Estimation of the total carbon sequestration potential of coconut-gliricidia mixed cropping systems in Sri Lanka
Abstract
Offsetting carbon footprints by sequestering carbon through plant biomasses has become a key concern under modern thinking on climate change mitigation. This study aimed to estimate the carbon sequestration capacities of coconut-based gliricidia mixed cropping systems in Sri Lanka and, importantly, to develop an allometric model for non-destructive estimation of carbon. Five major coconut age groups and four major gliricidia age groups were selected to estimate the total carbon stock. The age groups of coconut considered were 10, 20, 30, 40, and
Keywords
INTRODUCTION
Climate change is among the most urgent global challenges today, and carbon sequestration has become a key strategy for mitigating its effects[1,2]. Carbon sequestration is the process of keeping CO2 in the atmosphere for a long time by using terrestrial ecosystems, such as forests, soils, or agricultural systems[3]. This process is considered to play a key role in regulating the Earth's carbon cycle and it has received a lot of attention due to its potential to reduce the rising levels of greenhouse gases (GHGs) in the atmosphere. Sri Lanka, an island nation in the Indian Ocean, is especially susceptible to the effects of climate change, such as rising sea levels, shifting rainfall patterns, and the growing frequency and severity of extreme weather events[4]. As a signatory to the Paris Agreement, Sri Lanka has pledged to cut down on GHG emissions and increase its carbon sinks[5]. In this context, exploring sustainable agricultural practices that can contribute to carbon sequestration has become a priority[6].
Thus, a specific strategy, such as the use of coconut-gliricidia mixed cropping systems, which offers numerous advantages - including good soil fertility, balance of nature, and good economic returns to smallholder farmers - has been practiced in Sri Lanka[7-9]. Gliricidia is a nitrogen-fixing leguminous tree that is often intercropped with coconut plantations to provide shade and improve soil health[10]. Carbon sequestration is the possibility of integrating coconut and gliricidia in a mixed cropping system in various ways[11-13]. Coconut palms, which have a perennial nature and a dense root system, can accumulate carbon in their biomass and enhance soil carbon through the decomposition of leaf litter and root exudates[14]. Additionally, gliricidia, a fast-growing tree species, can sequester carbon in its aboveground and belowground biomass, while also enhancing soil organic matter through the decomposition of its nitrogen-rich litter and pruned biomass[15].
Different approaches have been created to measure carbon capture in multi-purpose tree planting, such as allometric equations, remote sensing techniques, and process-based models[16]. Allometric equations link easily measurable tree characteristics, such as diameter at breast height (DBH) and height, to estimates of biomass and carbon content[17]. The application of such an allometric equation proves a veritably convenient and alternative technique to the destructive sampling of the carbon stock[18]. Deriving from the empirical link among dimensions of trees and their carbon content, this method enables producing data with high accuracy, as well as the ones that can be reproduced, while contributing to our understanding of carbon capture processes occurring within the interpreted ecosystem[19]. Process-based models, on the other hand, simulate the dynamics of carbon fluxes and pools within the system, considering various environmental and management factors[20]. Estimating the total carbon sequestration potential of coconut-gliricidia mixed cropping systems in Sri Lanka requires a multidisciplinary approach, combining field measurements, laboratory analyses, and modeling techniques.
Calculating the total carbon sequestration capacity of coconut-gliricidia mixed cropping systems is imperative for assessing their contribution to climate change mitigation, as well as informing policy decisions related to sustainable land management and their practices[21,22]. This study aims to quantify the amount of carbon that can be stocked by the coconut-gliricidia mixed cropping systems rather than coconut monocropping systems and develop an allometric model for non-destructive estimation of carbon stock. It involves assessing the carbon stocks in various components of the system, including the coconut stands, gliricidia stands, ground cover, and soils. By estimating the total carbon sequestration potential, this study contributes to the development of strategies for enhancing carbon sinks in agricultural landscapes. It also supports the implementation of climate-smart agriculture practices and the promotion of agroforestry systems as a means of mitigating greenhouse gas emissions while ensuring food security and sustainable livelihoods for farming communities in Sri Lanka[6,23].
METHODOLOGY
Location of the experiment
The experiment was conducted at the Coconut Research Institute of Sri Lanka, Lunuwila
Figure 1. Experimental location. Created with QGIS (https://www.qgis.org/).
Data collection
Carbon stock of coconut
Coconut stands belonging to age groups of 10, 20, 30, 40, and 50 years were selected. These particular age categories define the most important phases in coconut palm development, which in turn enables a sequential study of the plant morphological changes, biomass production, and yield parameters[27]. Since coconut palms are long-lived plants, by studying palms at early productive ages (10 years), middle-aged palms (20-30 years), and senescent palms (40-50 years), researchers are able to unravel complex patterns of vegetative development, photosynthesis, nutrient partitioning, and reproduction[27,28]. An orderly selection of age groups enables the quantitative evaluation of physiological processes, such as alterations in the structure of the leaves, root formation, and carbon sequestration.
A 0.2-hectare plot was designated for each age category, with an average density of approximately 32 coconut palms per plot. From each age category, 09 palms were randomly selected to assess aboveground and belowground carbon stocks. This sample size corresponds to approximately 30% of the total palms within the specified area for each age category. Overall, a total of 45 palms, spanning all age groups, were sampled and analyzed for carbon content. The selected trees were chosen through random sampling within each age category, ensuring a diverse and unbiased representation of the coconut palm population. The nuts, stems, and fronds were considered the aboveground section of the coconut palm, while the roots were considered the belowground section. The following allometric equations were used to determine the total carbon stock in each component.
Carbon stock of the aboveground section
Aboveground carbon stock was determined using several equations. This approach breaks down the palm into its main components - nuts, stem, and fronds and calculates the carbon content for each part separately before combining them for a total estimate.
The dry weight of each nut was calculated using an equation developed for the tall variety of coconut[29].
where: DM - Total dry matter content of the nut (g); L - Vertical length of the nut along the vertical axis (cm).
To estimate the total dry weight of nuts on a palm, the average weight of nuts in a bunch was calculated. This average was multiplied by the number of nuts in the bunch. The weights of all bunches were summed to get the total dry weight of nuts per palm. This method allowed for a non-destructive estimation of the nut component of the aboveground carbon stock.
The carbon content of the stem part was calculated using equations from Friend & Corley[30]. For that, the stem density was estimated based on the age of the plantation. The stem dry weight of a palm was calculated by multiplying the stem's volume by its density (Friend & Corley[30], assuming the stem was cylindrical without considering its tapering towards the top.
where: D - Density (g cm-3); t - Age after field planting (years).
The dry weight of a frond was calculated by measuring the cross-sectional area of the petiole at the point where the lowest leaflet is attached, taking both the width and depth of the petiole on the coconut leaves into account[30]. The total dry weight of the crown was then determined by multiplying the dry weight of a single frond by the total number of fronds in the canopy.
where: W - Dry weight of the frond (g); C - Width × depth of the petiole.
The carbon content of the dry mass was assumed to be 0.5 g of carbon for 1 g of dry biomass[31,32].
Carbon stock of the belowground section
For the determination of the root carbon stock, root samples were collected by excavating a triangular pit in 1/8th of the root zone [Figure 2]. Soil particles were removed using a pressurized water gun and roots were collected to analyze the carbon content. The height of the coconut palms was measured utilizing a clinometer, a standard tool for height estimation in field studies. This device allows for accurate determination of tree height by measuring the angle between the observer's line of sight to the top of the tree and the ground.
Figure 2. 1/8th of the root zone in coconut palm. Created with GIMP (https://www.gimp.org/) and Canva (https://www.canva.com/free/).
Using the height of the coconut palms, the allometric relationship was created to accurately estimate the amount of carbon stocks in the roots of the entire palm[33].
where: R - Root Carbon Stock of the palm; h - Height of the palm (m).
The value per palm was multiplied by the number of existing coconut palms in one hectare to calculate the total carbon stock per hectare.
Taking 1/8th of a coconut palm root system can serve as a representative sample due to the root system's inherently uniform and symmetrical growth pattern. Coconut palms develop a fibrous, adventitious root system that grows radially and evenly from the base of the stem in all three dimensions, with the main roots being uniform in size[34]. While the total number of roots can vary from 1,500 to 7,000 (or rarely up to 11,360) depending on the palm’s age, bole girth, and soil characteristics, their distribution remains generally symmetrical[35]. The roots typically spread laterally up to 6 m from the base and can reach depths of
Carbon stock of gliricidia
Gliricidia stands belonging to age groups of 5, 10, 15, and 20 years were selected, and 5 palms were randomly selected from each age category to estimate the total above- and belowground carbon stock. Leaves, branches, and the main stem were considered for the aboveground sections, and roots were considered for the belowground section. All plant samples were taken destructively and analyzed by the wet oxidation method to calculate the carbon content[37].
Carbon stock of soil
Soil samples were randomly collected from the middle of the coconut square and the manure circle at a depth of 0-30 cm, using the soil core method with 10 replicates [Figure 3]. These samples represented a
Figure 3. Visual concept of coconut center square and manure circle. Created with GIMP (https://www.gimp.org/) and Canva (https://www.canva.com/free/).
Carbon stock in ground cover
The botanical composition of the ground cover was divided into two major categories: (1) live ground cover; and (2) dead ground cover. The live ground cover was again divided into two main components: aboveground (aboveground parts of grasses, legumes, broad leaves, and sedges) and belowground (roots of grasses, legumes, broad leaves, and sedges). The litter layer of the ground cover was considered the dead ground cover. A quadrant with a 1 m × 1 m area was used to take the samples of ground cover, and 10 random samples were taken from the area except the manure circle area. It is important to note that in well-managed plantations, the manure circle is typically not covered with grass; thus, grass cover in the manure circle was not included in the calculations. The samples were collected from the 30-year-old age category of coconut monocropping systems and 30-year-old coconut with 10-year-old gliricidia mixed cropping systems. The carbon contents of the dead ground cover, aboveground live cover, and belowground cover were determined separately using the wet oxidation method proposed by Walkley & Black[37].
Statistical analysis
All the statistical analyses were conducted with R (4.1.3) statistical software to identify statistically significant variations among the mean carbon stock across different criteria. One-way Analysis of Variance (ANOVA) was applied at a 5% level of significance. Subsequently, Tukey's honestly significant difference (HSD) test was used to conduct pairwise comparisons and identify specific differences between the means. Regression analysis was employed to develop an allometric model to estimate the total carbon stock of coconut and gliricidia.
RESULTS AND DISCUSSION
Carbon stock of coconut
Table 1 clearly outlines the growth characteristics of palm trees across five age categories: 10, 20, 30, 40, and
Mean heights and root dry weights of the palm for each age group
Age groups | Mean palm height (m) | Mean root dry biomass (kg) |
10 years | 4.6 | 91.48 |
20 years | 8.6 | 174.46 |
30 years | 13 | 200.02 |
40 years | 13.33 | 227.65 |
50 years | 14.8 | 291.48 |
Variations in the carbon stock among coconut palms of five different age categories were investigated in this study. Results showed carbon stocks in coconut fronds declined significantly with the maturity (P < 0.05). The highest carbon stock of 8.92 Mg[C]ha-1 was observed in the 10-year age group, while the lowest value of 3.38 Mg[C]ha-1 was recorded in the 50-year age group [Figure 4A]. According to previous studies, this decline can happen due to the elongation of leaves up to 10 years followed by progressive reduction, a decrease in the length and width of the longest leaflets with age, and a decline in the total number of leaflets in mature green leaves after 20 years[38]. Significant differences in the total carbon stock of the stems were notably observed across the five age categories. The highest carbon stock of 42.99 Mg[C]ha-1 was observed in the 50-year age group, while the lowest value of 7.02 Mg[C]ha-1 was recorded in the 10-year age group. However, there was no notable significant difference (P < 0.05) between the 40 and 50-year-old palms nor between the 10 and 20-year-old ones [Figure 4B].
Figure 4. Variation of coconut carbon stock with different age groups. (A) Carbon stock of fronds, (B) Carbon stock of stems, (C) Carbon stock of nuts, (D) Carbon stock of roots, (E) Total carbon stock of the palm (Means that do not share a letter are significantly different at P < 0.05). The assumption: There are 158 coconut palms in one hectare.
Carbon stock in coconut stems exhibited a consistent increase with age, with a notable accumulation observed between 20 and 40 years. Raveendra et al. reported similar carbon stock values for stem
Root carbon stocks also varied among the five age categories, with 30, 40, and 50-year-old palms exhibiting no statistical difference. The highest carbon content of 17.85 Mg[C]ha-1 was observed in the
Carbon stock of gliricidia
This study examined the variation in carbon stock across different age categories of gliricidia plants. Significant differences
Figure 5. Variation of gliricidia carbon stock with different age groups. (A) Leaves carbon stock, (B) Branches carbon stock, (C) Stem carbon stock, (D) roots carbon stocks, (E) Total plant carbon stock (Means that do not share a letter are significantly different at
The carbon stock of gliricidia main stem also exhibited significant differences [P < 0.05] between the 5 to 10-year age groups and the 15 to 20-year age groups [Figure 5C]. The highest carbon stock of
Soil carbon stock
According to the preliminary study, the mean values of soil characteristics showed minor variations across all selected research plots. The soil is slightly acidic, with a pH ranging from 6.0 to 6.5[41]. Electrical conductivity is low (0.02-0.03 S/m), indicating minimal salinity[42]. The bulk density ranged between 1.70 and 1.75 g/cm³, suggesting a moderately compact soil structure[43]. In terms of nutrients, total nitrogen levels range from 0.05% to 1.5%, phosphorus is moderate at 6-7 mg/kg, and potassium is relatively low at 0.02% to 0.035%. Soil texture analysis classifies the soil as sandy loam, primarily composed of sand (70%-75%), with smaller proportions of clay (20%-25%) and silt (8%-9%)[44]. The total carbon stock in a one-hectare coconut monocropping system was 13.39 Mg[C]ha-1 [Table 2]. In contrast, a mixed cropping system combining coconuts with Gliricidia showed a higher carbon stock of 14.49 Mg[C]ha-1 [Table 2]. According to the results, the total carbon stock in the coconut-gliricidia mixed cropping system was higher than in the coconut monocropping system. However, there was no significant difference in soil carbon stocks between the monocropping and mixed cropping systems. Nevertheless, a significant difference was observed in soil carbon stocks between the manure circles in both collection sites. Saha et al.. found that with increasing depth, carbon stocks declined, but no significant differences were observed between the topsoil layer and subsoil layer of coconut fields[45]. A study by Ranasinghe and Thimothias[46] found that soil carbon stocks in monoculture coconut systems varied between 14.15 to 44.17 Mg[C]ha-1. The differences observed between the studies might be attributed to variations in microclimate, soil, and related conditions across the study sites, as they were conducted in different agroecological areas and soil series. Soil properties such as texture, pH, bulk density, and nutrient levels create a complex framework that influences carbon dynamics[47]. Sandy soils may have a problem with carbon storage because the carbon decomposes faster than in other soils, while clay soils have high carbon storage capacity[48]. Optimal soil pH and nutrient availability promote microbial activity and plant productivity, which drive carbon inputs.
Soil carbon stock in different cropping systems
Cropping system | Soil carbon stock (Mg[C]ha-1) | |
In the manure circle | In the center square | |
Coconut monocropping system | 3.05 | 10.34 |
Coconut-gliricidia mixed cropping system | 3.22 | 11.27 |
Carbon stock in ground cover
In both the coconut monocropping and coconut-gliricidia mixed cropping systems, four main categories within the botanical composition of the ground cover: grasses, legumes, broad leaves, and sedges were identified. In the coconut monocropping system, the ground cover was predominantly composed of grasses, making up 78% of the total, followed by 10% legumes, 8% broad leaves, and 4% sedges [Figure 6A]. Under the mixed cropping system, the ground cover showed 75% grasses, 13% legumes, 8% broad leaves, and 4% sedges [Figure 6B]. Grasses were the dominant category at both sites compared to the other three categories. Additionally, sedges comprised the lowest composition at both respective sites. Across all collected samples, Pueraria phaseoloides was the most prominent legume species.
Figure 6. Botanical composition of the experimental field. (A) Coconut monocropping system; (B) Coconut gliricidia mixed cropping system.
According to the results, in coconut monocropping systems, the live aboveground ground cover contained
Figure 7. Total ground cover carbon stock with major components. (A) coconut monocropping system; (B) coconut gliricidia mixed cropping system. Created with GIMP (https://www.gimp.org/) and Canva (https://www.canva.com/free/).
Total agroecosystem carbon stock: 30-year-old coconut monocropping system vs. 30-year-old coconut with 10-year-old gliricidia mixed cropping system
Gliricidia as an intercrop is recommended to be introduced after 20 years of coconut plantations due to canopy shading. Therefore, when the coconut is 30 years old, the gliricidia crop reaches an age of
Figure 8. Visual concept of component-based carbon stock. (A) 30-year-old coconut monocrop system; (B) 30-year-old coconut and 10-year-old gliricidia mixed cropping systems. Created with GIMP (https://www.gimp.org/) and Canva (https://www.canva.com/free/).
Whereas the carbon stock of the 30-year-old coconut monocropping system was 67.76 Mg[C]ha-1 with a carbon sequestration rate of 2.25 Mg[C]ha-1yr-1 [Figure 9A], the carbon stock of the 30-year-old coconut-gliricidia mixed cropping system was 114.83 Mg[C]ha-1 with a carbon sequestration rate of
Figure 9. Visual concept of total carbon stock of (A) 30-year-old coconut monocrop systems and (B) 30-year-old coconut and 10-year-old gliricidia mixed cropping systems. Created with GIMP (https://www.gimp.org/) and Canva (https://www.canva.com/free/).
According to Table 3, the 30-year-old coconut monocrop agroecosystem abated 8.23 Mg[CO2]ha-1yr-1, while the 30-year-old coconut with 10-year-old gliricidia mixed cropping system abated 25.03 Mg[CO2]ha-1yr-1. The coconut-gliricidia mixed cropping system resulted in approximately three times greater CO2 sequestration rate compared to the coconut monocropping system. A study by Rathnayake & Mizunoya[49] showed a carbon sequestration rate of 4.89 Mg[C]ha-1yr-1 and an abated CO2 value of 17.78 Mg[CO2]ha-1yr-1 in a coconut agroecosystem. The difference in these values from our study is due to the difference in the age group of coconut and the differences in carbon stock in other ecosystem factors.
Summary of total carbon stock and total abated CO2 in 30-years-old coconut monocropping system and 30-years-old coconut with 10-years-old gliricidia in a coconut-gliricidia mixed cropping system
Cropping system | Crop carbon stock (Mg[C]ha-1) | Soil carbon stock (Mg[C]ha-1) | Ground cover carbon stock (Mg[C]ha-1) | Total carbon stock (Mg[C]ha-1) | Total carbon sequestration rate (Mg[C]ha-1yr-1) | Total abated CO2 (Mg[CO2]ha-1yr-1) |
Coconut monocropping | 53.13 | 13.39 | 1.24 | 67.76 | 2.25 | 8.23 |
Coconut-gliricidia mixed cropping | 53.13 + 46.16 | 14.49 | 1.05 | 114.83 | 6.84 | 25.03 |
Developed allometric models and validation
An allometric equation was developed to accurately estimate the total carbon stock in coconut palms. This equation, expressed as TC = (0.0275 × h) + 0.0039, where “TC” represents total carbon stock (Mg[C]palm) and "h" denotes plant height in meters, demonstrated a robust fit with an impressive R2 value of 0.92. This high R2 value affirms the model's efficacy and accuracy, indicating that plant height is a significant determinant in estimating carbon storage within coconut palm agroecosystems. Growers can apply this allometric model to accurately estimate the carbon sequestration potential of their coconut palm plantations. By measuring the height of their coconut palms, they can use the equation to determine the total carbon stock within their agroecosystems. This practical tool not only aids researchers in studying carbon dynamics but also enables practitioners to implement more effective carbon management and sustainability practices in their operations.
The derived equation thus serves as a valuable resource for both scientific research and practical application, providing a reliable means to evaluate and enhance the carbon sequestration capabilities of coconut palm agroecosystems.
A robust allometric equation was developed to accurately estimate the total carbon stock in gliricidia plants. The equation, represented as TG = (0.0011 × g) - 0.0095, where “TG” represents the total carbon stock (Mg[C]plant) in gliricidia and “g” signifies the girth taken at 30 cm from ground level plant height in cm, showcased a robust fit with an impressive R square (R2) value of 0. 90, affirming its efficacy as a predictive model. This equation provides a reliable framework for predicting carbon sequestration potential based on diameter at 30 cm gliricidia height, offering valuable insights for carbon accounting and management strategies in agroforestry systems. In allometric models, the R2 is a statistical statistic that denotes the proportional part of the complete variance of the dependent variable explained by independent variables. In models with higher R2 values, the data fit better, indicating that the model is more accurate[50,51].
CONCLUSION
The coconut-gliricidia intercropped system, while providing a promising agroforestry practice in Sri Lanka for carbon sequestration and mitigation of climate change effects, came out as a winner. The research results noted that a 30-year-old coconut plantation intercropped with a 10-year-old gliricidia stand sequestered an incredible amount of 69.46% higher carbon stock compared to a 30-year-old monocropping system based on coconut trees. The above-mentioned major disparity brings insight into the symbiotic benefits of combining coconut and gliricidia species by using their own soil carbon storage (biomass for soil). The research design of this study, which considered both groundcover and aboveground biomass, in conjunction with the soil, is a more holistic approach to understanding the carbon dynamics in this complex and intricate agroforestry system. With the use of destructive sampling and the conventional method of laboratory procedures, the study was based on a realistic and exact estimation of carbon stocks, not slipping the limitations of the previous studies that were only built on allometric equations. It turns out that the developed allometric models for coconut and gliricidia have become useful non-destructive methods for estimating the carbon stocks in these two commodities.
These models make it possible for scientists and staff to detect carbon more accurately without the use of destructive sampling in agricultural forestry. However, the paper goes further and shows how coconut-gliricidia associative farming contributes to the solution of interrelated issues of climate change, food shortage, and sustainable livelihoods at local and global levels. Through the method of carbon dioxide sequestration, improvement in soil capacity, and economic benefits, the systems take a complete system and can achieve the United Nations Sustainable Development Goals (SDGs), with particular attention to those related to life on land, responsible consumption and production, and climate action. This study serves as one of the main contributions in the research area of agroforestry and climate change mitigation in Sri Lanka. The findings provide an informed base to design sustainable and climate-resilient agricultural strategies. To enhance the broader applicability of these equations, future research should collect data across multiple agroecological zones, incorporate climate variables, and develop correction factors for different temperature and rainfall conditions.
DECLARATIONS
Acknowledgments
The authors would like to express sincere appreciation to the dedicated team at the Agronomy Division of the Coconut Research Institute of Sri Lanka for their invaluable assistance.
Authors’ contributions
Conceptualization: Nuwarapaksha, T. D.; Dilhan, R. M. C. P.; Udumann, S. S.; Atapattu, A. J.
Methodology: Nuwarapaksha, T. D.; Atapattu, A. J.
Validation: Atapattu, A. J.; Ranasinghe, C. S.; Egodawatta, W. C. P.
Writing-original draft preparation: Nuwarapaksha, T. D.; Dissanayaka, N. S.; Dilhan, R. M. C. P.
Writing-review and editing: Atapattu, A. J.; Nuwarapaksha, T. D.; Ranasinghe, C. S.; Egodawatta, W. C. P.
Supervision, project administration: Atapattu, A. J.
Visualization: Dissanayaka, N. S.; Udumann, S. S.
All authors have read and agreed to the published version of the manuscript.
Availability of data and materials
The data that support the findings of this study are available from the corresponding author upon reasonable request.
Financial support and sponsorship
None.
Conflicts of interest
All authors declared that there are no conflicts of interest.
Ethical approval and consent to participate
Not applicable.
Consent for publication
Not applicable.
Copyright
© The Author(s) 2025.
REFERENCES
1. Malhi, Y.; Franklin, J.; Seddon, N.; et al. Climate change and ecosystems: threats, opportunities and solutions. Philos. Trans. R. Soc. Lond. B. Biol. Sci. 2020, 375, 20190104.
2. Singh, S.; Kiran, B. R.; Mohan, S. V. Carbon farming: a circular framework to augment CO2 sinks and to combat climate change. Environ. Sci. Adv. 2024, 3, 522-42.
3. Kaur, R.; Kaur, N.; Kumar, S.; Dass, A.; Singh, T. Carbon capture and sequestration for sustainable land use - A review. Ind. J. Agri. Sci. 2023, 93, 124838.
4. Samaraweera, W. G. R. L.; Dharmadasa, R. A. P. I. S.; Kumara, P. H. T.; Bandara, A. S. G. S. Evidence of climate change impacts in Sri Lanka - a review of literature. Sri. Lanka. J. Econ. Res. 2024, 11, 69-94.
5. Ranasinghe, H. Carbon net-zero by 2050: benefits, challenges and way forward. J. Trop. For. Sci. 2022, 12.
6. Atapattu, A. J.; Ranasinghe, C. S.; Nuwarapaksha, T. D.; Udumann, S. S.; Dissanayaka, N. S. Sustainable agriculture and sustainable development goals (SDGs). In: Garwi, J.; Masengu, R.; Chiwaridzo, O. T.; editors. Emerging technologies and marketing strategies for sustainable agriculture; 2024. pp. 1-27.
7. Atapattu, A. A. A. J.; Pushpakumara, D. K. N. G.; Rupasinghe, W. M. D.; Senarathne, S. H. S.; Raveendra, S. A. S. T. Potential of Gliricidia sepium as a fuelwood species for sustainable energy generation in Sri Lanka. Agric. Res. J. 2017, 54, 34-9.
8. Dissanayaka, D. M. N. S.; Dissanayake, D. K. R. P. L.; Udumann, S. S.; Nuwarapaksha, T. D.; Atapattu, A. J. Agroforestry - a key tool in the climate-smart agriculture context: a review on coconut cultivation in Sri Lanka. Front. Agron. 2023, 5, 1162750.
9. Nuwarapaksha, T. D.; Udumann, S. S.; Dissanayaka, D.; Dissanayake, D.; Atapattu, A. J. Coconut based multiple cropping systems: an analytical review in Sri Lankan coconut cultivations. Circ. Agric. Syst. 2022, 2, 1-7.
10. Atapattu, A. J. Gliricidia as a beneficial crop in resource-limiting agroforestry systems in Sri Lanka. Ind. J. Agrofor. 2023, 25, 12-8. Available from: https://epubs.icar.org.in/index.php/IJA/article/view/129957 [Last accessed on 18 Jan 2025]
11. Dissanayaka, N. S.; Udumann, S. S.; Nuwarapaksha, T. D.; Atapattu, A. J. Agroforestry: an avenue for resilient and productive farming through integrated crops and livestock production; 2023, pp. 115-36.
12. Nuwarapaksha, T. D.; Udumann, S. S.; Dissanayaka, N. S.; Atapattu, A. J. Coconut-based livestock farming: a sustainable approach to enhancing food security in Sri Lanka; 2023, pp. 197-213.
13. Atapattu, A. J.; Udumann, S. S. Leveraging agroforestry principles for nature-based climate-smart solutions for coconut cultivation. In: Leal Filho, W.; Nagy, G. J.; Ayal, D. Y.; editors. Handbook of nature-based solutions to mitigation and adaptation to climate change. Cham: Springer International Publishing; 2023. pp. 1-28.
14. Subramanian, P.; Gupta, A.; Gopal, M.; et al. Coconut (Cocos nucifera L.). In: Thomas GV, Krishnakumar V, editors. Soil health management for plantation crops. Singapore: Springer Nature; 2024. pp. 37-109.
15. Ali, S.; Khan, S. M.; Abdullah, A.; Rana, M.; Ahmad, Z. Chapter 20 - Dryland agroforestry: mitigating role in reducing air pollution and climate change impacts. In: Agroforestry for carbon and ecosystem management. Elsevier; 2024. pp. 271-82.
16. Issa, S.; Dahy, B.; Ksiksi, T.; Saleous, N. A review of terrestrial carbon assessment methods using geo-spatial technologies with emphasis on arid lands. Remote. Sens. 2020, 12, 2008.
17. Sebrala, H.; Abich, A.; Negash, M.; Asrat, Z.; Lojka, B. Tree allometric equations for estimating biomass and volume of Ethiopian forests and establishing a database: review. Trees. Forests. People. 2022, 9, 100314.
18. Mahmood, H.; Siddique, M. R. H.; Islam, S. M. Z.; et al. Applicability of semi-destructive method to derive allometric model for estimating aboveground biomass and carbon stock in the Hill zone of Bangladesh. J. For. Res. 2020, 31, 1235-45.
19. Sun, W.; Liu, X. Review on carbon storage estimation of forest ecosystem and applications in China. For. Ecosyst. 2020, 7, 4.
20. Le Roux, X.; Lacointe, A.; Escobar-Gutiérrez, A.; Le Dizès, S. Carbon-based models of individual tree growth: a critical appraisal. Ann. For. Sci. 2001, 58, 469-506.
21. Raveendra, S. A. S. T.; Atapattu, A. A. A. J.; Senarathne, S. H. S.; Ranasinghe, C. S.; Weerasinghe, K. W. L. K. Evaluation of the carbon sequestration potential of intercropping systems under coconut in Sri Lanka. Int. J. Geol. Earth. Environ. Sci. 2017, 7, 1-7. Available from: https://www.cibtech.org/J-GEOLOGY-EARTH-ENVIRONMENT/PUBLICATIONS/2017/VOL_7_NO_1/01-JGEE-001-ATAPATTU-EVALUATION.pdf [Last accessed on 18 Jan 2025]
22. Raveendra, S. A. S. T.; Nissanka, S. P.; Somasundaram, D.; Atapattu, A. J.; Mensah, S. Coconut-Gliricidia mixed cropping systems improve soil nutrients in dry and wet regions of Sri Lanka. Agroforest. Syst. 2021, 95, 307-19.
23. Nuwarapaksha, T. D.; Udumann, S. S.; Atapattu, A. J. Fostering food and nutritional security through agroforestry practices. In: Raj, A.; Jhariya, M. K.; Banerjee, A.; Jha, R. K.; Singh, K. P.; editors. Agroforestry. Wiley; 2024. pp. 285-318.
24. Agro-ecological regions of Sri Lanka; 2003. Available from: https://doa.gov.lk/nrmc-downloads/ [Last accessed on 22 Jan 2025].
25. Panabokke, C. R. Soils and agro-ecological environments of Sri Lanka; 1996. pp. 133-4. Available from: https://www.amazon.com/Soils-Agro-Ecological-Environments-Sri-Lanka/dp/9555900051 [Last accessed on 18 Jan 2025].
26. Senarathne, S. H. S.; Udumann, S. S. Evaluation of coconut based anacardium occidentale agroforestry system to improve the soil properties of coconut growing lands in wet, Intermediate and Dry Zone of Sri Lanka. Coconut. Res. Dev. J. 2020, 35, 1-10.
27. Krishnakumar, V.; Thampan, P. K.; Nair, M. A. The coconut palm (Cocos nucifera L.) - research and development perspectives. Singapore: Springer; 2018. Available from: https://link.springer.com/book/10.1007/978-981-13-2754-4 [Last accessed on 18 Jan 2025].
28. George, M. L. C.; Bourdeix, R.; Konan, J. L. Regeneration guidelines: coconut. In: Crop-specific regeneration guidelines. Available from: https://www.researchgate.net/publication/292966940_Regeneration_guidelines_coconut [Last accessed on 18 Jan 2025].
29. Ranasinghe, C. S. Report of the plant physiology division. In: Jayasekara C, editor, Annual report of the coconut research institute. Bandirippuwa Estate, Lunuwila: Coconut Research Institute; 2008, pp. 198-207.
30. Friend, D.; Corley, R. H. V. Measuring coconut palm dry matter production. Exp. Agric. 1994, 30, 223-35.
31. Matthews, G. The carbon content of trees. Forestry Commission; 1993. Available from: https://books.google.com/books/about/The_Carbon_Content_of_Trees.html?id=2KUsAQAAMAAJ [Last accessed on 18 Jan 2025].
32. Navarro, M. N. V.; Jourdan, C.; Sileye, T.; et al. Fruit development, not GPP, drives seasonal variation in NPP in a tropical palm plantation. Tree. Physiol. 2008, 28, 1661-74.
33. Coconut Research Institute of Sri Lanka. Report of the agronomy division. In: annual report of the coconut research institute Bandirippuwa Estate, Lunuwila, Sri Lanka; 2022, pp. 386-8. Available from: https://cri.gov.lk/en/ar-publications/ [Last accessed on 22 Jan 2025].
34. Menon, K. P. V.; Pandalai, K. M. The coconut palm. A monograph. Ernakulam, India. 1958. Available from: https://catalogue.nla.gov.au/catalog/1014119 [Last accessed on 18 Jan 2025].
35. Niral, V.; Jerard, B. A. Botany, origin and genetic resources of coconut. In: Krishnakumar, V.; Thampan, P.; Nair, M.; editors, The Coconut Palm (Cocos nucifera L.) - Research and development perspectives. Singapore: Springer; 2018, pp. 57-111.
36. Chan, E.; Elevitch, C. R. Cocos nucifera (coconut). Species profiles for Pacific Island agroforestry; 2006. Available from: https://raskisimani.com/wp-content/uploads/2013/01/cocos-nucifera-coconut.pdf [Last accessed on 18 Jan 2025].
37. Walkley, A.; Black, I. A. An examination of the Degtjareff method for determining soil organic matter, and a proposed modification of the chromic acid titration method. Soil. Sci. 1934, 37, 29-38.
38. De Silva MAT, Abeyawardena V. Leaf growth in relation to age and the bearing status of coconut palms; 1970, pp. 59-62. Available from: https://dl.nsf.gov.lk/items/8bc0421e-8898-4bce-a61e-5d8cd6198dca [Last accessed on 18 Jan 2025].
39. Ella, A.; Blair, G. J.; Stür, W. W. Effect of age of forage tree legumes at the first cutting on subsequent production. Australia: Tropical Grasslands; 1991, pp. 275-80. Available from: https://fao-agris-review-search-zwcsjik2pa-uc.a.run.app/search/en/providers/122478/records/6477598c5eb437ddff764e95 [Last accessed on 18 Jan 2025]
40. Mulyana, B.; Soeprijadi, D.; Purwanto, R. H. Development of bioenergy plantation in Indonesia: yield regulation and above-ground carbon storage in Gliricidia (Gliricidia sepium) plantation. E3S. Web. Conf. 2020, 202, 08009.
41. Desta, G.; Kassawmar, T.; Tadesse, M.; Zeleke, G. Extent and distribution of surface soil acidity in the rainfed areas of Ethiopia. Land. Degrad. Dev. 2021, 32, 5348-59.
42. Gasim, M. B.; Khalid, N. A.; Muhamad, H. The influence of tidal activities on water quality of Paka River Terengganu, Malaysia. Malays. J. Anal. Sci. 2015, 19, 979-90. Available from: http://www.ukm.my/mjas/v19_n5/pdf/MuhammadBarzani_19_5_9.pdf [Last accessed on 18 Jan 2025]
43. Maina, M.; Maidugu, D. W.; Adamu, A.; Balami, S. D. Compaction as affected by bulk density and dry density of a soil. IOSR. J. Appl. Phys. 2020, 12, 6-13. Available from: https://www.iosrjournals.org/iosr-jap/papers/Vol12-issue4/Series-2/B1204020613.pdf [Last accessed on 18 Jan 2025]
44. Bockheim, J. G.; Hartemink, A. E.; Huang, J. Distribution and properties of sandy soils in the conterminous USA - A conceptual thickness model, and taxonomic analysis. CATENA. 2020, 195, 104746.
45. Saha, S. K.; Ramachandran, N. P. K.; Nair, V. D.; Mohan Kumar, B. Carbon storage in relation to soil size-fractions under tropical tree-based land-use systems. Plant. Soil. 2010, 328, 433-46.
46. Ranasinghe, C. S.; Thimothias, K. S. H. Estimation of carbon sequestration potential in coconut plantations under different agro-ecological regions and land suitability classes. J. Natl. Sci. Found. Sri. Lanka. 2012, 40, 77-93.
47. Doetterl, S.; Berhe, A. A.; Nadeu, E.; Wang, Z.; Sommer, M.; Fiener, P. Erosion, deposition and soil carbon: a review of process-level controls, experimental tools and models to address C cycling in dynamic landscapes. Earth. Sci. Rev. 2016, 154, 102-22.
48. Singh, M.; Sarkar, B.; Biswas, B.; Bolan, N. S.; Churchman, G. J. Relationship between soil clay mineralogy and carbon protection capacity as influenced by temperature and moisture. Soil. Biol. Biochem. 2017, 109, 95-106.
49. Rathnayake, H.; Mizunoya, T. A study on GHG emission assessment in agricultural areas in Sri Lanka: the case of Mahaweli H agricultural region. Environ. Sci. Pollut. Res. Int. 2023, 30, 88180-96.
50. Ozili, P. K. The acceptable R-square in empirical modelling for social science research. In: Saliya, C. A.; editor. Social Research Methodology and Publishing Results; 2023. pp. 134-43.
Cite This Article
How to Cite
Download Citation
Export Citation File:
Type of Import
Tips on Downloading Citation
Citation Manager File Format
Type of Import
Direct Import: When the Direct Import option is selected (the default state), a dialogue box will give you the option to Save or Open the downloaded citation data. Choosing Open will either launch your citation manager or give you a choice of applications with which to use the metadata. The Save option saves the file locally for later use.
Indirect Import: When the Indirect Import option is selected, the metadata is displayed and may be copied and pasted as needed.
About This Article
Copyright
Data & Comments
Data
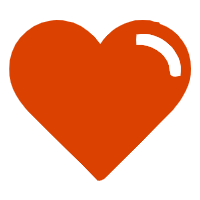
Comments
Comments must be written in English. Spam, offensive content, impersonation, and private information will not be permitted. If any comment is reported and identified as inappropriate content by OAE staff, the comment will be removed without notice. If you have any queries or need any help, please contact us at [email protected].