The cognitive functions of the cerebellum and its role in neurodegenerative diseases
Abstract
The cerebellum has long been associated with motor control. However, its role in cognitive functions has attracted increasing attention recently. The uniformity of cerebellar internal structure seems at odds with its involvement in such diverse cognitive functions. Nonetheless, in cerebellar diseases such as ataxia, there is a comorbidity of motor and cognitive impairments, raising essential questions about how and to what extent the cerebellum participates in cognitive functions. This review begins by tracing the historical development of cerebellar research, suggesting that the diverse connections between the cerebellum and cerebral cortex, basal ganglia, and other subcortical nuclei form the basis for the cerebellum’s role in regulating cognitive functions. We then delve into its involvement in language, reward-based learning, working memory, and spatial cognition. Additionally, we summarize the changes in the cerebellum observed in Alzheimer’s disease (AD), Parkinson’s disease (PD), and ataxias and their impact on cognitive functions. By discussing the role and mechanism of the cerebellum in cognition in physiology and pathology from the aspects of structure and function, we aim to shed light on promising new therapeutic targets related to the cerebellum for cognitive impairment.
Keywords
INTRODUCTION
The earliest study of the cerebellum was authored by Vincenzo Malacarne in 1776 when he was investigating the relationship between the size of the human cerebellum and intelligence, in which he described many structures of the cerebellum, such as vermis, tonsil, nodulus, and lingula[1]. Approximately half a century later, Jean Marie Pierre Flourens demonstrated through experiments on pigeons that damage to the cerebellum led to a decline in their ability to fly, and such decline was not due to a loss of muscle strength, but instead to a loss of the coordination of their voluntary wing movements[2]. Subsequently, a series of research studies conducted on cerebellum lesioned monkeys[3-5], along with clinical observations of patients with cerebellar injuries[6-11], reported that cerebellum syndrome was characterized by ataxic motor symptoms (e.g., incoordination of balance, gait, extremity, and eye movements) and vestibulo-cerebellar syndrome (e.g., imbalance, nystagmus, and vertigo). On the other hand, the cognitive and psychiatric symptoms resulting from the cerebellar lesions tend to be subtle, diverse, and less immediately apparent compared to overt motor deficits. This is probably why the cerebellum has been regarded as a center devoted solely to motor control (including vestibular and oculomotor) for nearly 200 years, overlooking its role in cognitive functions since then. It was not until the late 1980s and early 1990s that the cognitive functions of the cerebellum began to be explored, initiated by Schmahmann’s groundbreaking series of studies on patients with cerebellar lesions[12]. They conducted a clinical study of 20 patients with diseases confined to the cerebellum and found that those with lesions in the posterior lobe and vermis of the cerebellum exhibited the most significant clinical behavioral changes. These changes were characterized by impairments in executive functions, difficulties with spatial cognition, personality changes, and language deficits[13]. This newly defined clinical entity was termed the “cerebellar cognitive affective syndrome (CCAS)”, now also known as Schmahmann syndrome. Furthermore, with the establishment and development of theoretical models such as the universal cerebellar transform (UCT)[12,14-16], which proposes that the cerebellum performs a consistent type of information processing across its motor and non-motor functional domains, alongside additional anatomical evidence[17-20], attention has gradually shifted toward the cerebellum’s non-motor functions and the concept boundary of cerebellum syndrome has also been expanded. It is now well-known that CCAS has become the third cornerstone of clinical ataxiology[21], alongside the cerebellar motor syndrome and the vestibulo-cerebellar syndrome, caused by lesions in the cerebellar motor and vestibular regions, respectively.
In recent years, the role of the cerebellum in cognition has received increasing attention, leading to more in-depth research. Functional magnetic resonance imaging (fMRI) studies conducted on healthy humans have demonstrated that the cerebellum is involved in higher-order cognitive functions, including language function, reward and learning, working memory, and spatial cognition[22,23]. Additionally, as the role of cerebellum in neurodegenerative diseases such as Alzheimer’s disease (AD) and Parkinson’s disease (PD) gains increasing attention[24-26], along with the rising number of cognitive impairments observed in these conditions[27-29], there is also a growing focus on cerebellum’s contribution to the cognitive deficits in these diseases.
This review outlines the current research on the cerebellum’s involvement in cognition. We summarize evidence of the anatomical and neural circuits involved in cerebellar-related cognitive functions, the role of the cerebellum in various cognitive functions, and the cognitive impairments resulting from cerebellar lesions in the context of neurodegenerative diseases such as AD, PD, and ataxias, to help readers further understand the connection between the cerebellum and related cognitive functions, inspiring deeper exploration into the role of the cerebellum in cognition. Additionally, we include the changes in the cerebellum in relevant neurodegenerative diseases and their impact on cognitive impairments within these diseases, thereby implying that the cerebellum may be a potential therapeutic target for these conditions.
ANATOMICAL AND NEURAL CIRCUIT SUBSTRATES
Unlike the cerebral cortex, the neural circuits within the cerebellum exhibit a high degree of uniformity. Ramón y Cajal (1909) was the first to give a detailed description of the three-layer structure of the cerebellar cortex, identifying molecular layer, Purkinje cell layer, and granular cell layer. Climbing fibers transmit information from the olivary complex, while mossy fibers originate from various afferent sources. Ultimately, these inputs converge onto the Purkinje cells, which then relay the signals to the three deep cerebellar nuclei, the fastigial, interposed, and dentate nucleus, constituting the primary output of the cerebellum. Such a kind of modular circuity, including some inhibitory interneurons, remains largely fixed within the anatomical structure of the cerebellum[30]. Unipolar brush cells, the only excitatory interneurons in the cerebellum, may introduce some heterogeneity into internal neural circuity, but they are found only in the vestibulocerebellum[31].
From the perspective of its functions, the cerebellum can be broadly divided into two subregions: motor cerebellum and cognitive cerebellum. The motor cerebellum is primarily located in the anterior part of the cerebellum, including lobules V, VI, and VIII, while the cognitive cerebellum is situated in the posterior part, including crus I and II [Figure 1A]. Patients with cerebellar damage confined to the posterior part exhibited primarily cognitive dysfunctions, e.g., acute psychomotor retardation, infrequent speech, and mild cognitive decline, while their motor functions were largely unaffected[32,33]. In fMRI studies on healthy subjects, the activation patterns of the cerebellum were consistent with its anterior-posterior functional organization; during movement, there was significant activation in the anterior lobe and lobule VIII of the cerebellum, whereas cognitive tasks primarily activated the posterior and lateral regions of the cerebellum[34,35]. These anatomical locations correspond with the functional findings observed in patients with cerebellar lesions.
Figure 1. The anatomical and neural circuit basis of cerebellar involvement in cognitive functions. (A) An illustration of an unfolded cerebellum showing its ten lobules, depicting the topological structures of the motor, cognitive, and vestibular cerebellum. Notably, the anterior part of lobule VI is associated with the motor cerebellum, while the posterior part is linked to the cognitive cerebellum; (B) The connections related to cognition between the cerebellum and the cerebral cortex, basal ganglia, and other cognition-related subcortical brain regions.
The functional differences in distinct cerebellar regions are closely related to their connections with external brain regions, such as the cerebral cortex, basal ganglia, and other subcortical regions[18,36]. In contrast to the repeated canonical circuit architecture within the cerebellum, the afferent and efferent connections of the cerebellum exhibit significant heterogeneity and are characterized by high functional specificity.
Connections between cerebellum and cerebral cortex
The cortico-ponto-cerebellar and the cerebello-thalamo-cortical circuitry are the two main pathways connecting the cerebellum with the cerebral cortex [Figure 1B]. Feedforward information is transmitted from the cerebral cortex to the cerebellum through the former circuit, and the cerebellum then processes this information and provides feedback to the cerebral cortex. Using trans-synaptic tracing techniques, projections from the arm region of the primary motor cortex (M1) to lobules IV-VI of the cerebellar cortex have been identified, as well as projections from area 46 of the dorsolateral prefrontal cortex (PFC) to crus II and lobule X, indicating feedforward projections[37]. Furthermore, within the cerebellar nuclei, the dorsal part of the dentate nucleus and interposed nucleus project to M1, while the ventral part of the dentate nucleus projects to area 46[19,38], indicating feedback projections.
The conventional view suggests that the cerebellum connects with the motor cortex through the cortico-ponto-cerebellar and cerebello-thalamo-cortical pathways, participating in sensorimotor coordination. However, these parallel sub-circuits are widely present in the cerebrum and cerebellum and precisely connect different areas of the cerebral cortex with corresponding regions of the cerebellum, serving essential functions in various tasks. Anatomical studies have demonstrated that the cognitive-related cortical areas, such as the PFC, can also project to the cerebellum through cerebello-thalamo-cortical pathways, while the cerebellum reciprocally projects back to these brain regions via cortico-ponto-cerebellar pathways as well[39,40]. These connections have also been confirmed by functional connectivity observed in fMRI studies[41,42], suggesting that the cerebellum is involved in cognitive functions through its interactions with the cerebral cortex.
Connections between cerebellum and basal ganglia
The cerebellum and basal ganglia are widely regarded as two major subcortical motor structures. These two brain regions are extensively connected to the cerebral cortex through separate circuits[43]. It had been believed that the cerebellum and the basal ganglia were anatomically distinct subcortical systems that performed unique functional operations, and connections between these two regions occurred mainly at the level of the cerebral cortex[44,45]. However, anatomical connections between the cerebellum and basal ganglia have been explored and recognized during the past decade, with their functions extending beyond motor control[46-49] [Figure 1B].
In 2010, a study using retrograde transneuronal tracing techniques in Cebus monkeys revealed that the subthalamic nucleus sent a substantial disynaptic projection to the cerebellar cortex[46]. Two recent studies have confirmed that the subthalamic nucleus sends an afferent pathway that connects with the ipsilateral cerebellar cortex via the relay of pontine nuclei[47,48]. In addition, it has been reported that the efferent projection of the dentate nucleus passes through the thalamus and projects to the striatum and the external segment of the globus pallidus[47,48]. Furthermore, there is evidence of a direct circuit linking the dentate nucleus to the internal globus pallidus and substantia nigra based on a constrained spherical deconvolution tractography study carried out on humans[49]. Previous research has demonstrated a concurrent activation of the cerebellum and basal ganglia during reward-related learning[50,51], suggesting that these pathways may be involved in the cognitive processes underlying reward and learning. Moreover, Silveri noted that the circuit connections between the cerebellum and basal ganglia played a crucial role in language production[52], underscoring the diverse functions of cerebellar-basal ganglia pathways in various cognitive processes.
Connections between the cerebellum and other subcortical regions
Carta et al. reported in mice that the cerebellar nuclei directly project to the ventral tegmental area (VTA), a brain region crucial for processing and encoding reward[53]. It has been proved that the projection from the cerebellum to VTA is necessary for social preference in mice, and the cerebellar inputs to VTA inputs are rewarding[53]. Furthermore, optogenetic activation of this projection enhances social behaviors[53]. These findings indicate cerebellar inputs to VTA modulate the reward pathway and play a prominent role in social behavior [Figure 1B].
The hippocampus is a critical brain region for spatial memory and navigation, and it also has circuit connections with the cerebellum, involved in spatial cognition. Using retrograde transneuronal tracing techniques in mice, it has been discovered that the cerebellum is connected to the dorsal hippocampus via intermediary neurons[54]. A constrained spherical deconvolution analysis of humans also revealed the connection between the cerebellum and hippocampus[55], consistent with the findings observed in mice. A resting-state fMRI study in the human brain also indicated that abnormal changes in functional connectivity between the cerebellum and the left hippocampus, as well as between the cerebellum and the right cingulate gyrus, contribute to a range of cognitive impairments resulting from cerebellar infarction[56]. These anatomical and functional findings suggest that the connections between the cerebellum and the hippocampus play a significant role in cognition [Figure 1B].
Hypothalamus, an integral component in the limbic system, plays a pivotal role in stress responses and emotional regulation, primarily through pathways such as the hypothalamic-pituitary-adrenal (HPA) axis[57]. Studies have revealed intricate connections between the hypothalamus and the cerebellum. Our previous studies have suggested that the hypothalamus can modulate cerebellar neuronal activity through histamine and orexin, thereby influencing cerebellar motor control and participating in somatic and non-somatic integration[58-62]. Such histaminergic and orexinergic inputs are likely to be involved in not only basic somatic motor control but also higher cognitive and emotional functions[63,64] [Figure 1B].
The amygdala is a critical center of the limbic system, responsible for emotional processing and memory. Using fluorescence micro-optical sectioning tomography (fMOST) technology, we recently revealed a direct projection from the cerebellar dentate nucleus to the amygdala[62,65]. Clinical evidence shows that the severity of anxiety symptoms in patients with PD is inversely correlated with the activity of this circuit[62]. Animal studies indicate that this circuit can be activated by exercise and plays a crucial role in alleviating anxiety[62]. Notably, the amygdala has been recognized for its significant role in cognition, especially social cognition[66]. Whether the cerebello-amygdalar circuit contributes to cognitive functions still needs further investigation [Figure 1B].
ROLE OF CEREBELLUM IN COGNITION
The extensive connections between the cerebellum and cognition-related brain structures provide the anatomic basis for the involvement of the cerebellum in cognitive functions, including language function, reward and learning, working memory, and spatial cognition.
Cerebellum in language function
Complex language, a higher cognitive function unique to humans, involves the coordinated processing of multiple cortical and subcortical brain regions. Traditionally, it has been believed that language is primarily associated with Broca’s area in the frontal cortex and Wernicke’s area in the superior temporal cortex[67]. However, the role of the cerebellum in language processing is receiving increasing attention. As early as 1988, by using positron emission tomography (PET), a study measured regional changes in average blood flow during the processing of single auditory or visual words, and the cerebellum was found to be significantly activated in verb-for-noun generation paradigms[68]. Two fMRI studies in 2014 and 2017 are consistent with the findings. Increased activation in the right cerebellar lobule VII was observed during sentence completion tasks, where participants predicted the most suitable word to complete a sentence based on its context[69,70]. A recent study in 2022 demonstrated that the connections between cerebellar lobule VI and the dorsal anterior cingulate cortex/pre-supplementary motor cortex might hold a key position in the language control network[71] [Table 1].
The circuit mechanism studies of the cerebellum in cognition
Authors | Year | Circuits | Methods | Subjects | Functions |
Yuan et al.[71] | 2013 | Connections between cerebellar lobule VI and dorsal anterior cingulate cortex/pre-supplementary motor cortex | fMRI | Human | Language control |
Larry et al.[72] | 2024 | Caudate-SNpr-cerebellar vermis (Purkinje cell) pathway | In vivo electrophysiology | Macaca Fascicularis monkeys | Reward expectation and movement |
Carruzzo et al.[73] | 2023 | Connections between the cerebellum and ventral striatum | fMRI | Human | Reward anticipation |
Carta et al.[53] | 2023 | Connections between cerebellar nuclei (glutamatergic neurons) and VTA | Anterograde tracing and optogenetic manipulation | Mice | Reward in social behavior |
Li et al.[74] | 2022 | Connections between lobule VI and right cingulate cortex/bilateral superior frontal gyrus | fMRI | Human | Verbal working memory |
Bezdicek et al.[75] | 2021 | Connections between cerebellar lobule I-V and DLPFC | fMRI | PD patients | Working memory |
Sako et al.[76] | 2021 | Connections between cerebellar lobule VII and visuospatial-executive-domain-related/attention-domain-related networks | fMRI | PD patients | Visuospatial execution and attention |
Moreover, cerebellar lesions can adversely affect a patient’s language abilities. A study on a patient with neurogenic stuttering caused by brain infarction showed that preexisting thalamic hemorrhage or frontal lobe infarction did not lead to stuttering, while significant stuttering occurred after a cerebellar infarction[77]. A more in-depth study indicated that patients with cerebellar damage exhibited more metalinguistic deficits, while their grammatical and semantic abilities were relatively preserved[78]. This pattern of language impairment supports the dysmetria of thought theory, which posits that cerebellar cognitive deficits follow a logic like that of motor deficits: cerebellar damage disrupts the regulation of movement without impairing its generation (leading to dysmetria but not weakness), and similarly, it disrupts the regulation of language without impairing its generation (leading to metalinguistic deficits but not aphasia).
Another interesting phenomenon is the lateralization of cerebellar language functions, with a notable right-sided dominance. This may be due to the language centers in the left cerebral cortex, as the cerebellum is connected to the contralateral cerebral cortex[22,23]. Using anodal transcranial direct-current stimulation (tDCS) to modulate the right cerebellar crus I and II can alter the cerebellar signaling during predictive language processing and enhance functional connectivity within the reading/language network[70].
Cerebellum in reward and learning
The role of the cerebellum in reward and learning also attracts increasing attention. Using two-photon calcium imaging in behaving mice, a recent study showed that some cerebellar granule cells responded preferentially to reward or reward omission, whereas others selectively encoded reward anticipation[79]. Additionally, climbing fibers can not only directly respond to rewards[80-82] but also encode the magnitude of expected rewards[83,84]. This aligns with observations of cerebellar macro-activity. A meta-analysis of human brain fMRI studies demonstrated that reward anticipation was associated with regional activity in the bilateral anterior lobe, bilateral lobule VI, left Crus I, and the posterior vermis, while reward outcome was related to regional activity in the declive and left lobule VI[85]. These findings demonstrate the distinct involvement of the cerebellum in reward anticipation and outcome processing.
An experiment comparing patients with cerebellar ataxia to healthy controls showed that individuals with cerebellar ataxia had severely impaired reward learning from trial-and-error feedback but retained the ability to predict rewards based on contextual memory[86]. Their findings suggest that the cerebellum may play a special and necessary role in incremental learning based on reinforcement-reward associations. Additionally, this work proposes that the cerebellum collaborates with the basal ganglia to support reinforcement learning from rewards, extending beyond motor learning. A study on monkey’s eye movements indicates that the cerebellum inputs reward expectation and movement signals into the basal ganglia, which processes this information and outputs information with a higher signal-to-noise ratio[72] [Table 1]. Furthermore, fMRI functional connectivity analysis shows that connectivity between the ventral striatum and the cerebellum increases during reward anticipation[73] [Table 1].
The underlying basis of these phenomena may be the plasticity changes within the cerebellum itself and its connections with other brain regions, which may facilitate learning and memory. Several studies have demonstrated that the intrinsic plasticity of Purkinje cells within the cerebellum is vital for the consolidation of motor memory, and memory consolidation deficits ensue when the intrinsic plasticity is impaired[87,88]. Additionally, a single-cell RNA sequencing study revealed that Purkinje cells might be classified into two primary subgroups: Plcb4+ and Aldoc+, the former of which exhibited significant plasticity and played a crucial role in associative learning[89]. A fMRI study comparing young and older subjects also showed that associative learning altered the intrinsic functional connectivity strength within several cerebellar networks, e.g., frontal-cerebellar, temporal-cerebellar, and cerebello-cerebellar in younger subjects, with significantly lesser effects in older individuals. It suggests that the neural plasticity within these cerebellar networks may play a crucial role in associative learning[90].
The VTA and substantia nigra are two primary sources of dopamine, a neurotransmitter closely associated with reward learning, and are widely recognized for their roles in encoding and predicting rewards[91,92]. Recent anatomical studies have shown that the cerebellum sends extensive projections to both the VTA and substantia nigra[49,53] [Table 1]. A review focused on these cerebellar projections suggested that cerebellar connections to the VTA may influence reward-based learning, while the projections to the substantia nigra pars compacta (SNc) may contribute to motor vigor[93]. Additionally, it has been confirmed that the cerebellum receives dopaminergic innervation, with dopamine D1, D2, and D3 receptors detected within the cerebellum[94,95].
Cerebellum in working memory
Working memory, a cognitive system responsible for temporarily holding and manipulating information, relies heavily on the coordinated activity of several brain regions. The PFC, parietal lobe, and hippocampus are well-established contributors. With its extensive projections to these regions[48,93,94], the cerebellum is suggested to play a potential role in neural circuits related to working memory. A comprehensive study examining various pediatric cerebellar disorders - including tumors, cerebellar infarctions, congenital cerebellar malformations, and cerebellar abnormalities due to genetic or developmental causes - found that regardless of the etiology of cerebellar damage, patients consistently exhibited working memory impairments[96]. The detrimental impact of cerebellar damage on working memory persists into adulthood, maintaining the same impairments observed in earlier stages of life[97-99].
Recent studies combining working memory tasks and advanced technologies in healthy subjects have confirmed the cerebellum’s involvement in working memory. A structural MRI study demonstrated that larger volumes of cerebellar crus I and the entire cerebellum correlate with better performance on a 2-back visual working memory task[100]. A functional MRI study revealed that cerebellar lobule VIIb/VIIIa selectively responds to memorized stimuli, indicating its role in storing and retrieving visual information[101]. Resting-state functional connectivity analyses showed that the strength of cortico-cerebellar circuit connections is associated with performances in working memory tasks[74] [Table 1].
Studies manipulating the cerebellum through transcranial magnetic stimulation (TMS) have further elucidated its role in working memory. One study demonstrated that applying 5 and 20 Hz repetitive TMS to the cerebellar crus II region in healthy subjects enhanced prefrontal excitability and significantly improved brain network efficiency, resulting in superior performance on working memory tasks compared to a sham stimulation group[102]. However, another TMS experiment targeting the left superior and left inferior cerebellum demonstrated that stimulation of these regions led to decreased accuracy on visual working memory tasks. Although different TMS parameters affect the cerebellum’s influence on working memory tasks differently, these findings collectively highlight the critical role of the cerebellum in working memory processes[103].
Cerebellum in spatial cognition
Spatial cognition is an essential aspect of cognitive functions. Many studies have reported decreased spatial cognition in patients with bilateral cerebellar damage. These patients exhibit visuospatial organization disorders, have difficulties in planning daily activities[104], and even show deficits in spatial procedural learning[105,106]. Additionally, spatial learning impairments have been observed in mice with cerebellar mutation or rats with hemicerebellectomy[107].
Direct damage to the cerebellar nuclei also has various effects on spatial cognition. For instance, bilateral electrical lesions of the dentate nucleus in rats led to impaired learning in the hidden platform task of the Morris water maze. However, these lesions did not affect long-term retention, the probe trial, or the visuomotor guidance necessary for navigating toward a visible goal[108,109]. This selective impairment indicates the specific role of the dentate nucleus in spatial localization. In contrast, another study applied muscimol to completely inactivate all deep cerebellar nuclei, resulting in memory loss during the water maze task. Interestingly, this effect was not observed when the dentate nucleus was selectively inactivated, indicating that the fastigial nucleus and interposed nuclei are also critical regions for spatial cognition and may play a vital role in spatial memory[110].
The above evidence provides fundamental support for the involvement of the cerebellum in spatial cognition. However, the specific role it plays, and the types of neural signals it receives, integrates, and transmits, remain unclear. In addition, although the neural circuit connections between the cerebellum and hippocampus, the central structure for spatial memory and navigation, have been dissected, the role of these connections in spatial cognition remains unclear and requires further investigation.
CEREBELLUM AND COGNITIVE IMPAIRMENTS IN DISEASES
In this section, we will focus on the pathological changes of cerebellum in AD, PD, and ataxias, and explore the relationship between these changes and the associated cognitive impairments. Moreover, the impact of neuromodulation targeting the cerebellum on treating cognitive impairments in these diseases will be discussed.
Cerebellum and cognitive impairments in AD
AD is the most prevalent neurodegenerative disease worldwide. In 2015, approximately 29.8 million people globally were affected by AD[111], and the number of AD patients is expected to exceed 100 million by 2050[112]. The primary symptom of AD is dementia, which is often characterized by cognitive impairments such as memory loss, decline in spatial navigation abilities, and deterioration of language skills. AD has traditionally been associated with neurodegeneration in various cortical and subcortical brain regions, such as the hippocampus. However, accumulating evidence suggests that the cerebellum also undergoes age-related degenerative changes. A stereological study demonstrated that with aging, the total cortical volume of the cerebellum decreases by 10.8% over the lifespan, while its total volume declines by 25.9%, with various subregions experiencing different degrees of loss[113]. Accumulating studies show that cerebellar degeneration is also involved in the progression of AD.
At the molecular level, the cerebellar nuclei in AD patients also exhibit characteristic symptoms of AD-related cellular atrophy, such as ectopic cell cycle events and increased DNA damage. However, these alterations are primarily observed in the late stages of AD[114]. A voxel-based morphometry study revealed localized atrophy in the bilateral crus I and crus II regions of the cerebellum in AD patients, with the cerebellar regions showing atrophy also displaying stronger intrinsic functional connectivity with severely atrophic regions of the cerebrum. This suggests that cerebellar-cerebral circuit dysfunction may play a role in the pathogenesis of AD. Additionally, another study utilizing fluorodeoxyglucose-positron emission tomography (FDG-PET) scanned 830 AD patients and observed that a significant portion exhibited crossed cerebellar diaschisis (CCD), characterized by markedly reduced metabolic activity in the left temporal and occipital regions, as well as in the right cerebellum[115]. This further supports the notion of circuit dysfunction between the cerebral cortex and the cerebellum in AD patients. Moreover, a recent review indicated that degeneration of the inferior olivary nucleus in AD led to reduced input to the cerebellum, impairing its neuromodulatory functions, which may contribute to spatial navigation issues observed in the early stages of dementia[116]. A study involving 27 patients with AD also demonstrated that repetitive TMS (rTMS) targeting the bilateral cerebellum crus II significantly improved multi-domain cognitive functions, including overall cognitive levels, episodic memory, executive function, verbal ability, and visuospatial function[117]. These findings underscore the involvement of the cerebellum in AD and its significant role in the related symptoms.
Interestingly, the cerebellum appears to be relatively spared in the early stages of AD, leading some to speculate that the cerebellum may also play a compensatory role in the early cognitive deficits associated with AD[118], which is a hypothesis that warrants further investigation.
Cerebellum and cognitive impairments in PD
PD is the second most prevalent neurodegenerative disease in the world, following AD. People often focus more on the motor symptoms of PD, such as tremors, rigidity, postural instability, and akinesia, which are typically associated with dopaminergic neuron degeneration in the basal ganglia[24,25,119]. However, increasing evidence suggests that the cerebellum plays an important role in the motor symptoms seen in PD. As research progresses, there is growing attention to the cognitive impairments in PD and their association with the cerebellum[25].
At the molecular level, PD patients’ cerebellum exhibits α-synuclein aggregation and Lewy body formation and deposition[120,121], which are key molecular markers of neuronal degeneration in PD. A study on emotional vocal encoding indicated that PD patients with deficits in recognizing emotional vocals showed more severe lesions in the right hemisphere of the cerebellum[122]. Functional MRI connectivity studies demonstrated that PD patients with mild cognitive impairment exhibited disrupted resting-state functional connectivity between the bilateral dorsolateral prefrontal cortex (DLPFC) and the cerebellum, compared to PD patients with normal cognition. Furthermore, the weaker the functional connectivity between the DLPFC and the cerebellum, the poorer the performance on various working memory tasks[75] [Table 1]. In addition, a brain network study in PD patients identified the cerebellar lobule VII as a crucial node in visuospatial-executive-domain-related and attention-domain-related networks [Table 1].
Studies mentioned above indicate changes in the connectivity between the cerebellum and other brain regions in cognitive impairments associated with PD, while the cerebellum itself shows abnormally increased activity in PD. A PET study revealed that hypermetabolism in the anterior lobe and the vermis of the cerebellum correlated with the severity of motor dysfunction, while increased metabolic activity in the right crus I and crus II correlated with cognitive dysfunction[123]. Moreover, a neuroimaging meta-analysis indicated that PD patients with social perception impairments exhibited increased activation in the posterior cerebellum[124]. This increased neural activity in the cerebellum may play a compensatory role.
Neuromodulation targeting the cerebellum can also improve cognitive impairment in PD [Table 2]. In an open study[143], thirty patients with PD were stimulated with 1 Hz rTMS every half year for 1.5 years. After that, the tDCS was added to the stimulation over both sides of the cerebellum for the next 2 years. It was revealed that rTMS and tDCS improved the executive function of patients over 65 years. Given that cerebellar neuromodulation can also improve motor symptoms in PD [Table 2], the cerebellum emerges as a highly promising therapeutic target for ameliorating PD symptoms as well.
Neuromodulation studies targeting the cerebellum in the last fifteen years
Authors | Year | Method | Target | Disease | Efficacy | Improvement of cognition |
Grimaldi et al.[125] | 2013 | tDCS | Cerebellum (anode) and contralateral supra-orbital area (cathode) | Ataxia | Decreasing the amplitudes of long-latency stretch reflexes | |
Grimaldi et al.[126] | 2014 | tDCS | Cerebellum and contralateral motor cortex (both anode) | Ataxia (SCA2) | Improving voluntary movements of the upper limbs | |
Benussi et al.[127] | 2015 | tDCS | Cerebellum (anode) and right deltoid (cathode) | Ataxia | Improving posture, gait, and limb coordination | |
Benussi et al.[128] | 2017 | tDCS | Cerebellum (anode) and right deltoid (cathode) | Ataxia | Improving all ataxic symptoms | |
Bodranghien et al.[129] | 2017 | tDCS | Right cerebellar hemisphere (anode) and left motor cortex (cathode) | Ataxia (ARCA3) | Improving postural tremor | |
Grecco et al.[130] | 2017 | tDCS | Cerebellum (anode) and central supraorbital region (cathode) | Ataxia caused by cerebral palsy | Improving balance combined with treadmill training | |
Benussi et al.[131] | 2018 | tDCS | Cerebellum (anode) and spine (cathode) | Ataxia | Improving all ataxic symptoms | |
Benussi et al.[132] | 2021 | tDCS | Cerebellum (anode) and spine (cathode) | Ataxia | Improving motor and cognitive symptoms | √ |
Naeije et al.[133] | 2023 | tDCS | Cerebellum (anode) and right deltoid (cathode) | Ataxia (Friedreich’s ataxia) | Reducing motor and cognitive symptoms | √ |
Farzan et al.[134] | 2013 | TMS (rTMS) | Cerebellum | Ataxia | Improving limb coordination, speech, and gait | |
Bonnì et al.[135] | 2014 | TMS (iTBS) | Lateral cerebellum | Ataxia caused by cerebellar stroke | Improving ataxic gait and posture symptoms | |
Kim et al.[136] | 2014 | TMS (rTMS) | Cerebellar hemisphere ipsilateral to the ataxic side | Ataxia caused by acute posterior circulation stroke | Improving walking and balance | |
Cury et al.[137] | 2015 | TMS (rTMS) | Healthy dentate nucleus | Ataxia caused by unilateral cerebellar infarction | Improving tremor and cerebellar ataxia | |
Dang et al.[138] | 2019 | TMS (rTMS) | Cerebellum | Ataxia (SCA6) | Improving motor and speech function | |
França et al.[139] | 2020 | TMS | Cerebellum contralateral to the most clinically affected side | Ataxia | Improving ataxic symptoms | |
Teixeira et al.[140] | 2015 | DBS | Healthy dentate nucleus | Ataxia caused by cerebellar stroke | Improving tremor and cerebellar ataxia | |
Miterko et al.[141] | 2021 | DBS | Bilateral interposed cerebellar nuclei | Ataxia in Car8wdl mice | Improving motor behaviors of Car8wdl mice | |
Ferrucci et al.[142] | 2016 | tDCS | Cerebellum and M1 (anode) and right deltoid (cathode) | PD | Improving levodopa-induced dyskinesias | |
Málly et al.[143] | 2018 | rTMS and tDCS | Hemispheres of the cerebellum (anode) and middle part of the frontal area (cathode) for tDCS, and DLPFC and brainstem for rTMS | PD | Slowing the progression of PD in an age-dependent way | √ |
Workman et al.[144] | 2020 | tDCS | Cerebellar hemisphere contralateral to the more PD-affected side(anode) and contralateral upper arm or cerebellar hemisphere ipsilateral to the more PD-affected side (cathode) | PD | Improving balance performance | |
Koch et al.[145] | 2009 | TMS (cTBS) | Lateral cerebellum | PD | Decreasing levodopa-induced dyskinesias | |
Minks et al.[146] | 2011 | TMS (rTMS) | Right lateral cerebellum | PD | Improving gross motor skills and worsening fine motor skills | |
Brusa et al.[147] | 2012 | TMS (cTBS) | Lateral cerebellum | PD | Reducing levodopa-induced dyskinesia | |
Bologna et al.[148] | 2015 | TMS (cTBS) | Ipsilateral cerebellar hemisphere | PD | No influence on the generation of resting tremor in PD | |
Di Biasio et al.[149] | 2015 | TMS (cTBS) | Cerebellar hemisphere ipsilateral to the more affected side | PD | Improving STDT exclusively when patients were OFF therapy | |
Lefaivre et al.[150] | 2016 | TMS (rTMS) | Cerebellar vermis or lateral cerebellum | PD | Improving resting tremor (lateral cerebellum was better) | |
Di Lorenzo et al.[151] | 2013 | TMS (cTBS) | Posterior and superior lobules of the lateral cerebellum | AD | Affecting SLAI | |
Yao et al.[117] | 2022 | TMS (rTMS) | Bilateral cerebellum crus II | AD | Improving multi-domain cognitive functions | √ |
Demirtas-Tatlidede ei al.[152] | 2010 | TMS (iTBS) | Cerebellar vermis | Schizophrenia | Improving negative symptoms, mood, and cognition | √ |
Minichino et al.[153] | 2015 | tDCS | PFC (anode) and cerebellum (cathode) | Bipolar disorder | Improving visuospatial memory, executive function, and motor coordination | √ |
Sebastian et al.[154] | 2016 | tDCS | Cerebellum (anode) and right deltoid (cathode) | Aphasia | Augment spelling therapy | √ |
Cerebellum and cognitive impairments in ataxias
As previously mentioned, the cerebellum is traditionally associated with motor control. Consequently, cerebellar lesions often lead to noticeable motor impairments, with ataxias being the most characteristic condition, marked primarily by uncoordinated voluntary movements. Interestingly, ataxias, including pure spinocerebellar ataxia (SCA) and Friedreich ataxia (FRDA), are comorbid with cognitive impairments, highlighting the comorbidity of motor and cognitive impairments in cerebellar disorders.
A clinical study involving 35 SCA type 2 (SCA2) patients noted that 71.4% had cognitive impairments, including deficits in visuomotor construction, focused attention, learning and memory, language and fluency, and executive function[155]. Another psychometric evaluation revealed that SCA2 patients had lower general intelligence, executive function, and short-term and long-term verbal and visuospatial memory compared to age- and gender-matched healthy controls[156]. A review of SCA type 3 (SCA3) highlighted cognitive dysfunction, with multiple studies showing impairments, particularly in executive function[157]. Additionally, Orsi et al. identified memory, language, visuospatial, attention, executive, and emotional impairments across all gene-defined SCA categories[158].
Furthermore, a meta-analysis focusing on FRDA showed that individuals with FRDA performed significantly worse on most tasks related to language, attention, executive function, memory, visuospatial function, emotional regulation, and social cognition than those without FRDA. The degree of change in these cognitive functions was also correlated with cerebellar-related structural parameters, further indicating the cerebellum’s role in the pathophysiology of cognitive impairment in FRDA[159]. Several reviews on cerebellar ataxias have pointed out that patients with cerebellar ataxias endure numerous cognitive impairments, emotional issues, and some neuropsychiatric symptoms[160-162].
Studies utilizing tDCS have demonstrated that neuromodulation of the cerebellum improves cognitive dysfunction associated with ataxias [Table 2], and after anodal cerebellar tDCS and cathodal spinal tDCS, patients with cerebellar ataxias showed significant improvements in motor scores, cognitive function, and quality of life[132]. Additionally, a week of anodal cerebellar transcranial direct current stimulation (ctDCS) treatment alleviated both motor and cognitive symptoms in FRDA patients[133]. Interestingly, numerous studies have shown that cerebellar-targeted neuromodulation improves motor symptoms in ataxias [Table 2]. This suggests that the cerebellum may serve as a common target for treating both motor and cognitive impairments, highlighting its significant therapeutic potential in ataxias.
In summary, AD and PD patients with cognitive impairments show neurodegeneration in the cerebellum, and cerebellar ataxias are often accompanied by cognitive deficits. Therefore, cerebellar neurodegeneration may be actively involved in the cognitive dysfunction of AD, PD, and ataxias. These findings indicate that neuromodulation targeting the cerebellum may be a potentially effective strategy for alleviating cognitive symptoms in these disorders.
CONCLUSION
The cerebellum has historically been recognized for its role in motor control and coordination, but it is now increasingly regarded as crucial for various cognitive functions. We elucidated the extensive neural connections between the cerebellum and external brain regions, including the cerebral cortex, basal ganglia, and other subcortical areas. Building on this anatomical and neural circuit foundation, we further demonstrated the crucial role of the cerebellum in various higher-order cognitive processes, such as language, reward-based learning, working memory, and spatial cognition. Finally, we explored the changes in the cerebellum in different diseases, including ataxia, PD, and AD, and their impact on cognitive functions, and proposed that the cerebellum could serve as a therapeutic target for treating cognitive impairments associated with these diseases.
However, many questions in this field remain unresolved. Although the establishment of the UCT theory provides a reasonable framework for understanding the role of the cerebellum in both motor and cognitive functions, the specific mechanisms of how the cerebellum’s homogenous internal structure processes and outputs signals from different brain regions remain unclear. For example, at the molecular level, besides classical glutamatergic and GABAergic signals, diverse neurotransmitters and neuromodulators are present in the cerebellum, including amines like dopamine[79], norepinephrine[163], and histamine[60], and neuropeptides[164] like oxytocin[165], CRF[166], and orexin[167]. Their roles in processing motor and cognitive functions are still largely unknown. At the cellular level, single-cell sequencing has revealed the high heterogeneity of neurons in the cerebellum[89], but the roles of different cell clusters in cerebellar motor and cognitive control remain to be elucidated. Furthermore, it is essential to investigate the nature of the signals transmitted from the cerebellum to various brain regions, such as the cortex, basal ganglia, and amygdala, and how the cerebellum dynamically interacts with other brain areas to collectively perform a range of higher cognitive functions. In addition, from the perspective of pathophysiology, significant cerebellar deficits often occur in the late stage of AD and PD, suggesting that cerebellar dysfunction may arise as a secondary effect of widespread brain degeneration and network disruptions. However, considering the reciprocal connections between the cerebellum and cerebral cortex/basal ganglia, whether the cerebellum-mediated cognitive control holds a position in the early stage of AD and PD remains to be determined.
Lastly, as shown in Table 2, neuromodulation targeting the cerebellum in ataxias and PD predominantly focuses on improving motor functions, while neuromodulation for AD mainly targets DLPFC[168-179]. However, in certain psychiatric disorders, such as schizophrenia[152], bipolar disorder[153], and aphasia[154], cerebellar-targeted neuromodulation has been shown to improve related cognitive functions significantly. Although the precise targeting of specific cerebellar subregions or circuits for cognitive regulation still faces challenges, the potential of the cerebellum as a neuromodulation target for enhancing cognitive function in neurodegenerative diseases is likely underestimated and deserves more attention.
DECLARATIONS
Authors’ contributions
Literature search, writing, and original draft preparation: Xie YY
Conceptualization, review, revision, and editing: Zhu JN, Zhang XY
Availability of data and materials
Not applicable.
Financial support and sponsorship
This work was supported by the STI2030 Major Projects 2021ZD0202805; grants 32030044, 32171012, 31961160724, and 81671107 from the National Natural Science Foundation of China; grant BK20240168 and BK20190008 from the Natural Science Foundation of Jiangsu Province, China; the grant from the State Key Laboratory of Pharmaceutical Biotechnology (LNSN-202402), grants 020814380208 and 020814380197 from the Fundamental Research Funds for the Central Universities, China; and Nanjing University Integrated Research Platform of the Ministry of Education - Top Talents Program (2024300475).
Conflicts of interest
All authors declared that there are no conflicts of interest.
Ethical approval and consent to participate
Not applicable.
Consent for publication
Not applicable.
Copyright
© The Author(s) 2024.
REFERENCES
1. Zanatta A, Cherici C, Bargoni A, et al. Vincenzo Malacarne (1744-1816) and the first description of the human cerebellum. Cerebellum. 2018;17:461-4.
2. Pearce JM. Marie-Jean-Pierre Flourens (1794-1867) and cortical localization. Eur Neurol. 2009;61:311-4.
3. Turner WA. The cerebellum; new studies on normal and pathological physiology. J Anat Physiol. 1892;26:568-73.
4. Ferrier D, Turner WA. Record of experiments illustrative of the symptomatology and degenerations owing lesions of the cerebellum and its peduncles and related structures in nkeys. Proc R Soc Lond. 1894;185:719-78.
5. Russell JSR. II. Experimental researches into the functions of the cerebellum. Proc R Soc Lond. 1894;55:57-60.
7. Marie P. Cerebellar hereditary ataxia. Sem Med. 1893;13:444-7. Available from: https://scholar.google.com/scholar_lookup?&title=Sur%20l%E2%80%99h%C3%A9r%C3%A9do-ataxie%20c%C3%A9r%C3%A9belleuse&journal=Sem%20M%C3%A9d&volume=13&pages=444-447&publication_year=1893&author=Marie%2CP. [Last accessed on 13 Dec 2024].
8. Babinski J. Cerebellar asynergy. Rev Neurol. 1899;7:806-16. Available from: https://scholar.google.com/scholar?hl=en&as_sdt=0%2C5&q=Babinski+J.+De+l%E2%80%99asynergie+c%C3%A9r%C3%A9belleuse.+Rev+Neurol&btnG=. [Last accessed on 13 Dec 2024].
10. Holmes G. The symptoms of acute cerebellar injuries due to gunshot injuries. Brain. 1917;40:461-535.
12. Schmahmann JD. An emerging concept. The cerebellar contribution to higher function. Arch Neurol. 1991;48:1178-87.
14. Schmahmann JD. Dysmetria of thought: clinical consequences of cerebellar dysfunction on cognition and affect. Trends Cogn Sci. 1998;2:362-71.
15. Schmahmann JD. The role of the cerebellum in affect and psychosis. J Neurolinguist. 2000;13:189-214.
16. Schmahmann JD. Disorders of the cerebellum: ataxia, dysmetria of thought, and the cerebellar cognitive affective syndrome. J Neuropsych. 2004;16:367-78.
17. Schmahmann JD, Pandya DN. Anatomical investigation of projections to the basis pontis from posterior parietal association cortices in rhesus monkey. J Comp Neurol. 1989;289:53-73.
19. Middleton FA, Strick PL. Anatomical evidence for cerebellar and basal ganglia involvement in higher cognitive function. Science. 1994;266:458-61.
20. Levisohn L, Cronin-Golomb A, Schmahmann JD. Neuropsychological consequences of cerebellar tumour resection in children: cerebellar cognitive affective syndrome in a paediatric population. Brain. 2000;123:1041-50.
21. Manto M, Mariën P. Schmahmann’s syndrome - identification of the third cornerstone of clinical ataxiology. Cerebellum Ataxias. 2015;2:2.
22. Stoodley CJ, Valera EM, Schmahmann JD. Functional topography of the cerebellum for motor and cognitive tasks: an fMRI study. Neuroimage. 2012;59:1560-70.
23. Stoodley CJ, Valera EM, Schmahmann JD. An fMRI study of intra-individual functional topography in the human cerebellum. Behavioural Neurology. 2010;23:65-79.
25. Li T, Le W, Jankovic J. Linking the cerebellum to Parkinson disease: an update. Nat Rev Neurol. 2023;19:645-54.
26. Iskusnykh IY, Zakharova AA, Kryl’skii ED, Popova TN. Aging, neurodegenerative disorders, and cerebellum. Int J Mol Sci. 2024;25:1018.
27. Ding W, Ding LJ, Li FF, Han Y, Mu L. Neurodegeneration and cognition in Parkinson’s disease: a review. Eur Rev Med Pharmacol Sci. 2015;19:2275-81.
28. Schapira AHV, Chaudhuri KR, Jenner P. Non-motor features of Parkinson disease. Nat Rev Neurosci. 2017;18:435-50.
29. Gonzales MM, Garbarino VR, Pollet E, et al. Biological aging processes underlying cognitive decline and neurodegenerative disease. J Clin Invest. 2022;132:e158453.
31. Mugnaini E, Sekerková G, Martina M. The unipolar brush cell: a remarkable neuron finally receiving deserved attention. Brain Res Rev. 2011;66:220-45.
32. Stoodley CJ, MacMore JP, Makris N, Sherman JC, Schmahmann JD. Location of lesion determines motor vs. cognitive consequences in patients with cerebellar stroke. Neuroimage Clin. 2016;12:765-75.
33. Gok-Dursun E, Gultekin-Zaim OB, Tan E, Unal-Cevik I. Cognitive impairment and affective disorder: a rare presentation of cerebellar stroke. Clin Neurol Neurosurg. 2021;206:106690.
34. Bushara KO, Wheat JM, Khan A, et al. Multiple tactile maps in the human cerebellum. Neuroreport. 2001;12:2483-6.
35. Rijntjes M, Buechel C, Kiebel S, Weiller C. Multiple somatotopic representations in the human cerebellum. Neuroreport. 1999;10:3653-8.
36. Schmahmann JD. From movement to thought: anatomic substrates of the cerebellar contribution to cognitive processing. Hum Brain Mapp. 1996;4:174-98.
37. Kelly RM, Strick PL. Cerebellar loops with motor cortex and prefrontal cortex of a nonhuman primate. J Neurosci. 2003;23:8432-44.
38. Dum RP, Strick PL. An unfolded map of the cerebellar dentate nucleus and its projections to the cerebral cortex. J Neurophysiol. 2003;89:634-9.
39. Palesi F, Tournier JD, Calamante F, et al. Contralateral cerebello-thalamo-cortical pathways with prominent involvement of associative areas in humans in vivo. Brain Struct Funct. 2015;220:3369-84.
40. Palesi F, De Rinaldis A, Castellazzi G, et al. Contralateral cortico-ponto-cerebellar pathways reconstruction in humans in vivo: implications for reciprocal cerebro-cerebellar structural connectivity in motor and non-motor areas. Sci Rep. 2017;7:12841.
41. Guell X, Schmahmann JD, Gabrieli JDE, Ghosh SS. Functional gradients of the cerebellum. Elife. 2018;7:e36652.
42. Buckner RL, Krienen FM, Castellanos A, Diaz JC, Yeo BT. The organization of the human cerebellum estimated by intrinsic functional connectivity. J Neurophysiol. 2011;106:2322-45.
43. Middleton FA, Strick PL. Basal ganglia and cerebellar loops: motor and cognitive circuits. Brain Res Brain Res Rev. 2000;31:236-50.
44. Percheron G, François C, Talbi B, Yelnik J, Fénelon G. The primate motor thalamus. Brain Res Brain Res Rev. 1996;22:93-181.
46. Bostan AC, Dum RP, Strick PL. The basal ganglia communicate with the cerebellum. Proc Natl Acad Sci U S A. 2010;107:8452-6.
47. De Benedictis A, Rossi-Espagnet MC, de Palma L, Carai A, Marras CE. Networking of the human cerebellum: from anatomo-functional development to neurosurgical implications. Front Neurol. 2022;13:806298.
48. Quartarone A, Cacciola A, Milardi D, et al. New insights into cortico-basal-cerebellar connectome: clinical and physiological considerations. Brain. 2020;143:396-406.
49. Milardi D, Arrigo A, Anastasi G, et al. Extensive direct subcortical cerebellum-basal ganglia connections in human brain as revealed by constrained spherical deconvolution tractography. Front Neuroanat. 2016;10:29.
50. Doya K. Complementary roles of basal ganglia and cerebellum in learning and motor control. Curr Opin Neurobiol. 2000;10:732-9.
51. O’Doherty JP, Dayan P, Friston K, Critchley H, Dolan RJ. Temporal difference models and reward-related learning in the human brain. Neuron. 2003;38:329-37.
52. Silveri MC. Contribution of the cerebellum and the basal ganglia to language production: speech, word fluency, and sentence construction-evidence from pathology. Cerebellum. 2021;20:282-94.
53. Carta I, Chen CH, Schott AL, Dorizan S, Khodakhah K. Cerebellar modulation of the reward circuitry and social behavior. Science. 2019;363:eaav0581.
54. Watson TC, Obiang P, Torres-Herraez A, et al. Anatomical and physiological foundations of cerebello-hippocampal interaction. Elife. 2019;8:e41896.
55. Arrigo A, Mormina E, Anastasi GP, et al. Constrained spherical deconvolution analysis of the limbic network in human, with emphasis on a direct cerebello-limbic pathway. Front Hum Neurosci. 2014;8:987.
56. Fan L, Hu J, Ma W, Wang D, Yao Q, Shi J. Altered baseline activity and connectivity associated with cognitive impairment following acute cerebellar infarction: a resting-state fMRI study. Neurosci Lett. 2019;692:199-203.
57. James KA, Stromin JI, Steenkamp N, Combrinck MI. Understanding the relationships between physiological and psychosocial stress, cortisol and cognition. Front Endocrinol. 2023;14:1085950.
58. He YC, Wu GY, Li D, et al. Histamine promotes rat motor performances by activation of H2 receptors in the cerebellar fastigial nucleus. Behav Brain Res. 2012;228:44-52.
59. Zhang XY, Yu L, Zhuang QX, Zhang J, Zhu JN, Wang JJ. Hypothalamic histaminergic and orexinergic modulation on cerebellar and vestibular motor control. Cerebellum. 2013;12:294-6.
60. Li B, Zhu JN, Wang JJ. Histaminergic afferent system in the cerebellum: structure and function. Cerebellum Ataxias. 2014;1:5.
61. Zhang J, Zhuang QX, Li B, et al. Selective modulation of histaminergic inputs on projection neurons of cerebellum rapidly promotes motor coordination via HCN channels. Mol Neurobiol. 2016;53:1386-401.
62. Zhang XY, Wu WX, Shen LP, et al. A role for the cerebellum in motor-triggered alleviation of anxiety. Neuron. 2024;112:1165-81.e8.
63. Haines DE, Dietrichs E, Mihailoff GA, Mcdonald EF. The cerebellar-hypothalamic axis: basic circuits and clinical observations. Int Rev Neurobiol. 1997;41:83-107.
64. Zhu JN, Yung WH, Kwok-Chong Chow B, Chan YS, Wang JJ. The cerebellar-hypothalamic circuits: potential pathways underlying cerebellar involvement in somatic-visceral integration. Brain Res Rev. 2006;52:93-106.
66. Phelps EA. Emotion and cognition: insights from studies of the human amygdala. Annu Rev Psychol. 2006;57:27-53.
68. Petersen SE, Fox PT, Posner MI, Mintun M, Raichle ME. Positron emission tomographic studies of the cortical anatomy of single-word processing. Nature. 1988;331:585-9.
69. Moberget T, Gullesen EH, Andersson S, Ivry RB, Endestad T. Generalized role for the cerebellum in encoding internal models: evidence from semantic processing. J Neurosci. 2014;34:2871-8.
70. D’Mello AM, Turkeltaub PE, Stoodley CJ. Cerebellar tDCS modulates neural circuits during semantic prediction: a combined tDCS-fMRI study. J Neurosci. 2017;37:1604-13.
71. Yuan Q, Li H, Du B, et al. The cerebellum and cognition: further evidence for its role in language control. Cereb Cortex. 2022;33:35-49.
72. Larry N, Zur G, Joshua M. Organization of reward and movement signals in the basal ganglia and cerebellum. Nat Commun. 2024;15:2119.
73. Carruzzo F, Giarratana AO, Del Puppo L, Kaiser S, Tobler PN, Kaliuzhna M. Neural bases of reward anticipation in healthy individuals with low, mid, and high levels of schizotypy. Sci Rep. 2023;13:9953.
74. Li Y, Yang L, Li L, Xie Y, Fang P. The resting-state cerebro-cerebellar function connectivity and associations with verbal working memory performance. Behav Brain Res. 2022;417:113586.
75. Bezdicek O, Ballarini T, Albrecht F, et al. SERIAL-ORDER recall in working memory across the cognitive spectrum of Parkinson’s disease and neuroimaging correlates. J Neuropsychol. 2021;15:88-111.
76. Sako W, Abe T, Matsumoto Y, et al. The cerebellum is a common key for visuospatial execution and attention in Parkinson’s disease. Diagnostics. 2021;11:1042.
77. Tani T, Sakai Y. Stuttering after right cerebellar infarction: a case study. J Fluency Disord. 2010;35:141-5.
78. Guell X, Hoche F, Schmahmann JD. Metalinguistic deficits in patients with cerebellar dysfunction: empirical support for the dysmetria of thought theory. Cerebellum. 2015;14:50-8.
79. Wagner MJ, Kim TH, Savall J, Schnitzer MJ, Luo L. Cerebellar granule cells encode the expectation of reward. Nature. 2017;544:96-100.
80. Heffley W, Song EY, Xu Z, et al. Coordinated cerebellar climbing fiber activity signals learned sensorimotor predictions. Nat Neurosci. 2018;21:1431-41.
81. Heffley W, Hull C. Classical conditioning drives learned reward prediction signals in climbing fibers across the lateral cerebellum. Elife. 2019;8:e46764.
82. Kostadinov D, Beau M, Blanco-Pozo M, Häusser M. Predictive and reactive reward signals conveyed by climbing fiber inputs to cerebellar Purkinje cells. Nat Neurosci. 2019;22:950-62.
83. Larry N, Yarkoni M, Lixenberg A, Joshua M. Cerebellar climbing fibers encode expected reward size. Elife. 2019;8:e46870.
84. Ohmae S, Medina JF. Climbing fibers encode a temporal-difference prediction error during cerebellar learning in mice. Nat Neurosci. 2015;18:1798-803.
85. Kruithof ES, Klaus J, Schutter DJLG. The human cerebellum in reward anticipation and outcome processing: an activation likelihood estimation meta-analysis. Neurosci Biobehav Rev. 2023;149:105171.
86. Nicholas J, Amlang C, Lin CR, et al. The role of the cerebellum in learning to predict reward: evidence from cerebellar ataxia. Cerebellum. 2024;23:1355-68.
87. Jang DC, Shim HG, Kim SJ. Intrinsic plasticity of cerebellar purkinje cells contributes to motor memory consolidation. J Neurosci. 2020;40:4145-57.
88. Jang DC, Chung G, Kim SK, Kim SJ. Dynamic alteration of intrinsic properties of the cerebellar Purkinje cell during the motor memory consolidation. Mol Brain. 2023;16:58.
89. Chen X, Du Y, Broussard GJ, et al. Transcriptomic mapping uncovers Purkinje neuron plasticity driving learning. Nature. 2022;605:722-7.
90. Edde M, Di Scala G, Dupuy M, Dilharreguy B, Catheline G, Chanraud S. Learning-driven cerebellar intrinsic functional connectivity changes in men. J Neurosci Res. 2020;98:668-79.
91. Fibiger HC, Phillips AG. Mesocorticolimbic dopamine systems and reward. Ann N Y Acad Sci. 1988;537:206-15.
93. Yoshida J, Oñate M, Khatami L, Vera J, Nadim F, Khodakhah K. Cerebellar contributions to the basal ganglia influence motor coordination, reward processing, and movement vigor. J Neurosci. 2022;42:8406-15.
94. Hurley MJ, Mash DC, Jenner P. Markers for dopaminergic neurotransmission in the cerebellum in normal individuals and patients with Parkinson’s disease examined by RT-PCR. Eur J Neurosci. 2003;18:2668-72.
95. Giompres P, Delis F. Dopamine transporters in the cerebellum of mutant mice. Cerebellum. 2005;4:105-11.
96. Seese RR. Working memory impairments in cerebellar disorders of childhood. Pediatr Neurol. 2020;107:16-23.
97. Gottwald B, Wilde B, Mihajlovic Z, Mehdorn HM. Evidence for distinct cognitive deficits after focal cerebellar lesions. J Neurol Neurosurg Psychiatry. 2004;75:1524-31.
98. Ravizza SM, McCormick CA, Schlerf JE, Justus T, Ivry RB, Fiez JA. Cerebellar damage produces selective deficits in verbal working memory. Brain. 2006;129:306-20.
99. de Ribaupierre S, Ryser C, Villemure JG, Clarke S. Cerebellar lesions: is there a lateralisation effect on memory deficits?. Acta Neurochir. 2008;150:545-50; discussion 550.
100. Won J, Callow DD, Purcell JJ, Smith JC. Differential associations of regional cerebellar volume with gait speed and working memory. Sci Rep. 2022;12:2355.
101. Brissenden JA, Tobyne SM, Halko MA, Somers DC. Stimulus-specific visual working memory representations in human cerebellar lobule VIIb/VIIIa. J Neurosci. 2021;41:1033-45.
102. Yao J, Song B, Shi J, Yin K, Du W. Effects of repetitive transcranial magnetic stimulation at the cerebellum on working memory. Brain Sci. 2023;13:1158.
103. Viñas-Guasch N, Ng THB, Heng JG, et al. Cerebellar transcranial magnetic stimulation (TMS) impairs visual working memory. Cerebellum. 2023;22:332-47.
104. Botez MI. The neuropsychology of the cerebellum: an emerging concept. Arch Neurol. 1992;49:1229-30.
105. Molinari M, Leggio MG. Cerebellar information processing and visuospatial functions. Cerebellum. 2007;6:214-20.
106. Molinari M, Leggio MG, Solida A, et al. Cerebellum and procedural learning: evidence from focal cerebellar lesions. Brain. 1997;120:1753-62.
107. Petrosini L, Leggio MG, Molinari M. The cerebellum in the spatial problem solving: a co-star or a guest star?. Prog Neurobiol. 1998;56:191-210.
108. Joyal CC, Strazielle C, Lalonde R. Effects of dentate nucleus lesions on spatial and postural sensorimotor learning in rats. Behav Brain Res. 2001;122:131-7.
109. Locke TM, Soden ME, Miller SM, et al. Dopamine D1 receptor-positive neurons in the lateral nucleus of the cerebellum contribute to cognitive behavior. Biol Psychiatry. 2018;84:401-12.
110. Andre P, Zaccaroni M, Fiorenzani P, Della Seta D, Menzocchi M, Farabollini F. Offline consolidation of spatial memory: do the cerebellar output circuits play a role? A study utilizing a Morris water maze protocol in male Wistar rats. Brain Res. 2019;1718:148-58.
111. GBD 2015 Disease and Injury Incidence and Prevalence Collaborators. Global, regional, and national incidence, prevalence, and years lived with disability for 310 diseases and injuries, 1990-2015: a systematic analysis for the Global Burden of Disease Study 2015. Lancet. 2016;388:1545-602.
113. Andersen BB, Gundersen HJ, Pakkenberg B. Aging of the human cerebellum: a stereological study. J Comp Neurol. 2003;466:356-65.
114. Chen J, Cohen ML, Lerner AJ, Yang Y, Herrup K. DNA damage and cell cycle events implicate cerebellar dentate nucleus neurons as targets of Alzheimer’s disease. Mol Neurodegener. 2010;5:60.
115. Tripathi SM, Murray AD, Wischik CM, Schelter B. Crossed cerebellar diaschisis in Alzheimer’s disease. Nucl Med Commun. 2022;43:423-7.
116. Mirino P, Pecchinenda A, Boccia M, Capirchio A, D’Antonio F, Guariglia C. Cerebellum-cortical interaction in spatial navigation and its alteration in dementias. Brain Sci. 2022;12:523.
117. Yao Q, Tang F, Wang Y, et al. Effect of cerebellum stimulation on cognitive recovery in patients with Alzheimer disease: a randomized clinical trial. Brain Stimul. 2022;15:910-20.
118. Liang KJ, Carlson ES. Resistance, vulnerability and resilience: a review of the cognitive cerebellum in aging and neurodegenerative diseases. Neurobiol Learn Mem. 2020;170:106981.
119. Xu HT, Xi XY, Zhou S, et al. Histaminergic innervation of the ventral anterior thalamic nucleus alleviates motor deficits in a 6-OHDA-induced rat model of Parkinson’s disease. Neurosci Bull 2024.
120. Solano SM, Miller DW, Augood SJ, Young AB, Penney JB Jr. Expression of alpha-synuclein, parkin, and ubiquitin carboxy-terminal hydrolase L1 mRNA in human brain: genes associated with familial Parkinson’s disease. Ann Neurol. 2000;47:201-10.
121. Seidel K, Bouzrou M, Heidemann N, et al. Involvement of the cerebellum in Parkinson disease and dementia with Lewy bodies. Ann Neurol. 2017;81:898-903.
122. Thomasson M, Benis D, Voruz P, et al. Crossed functional specialization between the basal ganglia and cerebellum during vocal emotion decoding: insights from stroke and Parkinson’s disease. Cogn Affect Behav Neurosci. 2022;22:1030-43.
123. Riou A, Houvenaghel JF, Dondaine T, et al. Functional role of the cerebellum in Parkinson disease: a PET study. Neurology. 2021;96:e2874-84.
124. Arioli M, Cattaneo Z, Rusconi ML, Blandini F, Tettamanti M. Action and emotion perception in Parkinson’s disease: a neuroimaging meta-analysis. Neuroimage Clin. 2022;35:103031.
125. Grimaldi G, Manto M. Anodal transcranial direct current stimulation (tDCS) decreases the amplitudes of long-latency stretch reflexes in cerebellar ataxia. Ann Biomed Eng. 2013;41:2437-47.
126. Grimaldi G, Oulad Ben Taib N, Manto M, Bodranghien F. Marked reduction of cerebellar deficits in upper limbs following transcranial cerebello-cerebral DC stimulation: tremor reduction and re-programming of the timing of antagonist commands. Front Syst Neurosci. 2014;8:9.
127. Benussi A, Koch G, Cotelli M, Padovani A, Borroni B. Cerebellar transcranial direct current stimulation in patients with ataxia: a double-blind, randomized, sham-controlled study. Mov Disord. 2015;30:1701-5.
128. Benussi A, Dell’Era V, Cotelli MS, et al. Long term clinical and neurophysiological effects of cerebellar transcranial direct current stimulation in patients with neurodegenerative ataxia. Brain Stimul. 2017;10:242-50.
129. Bodranghien F, Oulad Ben Taib N, Van Maldergem L, Manto M. A postural tremor highly responsive to transcranial cerebello-cerebral DCS in ARCA3. Front Neurol. 2017;8:71.
130. Grecco LA, Oliveira CS, Duarte NA, Lima VL, Zanon N, Fregni F. Cerebellar transcranial direct current stimulation in children with ataxic cerebral palsy: a sham-controlled, crossover, pilot study. Dev Neurorehabil. 2017;20:142-8.
131. Benussi A, Dell’Era V, Cantoni V, et al. Cerebello-spinal tDCS in ataxia: a randomized, double-blind, sham-controlled, crossover trial. Neurology. 2018;91:e1090-101.
132. Benussi A, Cantoni V, Manes M, et al. Motor and cognitive outcomes of cerebello-spinal stimulation in neurodegenerative ataxia. Brain. 2021;144:2310-21.
133. Naeije G, Rovai A, Destrebecq V, Trotta N, De Tiège X. Anodal cerebellar transcranial direct current stimulation reduces motor and cognitive symptoms in Friedreich’s ataxia: a randomized, sham-controlled trial. Mov Disord. 2023;38:1443-50.
134. Farzan F, Wu Y, Manor B, et al. Cerebellar TMS in treatment of a patient with cerebellar ataxia: evidence from clinical, biomechanics and neurophysiological assessments. Cerebellum. 2013;12:707-12.
135. Bonnì S, Ponzo V, Caltagirone C, Koch G. Cerebellar theta burst stimulation in stroke patients with ataxia. Funct Neurol 2014. Available from: https://www.semanticscholar.org/paper/Cerebellar-theta-burst-stimulation-in-stroke-with-Bonn%C3%AC-Ponzo/90efef491f1758d1471bac14c93fdac91256097f. [Last accessed on 11 Dec 2024].
136. Kim WS, Jung SH, Oh MK, Min YS, Lim JY, Paik NJ. Effect of repetitive transcranial magnetic stimulation over the cerebellum on patients with ataxia after posterior circulation stroke: a pilot study. J Rehabil Med. 2014;46:418-23.
137. Cury RG, Teixeira MJ, Galhardoni R, et al. Neuronavigation-guided transcranial magnetic stimulation of the dentate nucleus improves cerebellar ataxia: a sham-controlled, double-blind n = 1 study. Parkinsonism Relat Disord. 2015;21:999-1001.
138. Dang G, Su X, Zhou Z, et al. Beneficial effects of cerebellar rTMS stimulation on a patient with spinocerebellar ataxia type 6. Brain Stimul. 2019;12:767-9.
139. França C, de Andrade DC, Silva V, et al. Effects of cerebellar transcranial magnetic stimulation on ataxias: a randomized trial. Parkinsonism Relat Disord. 2020;80:1-6.
140. Teixeira MJ, Cury RG, Galhardoni R, et al. Deep brain stimulation of the dentate nucleus improves cerebellar ataxia after cerebellar stroke. Neurology. 2015;85:2075-6.
141. Miterko LN, Lin T, Zhou J, et al. Neuromodulation of the cerebellum rescues movement in a mouse model of ataxia. Nat Commun. 2021;12:1295.
142. Ferrucci R, Cortese F, Bianchi M, et al. Cerebellar and motor cortical transcranial stimulation decrease levodopa-induced dyskinesias in Parkinson’s disease. Cerebellum. 2016;15:43-7.
143. Málly J, Stone TW, Sinkó G, Geisz N, Dinya E. Long term follow-up study of non-invasive brain stimulation (NBS) (rTMS and tDCS) in Parkinson’s disease (PD). Strong age-dependency in the effect of NBS. Brain Res Bull. 2018;142:78-87.
144. Workman CD, Fietsam AC, Uc EY, Rudroff T. Cerebellar transcranial direct current stimulation in people with Parkinson’s disease: a pilot study. Brain Sci. 2020;10:96.
145. Koch G, Brusa L, Carrillo F, et al. Cerebellar magnetic stimulation decreases levodopa-induced dyskinesias in Parkinson disease. Neurology. 2009;73:113-9.
146. Minks E, Mareček R, Pavlík T, Ovesná P, Bareš M. Is the cerebellum a potential target for stimulation in Parkinson’s disease? Results of 1-Hz rTMS on upper limb motor tasks. Cerebellum. 2011;10:804-11.
147. Brusa L, Ceravolo R, Kiferle L, et al. Metabolic changes induced by theta burst stimulation of the cerebellum in dyskinetic Parkinson’s disease patients. Parkinsonism Relat Disord. 2012;18:59-62.
148. Bologna M, Di Biasio F, Conte A, Iezzi E, Modugno N, Berardelli A. Effects of cerebellar continuous theta burst stimulation on resting tremor in Parkinson’s disease. Parkinsonism Relat Disord. 2015;21:1061-6.
149. Di Biasio F, Conte A, Bologna M, et al. Does the cerebellum intervene in the abnormal somatosensory temporal discrimination in Parkinson’s disease?. Parkinsonism Relat Disord. 2015;21:789-92.
150. Lefaivre SC, Brown MJ, Almeida QJ. Cerebellar involvement in Parkinson’s disease resting tremor. Cerebellum Ataxias. 2016;3:13.
151. Di Lorenzo F, Martorana A, Ponzo V, et al. Cerebellar theta burst stimulation modulates short latency afferent inhibition in Alzheimer’s disease patients. Front Aging Neurosci. 2013;5:2.
152. Demirtas-Tatlidede A, Freitas C, Cromer JR, et al. Safety and proof of principle study of cerebellar vermal theta burst stimulation in refractory schizophrenia. Schizophr Res. 2010;124:91-100.
153. Minichino A, Bersani FS, Bernabei L, et al. Prefronto-cerebellar transcranial direct current stimulation improves visuospatial memory, executive functions, and neurological soft signs in patients with euthymic bipolar disorder. Neuropsychiatr Dis Treat. 2015;11:2265-70.
154. Sebastian R, Saxena S, Tsapkini K, et al. Cerebellar tDCS: a novel approach to augment language treatment post-stroke. Front Hum Neurosci. 2016;10:695.
155. Stezin A, Bhardwaj S, Hegde S, et al. Cognitive impairment and its neuroimaging correlates in spinocerebellar ataxia 2. Parkinsonism Relat Disord. 2021;85:78-83.
156. Gigante AF, Lelli G, Romano R, et al. The relationships between ataxia and cognition in spinocerebellar ataxia type 2. Cerebellum. 2020;19:40-7.
157. Cundari M, Vestberg S, Gustafsson P, Gorcenco S, Rasmussen A. Neurocognitive and cerebellar function in ADHD, autism and spinocerebellar ataxia. Front Syst Neurosci. 2023;17:1168666.
158. Orsi L, D’Agata F, Caroppo P, et al. Neuropsychological picture of 33 spinocerebellar ataxia cases. J Clin Exp Neuropsychol. 2011;33:315-25.
159. Naeije G, Schulz JB, Corben LA. The cognitive profile of Friedreich ataxia: a systematic review and meta-analysis. BMC Neurol. 2022;22:97.
160. Radmard S, Zesiewicz TA, Kuo SH. Evaluation of cerebellar ataxic patients. Neurol Clin. 2023;41:21-44.
161. Karamazovova S, Matuskova V, Ismail Z, Vyhnalek M. Neuropsychiatric symptoms in spinocerebellar ataxias and Friedreich ataxia. Neurosci Biobehav Rev. 2023;150:105205.
162. Lin CR, Kuo SH, Opal P. Cognitive, emotional, and other non-motor symptoms of spinocerebellar ataxias. Curr Neurol Neurosci Rep. 2024;24:47-54.
163. Stanley AT, Post MR, Lacefield C, Sulzer D, Miniaci MC. Norepinephrine release in the cerebellum contributes to aversive learning. Nat Commun. 2023;14:4852.
164. Li ZH, Li B, Zhang XY, Zhu JN. Neuropeptides and their roles in the cerebellum. Int J Mol Sci. 2024;25:2332.
165. Shen LP, Li W, Pei LZ, et al. Oxytocin receptor in cerebellar purkinje cells does not engage in autism-related behaviors. Cerebellum. 2023;22:888-904.
166. Wang Y, Chen ZP, Zhuang QX, et al. Role of corticotropin-releasing factor in cerebellar motor control and ataxia. Curr Biol. 2017;27:2661-69.e5.
167. Yu L, Zhang XY, Zhang J, Zhu JN, Wang JJ. Orexins excite neurons of the rat cerebellar nucleus interpositus via orexin 2 receptors in vitro. Cerebellum. 2010;9:88-95.
168. Cotelli M, Calabria M, Manenti R, et al. Improved language performance in Alzheimer disease following brain stimulation. J Neurol Neurosurg Psychiatry. 2011;82:794-7.
169. Ahmed MA, Darwish ES, Khedr EM, El Serogy YM, Ali AM. Effects of low versus high frequencies of repetitive transcranial magnetic stimulation on cognitive function and cortical excitability in Alzheimer’s dementia. J Neurol. 2012;259:83-92.
170. Drumond Marra HL, Myczkowski ML, Maia Memória C, et al. Transcranial magnetic stimulation to address mild cognitive impairment in the elderly: a randomized controlled study. Behav Neurol. 2015;2015:287843.
171. Rabey JM, Dobronevsky E. Repetitive transcranial magnetic stimulation (rTMS) combined with cognitive training is a safe and effective modality for the treatment of Alzheimer’s disease: clinical experience. J Neural Transm. 2016;123:1449-55.
172. Zhao J, Li Z, Cong Y, et al. Repetitive transcranial magnetic stimulation improves cognitive function of Alzheimer’s disease patients. Oncotarget. 2017;8:33864-71.
173. Koch G, Bonnì S, Pellicciari MC, et al. Transcranial magnetic stimulation of the precuneus enhances memory and neural activity in prodromal Alzheimer’s disease. Neuroimage. 2018;169:302-11.
174. Padala PR, Padala KP, Lensing SY, et al. Repetitive transcranial magnetic stimulation for apathy in mild cognitive impairment: a double-blind, randomized, sham-controlled, cross-over pilot study. Psychiatry Res. 2018;261:312-8.
175. Zhang F, Qin Y, Xie L, Zheng C, Huang X, Zhang M. High-frequency repetitive transcranial magnetic stimulation combined with cognitive training improves cognitive function and cortical metabolic ratios in Alzheimer’s disease. J Neural Transm. 2019;126:1081-94.
176. Bagattini C, Zanni M, Barocco F, et al. Enhancing cognitive training effects in Alzheimer’s disease: rTMS as an add-on treatment. Brain Stimul. 2020;13:1655-64.
177. Brem AK, Di Iorio R, Fried PJ, et al. Corticomotor plasticity predicts clinical efficacy of combined neuromodulation and cognitive training in Alzheimer’s disease. Front Aging Neurosci. 2020;12:200.
178. Padala PR, Boozer EM, Lensing SY, et al. Neuromodulation for apathy in Alzheimer’s disease: a double-blind, randomized, sham-controlled pilot study. J Alzheimers Dis. 2020;77:1483-93.
Cite This Article

How to Cite
Download Citation
Export Citation File:
Type of Import
Tips on Downloading Citation
Citation Manager File Format
Type of Import
Direct Import: When the Direct Import option is selected (the default state), a dialogue box will give you the option to Save or Open the downloaded citation data. Choosing Open will either launch your citation manager or give you a choice of applications with which to use the metadata. The Save option saves the file locally for later use.
Indirect Import: When the Indirect Import option is selected, the metadata is displayed and may be copied and pasted as needed.
About This Article
Special Issue
Copyright
Data & Comments
Data
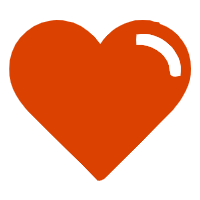
Comments
Comments must be written in English. Spam, offensive content, impersonation, and private information will not be permitted. If any comment is reported and identified as inappropriate content by OAE staff, the comment will be removed without notice. If you have any queries or need any help, please contact us at [email protected].