, in the context of supply-side structural reform, the financing expenditures for steel companies are indirectly increased by policies limiting the financing of new capacity in the industry, with financing expenditures being 30-82 basis points higher compared to non-overcapacity industries[50].
Regarding the choice of financing methods, Myers and Majluf's pecking order theory suggests that firms should prioritize internal financing, followed by debt financing, and equity financing as a last resort[51]. In the capital structure of China's iron and steel industry, debt financing outweighs equity financing[52]. According to China Stock Market & Accounting Research (CSMAR) data, the asset-liability ratio of 23 publicly listed steel production companies in 2022 was 56.64%, with some companies’ asset-liability ratios exceeding 70%. However, credit rationing assesses the relationship between risk and cost, and projects with excessive risk will not be financed through debt[53]. Therefore, less mature technologies require a lower debt-to-equity ratio, indicating that the current capital structure of the iron and steel industry does not align with the needs of low-carbon transition[10]. Moreover, insufficient financial policies have made it difficult for the steel industry to alter its existing capital structure, resulting in financing difficulties during its transformation process. Due to limited participation from entities offering green finance, the small number of specialized institutions providing green financial services, and the lack of diversified financing instruments, the development of financing to meet these needs remains inadequate. Additionally, inadequate information transparency and insufficient implementation of financial instrument standards further hinder progress[54,55]. As a result, China’s iron and steel industry has had limited involvement in green finance-related funding, making it difficult to effectively support the development of low-carbon technologies[48].
FINANCING PATHS FOR THE LOW-CARBON TRANSITION OF THE IRON AND STEEL INDUSTRY
Traditional financial markets are insufficient to meet the financing needs for the transition of the iron and steel industry, so the support brought by green finance policies must be considered. As the primary driver of green finance, the government’s involvement in green investment is crucial to the sustainability of companies’ green development[56]. Additionally, the stability of climate policies over time can better motivate stakeholders to invest early in low-carbon technologies[57,58]. Numerous studies have found that green finance policies can increase financial institutions' investment in companies with better environmental performance[56,59]. On one hand, this can help enterprises improve debt maturity mismatches[60], reduce financing costs for green projects[61], and provide more funds to conduct research and development, promoting green innovation[62,63]. Notably, among industries that are both heavily polluting and financially constrained, green finance policies have a more pronounced effect in promoting green innovation[64]. On the other hand, through debt financing constraints and equity financing constraints, green finance policies have also indirectly driven the transformation of heavily polluting enterprises[55,65]. Currently, in China, the development of green finance largely relies on the government and banks to provide green credit, bonds, and other financial services to reduce financing costs and mitigate investment risks for green industries[55]. It is noteworthy that, although the global green bond market is predominantly led by developed countries, China has emerged as the world's largest issuer of green bonds since 2022. In 2023, China issued USD 83.5 billion in green bonds, accounting for 14% of the global total, with Germany and the United States ranking second and third, respectively[66,67]. Nonetheless, introducing international linkages, with attracting international investors, can enhance the compliance of Chinese green bond issuers[65]. Furthermore, incorporating diversified financing channels like equity financing can alleviate the potential capital structure issues arising from green credit and green bonds, thereby reducing corporate debt pressure[55].
Carbon pricing policies targeting high-pollution industries such as the iron and steel industry, including carbon tax or carbon market schemes, can channel funds toward companies with better environmental benefits through information transmission and capital redistribution[68,69]. Emission trading schemes have been widely adopted in the European Union, the United States, Germany, China, and other regions[70]. However, in 2024, the carbon market price in China was much lower than that in the European Union's carbon market[70]. Promoting a steady increase in carbon prices will channel more funds to steel enterprises with good environmental performance[71]. Carbon financial derivatives, such as carbon forwards, carbon futures, and carbon options, have further increased the activity of the carbon trading market[72]. Additionally, a high-potential global steel sector emission reduction scheme indicated that revenue generated from these measures could offset the costs of the iron and steel industry’s transformation, supporting the effectiveness of carbon revenue recycling[73]. Moreover, the low-carbon research and development subsidies provided by the government, through the effect of research and learning, will effectively accelerate the cost reduction of low-carbon technologies in their early stages[74,75], thereby minimizing the costs associated with large-scale deployment[76]. Furthermore, project subsidies, tax credits, and the establishment of reasonable risk assessment metrics to overcome regulatory barriers are also key in fostering low-carbon innovation and development[47]. These carbon finance policies not only reduce the risks associated with the steel industry's transition but also enhance investment returns[77]. Specifically, on one hand, they lower the downside risks of low-carbon investments by simplifying project approvals, providing subsidies, investing in emission-reduction technology innovations, and utilizing equity financing to diversify funding channels. On the other hand, they increase the returns on low-carbon investments through mechanisms such as green certificates, carbon pricing, tax incentives, and concessional loans, while simultaneously reducing the profitability of high-carbon investments[78-80].
The financing needs for the low-carbon transition involve various investors and financial institutions and the funding mix required for technology development changes with the technology life cycle. Therefore, appropriate financing at different stages of the “financing lifecycle” is essential to facilitate the necessary speed of technology diffusion[81]. To effectively mobilize the needed capital, it is crucial to map the investment needs of technology to the appropriate sources of funding[45]. Public funds often finance mission-oriented R&D projects, which can give rise to entirely new industries[82]. As technology matures and risk becomes more manageable, uncertainty decreases, allowing for the use of cheaper sources of capital to fund more mature projects[40]. In this process, effectively mobilizing and directing public and private capital into climate-related projects is crucial[83]. Green finance policies can signal the market, attracting more private capital into green investment sectors through direct or indirect financing[77,84]. At the same time, the nature of investors changes as private capital's willingness to invest grows. The capital structure shifts from government-led investments to the involvement of venture capitalists, and then to investments from insurance companies, banks, pension funds, and even international financing channels[44,85]. During this process, policy frameworks evolve as well[86], shifting from funding R&D and deployment to establishing project certification standards, relevant regulations, and scientific performance evaluation mechanisms, thereby ensuring the efficient use of transformation funds[87,88]. This transition enables the development of technology from policy-driven to market-driven, completing the process where public capital mobilizes private investment. See Table 1 for an overview of key policy instruments and their definitions. Future research can further explore how to better align financial needs with technological development and investigate more effective policy measures to promote the widespread adoption of low-carbon technologies, thereby accelerating the iron and steel industry's low-carbon transition and providing strong support for China's carbon neutrality goals.
Policy instruments and their definitions
Policy | Definition |
Green bonds | Bonds that provide funding for projects that meet environmental-friendly standards[55] |
Carbon pricing | Measures to curb greenhouse gas emissions by charging for carbon dioxide emissions or providing incentives for emission reductions[89] |
Carbon derivatives | Derivative financial instruments based on carbon emission rights. Common types of carbon derivatives include futures, options, and swaps[90] |
Carbon revenue recycling | The recycling of revenues raised by governments through carbon pricing policies, including reductions in VAT, corporate and household income taxes, increased social security and welfare for low-income families, one-time transfer payments, investments in green project R&D and construction, etc.[91-94] |
R&D subsidies | Financial support for research and development of projects, such as grants[95] |
Concessional finance | Below-market-rates access to green finance[96] |
In summary, based on an overview of emission reduction technological pathways for China’s iron and steel industry, we analyze the financing challenges faced in the low-carbon transition, including significant funding gaps and mismatches between capital structure and technological development. To address these issues, we emphasize the government’s leading role in financing the low-carbon transition and highlight the roles of policies such as green credit, carbon pricing, and R&D subsidies in promoting low-carbon technology development and reducing financing costs to advance the iron and steel industry’s low-carbon development. Similar to other studies analyzing transition financing, we summarize the impacts of various financial instruments. However, we further propose specific financing strategies tailored to the unique technological and financing challenges of China's iron and steel industry. Additionally, we underscore the need for close integration of financial policies with technological development and the importance of flexible policy adjustments throughout the "financing lifecycle" to maximize the role of financial policies in facilitating the industry's transition. There is still room for improvement in the design and implementation details of existing carbon finance policies. Furthermore, balancing the long-term stability of policies with fiscal expenditure pressures represents a direction for further research. Meanwhile, the adaptability and fairness of carbon finance policies among enterprises of different sizes deserve attention, in order to explore more flexible policy tools, enhance policy precision and execution effectiveness, and strive to cover emission reductions across all industries. Additionally, it is also noteworthy how policy instruments can be leveraged to mobilize and guide private capital participation, realize the market-oriented development of low-carbon technologies, and promote efficient capital allocation and synergies through public-private partnership models.
DECLARATIONS
Acknowledgements
The authors acknowledge the financial support provided by the National Key Research and Development Program of China, the National Natural Science Foundation of China, and the Shanghai Pujiang Program.
Authors’ contributions
Made substantial contributions to the conception and design of the writing and revision: Liu, J.
Performed the collection of literature and writing: Jiang, X.
Availability of data and materials
Not applicable.
Financial support and sponsorship
This work was financially supported by the National Key Research and Development Program of China (2019YFC1908501), the National Natural Science Foundation of China (72004134, 72088101, 71810107001), and the Shanghai Pujiang Program (2020PJC075).
Conflicts of interest
Both authors declared that there are no conflicts of interest.
Ethical approval and consent to participate
Not applicable.
Consent for publication
Not applicable.
Copyright
© The Author(s) 2025.
REFERENCES
1. IEA. Iron and steel technology roadmap. 2020. Available from: https://www.iea.org/reports/iron-and-steel-technology-roadmap [Last accessed on 19 Mar 2025].
2. World steel in Figures. 2024. Available from: https://worldsteel.org/data/world-steel-in-figures-2024/ [Last accessed on 26 Mar 2025].
3. China Iron and Steel Association Research Group on the Development of Electric Arc Furnace Short Process Steelmaking. Research on the development of electric Arc furnace short process steelmaking in China. Metall. Manag. 2023, 20, 4-20. Available from: https://kns.cnki.net/kcms2/article/abstract?v=hW9FdoQEerqEvapyxeKkqpQu87yyvmY_qg8pqoHdL3-vH_Si2cyMUo8ugHMvVVcFeeDan9949eTRXXenpOz8pifitJkZkZmopZfDse0MBz54bmneOD-hn4Xi0v41ZPrKqdK3boG13FPsM3JHXHeKl9mjfzOm2ViAWNiLAM4gcGP0h1zPgaYg5me0dLICSNVr&uniplatform=NZKPT&language=CHS [Last accessed on 19 Mar 2025]
4. Steel statistical yearbook 2022. Available from: https://worldsteel.org/wp-content/uploads/Steel-Statistical-Yearbook-2022.pdf?utm [Last accessed on 19 Mar 2025].
5. Wang, K.; Che, L.; Ma, C.; Wei, Y. M. The shadow price of CO2 emissions in China’s iron and steel industry. Sci. Total. Environ. 2017, 598, 272-81.
6. Zhang, Q.; Xu, J.; Wang, Y.; et al. Comprehensive assessment of energy conservation and CO2 emissions mitigation in China’s iron and steel industry based on dynamic material flows. Appl. Energy. 2018, 209, 251-65.
7. International Energy Agency. IEA world energy outlook 2012. Available from: https://iea.blob.core.windows.net/assets/ebe15dfb-30c8-42cf-8733-672b3500aed7/WEO2012_free.pdf [Last accessed on 19 Mar 2025].
9. Steffen, B.; Schmidt, T. S. Strengthen finance in sustainability transitions research. Environ. Innov. Soc. Trans. 2021, 41, 77-80.
10. Sanders, M.; Serebriakova, A.; Fragkos, P.; Polzin, F.; Egli, F.; Steffen, B. Representation of financial markets in macro-economic transition models - a review and suggestions for extensions. Environ. Res. Lett. 2022, 17, 083001.
11. Li, W.; Lu, C.; Ding, Y.; Zhang, Y. The impacts of policy mix for resolving overcapacity in heavy chemical industry and operating national carbon emission trading market in China. Appl. Energy. 2017, 204, 509-24.
12. Yang, G.; Fukuyama, H.; Song, Y. Estimating capacity utilization of Chinese manufacturing industries. Soc. Econ. Plan. Sci. 2019, 67, 94-110.
13. Wang, P.; Li, W.; Kara, S. Cradle-to-cradle modeling of the future steel flow in China. Resour. Conserv. Recy. 2017, 117, 45-57.
14. Wang, Y.; Liu, J.; Tang, X.; Wang, Y.; An, H.; Yi, H. Decarbonization pathways of China's iron and steel industry toward carbon neutrality. Resour. Conserv. Recy. 2023, 194, 106994.
15. Wårell, L. Trends and developments in long-term steel demand - the intensity-of-use hypothesis revisited. Resour. Policy. 2014, 39, 134-43.
16. Xuan, Y.; Yue, Q. Forecast of steel demand and the availability of depreciated steel scrap in China. Resour. Conserv. Recy. 2016, 109, 1-12.
17. Yu, X.; Tan, C. China’s pathway to carbon neutrality for the iron and steel industry. Global. Environ. Chang. 2022, 76, 102574.
18. Ren, L.; Zhou, S.; Peng, T.; Ou, X. A review of CO2 emissions reduction technologies and low-carbon development in the iron and steel industry focusing on China. Renew. Sustain. Energy. Rev. 2021, 143, 110846.
19. Li, Z.; Hanaoka, T. Plant-level mitigation strategies could enable carbon neutrality by 2060 and reduce non-CO2 emissions in China’s iron and steel sector. One. Earth. 2022, 5, 932-43.
20. Li, Z.; Dai, H.; Song, J.; et al. Assessment of the carbon emissions reduction potential of China's iron and steel industry based on a simulation analysis. Energy 2019, 183, 279-90.
21. Sun, Q. Carbon dioxide emission scenario simulation and emission reduction path research of China's steel industry under the background of "Double Carbon”. 2023 Available from: https://kns.cnki.net/kcms2/article/abstract?v=uQzRnDzoTXH6CEHXv0aHDfamE4A6RxcO9vzM5VCfr8k32YzLsupkPWrgfTH5ds038FtcyKWR3PX6tSy4ogTA6UTB95plqZ7fjb-YnpYL94UJw42Qmjugcp1bn4i7Ra2YUNw72rSEC-mbaIK7cPjhk2c4VRhieBMn59OCAmtO32xPHqB0awDhU6x-g2AVcs1bCHRtCgx0iZMiK-gp4Bn0nQ==&uniplatform=NZKPT [Last accessed on 26 Mar 2025].
22. World steel in Figures. 2021. Available from: https://worldsteel.org/wp-content/uploads/2021-World-Steel-in-Figures.pdf?utm [Last accessed on 19 Mar 2025].
23. Kuramochi, T. Assessment of midterm CO2 emissions reduction potential in the iron and steel industry: a case of Japan. J. Clean. Prod. 2016, 132, 81-97.
24. Zhu, X.; Li, H.; Chen, J.; Jiang, F. Pollution control efficiency of China's iron and steel industry: evidence from different manufacturing processes. J. Clean. Prod. 2019, 240, 118184.
25. Long, W.; Wang, S.; Lu, C.; et al. Quantitative assessment of energy conservation potential and environmental benefits of an iron and steel plant in China. J. Clean. Prod. 2020, 273, 123163.
26. Hasanbeigi, A.; Jiang, Z.; Price, L. Retrospective and prospective analysis of the trends of energy use in Chinese iron and steel industry. J. Clean. Prod. 2014, 74, 105-18.
27. Wang, X.; Yu, B.; An, R.; Sun, F.; Xu, S. An integrated analysis of China’s iron and steel industry towards carbon neutrality. Appl. Energy. 2022, 322, 119453.
28. Wen, Z.; Meng, F.; Chen, M. Estimates of the potential for energy conservation and CO2 emissions mitigation based on Asian-Pacific Integrated Model (AIM): the case of the iron and steel industry in China. J. Clean. Prod. 2014, 65, 120-30.
29. Chen, W.; Yin, X.; Ma, D. A bottom-up analysis of China’s iron and steel industrial energy consumption and CO2 emissions. Appl. Energy. 2014, 136, 1174-83.
30. Ma, D.; Chen, W.; Yin, X.; Wang, L. Quantifying the co-benefits of decarbonisation in China’s steel sector: an integrated assessment approach. Appl. Energy. 2016, 162, 1225-37.
31. An, R.; Yu, B.; Li, R.; Wei, Y. M. Potential of energy savings and CO2 emission reduction in China’s iron and steel industry. Appl. Energy. 2018, 226, 862-80.
32. Li, W.; Zhang, S.; Lu, C. Research on the driving factors and carbon emission reduction pathways of China's iron and steel industry under the vision of carbon neutrality. J. Clean. Prod. 2022, 357, 131990.
33. Morfeldt, J.; Nijs, W.; Silveira, S. The impact of climate targets on future steel production - an analysis based on a global energy system model. J. Clean. Prod. 2015, 103, 469-82.
34. Shen, F.; Jiang, X.; Gao, Q.; Wei, G.; Zheng, H. Situation and prospect on production technology of direct reduction iron. Iron. Steel. 2017, 52, 7-12.
35. Moglianesi, A.; Keppo, I.; Lerede, D.; Savoldi, L. Role of technology learning in the decarbonization of the iron and steel sector: an energy system approach using a global-scale optimization model. Energy 2023, 274, 127339.
36. Ren, M.; Lu, P.; Liu, X.; et al. Decarbonizing China’s iron and steel industry from the supply and demand sides for carbon neutrality. Appl. Energy. 2021, 298, 117209.
37. Egli, F.; Steffen, B.; Schmidt, T. S. A dynamic analysis of financing conditions for renewable energy technologies. Nat. Energy. 2018, 3, 1084-92.
38. Iyer, G. C.; Clarke, L. E.; Edmonds, J. A.; et al. Improved representation of investment decisions in assessments of CO2 mitigation. Nat. Clim. Chang. 2015, 5, 436-40.
39. Wang, Z. Research on the path of enterprise performance improvement under the support of green financing - A case study of Shandong Iron and Steel Group. 2023. Available from: https://kns.cnki.net/kcms2/article/abstract?v=Kk8bzUe9ukr3cQD9kAOk46lkhTIByU34MgdpY-nEb-dPYqJvxb7qlWUBa5auloHW1vwbhR511CltiCKLReUo-LDwcl0D0_c-e3zH6FrE28lucqpsQwDTty5rmbdtk2QDPDRsmy5xWRKpQYB6D9Syew7JYBItMzPeOroAemjHFFwdYcAii1iZav-owJgvY7XRy5ayx0-P0Rc=&uniplatform=NZKPT&language=CHS [Last accessed on 19 Mar 2025]
40. Polzin, F.; Sanders, M.; Serebriakova, A. Finance in global transition scenarios: mapping investments by technology into finance needs by source. Energy. Econ. 2021, 99, 105281.
41. Pollitt, H.; Mercure, J. The role of money and the financial sector in energy-economy models used for assessing climate and energy policy. Clim. Policy. 2018, 18, 184-97.
42. Mercure, J.; Knobloch, F.; Pollitt, H.; Paroussos, L.; Scrieciu, S. S.; Lewney, R. Modelling innovation and the macroeconomics of low-carbon transitions: theory, perspectives and practical use. Clim. Policy. 2019, 19, 1019-37.
43. Polzin, F.; Sanders, M.; Täube, F. A diverse and resilient financial system for investments in the energy transition. Curr. Opin. Environ. Sustain. 2017, 28, 24-32.
44. Mazzucato, M.; Semieniuk, G. Financing renewable energy: who is financing what and why it matters. Technol. Forecast. Soc. Chang. 2018, 127, 8-22.
45. Steffen, B. The importance of project finance for renewable energy projects. Energy. Econ. 2018, 69, 280-94.
46. Campiglio, E.; Spiganti, A.; Wiskich, A. Clean innovation, heterogeneous financing costs, and the optimal climate policy mix. J. Environ. Econo. Manag. 2024, 128, 103071.
47. Polzin, F.; Sanders, M. How to finance the transition to low-carbon energy in Europe? Energy. Policy. 2020, 147, 111863.
48. Wang, N. Research on financial support for the green and low-carbon transition of the steel industry under the dual carbon goals. Hebei. Financ. 2021, 10, 15-16, 20.
49. Transition Asia. Green steel premium: pricing and market development in China. 2023. Available from: https://transitionasia.org/wp-content/uploads/2023/02/TA-GreenSteelPremium-China_Feb2023-3.pdf?utm [Last accessed on 19 Mar 2025].
50. Wu, X. Selection of financing mode for iron and steel enterprises under the background of supply-side structural reform. 2019. Available from: https://kns.cnki.net/kcms2/article/abstract?v=hW9FdoQEerokow44JaRM7UU2Ko2E3oOptnYE2esm3RGco175TmfXYgAYfnK19pTsE4CuBOejhOZ2SAXG_MBqal_QIAsKD8TYUwaJttvpXz9Fk9F8Y45ExO-qAXERcfCPtCjuUqZxosRJGATTssjaCTEhpBCi9oe0rwpPwSqCrTiapm5tosm1KBSuW2SqtOGjXlO1v5q3IZc=&uniplatform=NZKPT&language=CHS [Last accessed on 19 Mar 2025].
51. Myers, S. C.; Majluf, N. S. Corporate financing and investment decisions when firms have information that investors do not have. J. Financ. Econ. 1984, 13, 187-221.
52. Mo, J.; Agnolucci, P.; Jiang, M.; Fan, Y. The impact of Chinese carbon emission trading scheme (ETS) on low carbon energy (LCE) investment. Energy. Policy. 2016, 89, 271-83.
53. Stiglitz, J. E.; Weiss, A. Credit rationing in markets with imperfect information. The American Economic Review; 1981, pp. 393-410. Available from: https://www.jstor.org/stable/1802787?utm [Last accessed on 19 Mar 2025]
55. Lee, J. W. Green finance and sustainable development goals: the case of China. J. Asian. Financ. Econ. Bus. 2020, 7, 577-86.
56. Yang, W. E.; Lai, P. W.; Han, Z. Q.; Tang, Z. P. Do government policies drive institutional preferences on green investment? Evidence from China. Environ. Sci. Pollut. Res. 2023, 30, 8297-316.
57. Kalkuhl, M.; Steckel, J. C.; Edenhofer, O. All or nothing: climate policy when assets can become stranded. J. Environ. Econ. Manag. 2020, 100, 102214.
58. Rozenberg, J.; Vogt-Schilb, A.; Hallegatte, S. Instrument choice and stranded assets in the transition to clean capital. J. Environ. Econ. Manag. 2020, 100, 102183.
59. Monasterolo, I.; de Angelis, L. Blind to carbon risk? An analysis of stock market reaction to the Paris agreement. Ecol. Econ. 2020, 170, 106571.
60. Wang, Y. Greenwashing or green evolution: can transition finance empower green innovation in carbon-intensive enterprise? Int. Rev. Financ. Anal. 2025, 97, 103826.
61. Shi, J.; Yu, C.; Li, Y.; Wang, T. Does green financial policy affect debt-financing cost of heavy-polluting enterprises? An empirical evidence based on Chinese pilot zones for green finance reform and innovations. Technol. Forecast. Soc. Chang. 2022, 179, 121678.
62. Yang, Y.; Su, X.; Yao, S. Can green finance promote green innovation? The moderating effect of environmental regulation. Environ. Sci. Pollut. Res. Int. 2022, 29, 74540-53.
63. Xu, X. Does green finance promote green innovation? Evidence from China. Environ. Sci. Pollut. Res. Int. 2023, 30, 27948-64.
64. Shi, R.; Zhou, H.; Li, X.; Miao, X.; Zhao, X. Green finance, investor preferences, and corporate green innovation. Financ. Res. Lett. 2024, 66, 105676.
65. Tian, C.; Li, X.; Xiao, L.; Zhu, B. Exploring the impact of green credit policy on green transformation of heavy polluting industries. J. Clean. Prod. 2022, 335, 130257.
66. Filipava, L.; Murshudli, F. The development of the global green finance market: the role of banks and non-banking institutional investors. In: Naifar N, Elsayed A, editors. Sustainable Finance. Cham: Springer; 2023.
67. CBI. Sustainable debt global state of the market. 2023. Available from: https://www.climatebonds.net/files/reports/cbi_sotm23_02h.pdf [Last accessed on 19 Mar 2025].
68. Faccini, R.; Matin, R.; Skiadopoulos, G. Dissecting climate risks: are they reflected in stock prices? J. Bank. Financ. 2023, 155, 106948.
69. Wen, F.; Wu, N.; Gong, X. China's carbon emissions trading and stock returns. Energy. Econ. 2020, 86, 104627.
70. World Bank Group. State and trends of carbon pricing 2024. Available from: https://hdl.handle.net/10986/41544 [Last accessed on 26 Mar 2025].
71. Pan, J.; Cross, J. L.; Zou, X.; Zhang, B. To tax or to trade? A global review of carbon emissions reduction strategies. Energy. Strategy. Rev. 2024, 55, 101508.
72. Zhou, K.; Li, Y. Carbon finance and carbon market in China: progress and challenges. J. Clean. Prod. 2019, 214, 536-49.
73. Sun, Y.; Tian, S.; Ciais, P.; Zeng, Z.; Meng, J.; Zhang, Z. Decarbonising the iron and steel sector for a 2 °C target using inherent waste streams. Nat. Commun. 2022, 13, 297.
74. Badgett, A.; Ruth, M.; James, B.; Pivovar, B. Methods identifying cost reduction potential for water electrolysis systems. Curr. Opin. Chem. Eng. 2021, 33, 100714.
75. Tsiropoulos, I.; Tarvydas, D.; Zucker, A. Cost development of low carbon energy technologies: Scenario-based cost trajectories to 2050, 2017 edition. Available from: https://publications.jrc.ec.europa.eu/repository/bitstream/JRC109894/cost_development_of_low_carbon_energy_technologies_v2.2_final_online.pdf?utm [Last accessed on 19 Mar 2025].
76. McDonald, A.; Schrattenholzer, L. Learning curves and technology assessment. Int. J. Technol. Manag. 2002, 23, 718-45.
77. Polzin, F.; Egli, F.; Steffen, B.; Schmidt, T. S. How do policies mobilize private finance for renewable energy? - A systematic review with an investor perspective. Appl. Energy. 2019, 236, 1249-68.
78. Corrocher, N.; Cappa, E. The role of public interventions in inducing private climate finance: an empirical analysis of the solar energy sector. Energy. Policy. 2020, 147, 111787.
79. Weyant, J. P. Accelerating the development and diffusion of new energy technologies: beyond the “valley of death”. Energy. Econ. 2011, 33, 674-82.
80. Curtin, J.; Mcinerney, C.; Gallachóir, B. Ó. Financial incentives to mobilise local citizens as investors in low-carbon technologies: a systematic literature review. Renew. Sustain. Energy. Rev. 2017, 75, 534-47.
81. Polzin, F.; Sanders, M.; Steffen, B.; et al. The effect of differentiating costs of capital by country and technology on the European energy transition. Clim. Chang. 2021, 167, 3163.
82. Foray, D.; Mowery, D. C.; Nelson, R. R. Public R&D and social challenges: what lessons from mission R&D programs? Res. Policy. 2012, 41, 1697-702.
83. Bhandary, R. R.; Gallagher, K. S.; Zhang, F. Climate finance policy in practice: a review of the evidence. Clim. Policy. 2021, 21, 529-45.
84. Khurshed, A.; Paleari, S.; Pande, A.; Vismara, S. Transparent bookbuilding, certification and initial public offerings. J. Financ. Markets. 2014, 19, 154-69.
85. Mazzucato, M.; Penna, C. C. Beyond market failures: the market creating and shaping roles of state investment banks. J. Econ. Policy. Reform. 2016, 19, 305-26.
86. Rogge, K. S.; Reichardt, K. Policy mixes for sustainability transitions: an extended concept and framework for analysis. Res. Policy. 2016, 45, 1620-35.
87. Delmas, M. A.; Montes-Sancho, M. J. U.S. state policies for renewable energy: context and effectiveness. Energy. Policy. 2011, 39, 2273-88.
88. Carley, S. State renewable energy electricity policies: an empirical evaluation of effectiveness. Energy. Policy. 2009, 37, 3071-81.
89. UNFCCC. About carbon pricing. 2024. Available from: https://unfccc.int/about-us/regional-collaboration-centres/the-ciaca/about-carbon-pricing [Last accessed on 19 Mar 2025].
90. Kaifeng, L.; Chuanzhe, L. Construction of carbon finance system and promotion of environmental finance innovation in China. Energy. Procedia. 2011, 5, 1065-72.
91. Shaw, D.; Fu, Y. Climate clubs with tax revenue recycling, tariffs, and transfers. Clim. Chang. Econ. 2020, 11, 2040008.
92. Aleluia Reis, L.; Vrontisi, Z.; Verdolini, E.; Fragkiadakis, K.; Tavoni, M. A research and development investment strategy to achieve the Paris climate agreement. Nat. Commun. 2023, 14, 3581.
93. Woerner, A.; Imai, T.; Pace, D. D.; Schmidt, K. M. How to increase public support for carbon pricing with revenue recycling. Nat. Sustain. 2024, 7, 1633-41.
94. Mohammadzadeh Valencia, F.; Mohren, C.; Ramakrishnan, A.; Merchert, M.; Minx, J. C.; Steckel, J. C. Public support for carbon pricing policies and revenue recycling options: a systematic review and meta-analysis of the survey literature. NPJ. Clim. Action. 2024, 3, 153.
95. Eyraud, L.; Clements, B.; Wane, A. Green investment: trends and determinants. Energy. Policy. 2013, 60, 852-65.
Cite This Article

How to Cite
Jiang, X.; Liu, J. Achieving low carbon finance of the iron and steel industry in China. Carbon Footprints 2025, 4, 9. http://dx.doi.org/10.20517/cf.2024.55
Download Citation
Export Citation File:
Type of Import
Tips on Downloading Citation
Citation Manager File Format
Type of Import
Direct Import: When the Direct Import option is selected (the default state), a dialogue box will give you the option to Save or Open the downloaded citation data. Choosing Open will either launch your citation manager or give you a choice of applications with which to use the metadata. The Save option saves the file locally for later use.
Indirect Import: When the Indirect Import option is selected, the metadata is displayed and may be copied and pasted as needed.
About This Article
Special Issue
Copyright
Data & Comments
Data
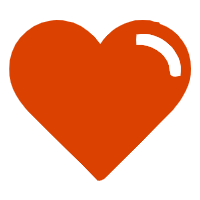
Comments
Comments must be written in English. Spam, offensive content, impersonation, and private information will not be permitted. If any comment is reported and identified as inappropriate content by OAE staff, the comment will be removed without notice. If you have any queries or need any help, please contact us at [email protected].