Examining the effect of preoperative antibiotics for skin grafting surgery on the microbiome in burn patients: a pilot study
Abstract
Aim: Severely burn injured patients present in an immunocompromised state with loss of skin integrity. Systemic antibiotics are necessary to treat infection in this population, but even small doses may select resistant organisms and alter the host microbiome. Despite this, preoperative antibiotics in burn injury remain debated and their impact on the host microbiome has not been fully elucidated. This work examined the effect of a single preoperative antibiotic dose on the microbiome and clinical outcomes in burn patients.
Methods: Patients with burns < 10% total body surface area (TBSA) requiring a single excision and grafting operation were enrolled and randomized to receive a single preoperative dose of cefazolin (ABX) or no antibiotics (no-ABX). Blood samples, wound swabs, and buccal swabs were obtained serially during and after hospitalization to determine bacterial taxonomy characteristics. Graft loss was determined at clinic follow-up.
Results: In ABX patients, there were 29 enriched bacterial taxa within wound beds at dressing takedown [log(LDA) ≥ 2, P ≤ 0.05] and greater than 10 bacterial taxa in buccal swabs at follow-up [log(LDA) ≥ 2, P ≤ 0.05]. There was increasing alpha diversity in ABX patients intraoperatively and at dressing takedown. There were no significant differences in graft loss between groups. Extremophile infiltration was noted in oral and wound microbiomes in ABX patients.
Conclusion: Though not affected clinically, these data suggest that a single preoperative antibiotic dose significantly impacts the wound and oral microbiome. Innovative approaches to examining the antibiotic impact on the host microbiome of burn patients may help better tailor antibiotic stewardship.
Keywords
INTRODUCTION
Advances in burn care and wound management in recent decades, including the use of topical antimicrobials, improved resuscitation strategies, judicious systemic antibiotics, and early excision and grafting, have resulted in improved wound healing and mortality. However, burn wound sepsis remains a significant cause of morbidity and mortality, accounting for approximately 50% of all burn-related deaths[1]. Disruption of the skin barrier due to burn injury attenuates homeostasis mechanisms and represents an entry point for bacteria, fungi, and viruses. Increased burn injury size is correlated with increased risk for local and systemic infection[2-4]. Dermal ischemia and avascular necrotic tissue provide a protein-rich environment that is advantageous for the colonization of microbes[5]. Rapid onset of burn wound edema promotes an infectious environment by deoxygenating surrounding tissues. This environment, along with an early systemic hyperinflammatory response, increases vasodilation and vascular permeability, leading to microvascular leakage[3-6]. A dysregulation of homeostatic mechanisms follows, resulting in both local and systemic responses to injury[7].
Burn-injured patients present in a more immunocompromised state due to the significant inflammatory response that follows their injury and the loss of their skin integrity, which functions as a natural barrier against infection. Initial burn wound colonization is gram-positive in nature as commensal colonizers of the skin easily infect the wound and include species such as Staphylococcus, Enterococcus, Streptococcus, Corynebacterium, and Micrococcus species (spp.)[2,8-11]. Due to the induction of gut permeability in more severe burn injuries, the microbial community eventually shifts to a predominantly gram-negative environment with Pseudomonas aeruginosa, Acinetobacter baumannii, Escherichia coli, Enterobacter cloacae, Klebsiella spp., and Enterococcus spp. representing common pathogens of infection. Multi-drug resistant organisms (MDRO) such as methicillin-resistant Staphylococcus aureus (MRSA), resistant Acinetobacter, and Pseudomonas spp. may develop during prolonged hospital stays and pose a significant risk to morbidity and mortality in patients with severe burn injury[2,8-12]. Extended hospitalization facilitates transmission of MDRO, leading to hospital-acquired infections (HAI). Therapy for these microbes is confounded by their drug resistance, requiring increasing demand for the development of novel antibiotics and meticulous isolation protocols[2]. Early tangential burn wound excision reduces the interval between injury and removal of the damaged, inflammatory necrotic tissue, and subsequent closure through skin grafting helps attenuate this risk[10]. However, wound infections, particularly from common MDROs like MRSA or other microbes, contribute to significant graft loss and wound healing morbidity. Studies on the microbiome in patients with burn injury are limited, and even fewer have examined the effect of antibiotics on the microbiome in this population[12]. Even small or short-course antibiotics may select resistant organisms that can become problematic for antibiotic selection later in a patient’s hospital course[1,2,13].
Examination of small or short-course antibiotic use in burn-injured patients may provide a novel and innovative way to examine the influence of antibiotics on outcomes and the microbiome. One such example of short-course antibiotic use is in preoperative antibiotic selection. Much has been written about the benefits of preoperative antibiotic use to avoid surgical site infections and other complications in abdominal surgery. However, the role of systemic antibiotic prophylaxis is debated in burn care. Preoperative antibiotics, in general, are employed for the prevention of surgically acquired infections. It has been suggested that the timing of these antibiotics helps reduce wound bed infection, maintain graft adherence, and prevent more invasive bloodstream infections from occurring[14-16]. However, duration postoperatively, dose, and antimicrobial selection are still debated. In a review by Ramos et al., the use of systemic antibiotic prophylaxis for burn patients requiring mechanical ventilation and/or split-thickness skin grafts was suggested to be beneficial[17]. In a study conducted from October 2001 to October 2006, the autograft survival rate was 97% in patients with preoperative and perioperative antibiotic administration, whereas it was significantly lower at 87% in those with no antibiotic treatment[15]. Skin colonizing microbiota may play a role in maintaining skin homeostasis but may contribute to aberrant wound healing when disrupted[18]. One study by Jung et al. noted permanent changes in healed skin microbiomes following burn injury and suggested that specific skin microorganisms correlated with biomechanical scar dynamics[19].
Empiric antibiotic use is sometimes employed in the management of burn wounds following mass casualty incidents, or in severe burns with concomitant trauma where burn wounds are more likely to be contaminated by contact with the external environment[11,20-22]. In mass casualty incidents, adherence to the well-studied standards of burn care, such as frequent dressing changes with topical antimicrobials and early excision and grafting, becomes difficult secondary to logistic constraints in the delivery of nursing and surgical care, respectively. Hemodynamic and respiratory stabilization is often a longer process in these cases when concurrent traumas may need to be assessed as well. These combined factors make systemic antibiotic treatment favorable even before wounds are cultured to proactively counteract the expected onset of infection[11,20,21,23]. One study investigating the use of systemic antibiotic prophylaxis after a mass casualty burn incident caused by a cornstarch explosion demonstrated a 45% reduction in wound infection rate at one week postburn. Moreover, despite the development of bloodstream infections in some patients, no mortality occurred in more severe higher total body surface area (TBSA) burn patients[11].
Despite these findings, literature on the long-term effects of systemic antibiotic administration in burn patients is limited. In the previously mentioned study on burn mass casualty, though antibiotic prophylaxis usage lowered rates of wound infection, it was associated with the development of fungal and MDRO infections[11]. In another study, systemic antibiotic prophylaxis was associated with subsequent growth of
METHODS
Study protocol, inclusion and exclusion criteria
The protocol was approved by Medstar Health Research Institute’s Institutional Review Board (Protocol #2017-186). This single-site, randomized pilot study was conducted at MedStar Washington Hospital Center (MWHC) from 2018-2020. During this period, 31 patients were enrolled. Patients were randomized into two groups and blinded to their intervention status. Randomization was achieved using a computerized random number generator. Patients and assessors remained blinded throughout the study. Cefazolin is a common preoperative antibiotic used due to its coverage of common skin flora organisms, wide availability, and ease of administration within the perioperative setting. This antibiotic was chosen for the study due to its availability at our institution. Preoperative antibiotic administration was chosen over perioperative antibiotic use, where antibiotics could be administered following operative intervention for better experimental control. One group (ABX) received a single dose of weight-based cefazolin upon induction of anesthesia. Patients weighing less than 120 kg received 2 g of cefazolin intravenously. Patients over 120 kg received 3 g of cefazolin. Patients were re-dosed every 4 h if they remained in the operating room. The non-antibiotics group (no-ABX) received the standard of care at this institution, which does not include perioperative antibiotics.
For all eligible patients included, informed consent was obtained before study participation. Subjects included in the study were 18 years or older at the time of consent and presented to our institution within 48 h after sustaining a thermal injury. Patients with a TBSA of ≤ 10% who were anticipated to require a single grafting procedure were considered eligible. Patients with TBSA burn sizes above this often have earlier and more complications compared to lower-sized TBSA injuries that may include earlier infections of various sources and earlier antibiotic administration. A smaller TBSA burn injury allowed for better experimental control over the administration of a single antibiotic dose, with closely monitored follow-up and minimization of complications related to the injury. Patients were excluded from the study if they received antibiotics within 30 days prior to admission, already had burn wound cellulitis or an infection diagnosed preoperatively, or an infection discovered during burn excision. Incarcerated individuals and/or a positive pregnancy test in females were also excluded from the study.
All excision and grafting procedures were performed early, within approximately 72 to 96 h after the burn injury. This minimized the risk of microbial colonization over time, which could have affected clinical outcomes or microbiome analysis. Following burn wound excision, an attending surgeon assessed the wound bed and decided on either porcine xenograft or autograft. Any burn that was anticipated to require autografting but was treated with an interval xenograft was still included in the study[25]. Split-thickness skin grafts were obtained using a dermatome (Humeca, Woodstock, GA) at a depth of 0.008-0.012 and then meshed at expansion ratios of 1:1, 2:1, or 3:1, depending on the size and location of the wound (Brennan Medical, St. Paul, MN). Petroleum jelly-impregnated non-adherent dressings were then applied. All autografts were dressed with a negative pressure wound therapy device (KCI, San Antonio, TX) set to
Sample collection
Given the systemic inflammatory response that follows burn injury, there is potential for multiple microbiomes to be affected on a given host. Among organs, gut permeability increases in severe burn injury and may provide a nidus for gram-negative bacterial translocation. Although a host may harbor diverse microbiomes, an interrelationship can exist among them due to the inflammatory processes that follow burn injuries, which may make them comparable to one another. Sampling areas such as the buccal microbiome can be an indicator of gut microbiome taxonomy or behavior. To observe this over time, biological samples including wound swabs, buccal swabs, and blood samples were taken at serial time points from each patient: intraoperatively prior to wound bed preparation (pre-excision), post-excision, during the initial postoperative dressing takedown (1-3 days post grafting), and follow-up (7-10 days post discharge). Digital photography was also performed at each time point.
Swabs of the wound bed and donor site were obtained using BD BBLTM CultureSwabTM (Becton Dickinson, Franklin Lakes, NJ). Swabs were deposited in iSwab microbiome collection tubes (Mawi DNA Technologies, Hayward, CA) and stored at -20 °C for 16S rRNA analysis[26]. Buccal swabs were collected, deposited in iSwab microbiome collection tubes (Mawi), and stored at -20 °C for 16S rRNA analysis.
DNA isolation from iSwabs
The ZymoBIOMICS DNA/RNA Miniprep kit (Zymo Research, Irvine, CA) was used to extract DNA from samples per the manufacturer’s protocol and eluted using 50 µL of DNase/RNase-free water. DNA concentrations in extracts were then quantified using an Invitrogen Qubit 4 Fluorometer and 1X Qubit dsDNA High Sensitivity Assay Kit (ThermoFisher Scientific, Waltham, MA). All 16S rRNA illumina-tag PCR reactions were performed on DNA extracts per the Earth Microbiome Project’s protocol[27]. Negative controls (PCR grade nuclease-free water) were processed along with DNA extracts for PCR amplification. PCR products and negatives were pooled. Gel purification was done with the pool and a 2% agarose gel using the QIAquick Gel Purification Kit (Qiagen, Frederick, MD). The purified pool was subsequently assessed for quality using an Agilent 2100 BioAnalyzer and Agilent DNA High Sensitivity DNA kit (Agilent Technologies, Santa Clara, CA). The purified pool was stored at -20 °C and then shipped to Laragen Inc. (Culver City, CA), which sequenced it using an Illumina MiSeq with v2 chemistry to generate 250 base pair paired-end reads.
16S rRNA data pre-processing
QIIME2 was used to process and analyze raw data[28]. Cumulative expected error for each position was determined with VSEARCH[29]. QIIME2’s implementation of the DADA2 pipeline was used to subject raw sequence data to quality filtering, pairing, chimera removal, and ASV grouping. Based on VSEARCH’s calculated expected errors, forward reads were truncated at a length of 211, with a maximum expected error of 0.5, and reverse reads were truncated at a length of 196, with a maximum expected error of 0.5. This produced a table of ASVs and a file containing one representative sequence for each ASV.
Taxonomy was assigned to the representative sequences with a Naive Bayes classifier implemented in QIIME2 and a pre-trained Silva 138 database containing 515F/806R sequences[30]. A rooted phylogenetic tree was created with the representative sequences as well, with QIIME2’s implementation of MAFFT and FastTree[31,32]. The decontam package in R (R Core Team 2020) was used to identify likely contaminants based on the 11 negatives[33,34]. The ASVs identified as contaminants and negatives were then removed from the table. ASVs identified as mitochondria or chloroplasts were also filtered out on the basis that they likely represented eukaryotic contamination, instead of true bacterial signal. Lastly, samples with fewer than 1,000 sequences after all filtering steps were removed.
Diversity and differential abundance analysis
Alpha diversity was calculated by subsampling the ASV table at 10 different depths (100 to 1,000 sequences) for Faith’s Phylogenetic Diversity, Observed Features, and Pielou’s Evenness metrics[35,36]. At every depth, twenty iterations were performed, with their results being averaged for each of the different metrics. A rarefaction plot was created to confirm that diversity approached an asymptote and slope decreased as sampling depth increased. Averages for the greatest depth (1,000 sequences) were used to determine if any of the alpha diversity metrics differed significantly based on the antibiotics group (Yes/No) within the same time point (Intraop, Dressing, and Follow-Up) (Kruskal-Wallis, P ≤ 0.05). Kruskal-Wallis tests were also performed to evaluate overall alpha diversity among all groups within the same sample type.
Beta diversity analysis was conducted with the ASV table, which was first normalized with the cumulative sum scaling method[37]. Distances between samples were calculated using the weighted Unifrac metric based on the normalized table and rooted tree and visualized as a Principal Coordinates Analysis (PCoA) plot for both buccal swab and wound bed swab samples[38]. Statistical differences within time points based on antibiotics were evaluated, as well as overall differences among all groups within the same sample type (PERMANOVA, P ≤ 0.05).
LEfSe was used to identify taxa that had significantly different abundances based on the administration of antibiotics within the buccal swab and wound bed swab time points[39]. The ASV table was collapsed to the species level and normalized with the counts per million method. Enriched taxa were those taxa that were identified as having significantly differential abundance (Kruskal-Wallis, P ≤ 0.05) with a log(LDA) score of at least 2.0.
Assessing graft loss and graft-loss grading scale
Digital pictures were used to assess reepithelization in autografted and xenografted wounds by three independent, blinded burn surgery attendings at patient follow-up visits. The percentage of re-epithelialization was assessed from zero to 100% and the grade of graft loss was determined. Graft loss grading was determined based on a previously published scale, as summarized in Table 1[40]. The percentage of re-epithelialization and graft loss grade were then collated from assessors and summarized for comparison purposes.
Graft loss grading scale
Grade | Area lost | Topical wound care required | Surgery indicated |
I | Minimal | - | - |
II | < 50% | +/- | - |
III | < 50% | + | +/- |
IV | > 50% | + | + |
Statistical analysis
Statistical analyses were performed using GraphPad Prism version 9.0. with P ≤ 0.05 deemed as statistically significant. Student’s t-tests were used to compare percent reepithelization between ABX or non-ABX autografted and xenografted wounds. Results are reported as standard error of the mean (SEM). Univariate analysis was used to compare relationships in demographic data. Culture data were assessed for normality using Anderson-Darling normality tests and all data were nonparametric. Un-paired Mann-Whitney tests were used to assess culture data and nonparametric data are presented as median and interquartile range.
RESULTS
Patient demographics
Of the patients assessed for eligibility, 31 patients were prospectively studied [Figure 1]. The average time from admission to OR was 2.2 days (IQR, 1-3). Patients had a mean age of 40.8 ± 14.2, with the majority being male (64.5%). The mean TBSA was 3.4 ± 2.8. Of the study participants, 13 received antibiotics (41.9%) and 18 received center-specific standard of care (58.1%). Of the 31 patients, 18 patients received autografts and 13 received xenografts. Among the ABX group, about half were autografted (n = 7, 53.9%) and the other half were xenografted (n = 6, 46.2%). In the no-ABX group, 11 (61.1%) patients received autografts and seven (38.9%) received xenografts. Between the ABX and no-ABX groups, there was no difference in length of stay (LOS), with the average hospital LOS being 5.8 days (P = 0.26). Demographic information is summarized in Table 2.
Demographics and injury characteristics
Characteristic | All | ABX group | No-ABX group | P-value |
No. of patients, No. (%) | 31 (100%) | 13 (41.9%) | 18 (58.1%) | |
Male, No. (%) | 20 (64.5%) | 6 (46.2%) | 14 (77.8%) | 0.12 |
Age, year, mean ± SD | 40.8 ± 14.2 | 44.5 ± 12.5 | 38.2 ± 15.0 | 0.22 |
Ethnicity, No. (%) | 0.65 | |||
Caucasian | 10 (32.3%) | 4 (30.8%) | 6 (33.3%) | |
African American | 16 (51.6%) | 7 (53.8%) | 9 (50%) | |
Hispanic | 3 (9.7%) | 1 (7.7%) | 2 (11.1%) | |
Other | 1 (3.2%) | 1 (7.7%) | 0 (0%) | |
Burn location, No. (%) | ||||
Hand | 11 (35.5%) | 3 (23.1%) | 8 (44.4%) | |
Arm | 6 (19.4%) | 2 (15.4%) | 4 (22.2%) | |
Chest | 1 (3.2%) | 1 (7.7%) | 0 (0.0%) | |
Abdomen | 1 (3.2%) | 1 (7.7%) | 0 (0.0%) | |
Back | 3 (9.7%) | 2 (15.4%) | 1 (5.6%) | |
Leg | 6 (19.4%) | 3 (23.1%) | 3 (16.7%) | |
Foot | 3 (9.7%) | 1 (7.7%) | 2 (11.1%) | |
BMI, mean ± SD | 29.4 ± 5.2 | 30.5 ± 5.3 | 28.6 ± 5.2 | 0.32 |
Autograft, No. (%) | 18 (58.1%) | 7 (53.8%) | 11(61.1%) | 0.73 |
%TBSA burned, median (IQR) | 3.4 (1.5-5.5) | 3.5 (1.5-5.8) | 3.3 (1-5.6) | 0.83 |
Time ADM to OR (days), median (IQR) | 2 (1-3) | 2 (1.5-3) | 2 (1-3) | 0.81 |
LOS (days), median (IQR) | 6 (4-7) | 5 (3-6) | 6.5 (4-8) | 0.26 |
NGS analysis of buccal and wound bed bacterial composition
Sequencing of the 16S rRNA hypervariable V4 region generated over 13,000,000 sequences, allowing for robust analysis of community composition as it related to antibiotics administration with the sample types (buccal swab and wound bed swab) and time point (intraop, dressing takedown, and follow-up). After quality filtering and decontamination, sequence counts ranged from 1,299-122,456 per sample, with a total of eighty-three buccal swab and one hundred and six wound bed swab samples being used for downstream analysis.
Antibiotic administration and its impact on overall diversity
Alpha diversity among the buccal swab samples did not differ significantly for any of the three metrics (Faith’s Phylogenetic Diversity, Observed Features, and Pielou’s Evenness) based on ABX administration, with the exception of dressing takedown, in which the ABX group had significantly higher evenness than the no-ABX group. Similarly, alpha diversity among the wound bed samples also did not differ significantly for any of the three metrics. Likewise, samples did not significantly (PERMANOVA, P ≤ 0.05) cluster within any time point based on ABX administration for both buccal swab and wound bed samples.
LEfSe plot comparisons of wound bed culture swabs at take down revealed 29 enriched taxa in the ABX group compared to the no-ABX group [P ≤ 0.05, log(LDA) ≥ 2]. Some ABX-enriched taxa include Acinetobacter, Micrococcales, Intrasporangiaceae, and Meiothermus [Figure 2]. Furthermore, the entire domain of bacteria was enriched at follow-up in the wound bed ABX patients, whereas only 12 taxa were enriched in the no-ABX patients at this time point [Figure 3A]. Enriched no-ABX taxa included Haemophilus, Veillonella, Pasteurellales, Methylobacterium, and Oscillospirales. Besides the wound bed swab follow-up LEfSe comparison, the only other comparison with more taxa enriched in the no-ABX group was the buccal swab intraoperative comparison, which only had one taxa (Streptococcus anginosus) enriched. As seen in Figure 3B, buccal swab samples revealed more than 10 enriched taxa at follow-up in the ABX group [P ≤ 0.05, log(LDA) ≥ 2], including common human commensals such as Corynebacteriaceae and Lactobacillaceae.
Figure 2. LEfSe enrichment plots display significantly enriched [P ≤ 0.05, log(LDA) ≥ 2] taxa within the groups. LDA scores are displayed on the X-axis and quantify the strength of enrichment within each respective categorical group, in this case, wound bed swab dressing takedown and wound bed swab dressing takedown antibiotics groups. Two significantly enriched taxa were detected in the no-ABX group, and twenty-nine were detected in the ABX group. ABX: Antibiotics; no-ABX: no antibiotics.
Figure 3. (A) In the wound bed at follow-up, the entire Bacteria domain was identified as being enriched in the ABX group, while the no-ABX group had twelve enriched taxa [P ≤ 0.05, log(LDA) ≥ 2]; (B) In the buccal swabs at follow-up, three taxa were enriched in the no-ABX group, and eleven were enriched in the ABX group [P ≤ 0.05, log(LDA) ≥ 2]. ABX: Antibiotics; no-ABX: no antibiotics.
Assessing reepithelization
The grafted wounds healed throughout the treatment period and follow-up, with minimal graft loss and no postoperative infections. Representative images of ABX and no-ABX autografted wounds at all four time points can be seen in Figure 4. At follow-up, there are no significant differences between ABX and no-ABX in percent reepithelization in autografted and xenografted wounds. Nonparametric t-tests revealed that the variance in the follow-up visit day and the subsequent graft loss grading did not impact the differences in reepithelization between the autograft (P = 0.38) or xenograft (P = 0.19) groups. Autografted ABX wounds were 89.2% re-epithelized at follow-up, whereas no-ABX wounds were 87.1% (SEM = 9.8; P = 0.84). In xenografted wounds, ABX wounds were 71.9% re-epithelized and no-ABX wounds were 81.2% re-epithelized (SEM = 12.8; P = 0.49) [Figure 5].
Figure 4. Digital images of ABX (top) and no-ABX (bottom) wounds from patients who received autografts at all four time points. ABX: Antibiotics; no-ABX: no antibiotics.
Figure 5. Percent reepithelization in autografted and xenografted patients. Autografted patients showed higher reepithelization at follow-up compared to xenografted patients. There was no significant difference in reepithelization between ABX and no ABX patients in either xenografted or autografted wounds (P > 0.05). ABX: Antibiotics; no-ABX: no antibiotics.
DISCUSSION
The pathophysiologic outcome of burn injury leads to coagulative necrosis of burned tissue, immune deficiency, hyperinflammation, and vascular leakage. This unique environment facilitates colonization and subsequent infection of the wound bed. Early wound excision, grafting, nutrition maintenance, and resuscitation are clinical interventions that are considered to stem infection and speed wound closure. The use and classification of antibiotics often increase in patients with greater TBSA burn injuries where the risk of blood-borne infection and sepsis is higher[11,15]. Antibiotic efficacy must be weighed against the risk of altering the resident microbiome and developing MDRO[1,15,23,41,42]. Even small doses of antibiotics risk perturbation of the microbiome and selection for resistant organisms that can become problematic later in a patient’s hospital course. This study provided a novel setting to examine the effect of the administration of a short course of preoperative antibiotics in a controlled setting. Serial examination of the microbiome following a single dose preoperative antibiotic administration is an innovative approach to assessing microorganism changes over time. While clinical outcomes were not significantly different between groups, samples collected from burn patients demonstrated a significantly altered microbiome following the administration of perioperative antibiotics.
Clinical outcomes
Preoperative antibiotics are commonly used in the world of surgery, and multiple clinical practice guidelines have been produced based on previous literature and guidelines put forth by various medical organizations to reduce the occurrence of surgical site infections. These guidelines are often developed from data produced from abdominal-based surgeries but have been adapted to a wide variety of surgical procedures among various surgical subspecialties. Burn injuries are inherently different from other trauma, surgically created wounds, and chronic wounds due to the nature of the injury, mechanism of burn wound infection, and management of associated burn wound sepsis. Furthermore, this patient population presents in an immunocompromised state due to the severe inflammatory response following burn injuries and the loss of skin integrity, making them especially susceptible to opportunistic pathogens and organisms. The serial grafting procedures that often hallmark burn care mean some degree of open wound burden, and thus, the opportunity for invasive organisms to flourish remains high. As a result, antibiotic use in this population is rigorously regimented to avoid the development of resistant bacterial species[12]. Despite this, preoperative antibiotic use in burn injury remains debated, often in the context of the classic teaching of preoperative antibiotic use, as previously mentioned. Antibiotic administration, even as a single dose prior to surgery, risks the development of resistant bacterial species in burn-injured patients.
Cefazolin is a cephalosporin commonly used as a prophylactic antibiotic in surgical interventions to prevent surgical site infection. A single high-concentration dose administered intravenously is above the minimum inhibitory concentrations (MIC) of most commensal pathogens that are the cause of initial infection, such as Staphylococcus aureus, Staphylococcus epidermis, and Escherichia coli, making the perioperative dose advantageous compared to a continuous infusion[43]. In this study, antibiotics did not affect LOS, reepithelization, or graft loss. The LOS of ABX patients and no-ABX patients was similar, with a median of 6 days (IQR: 4-7). All wounds were mostly epithelized at follow-up, which occurred at an average of 14 days post grafting (IQR: 8-27). This finding is corroborated by the literature. For example, Hill et al. found that antibiotic administration in less severe burns (< 20% TBSA) was unneeded antimicrobial exposure and did not change the success rate as measured by graft loss, bacteremia, or surgical site infection[44]. However, this study did not examine changes in biodiversity or wound microbiome following preoperative antibiotic administration, making our assessment unique in this context.
Superfluous antibiotic administration should not be disregarded as there is an abundance of literature highlighting the development of MDRO through inappropriate antibiotic prophylaxis[2,9,11,22,43,45]. Not only has the increasing incidence of MDRO been reported in burn centers, but the effects of antibiotics on the resident microbiome due to antibiotic misuse are also becoming more prevalent[41]. Putra et al. discovered in a retrospective analysis that the use of prophylactic antibiotics such as cefazolin, ceftazidime, and ceftriaxone during burn wound debridement led to an increase in MDROs[43]. Moreover, Timmons et al. argued that systemic antibiotic administration is ineffective at discretely targeting avascular burn eschar where organisms such as S. aureus and P. aeruginosa are favored to grow[46]. This work examining the microbiome of groups following antibiotic administration shows that even short-course single antibiotic administration can have a major effect on bacterial taxonomy.
Preoperative antibiotics and burn wound bed microbiome
Commensal microbes can protect the wound bed from infection and aid in healing. Common commensals such as Propionibacterium and Cutibacterium were enriched in the wound bed swabs of ABX patients intraoperatively (results not shown). It has been shown that Propionibacterium provides protective effects against Pseudomonas infection, with lower abundances of Propionibacterium leading to an increase in Pseudomonas infection[1,47,48]. However, in the wound beds at dressing takedown in the ABX group, rather than commensal microbes, the bacteria most significantly enriched were predominantly extremophiles such as Anoxybaccillus, Effusibacillus, Meiothermus, and Thermoflavifilum. Plichta et al. describe a similar manifestation of thermophiles in the wound post burn injury. They hypothesized that cutaneous ionic and osmolarity imbalances create an environment in the burn eschar that is more favorable to these types of organisms where normal skin is prohibitive[1]. Another hypothesis for extremophile colonization of the wound bed is exposure from the environment. The hospital exposome could serve as the partial source of invasion through contact with water during debridement or other sources[1,42]. There is a paucity of literature examining antibiotic resistant patterns in extremophiles in general, including the use of cefazolin; however, some mechanisms have been suggested. Similar to other resistant bacterial species, extremophiles may accumulate genes or plasmids for multiple drug resistance. This may lead to the development of enzymes like penicillinase that enable propagation in spite of antimicrobial use[49]. Another enriched organism of the wound bacteriome at this time point was Acinetobacter. Acinetobacter is prevalent in burn wound infections and multi-drug resistant Acinetobacter has notably been associated with delayed healing sepsis, shock, and death[4]. The only member enriched in the no-ABX wound bed at takedown was of the phylum Verrucomicrobia, which is commonly found in the environment and is relatively inactive.
Despite the enrichment of extremophiles and pathogenic species as well as enrichment of the whole domain of Bacteria at follow-up in the wound bed, alpha diversity of the wound bed was not significantly different at any time point. Instead, wound bed diversity was slightly elevated in ABX patients. This is contrary to several studies that point to a resulting dysbiosis and lower community richness due to burn injury dynamics alone and after antibiotic treatment[1,5,42,50]. By decreasing the number of commensal microbes, antibiotics may allow for the invasion of species that are more niche-specific, which may not otherwise have an opportunity. The increasing enrichment of non-commensal microbes throughout the time course (from dressing removal through clinical follow-up) leads to the question of when the microbial transition from burn wound dysbiosis to normal healed wound homeostasis occurs and what role clinical therapeutic agents play in that transition.
Preoperative antibiotics and the oral microbiome
The oral microbiome at follow-up in ABX patients displayed enrichment of endogenous flora such as Corynebacterium, Lactobacillus, Rothia, and Granulicatella. Corynebacterium has been associated with a decrease in sepsis in burn patients and a decrease in S. aureus virulence[1,51]. Delanghe et al. found that Lactobacilli play a profound role in inhibiting skin pathogens by modulating the inflammatory response, producing antimicrobial metabolites, and enhancing skin barrier function[52]. Rothia is a known commensal of the oral microbiome. Though it can be pathogenic, its virulence as an opportunistic pathogen is mainly a concern for immunocompromised individuals.
In the no-ABX oral microbiome at follow-up, Defluviitaleaceae of the phylum Firmicutes were enriched. This finding is similar to Tsuzukibashi et al.’s rat study of non-antibiotic cutaneous post-burn bacteriome, in which Firmicutes accounted for 63.8% of the enriched microbial members[53]. The abundance of commensal flora in the oral microbiome at follow-up, in contrast to the entirety of the domain of Bacteria in the wound bed, suggests the integrity and continuity of the oral microbiome despite ABX treatment. The compositional differences in the post-burn microbiome in the oral cavity and wound bed are striking because commensals are not present where they would be most effective in supporting host immune deficiencies (i.e., the wound bed). Further research could characterize and contrast microbiome differences in sites localized to the burn injury, uninjured distant skin, the oral microbiome, and the gut microbiome to bolster our understanding of the global response to post-burn perioperative antibiotic treatment.
Study limitations
This work represents a preliminary investigation into the effect of a short course of antibiotics on the burn patient microbiome. This study has several limitations. While the total sample size of the study is large, the number of patients included is small. Given the translational nature of the study, the need for microbiome analysis, and sample collection techniques, the number of participants we could enroll was limited. Having an adequately powered study is critical to draw more robust and definitive conclusions about the full relationship between preoperative antibiotics, the microbiome, and infection. Additionally, these findings are specific to burn-injured patients and are not generalizable to other specific or general patient populations. Furthermore, there are many methods of microorganism identification, such as biopsies for the detection of deep-colonized species. Unfortunately, biopsies are unable to be obtained due to the preliminary nature of the study and, as such, represent a limitation of the study. Broader and combined antibiotic regimens are also used in burn-injured patients and are not represented in this microbiome analysis. All patients were assumed to receive center-specific standard burn care treatment and differences in clinical care were not accounted for if they occurred. Longitudinal characterization of clinical outcomes, taken months to years after initial presentation, could have been important to clinical outcome reporting and could have also revealed changes or reestablishment of resident flora.
This study demonstrates that a perioperative dose of antibiotics is impactful to the host microbiome in smaller burns. Responsible administration of antibiotic prophylaxis is important to mitigate the increasing prevalence of MDRO. Understanding the differing abundant taxa in the oral and wound microbiomes may inform systemic antibiotics’ impact on the host. Infiltration of extremophiles and pathogens such as Acinetobacter into the oral and wound microbiome suggests augmenting antimicrobial usage to specific pathogens and only when needed. Further research involving additional bacterial sequencing or probiotic pre-treatment to reintroduce helpful commensal microorganisms may help to understand the mechanisms of the wound microbiome.
DECLARATIONS
Authors’ contributions
Wrote the manuscript: Tejiram S, Keyloun JW, Oliver MA, Chen See JR, Pinto DN
Collected clinical samples, conducted lab assays, and analyzed the data: Keyloun JW, Ball RL
Conducted lab assays and analyzed the data: Oliver MA
Analyzed and interpreted the data: Wright J
Contributed to project conception and protocolization: Nosanov L, McLawhorn MM
Contributed to the analysis and interpretation of data: Lamendella R
Contributed to the editing and revision of the manuscript: Tejiram S, Keyloun JW, Carney BC, Travis TE, Moffatt LT, Shupp JW
Conceived the project, contributed to the analysis and interpretation of data, and edited and revised the manuscript: Shupp JW
Availability of data and materials
The authors confirm that the data supporting the findings of this study are available within the article or from the corresponding author upon reasonable request.
Financial support and sponsorship
None.
Conflicts of interest
All authors declared that there are no conflicts of interest.
Ethical approval and consent to participate
The protocol was approved by Medstar Health Research Institute’s Institutional Review Board (Protocol #2017-186). Informed consent was obtained before study participation.
Consent for publication
The consent for publication was obtained.
Copyright
© The Author(s) 2025.
REFERENCES
1. Plichta JK, Gao X, Lin H, et al. Cutaneous burn injury promotes shifts in the bacterial microbiome in autologous donor skin: implications for skin grafting outcomes. Shock. 2017;48:441-8.
2. Branski LK, Al-Mousawi A, Rivero H, Jeschke MG, Sanford AP, Herndon DN. Emerging infections in burns. Surg Infect. 2009;10:389-97.
3. Jeschke MG, van Baar ME, Choudhry MA, Chung KK, Gibran NS, Logsetty S. Burn injury. Nat Rev Dis Primers. 2020;6:11.
4. Church D, Elsayed S, Reid O, Winston B, Lindsay R. Burn wound infections. Clin Microbiol Rev. 2006;19:403-34.
5. Zhang P, Zou B, Liou YC, Huang C. The pathogenesis and diagnosis of sepsis post burn injury. Burns Trauma. 2021;9:tkaa047.
6. Nielson CB, Duethman NC, Howard JM, Moncure M, Wood JG. Burns: pathophysiology of systemic complications and current management. J Burn Care Res. 2017;38:e469-81.
7. Hettiaratchy S, Dziewulski P. ABC of burns: pathophysiology and types of burns. BMJ. 2004;328:1427-9.
8. Chen YY, Wu PF, Chen CS, Chen IH, Huang WT, Wang FD. Trends in microbial profile of burn patients following an event of dust explosion at a tertiary medical center. BMC Infect Dis. 2020;20:193.
9. van Duin D, Strassle PD, DiBiase LM, et al. Timeline of health care-associated infections and pathogens after burn injuries. Am J Infect Control. 2016;44:1511-6.
10. Barret JP, Herndon DN. Effects of burn wound excision on bacterial colonization and invasion. Plast Reconstr Surg. 2003;111:744-50.
11. Yeong EK, Sheng WH, Hsueh PR, et al. The wound microbiology and the outcomes of the systemic antibiotic prophylaxis in a mass burn casualty incident. J Burn Care Res. 2020;41:95-103.
13. Thabet L, Turki A, Ben Redjeb S, Messadi Aa. [Bacteriological profile and antibiotic resistance of bacteria isolates in a burn department]. Tunis Med. 2008;86:1051-4.
14. Alexander JW, MacMillan BG, Law EJ, Krummel R. Prophylactic antibiotics as an adjunct for skin grafting in clean reconstructive surgery following burn injury. J Trauma. 1982;22:687-90.
15. Ramos G, Resta M, Machare Delgado E, Durlach R, Fernandez Canigia L, Benaim F. Systemic perioperative antibiotic prophylaxis may improve skin autograft survival in patients with acute burns. J Burn Care Res. 2008;29:917-23.
16. Griswold JA, Grube BJ, Engrav LH, Marvin JA, Heimbach DM. Determinants of donor site infections in small burn grafts. J Burn Care Rehabil. 1989;10:531-5.
17. Ramos G, Cornistein W, Cerino GT, Nacif G. Systemic antimicrobial prophylaxis in burn patients: systematic review. J Hosp Infect. 2017;97:105-14.
18. Yang Y, Huang J, Zeng A, Long X, Yu N, Wang X. The role of the skin microbiome in wound healing. Burns Trauma. 2024;12:tkad059.
19. Jung Y, Park C, Lee H, et al. Association of the skin microbiome with the biomechanical scar properties in patients with burns. Burns. 2025;51:107372.
20. Barajas-Nava LA, López-Alcalde J, Roqué i Figuls M, Solà I, Bonfill Cosp X. Antibiotic prophylaxis for preventing burn wound infection. Cochrane Database Syst Rev. 2013;2013:CD008738.
21. Fitzwater J, Purdue GF, Hunt JL, O’Keefe GE. The risk factors and time course of sepsis and organ dysfunction after burn trauma. J Trauma. 2003;54:959-66.
22. Avni T, Levcovich A, Ad-El DD, Leibovici L, Paul M. Prophylactic antibiotics for burns patients: systematic review and meta-analysis. BMJ. 2010;340:c241.
23. Ugburo AO, Atoyebi OA, Oyeneyin JO, Sowemimo GO. An evaluation of the role of systemic antibiotic prophylaxis in the control of burn wound infection at the Lagos university teaching hospital. Burns. 2004;30:43-8.
24. Csenkey A, Jozsa G, Gede N, et al. Systemic antibiotic prophylaxis does not affect infectious complications in pediatric burn injury: a meta-analysis. PLoS One. 2019;14:e0223063.
25. Devgan L, Bhat S, Aylward S, Spence RJ. Modalities for the assessment of burn wound depth. J Burns Wounds. 2006;5:e2.
26. Alqattan H, Edris S, A.Abulfaraj A, et al. Microbiome signature and diversity profiling of normal skin of human in Saudi Arabia. Jundishapur J Microbiol. 2021;14:e113355.
27. Walters W, Hyde ER, Berg-Lyons D, et al. Improved bacterial 16S rRNA gene (V4 and V4-5) and fungal internal transcribed spacer marker gene primers for microbial community surveys. mSystems. 2015;1:e00009-15.
28. Bolyen E, Rideout JR, Dillon MR, et al. Reproducible, interactive, scalable and extensible microbiome data science using QIIME 2. Nat Biotechnol. 2019;37:852-7.
29. Rognes T, Flouri T, Nichols B, Quince C, Mahé F. VSEARCH: a versatile open source tool for metagenomics. PeerJ. 2016;4:e2584.
30. Quast C, Pruesse E, Yilmaz P, et al. The SILVA ribosomal RNA gene database project: improved data processing and web-based tools. Nucleic Acids Res. 2013;41:D590-6.
31. Price MN, Dehal PS, Arkin AP. FastTree 2--approximately maximum-likelihood trees for large alignments. PLoS One. 2010;5:e9490.
32. Katoh K, Standley DM. MAFFT multiple sequence alignment software version 7: improvements in performance and usability. Mol Biol Evol. 2013;30:772-80.
33. Davis NM, Proctor DM, Holmes SP, Relman DA, Callahan BJ. Simple statistical identification and removal of contaminant sequences in marker-gene and metagenomics data. Microbiome. 2018;6:226.
34. Team RC. R: a language and environment for statistical computing. Available from: https://cran.r-project.org/doc/manuals/r-release/fullrefman.pdf. [Last accessed on 8 Apr 2025].
35. Faith DP, Baker AM. Phylogenetic diversity (PD) and biodiversity conservation: some bioinformatics challenges. Evol Bioinform Online. 2007;2:121-8.
36. Pielou E. The measurement of diversity in different types of biological collections. J Theor Biol. 1966;13:131-44.
37. Paulson JN, Stine OC, Bravo HC, Pop M. Differential abundance analysis for microbial marker-gene surveys. Nat Methods. 2013;10:1200-2.
38. Lozupone CA, Hamady M, Kelley ST, Knight R. Quantitative and qualitative beta diversity measures lead to different insights into factors that structure microbial communities. Appl Environ Microbiol. 2007;73:1576-85.
39. Segata N, Izard J, Waldron L, et al. Metagenomic biomarker discovery and explanation. Genome Biol. 2011;12:R60.
40. Nosanov LB, McLawhorn MM, Hassan L, et al. Graft loss: review of a single burn center’s experience and proposal of a graft loss grading scale. J Surg Res. 2017;216:185-90.
41. Corcione S, Lupia T, De Rosa FG; Host and Microbiota Interaction Study Group (ESGHAMI) of the European Society of Clinical Microbiology and Infectious Diseases (ESCMID). Microbiome in the setting of burn patients: implications for infections and clinical outcomes. Burns Trauma. 2020;8:tkaa033.
42. Sanjar F, Weaver AJ, Peacock TJ, Nguyen JQ, Brandenburg KS, Leung KP. Identification of metagenomics structure and function associated with temporal changes in rat (rattus norvegicus) skin microbiome during health and cutaneous burn. J Burn Care Res. 2020;41:347-58.
43. Putra ON, Saputro ID, Hidayatullah AYN. A retrospective surveillance of the prophylactic antibiotics for debridement surgery in burn patients. Int J Burns Trauma. 2021;11:96-104.
44. Hill DM, Guido A, Sultan-Ali I, Arif F, Velamuri SR. A non-inferiority study comparing efficacy of preoperative prophylactic antibiotics for preventing infectious complications in patients with less severe burns. Burns. 2021;47:67-71.
45. van Langeveld I, Gagnon RC, Conrad PF, et al. Multiple-drug resistance in burn patients: a retrospective study on the impact of antibiotic resistance on survival and length of stay. J Burn Care Res. 2017;38:99-105.
46. Timmons MJ. Are systemic prophylactic antibiotics necessary for burns? Ann R Coll Surg Engl. 1983;65:80-2.
47. Lai Y, Cogen AL, Radek KA, et al. Activation of TLR2 by a small molecule produced by Staphylococcus epidermidis increases antimicrobial defense against bacterial skin infections. J Invest Dermatol. 2010;130:2211-21.
48. Hruz P, Zinkernagel AS, Jenikova G, et al. NOD2 contributes to cutaneous defense against Staphylococcus aureus through alpha-toxin-dependent innate immune activation. Proc Natl Acad Sci U S A. 2009;106:12873-8.
49. Gabani P, Prakash D, Singh OV. Emergence of antibiotic-resistant extremophiles (AREs). Extremophiles. 2012;16:697-713.
50. Liu SH, Huang YC, Chen LY, Yu SC, Yu HY, Chuang SS. The skin microbiome of wound scars and unaffected skin in patients with moderate to severe burns in the subacute phase. Wound Repair Regen. 2018;26:182-91.
51. Ramsey MM, Freire MO, Gabrilska RA, Rumbaugh KP, Lemon KP. Staphylococcus aureus shifts toward commensalism in response to corynebacterium species. Front Microbiol. 2016;7:1230.
52. Delanghe L, Spacova I, Van Malderen J, Oerlemans E, Claes I, Lebeer S. The role of lactobacilli in inhibiting skin pathogens. Biochem Soc Trans. 2021;49:617-27.
Cite This Article

How to Cite
Download Citation
Export Citation File:
Type of Import
Tips on Downloading Citation
Citation Manager File Format
Type of Import
Direct Import: When the Direct Import option is selected (the default state), a dialogue box will give you the option to Save or Open the downloaded citation data. Choosing Open will either launch your citation manager or give you a choice of applications with which to use the metadata. The Save option saves the file locally for later use.
Indirect Import: When the Indirect Import option is selected, the metadata is displayed and may be copied and pasted as needed.
About This Article
Special Issue
Copyright
Data & Comments
Data
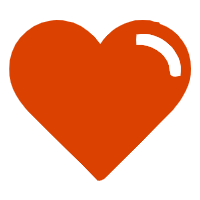
Comments
Comments must be written in English. Spam, offensive content, impersonation, and private information will not be permitted. If any comment is reported and identified as inappropriate content by OAE staff, the comment will be removed without notice. If you have any queries or need any help, please contact us at [email protected].