How plastic waste management affects the accumulation of microplastics in waters: a review for transport mechanisms and routes of microplastics in aquatic environments and a timeline for their fate and occurrence (past, present, and future)
Abstract
An increasing global problem is the buildup of improperly handled plastic garbage in the environment. One of the biggest environmental issues facing aquatic ecosystems today is contamination from bulk plastics and plastic detritus. Specifically, microplastics (MPs) and nanoplastics, which are small-scale plastic waste, are now the main causes of pollution in freshwater and marine environments. On the other hand, contamination of aquatic systems is now acknowledged as one of the major environmental hazards facing our world. Currently, concerns have been raised regarding the breakdown of plastic products into micro and nanosized particles, because of the ineffective plastic waste management. To prioritize regions for mitigation policy implementation, it is critical to pinpoint the precise MPs’ transport mechanisms and the locations where trash is created. In order to show the historical and contemporary circumstances as well as forecasts and scenarios of global plastic waste management from now until 2060, we used continent-level data on trash management. This study, finally, presents a potential future scenario of estimates on the destiny, transport, and occurrence of plastic waste in aquatic habitats, highlighting the different factors that trigger the transport of MPs into water and the necessity of rational management of plastic waste.
Keywords
INTRODUCTION
Plastics are currently a great material that is employed in many aspects of daily life, as they are utilized in nearly every industry, including packaging, vehicle, aquaculture, fisheries, biomedical, shipping, agriculture, building and construction, telecommunications, furniture, transportation, textiles, apparel, and personal care items. Because of their low weight, elasticity, resilience, flexibility, low cost, long-term persistence, corrosion resistance, high strength-to-weight ratio, and water-resistant qualities, plastics have supplanted more traditional materials such as glass and metals[1-3].
The number of anthropogenic stressors in aquatic media, including microplastics (MPs), is making a significant contribution to the increasing pollution of the environment[4]. Because plastic goods are used so widely in daily life, an increasing number of plastic particles - primary MPs - are making their way into the water. The global danger that MP pollution poses to human and ecological health is causing the public to grow more concerned about this issue[5,6]. According to the National Oceanic and Atmospheric Administration (NOAA) of the United States, fragments of any kind of plastic that are smaller than 5.0 mm can generally be categorized as MPs[7,8]. It is usual to use the mesh size of the neuston nets (333 μm or
MPs are created when plastics undergo physical, chemical, mechanical, and biological degradation or break down into tiny fractions[9]. Due to their ongoing manufacture, non-biodegradable nature, persistence, and extended environmental life span, these plastics are tiny, ubiquitous particles that have been steadily accumulating in the environment[10]. According to Constant et al. (2020), they have been identified as one of the ten emerging pollutants in the United Nations Environmental Programme (UNEP) Year Book 2014 that may pose a hazard to the health of humans and other creatures across all biomes[11].
Scientists worldwide are increasingly concerned about the buildup of MPs in environmental components, with densely inhabited areas showing higher concentrations in lakes, rivers, estuaries, seas, and beaches[12,13]. Due to their tiny size, MPs can potentially affect human health when they enter the human food chain through the consumption of seafood and other terrestrial foods[14-17]. Furthermore, the disposal of plastic trash in municipal waste disposal systems generates toxic leachate, which can damage land and water[18]. Plastic trash will keep rising as a result of inappropriate waste management practices and unprecedented consumption of plastic items[13,19].
Global efforts are focusing on quantifying plastic pollution in seas and freshwater, as it affects marine life and people. The breakdown of macroplastics into MPs complicates identifying pollution sources and implementing mitigation strategies. Studying MP life cycles without estuaries undervalues their contribution to oceans[20]. Over the past 10 years, the relevance of MP debris in freshwater and marine ecosystems has increased[21,22]. The impact of MPs on aquatic organisms is underexplored, with plastic fragmentation primarily induced by weathering, similar to sunlight photodegradation. In marine environments, plastics have a slower rate of degradation due to lower temperatures[21,23,24]. Mechanical elements like turbulence and wave action can fragment plastic debris, while improper disposal can cause secondary MP buildup in marine environments due to non-biodegradable synthetic polymers. Furthermore, the anerobic conditions required for the breakdown of biodegradable polymers are absent from saltwater[4,25,26]. Understanding the mechanisms affecting marine plastic trash dispersion and transit is crucial due to the heterogeneity of plastic particles and their non-linear behavior throughout the water column[27,28]. Variations in density, buoyancy, and residence durations that occur during the MP particle’s life cycle in the water are linked to discrepancies in their behavior[28].
The current focus of the study is to gain a better understanding of this behavior, as knowledge of these processes is essential for assessing ecological implications and potential health hazards to humans. Additionally, it helps develop policies and standards for managing coastal areas and put in place appropriate monitoring systems[29]. According to Hardesty et al. (2017), numerical models hold great potential for improving our understanding of plastics’ behavior in dynamic flow systems. Numerous intricacies linked to flow dynamics can be calculated using numerical models. Furthermore, developments in numerical methods and processing power have made it possible to apply fine grid resolution for the computation of precise and detailed flow patterns, which serve as MP drivers[28,30].
Thus, the goals of this work are to: (a) Review the manner in which fresh water is contaminated by various transport mechanisms; (b) summarize the primary transport mechanisms in the estuaries of some of the heavily polluted rivers or lakes that leak MPs into the seas, (c) to present MPs’ routes of transportation via several environmental divisions; (d) to correlate plastic waste management and MPs’ occurrence in water; and (e) to analyze the forecasts for plastics and MPs in water by 2060, taking into account the improper handling of plastic trash in the present and the upcoming years.
METHODOLOGY
This mini-review compiles findings from 100 studies about MP transport processes in rivers, lakes, and oceans. The terms “MPs” and “estuary” were combined with the following keywords to extract literature from the databases of Google Scholar, Scopus, and Web of Science: “transport”, “fate”, “plastic waste”, “nanoplastics”, “litter”, “migration behavior”, and “contamination”. The retrieved studies were categorized according to the transport mechanisms they covered. Each article located was released between 2010 and 2023. Research addressing the movement of MPs in estuary sediments and water columns was incorporated, whereas studies examining the effects of MPs on estuarine biota were not. Moreover, research conducted in aquatic environments - such as lakes, rivers, and oceans - was also included.
We stopped our search when multiple pages of results in Scopus, Google Scholar, and Web of Science yielded no relevant papers. Since the search was focused on finding publications with literature reviews, case studies, book chapters, conference papers, postgraduate and doctoral theses, and papers written in languages other than English were all excluded. As a result, published articles were filtered using the previously indicated criteria (title, keywords, and abstract), and relevant publications were included in our collection. We reviewed the content of each article, paying particular attention to the abstracts and conclusions. The ideal reference criteria for systematic reviews and meta-analyses set by Moher et al. were used in this review paper’s research approach [Figure 1][31].
Figure 1. Comprehensive workflow of the research project[31].
The datasets used in this paper have been taken by OECD Library Global Plastics Outlook as Excel files, and they provide estimates of plastic waste for the different global regions and/or continents. These datasets also provide estimates of plastic waste measured in millions of tons per year, per end-of-life fate after accounting for recycling losses and collected littering, etc.[32].
TRANSPORT MECHANISMS OF MPS IN FRESHWATER, RIVERS, LAKES AND MARINE ENVIRONMENTS
MPs’ occurrence and destiny are influenced by physical properties, environmental interactions, and meteorological and hydrodynamic factors. Factors like size, density, and morphology also influence their dispersion and sinking rates[33]. MPs, due to their size, shape, and durability, are easily transported by wind and water, influenced by human behavior, particle characteristics, weather, and environmental topography[34].
They can enter aquatic habitats through various routes, including runoff, garbage dumping, air deposition, and wastewater treatment plant outfall, as mentioned before. Although treatment plants can retain MPs and filter out some particles, they struggle with synthetic microfibers and nanoplastics[35,36]. Other MP entry mechanisms into terrestrial and aquatic environments relate to agricultural sources, including organic fertilizer products[37].The physical and chemical characteristics of MPs, substrate conditions, and hydrodynamics at the sediment-water interface affect their transit in lakes and rivers, with different origins affecting transport qualities, burial likelihood, and interactions[36,38].
Plastics and MPs can enter the environment through various channels, including terrestrial and marine-based activities, as well as home or commercial drainage systems. For instance, tiny plastic beads commonly found in toothpaste, scrubs, and clothes can easily find their way into the aquatic ecosystem. Similar to this, clothing made of synthetic fibers sheds MPs, which end up as effluents in wastewater treatment facilities or water supplies[39]. Additional pathways for MPs to infiltrate the marine environment include storm drains, wind, and waves. Runoff carries some of them out to sea. Unfavorable weather conditions increase the deposit of macroplastic at shorelines, and the path often passes via landfills and marine recycling ports, which contribute to the breakdown of macroplastic garbage. Sewage sludge, which enters the aquatic ecosystem containing more MPs than garbage, is another possible cause of MP pollution[40,41]. The low concentrations and huge size of MPs allow currents to carry them over greater distances and distribute them widely[42].
Additionally, according to De Witte et al. (2014) and Gauquie et al. (2015), MPs may be found in a variety of marine animals, including fish, seabirds, bivalves, mammals, and crabs, as well as on beaches and in seabed sediments and surface waters[43,44]. The excrement of zooplankton is another way that MPs might enter the ocean. The MPs were easily consumed by the organisms as they passed through their digestive tracts, were encapsulated in excrement, and were ingested. After being eaten, the excrement dropped to the bottom of the exposure vessel, where it was later consumed by the bigger copepod[45].
Municipal wastewater treatment plants are significant sources of MPs in the environment, primarily removed through sludge settling and solids skimming. The effluent discharges from these plants can influence MP burdens in oceans and surface water habitats[46]. For instance, MP parts from synthetic fibers in garments end up as effluents in wastewater treatment facilities or water, and each day, about 65 million MP particles are released into the receiving water by Glasgow’s wastewater treatment works (WWTW), which is situated on the River Clyde[39,45]. Thus, wastewater treatment plant (WWTP) effluent has been highlighted in a number of published studies as a major pathway for the direct transfer of MPs into aquatic and soil habitats[47]. As we said before, in the first stages of the WWT process, large-size MPs and other particles may be held in the grit chamber and mechanical screen. The coagulation/flocculation mechanism causes MP fibers to settle by gravity during the initial treatment phase[13].
The human respiratory system is harmed by MPs of various morphologies that are constantly being carried and settling in soil or sediment after being discharged into the atmosphere. Beaches, seabed sediments, surface waters, and a variety of marine organisms, such as fish, seabirds, bivalves, mammals, and crabs, among others, have been reported to contain MPs[45,48]. MPs can travel by atmospheric fall and marine movement. Its low density and tiny size contribute significantly to the widespread dissemination of MPs in all ecosystems worldwide. According to Obbard et al. (2014), polar ice and marine sediments have therefore turned into a worldwide sink for MPs[49].
As to terrestrial system concerns, according to He et al. (2018), agricultural operations, precipitation, fractures, and soil organism activities can all introduce MPs into the subsurface soil[50]. These mechanisms have the potential to induce MPs to seep into deep soils containing water and eventually find their way into groundwater[51]. Furthermore, through ingestion, redox, and excretion processes, soil organisms, such as earthworms, insects, nematodes, bacteria, fungi, algae, etc., affect the transport and destiny of MPs[13,52]. MP fragments from wastewater treatment facilities are often retained in sludge due to high efficiency, and large volumes are dumped into Europe for composting and agricultural fertilizer[34]. MPs are not yet taken into account by regulations on hazardous substances found in sludge applied to land, so the mass of MPs unintentionally applied to land each year may surpass 400,000 tons[53]. Sewage treatment effluent contains MPs that can enter the environment through rivers’ effluent intake. These particles are primarily applied to land as sludge or discharged directly into the environment. Other methods for disposing of sludge include burning, landfilling, and cement production, making it unlikely for plastic particles to leak[34,51,54]. Other techniques for getting rid of sludge include burning it, dumping it in a landfill, and even using it to make cement that is used in buildings. Under these circumstances, it is improbable that plastic particles would leak into the environment because they are well-contained[51,54,55]. MPs in wastewater treatment plant sewage sludge can be as high as 15,385 items/kg, contaminating agricultural soils. Annual discharges in North America and Europe are estimated to be 63,000-430,000 tons and 440,00-300,000 tons, respectively[53,56]. Organic fertilizers made from composting and fermenting biowaste can introduce MPs into soils, which are often applied to agricultural crops after drying, and are transported through physical, chemical, and biological processes[37]. Plastic film mulching and greenhouse covering have been widely and heavily used in agricultural production because of their efficaciousness in enhancing agricultural quality and production and can be one extra factor for the appearance of MPs in soils[57,58]. The increased concentration of MPs in terrestrial soils may also be caused by other factors such as roadside littering, illicit trash disposal, and tire abrasion[52].
The air-water transfer of materials is influenced by aquatic conditions, including salinity and organic colloids. Bubble bursting, a phenomenon where plastic particles transfer from water to air, is influenced by salinity and gel concentration. In high salinity settings, gel concentration and viscosity significantly affect MP mobility. The addition of proxies for sea surface microlayer components increases the particle transfer rate[59].
The air-water transfer is also reinforced by rivers that are crucial entry points for MPs into the ocean, but the lack of data on their environmental fate makes it difficult to assess danger and design regulatory responses. MP prevalence increases upstream and downstream, correlated with natural and man-made factors. The density and size of MPs influence migration across compartments, with geographic distance being a strong determinant[60,61]. The prevalence of MPs demonstrated significant associations with the increase and geographic location of sampling sites, the distance from the closest city, demographic density, and the rate of urbanization, according to Pearson’s correlation analysis provided in a study by Yuan et al. 2022[60]. MPs’ circulation and final sink are influenced by environmental factors, density, shape, size, hydrodynamics, meteorological conditions, habitat characteristics, and interactions with aquatic biota[62]. The upper soil level is a degradative environment for plastic trash, aided by strong heat, oxygen, and sunlight. Plastics undergo biodegradation and macroplastic breakdown, accelerated by agricultural practices. However, plastic degradation takes decades. MPs enter groundwater systems through leaching and are transported via the biosphere, with soil biota playing a key role in this process[63].
Improper management of rubbish and wind-blown littering might unintentionally introduce plastics from landfills into the terrestrial ecosystem. MPs can travel through the ecosystem thanks to landfills. The polymers might be dispersed far from their sources by wind action[63]. Alternatively, toxic materials derived from plastic garbage may leak into landfills when plastic waste is disposed of there. If left untreated, the leachate from the breakdown and leaching of additives such as plasticizers might release toxins that contaminate the surrounding rivers and groundwater system[64,65].
The amount of plastic litter that is discharged into the environment is difficult to estimate since there are few data and disposal practices for plastic debris vary between countries. Wind-blown trash and plastic discharged onto the land are examples of mismanaged garbage. According to estimates from 2010, the amount of garbage that is mishandled in the European Union (EU) is comparable to that in the US. As demonstrated by the implementation of EU-wide waste management regulations, this is a realistic assumption based on parallels in national income and the development of waste management infrastructure[66]. As mentioned before, the amount of mismanaged plastic garbage (plastic waste - managed waste) in the EU is estimated at 520,000 metric tons. Furthermore, sewage sludge is thought to deposit between 63,000 and 430,000 metric tons of MPs onto land each year[53].
Larger proportions will eventually find their way into the marine environment as a consequence of increased aggregation brought on by rising plastic manufacture and natural erosion. Nonetheless, it is still probable that for a long while to come, more plastic will be deposited and kept in continental ecosystems than will enter the oceans. According to estimates, rivers serve as conduits for the transportation of 70%-80% of marine plastics into the ocean. In addition to being carried by rivers from the land to the ocean, plastic waste may also come back to the ground during high tides or floods. The same elements that influence the movement of sediment also influence the movement of plastic particles within river systems, including environmental parameters and hydrological features[34,53]. Anything may be deposited in areas with low flows and changes in stream depth or flow (such as on bends); on the other hand, high-velocity rainfall and deterioration dislodge previously settled debris to be mobilized instead of introducing particles through runoff[34].
All of the above has been derived from a thorough study of all the published papers included in this review, which include the most prevalent sources and mechanisms of MP transport in aquatic environments. Below in Figure 2, the primary mechanisms of MP transport are illustrated, arranged and quantified based on statistical analysis, ranking from the most dominant and frequent to the least important. More specifically, the main mechanisms that are referred to in the reviewed published papers have been classified in the categories below. This was followed by a quantification of the frequency of occurrence in the examined published papers.
MPS’ ROUTES OF TRANSPORTATION VIA SEVERAL ENVIRONMENTAL DIVISIONS
Atmospheric environment
Due to the lack of limits in the air and the fact that the speed, as well as the direction of the air, primarily affect the MPs, the moving of MPs in the atmosphere might result in a large dispersal range for MPs. Variables like temperature, precipitation, wind direction, speed, and pollution concentration gradient influence MPs’ movement in the atmosphere, with wind modulation impacting urban transportation and suspension[67,68]. Particle density and sedimentation, wind, and precipitation all affect how long particles remain in the atmosphere and how they fall out or deposit[69]. Low-density MPs are frequently carried by the wind over great distances, which ultimately leads to MP pollution on land and in water[70].
Boucher and Friot (2017) found that wind-borne atmospheric MP transport accounts for 7% of ocean MP contamination. According to air mass trajectories, MPs were carried up to 95 kilometers from their point of origin to a region with a low population density[71]. According to Abbasi et al. (2019) and Prata (2018), atmospheric particulates that have settled in the terrestrial environment may also be resuspended in the air or carried to aquatic systems by rainfall or surface runoff and because MPs and dust share the same pattern of degradation, MPs observed in the marine environment can be linked to atmospheric dust fallout[68,72].
Terrestrial environment
Because MPs are resilient, they will build up and come to high concentration levels in the soil, which may have an impact on the biodiversity of soil[73]. Many variables, including abiotic and biotic transport as well as vertical and horizontal mobility, affect how MPs migrate through the soil. The horizontal and vertical motions of MPs in soil are controlled by many elements, such as the surface of the soil, accumulation, and agricultural operations (such as harvesting)[51]. The changes in MP properties and structure (size, shape, hydrophobicity, and chemical composition) also have key roles in MP transport in soil[74]. The retention mechanisms of MPs alter as the size of particles grows, and their attachment effectiveness rises noticeably[75]. Mobility of MP spherules in soil may be improved by raising the UV hydrophilicity and negative surface charge of MP particles[76]. Organic matter and soil water flow rate affect MP transport, with organic matter increasing transport mechanisms and reducing stability. MPs can be transported through natural and man-made processes, and most are fibers, which are easily lifted into the atmosphere. Soil serves as both a source and sink[77,78].
Aquatic environment
Strong hydrodynamic forces, such as wind, waves, tides, and thermohaline gradients, constantly affect the aquatic environment, making it an active zone. Thus, climatic pressures and coastal transport mechanisms alter the temporal and geographical distributions of MPs[79]. Plastics are carried by beaching, drifting, and settling pathways from terrestrial, riverine, and marine sources to transient reservoirs on beaches, in tidal wetlands, and in marine sediments. When floodwaters release from upstream locations during wet periods, the largest concentrations of MPs are found around river mouth areas. Although there is little knowledge of the physical mechanisms underlying this process, plastics that are carried to aquatic habitats are maintained nearshore in estuaries, wetland sediments, and beaches[77]. MPs are transported further into grounds and ocean sediments by both terrestrial and aquatic biota, primarily via the processes of bioturbation and biofouling. Various aquatic and soil species can actively participate in the transportation of MPs[50]. As mentioned above, MPs’ physical characteristics are influenced by coagulation, photochemical deterioration, mechanical decomposition, and biofouling, affecting particle density, size distribution, geometric form, and surface properties, consequently shaping their ultimate destiny[80,81].
One important factor influencing the buoyancy and movement of MPs in water is particle density. Neutral particles with densities less than seawater float at or close to the sea surface, whereas nonfloating particles with densities greater than seawater often sink to the sea floor[25]. Low-density particles have been discovered in sediments in coastal waters and on the deep ocean floor, suggesting that high-density particles may travel great distances. Because plastics have a high material density and coastal seas are turbulent, it is anticipated that non-buoyant plastic particles will mostly travel by suspended load[82].
Additionally, the size of plastic particles, which plays an instrumental role in the transport of MPs, may be altered by a number of processes, such as flocculation, aggregation, biofouling, and fragmentation. Regardless of their densities, microscopic plastic pieces exhibit the behavior of colloidal particles and typically exist as suspended particles in the water column[80]. As such, the size distribution of plastic particles must be considered a dynamic characteristic that varies with residence duration and transit routes[45]. MPs can become larger and denser by aggregation with both organic and inorganic particles, leading to a quicker deposition of the particles on sediments. Given the large quantities of suspended sediments, particulate organic matter, and detrital particles in the coastal environment, aggregation and subsequent sedimentation could dominate the fate and dispersion of MPs[83].
It has been shown that, like MPs, the Schulze Hardy rule and Derjaguin Landau Verwey Overbeek (DLVO) theory may be used to understand the process behind the behavior of MPs. To put it simply, ionic strength, organic matter, flow velocity, size, and surface charge can all have an impact on MP mobility[83,84]. In relation to their original form (plastic pieces, pellets, fibers, or filaments) and fragmentation processes, marine plastic particles come in a variety of forms[85]. Additionally, the shape and specific surface area of the particles have a significant role in defining the aggregation and biofouling processes that affect the MPs’ transit channel. Particles having high surface area to volume ratios (films, fibers, foams) have a greater rate of aggregation/biofouling and may sink sooner than big things[81,83].
All in all, taking into consideration all the aforementioned, we can conclude that MPs can be carried by atmospheric or aquatic currents depending on their weight and density. MPs may move from the pedosphere into the hydrosphere via surface runoff, rainfall, and ocean circulation. Ocean circulation allows MPs to not only move from land to water, but also from water to land[86,87]. In addition, smaller and lighter MPs have the ability to travel great distances, including high altitudes and glacier zones, as they become airborne and are transported by the wind. Denser MPs may collect in the pedosphere (soil) or be buried there, whereas lighter MPs may be carried by wind over the pedosphere[34]. Surface runoff from cities and agricultural regions, together with heavy rains, can introduce MPs into surface waterways (the hydrosphere). Research has indicated that the utilization of plastic mulches to enhance crop development or the addition of household sewage sludge as a soil amendment in agricultural activities may result in the introduction of MPs into the soil[88]. Furthermore, stormwater runoff transports the MPs that are produced by tires wearing down naturally to nearby surface waterways[34]. Furthermore, airborne MPs made of light fibers from trash incineration, landfills, and clothing can travel great distances and deposit themselves in remote locations by atmospheric fallout[88].
PLASTIC WASTE MANAGEMENT AND MPS
Plastic particles are present in the air, drinking water, sediments, seas, snow ice, and agricultural soils, among other things. MPs are being released into the environment at an accelerated rate worldwide, which has an impact on biodiversity, conservation, and water quality. An understanding of the origins, transit, and destiny of plastics in the environment, as well as multidisciplinary research, and regional and global collaboration, are necessary for managing plastics in the environment and guaranteeing effective intervention policies and practices. The accumulation of plastic particles has been found to vary both temporally and geographically, with differences in dispersal and distribution being attributed to anthropogenic causes, time, places, hydrodynamic conditions, and environmental pressure[89]. In addition, the varied distribution of MPs, particularly in adjacent rivers, has been linked to population density, industrial activity, and hydrological conditions[90].
Municipal solid trash contains a sizable portion of post-consumer plastic garbage. Since the 1950s, when commercial manufacture of plastics began, 8,600 million metric tons of plastic have been produced. Of this garbage, 70 percent has been diverted to landfills and the natural environment[19]. For example, plastic leakage in South Africa results in an annual dumping of approximately 40,000 metric tons of plastic waste into the ocean. Concerns about municipal plastic waste (MPW) have arisen in every country, prompting proposals for global legislation and control. Currently, many other nations have banned or imposed taxes on plastics due to popular outcry and political commitment[90].
The greatest solution for a plastics circular economy has been identified as landfill diversion through recycling and energy recovery. On the other hand, the majority of African countries choose to leave their garbage - plastic waste in particular - in landfills or rubbish dumps, with the rare badly managed landfill. While recyclable and reusable items, including plastics, are scavenged and recycled back into the economy, biodegradable materials use these landfills and dumps as composting beds. Africa has a notably distinct approach to managing plastics for socioeconomic and political reasons. Systematic recycling is quite restricted in Africa; the combined capacity for recycling in the most developed systems - South Africa, Ethiopia, Uganda, Rwanda, Morocco, Algeria, Madagascar, Cameroon, and Mozambique - is less than 5%[90,91].
In locations where they have been used, recuperative energy interventions in the plastic waste handling order, such as gasification, pyrolysis, and incineration, have guaranteed a closed-loop system for plastic waste management. However, only developed and middle-income nations that emphasize waste management with circular economy concerns are currently able to implement these costly and more advanced energy recovery technologies. In Africa, there is a dearth of plastic waste-to-energy conversion technologies, which forces people and organizations to engage in open, unregulated burning activities with hazardous environmental effects[90,91].
The growth of plastic garbage in many developing nations has been linked to behavioral psychology, changing consumption habits, and inadequate waste collection and treatment infrastructures[92]. Developing nations frequently have an overwhelming amount of urgent social and infrastructure demands, which prevents them from coordinating appropriate waste management programs with national development initiatives. Thus, as indicated by the widening of streets and rivers, overflow from waste disposal sites, increased composition in municipal solid waste, contamination of shorelines and beaches, and pollution of aquatic systems, the plastic threat is more severe in low- and middle-income nations[93].
Africa and the Middle East stand out as the two continents where the growing buildup of improperly handled plastics has been found. For example, plastic waste in Ghana has been attributed to improper consumption patterns and the rising income of the country’s middle class. The story of plastic leakage is a prevalent environmental aspect, especially in metropolitan areas where careless dumping has led to clogged drains, flooding, cholera and diarrhea outbreaks, an ugly landscape, and a threat to tourism[90,94]. The majority of Africa’s carbon emissions equations do not account for the impact of environmental waste plastics and their carbon footprints, despite the fact that the manufacture of plastic, burning, and poor waste management release greenhouse gases that fuel climate change. The outwardness of plastic mismanagement is mainly attributed to inappropriate social practices and bad political decisions made at the central government level. Africa is thought to have the greatest number of country-level plastic waste management systems worldwide, but the continent is also hindered by a lack of producer-consumer responsibilities, investment and funding channels, and trash management policies[90].
There are huge differences in waste management systems around the globe, and many nations lack any kind of public trash management system. A significant amount of plastic in mixed household garbage nowadays is non-biodegradable and cannot be composted. As a result, over time, plastic will accumulate as a result of traditional garbage disposal techniques including dumping in backyards, rivers, or private or public landfills. Even tiny towns may experience significant environmental pollution due to plastic litter[95]. Furthermore, nations even with well-established waste management systems annually deposit millions of tons of plastic waste into landfills, which is comprised of a sizable amount of controlled and collected post-consumer garbage[96]. Although the implications in terrestrial systems have not yet been well documented, there have been reports of animal consumption and livestock and wildlife entanglement that can prove lethal. According to Lebreton and Andrady (2019), there is a significant potential to reduce the input of plastic and therefore MP (defined as plastic particles < 5 mm) to the environment through better waste management and waste reduction[92]. The annual amounts of mismanaged waste are estimated to triple by 2060 due to continued poor waste management, growing plastic production, and a growing population[96].
As has been noted, municipal trash contains a large number of plastic products, which may wind up in the environment and disintegrate over time, creating secondary MPs. Consequently, municipal garbage represents a huge prospective supply of MPs in the future. Globally, the yearly volumes of garbage have reached hundreds of tonnes and are growing. Consequently, although they mostly exist as macroplastic products now, municipal garbage has the potential to become the greatest source of MPs in the future[92,96].
The majority of plastic debris that finds its way into the marine ecosystem comes from terrestrial sources. Among the involved transportation mechanisms are: (1) rain and wind carry street debris into rivers; (2) inappropriate or forbidden garbage disposal; (3) inadequately maintained landfills; (4) facilities for the production and processing of plastic; (5) spills from drains and treatment of waste; (6) fishing activities; and (7) facilities for disposing of solid waste along the coast[97,98].
Recycling is now the most extensively used method of handling plastics. Global rates have progressively increased to represent 24% of non-fiber plastic garbage generated in 2014. The countries with the highest recycling rates in that year were China (25%) and Europe (30%), with the United States having a rate of 9%, which was average for the globe[19]. Currently, textiles are either burned or disposed of together with other solid trash instead of being recycled. Approximately 53% of plastic trash is recycled, 46% is utilized for energy, and 1% is dumped in landfills. Recycling can extend the lifespan of plastics before they are discarded into the environment, but the recycling process may result in the generation of MPs[97].
The dataset below, from OECD Library Global Plastics Outlook, provides estimates of plastic waste for the different global regions and/or continents. This dataset also provides estimates of plastic waste measured in millions of tons per year, per end-of-life fate after accounting for recycling losses and collected littering, for the period 2000-2019[32]. It is clear that throughout the last two decades, USA, Canada, the European Union, and Asia have been the dominant regions that contribute to the pollution of aquatic environments due to the great increase in plastic waste. In Figure 3, it is clear that industrialized and developed regions produce a higher amount of plastic waste compared to continents such as Oceania, which do not exhibit the same level of industrial development or population as regions like Asia.
Figure 3. Total plastic waste by region (2000-2019)[32].
The likelihood that poorly managed plastic garbage will find its way into river systems and eventually the ocean varies. Mismanaged plastic waste’s likelihood of ending up in the ocean is influenced by a number of factors, including climate, geography, land use, and distances within river basins. The likelihood of improperly managed garbage being released into the ocean is displayed in Figures 4 and 5.
Figure 4. Accumulated stock in rivers and lakes (2000-2019)[32].
Figure 5. Plastic leakage to oceans (2000-2019)[32].
To begin, in areas lacking adequate local waste management techniques, plastic pollution prevails. This indicates that a significant amount of improperly managed plastic debris may initially ends up in rivers and the ocean, underscoring the necessity for improved waste management practices. In addition, cities closest to major polluters typically featured paved areas where plastic waste and water runoff can easily reach river outlets.
While cities in Asia, Africa, or Latin America are often situated alongside relatively small rivers, they contribute significantly to the continent’s plastic pollutants. Additionally, possible abundant rainfall in these riverine regions exacerbates the issue, leading to the rapid transportation of plastics into the rivers, which then swiftly flow into the ocean. Furthermore, proximity matters, as cities near the ocean and major river sources tend to cause the highest concentrations of MPs in their waters.
Plastic that ends up in our seas can come from both marine and terrestrial sources. The term “plastics pollution from marine sources” describes the pollution left behind by fishing fleets that employ abandoned boats, fishing nets, lines, and ropes. The proportional contribution of terrestrial and marine sources to ocean pollution is a topic of frequent and heated discussion. Figures 6 and 7 present the origin of MPs in the ocean (regions and application of use correspondingly).
Figure 6. Transport of MPs to oceans (2000-2019)[32]. MPs: Microplastics.
Figure 7. Sources of MPs: Plastic use by application (2000-2019)[32]. MPs: Microplastics.
However, it is necessary to underline that surface movements and the direction of the winds in the water have a significant impact on the dispersion and buildup of plastic debris in the ocean. Plastics often travel along the primary surface currents and wind patterns due to their buoyancy, allowing them to float on the ocean’s surface. Consequently, they tend to accumulate in gyres within marine cycles, with the highest concentrations found at the centers of ocean basins and lower concentrations at the periphery. Once plastics enter ocean basins from coastal areas, they exhibit a propensity to move toward their central regions.
The most recent estimates indicate that, globally, land-based sources account for around 80% of ocean plastics, with marine sources contributing to the remaining 20%. Roughly half (10 percentage points) of the 20% derived from marine sources are thought to come from fishing fleets (such as nets, lines, and damaged ships)[4]. The usage of main plastics by industry is depicted in Figure 5, along with the industry’s contribution to plastic trash creation. In addition to the product lifespan, primary plastic use has a significant impact on the development of plastic trash. For example, the active lifespan of packaging is quite short - typically no more than six months. In comparison, plastic use in architecture (building),construction, or transportation has an average lifespan of 35 years. Thus, packaging is the primary source of plastic trash, accounting for about half of the total worldwide.
Figure 8 shows the percentage of plastic garbage generated worldwide in 2000–2019 that is disposed of, recycled (A), burned (B), landfilled (C), littered (D), and mismanaged (E). It is obvious that each region/continent manages the plastic waste in a different way and this has a possible impact or a footprint in the accumulation of MPs in water that is observed. In the past, plastic was typically discarded intact, as recycling or incineration were uncommon practices. However, starting around 1980, the rate of incineration began to increase, and from 1990 onwards, there was a notable rise in recycling rates.
Figure 8. Plastic waste by region (2000-2019). (A) Recycled; (B) Incinerated; (C) Landfilled; (D) Littered; and (E) Mismanaged[32].
The greatest solution for a plastics circular economy has been identified as landfill diversion through recycling and energy recovery. Currently, the United States, European Union, and Asia have the highest rates of plastics ending up in landfills. On the other hand, the majority of African countries choose to leave their garbage - plastic waste in particular - in landfills or rubbish dumps, often with inadequate management practices. Africa’s approach to plastic waste management is notably distinct due to socioeconomic and political reasons. Formal recycling infrastructure in Africa is relatively limited, with even modern systems in South Africa, Ethiopia, Uganda, Rwanda, Morocco, Algeria, Madagascar, Cameroon, and Mozambique collectively showing less than 5% capacity for recycling. Plastic packaging contributes significantly to Africa’s waste stream, especially with the widespread use of bottled and sachet “pure water”. In South Africa, for instance, plastic leakage results in an annual oceanic loading of approximately 40,000 metric tons of plastic waste[90].
Figures 9 and 10 focus on the analysis of data from the year 2019, specifically examining the presence of macroplastics and MPs in oceans, rivers, and lakes, as well as their origins. Macroplastics in aquatic environments predominantly stem from mismanaged plastic waste, particularly originating from countries with weak economies or/and in stages of development, primarily in Africa, Asia and Latin America. In Figure 10, it is obvious that the main sources of immediate MPs in aquatic environments are MP dust, tyre abrasion, primary pellets, wastewater (WW) sludge, and particles from materials used in road construction.
Figure 9. Macroplastics in aquatic environments in 2019[32].
Figure 10. Microplastics in aquatic environments in 2019[32].
In the next section, future prospects and estimations concerning the fate of MPs in rivers/lakes and oceans are presented, juxtaposed with the future strategies for plastic waste management. Put simply, by the end of 2060, as depicted in Figure 11, global plastic waste will either undergo recycling or be directed toward landfilling, followed by incineration or, in the worst-case scenario, complete mismanagement. It is imperative to address plastic waste appropriately to mitigate future pollution effects. Whenever feasible, plastics should undergo recycling, and when that is not possible, they should be landfilled hygienically or incinerated with energy recovery. This reduces the amount of improperly managed trash and leaves only difficult-to-treat sources of leakage, such as uncollected litter and MPs. Consequently, international recycling rates will ascend, while rates of improper waste disposal will decline.
Figure 11. Global estimates of plastic waste per end-of-life fate by 2060[32].
The goal of curbing plastic waste reaching rivers and oceans by 2060 hinges on minimizing leakage to aquatic habitats. However, the accumulation of plastic in these water bodies persists until all avenues of leakage are sealed off and the flow completely ceases. Despite efforts, substantial amounts of plastics continue to leak into the environment [Figure 11], with large volumes ending up in aquatic areas. This is due to the approximately 40-year timeframe required to decrease mismanaged garbage to minimal levels. The cumulative volume of plastic will keep rising, mostly in rivers and lakes and less in oceans.
In addition, a future scenario anticipates a decrease in the release of MPs into the environment [Figure 12]. This decline is attributed to the overall economy utilizing less plastic, primarily through recycling and reusing practices. As a consequence of the worldwide effort to control the primary sources of MPs in the water in a sustainable manner, primary pellets, wastewater sludge, and road markings account for the most significant decreases in MP leakage. Future policy measures are poised to prevent a significant rise in primary plastic consumption, with projections indicating an 11% increase in primary plastic production between 2019 and 2060 [Figure 13]. This trend stems from secondary plastics penetrating the market more quickly alongside a diminishing total demand. As targets for recycled content escalate in the coming years, secondary manufacturing is experiencing notably robust growth. This surge is attributable to an overall reduction in plastic manufacturing, leading to decreased demand for plastics and subsequent waste generation, thereby decreasing the amount of recyclable plastic scrap available. Finally, forecasts regarding plastic usage by type up to 2060 [Figure 14], indicate that 89% of MP concentration in aquatic environments will come mainly from the aforementioned primary MPs, shedding light on their prevalent causes and sources.
Figure 12. Estimates of plastic leakage [in millions of Tons (t)] to the aquatic environments at the global level by 2060[32].
Figure 13. Global Ambition by 2060-Plastic category in aquatic environments[32].
Figure 14. Estimates of plastics use disaggregated by type by 2060[32].
The amount of plastic debris and particles is growing annually due to the rising global population and greater consumption. These substances pose unknown and untested threats to human health and the environment since they are easily transported over great distances and widely distributed on land, in the sea, and in the air. Despite the possible risks to environmental health, there are very few documented cases of plastic particle pollution of environmental media in Africa. Understanding the origins, transit, and destiny of plastic particles via regional and international collaboration and multidisciplinary research is necessary for managing environmental waste plastics and plastic particles and guaranteeing successful intervention policies and practices. We advocate for special control of MPs in consumer items due to the possible harm that plastic particles might cause to ecological functions and health hazards to humans. The data presented in this work contribute to the expanding corpus of knowledge in the global research of micro- and nanoplastic particles, with a focus on developing nations[90].
RESEARCH AND KNOWLEDGE GAPS
The amount of micro- and nanoplastics that are present in the globe is unknown, but due to their detrimental impact on the ecosystem, it is critical to assess the degree of their contamination. It is challenging to assess the degree of damage to human health due to the information gap surrounding this pollution, especially in light of the lack of conclusive data about the impact of micro- and nanoplastics on human health in the human diet. Additionally, it is imperative to furnish precise data to evaluate the exact extent of human exposure to these particles in their everyday diets. This can be achieved through the establishment of standard methodologies for detecting and characterizing micro- and nanoplastics[99,100].
It is difficult to determine the presence of micro- and nanoplastics in marine water and sediment since it depends on the collection technique, sample preparation, and analytical approach. The concentration of particles in nature and the laboratory varied significantly from one another. Furthermore, despite numerous attempts, many environmental influences are unfeasible to replicate in the laboratory; as a result, experimental laboratory conditions and related outcomes are not representative of the field[101].
The fate of micro- and nanoplastics during the metabolism of seafood is not well understood. Opportunities exist in the field of scientific research to close the information gap about the long-term effects of micro- and nanoplastics, particularly with regard to human risk assessment. Numerous investigations have revealed a connection between metabolic, morphological, and behavioral alterations and the presence of micro- and nanoplastics in the food chain. The effects of plastic particle pollution will therefore reach the level of ecosystems. However, the fate of micro- and nanoplastics in marine environments and their consequent environmental effects remain unclear. This lack of clarity poses a challenge in accurately assessing the potential harm to human health posed by micro- and nanoplastics[99].
CONCLUSIONS
This paper was organized into two parts: The first part provides an overview of all published papers focusing on the transport routes of MPs, while the second part analyzes the correlation between improper plastic waste management and high levels of MP accumulation. Identifying precisely where litter is generated is crucial for targeting priority areas for future implementation of mitigation policies regarding MPs in water. Aquatic habitats are threatened by MPs, which have long been recognized as a severe category of pollutants. Therefore, this review covers the mechanics of MPs transport and the handling of plastic trash. The relationship between improperly managed plastic trash and the processes leading to MP accumulation in waterways is not well understood, necessitating further research in this area. After outlining the potential future paths for MPs, we conducted a statistical analysis of several approaches to managing plastic waste to reduce MP pollution. The pervasive presence of MPs in aquatic ecosystems poses a serious threat to both freshwater and marine life. Although research on the presence of MPs in rivers, lakes, and seas has expanded recently, more needs to be learned about the external factors influencing their transit and dispersion in freshwater systems. Given the increasing input of plastic into the environment, the distribution and abundance of MPs in aquatic systems are likely to rise significantly in the coming decades. Unless urgent global action is taken, it is estimated that by 2060, there will be more plastic in the ocean than fish.
DECLARATIONS
Authors’ contributions
Validation, formal analysis, investigation, resources, data curation, writing - original draft preparation: Belioka MP
Conceptualization, methodology, writing - review and editing, supervision: Belioka MP, Achilias DS
Both authors have read and agreed to the published version of the manuscript.
Availability of data and materials
The authors used the raw data provided by OECD Library. Global plastics outlook. Available from: https://www.oecd-ilibrary.org/environment/data/global-plastic-outlook_c0821f81-en. [Last accessed on 16 May 2024] and they continued with the further process, using the Microsoft Excel software. Moreover, this is a review paper and all the data and information have been found in the different sources that are mentioned in the “REFERENCES” section.
Financial support and sponsorship
None.
Conflicts of interest
Both authors declared that there are no conflicts of interest.
Ethical approval and consent to participate
Not applicable.
Consent for publication
Not applicable.
Copyright
© The Author(s) 2024.
REFERENCES
1. Pellini G, Gomiero A, Fortibuoni T, et al. Characterization of microplastic litter in the gastrointestinal tract of Solea solea from the Adriatic Sea. Environ Pollut 2018;234:943-52.
2. Zhang K, Hamidian AH, Tubić A, et al. Understanding plastic degradation and microplastic formation in the environment: a review. Environ Pollut 2021;274:116554.
3. Ram B, Kumar M. Correlation appraisal of antibiotic resistance with fecal, metal and microplastic contamination in a tropical Indian river, lakes and sewage. npj Clean Water 2020;3:3.
4. Belioka MP, Achilias DS. Microplastic pollution and monitoring in seawater and harbor environments: a meta-analysis and review. Sustainability 2023;15:9079.
5. Li W, Luo Y, Pan X. Identification and characterization methods for microplastics basing on spatial imaging in micro-/nanoscales. In: He D, Luo Y, editors. Microplastics in terrestrial environments: emerging contaminants and major challenges. Springer, Cham. 2020. pp. 25-37.
6. Herbort AF, Schuhen K. A concept for the removal of microplastics from the marine environment with innovative host-guest relationships. Environ Sci Pollut Res Int 2017;24:11061-5.
7. Arthur C, Baker JE, Bamford HA. Proceedings of the international research workshop on the occurrence, effects, and fate of microplastic marine debris, September 9-11, 2008, University of Washington Tacoma, Tacoma, WA, USA. 2009. Available from: https://repository.library.noaa.gov/view/noaa/2509. [Last accessed on 16 May 2024]
8. Collignon A, Hecq JH, Galgani F, Collard F, Goffart A. Annual variation in neustonic micro-and meso-plastic particles and zooplankton in the Bay of Calvi (Mediterranean-Corsica). Mar Pollut Bull 2014;79:293-8.
9. Lestari P, Trihadiningrum Y, Wijaya BA, Yunus KA, Firdaus M. Distribution of microplastics in Surabaya river, Indonesia. Sci Total Environ 2020;726:138560.
10. Chamas A, Moon H, Zheng J, et al. Degradation rates of plastics in the environment. ACS Sustain Chem Eng 2020;8:3494-511.
11. Constant M, Ludwig W, Kerhervé P, et al. Microplastic fluxes in a large and a small Mediterranean river catchments: the Têt and the Rhône, Northwestern Mediterranean Sea. Sci Total Environ 2020;716:136984.
12. He B, Smith M, Egodawatta P, Ayoko GA, Rintoul L, Goonetilleke A. Dispersal and transport of microplastics in river sediments. Environ Pollut 2021;279:116884.
13. Rose PK, Jain M, Kataria N, Sahoo PK, Garg VK, Yadav A. Microplastics in multimedia environment: a systematic review on its fate, transport, quantification, health risk, and remedial measures. Groundw Sustain Dev 2023;20:100889.
14. Bondelind M, Sokolova E, Nguyen A, Karlsson D, Karlsson A, Björklund K. Hydrodynamic modelling of traffic-related microplastics discharged with stormwater into the Göta River in Sweden. Environ Sci Pollut Res Int 2020;27:24218-30.
15. Rist S, Almroth BC, Hartmann NB, Karlsson TM. A critical perspective on early communications concerning human health aspects of microplastics. Sci Total Environ 2018;626:720-6.
16. Sharma S, Chatterjee S. Microplastic pollution, a threat to marine ecosystem and human health: a short review. Environ Sci Pollut Res Int 2017;24:21530-47.
17. Barboza LGA, Gimenez BCG. Microplastics in the marine environment: current trends and future perspectives. Mar Pollut Bull 2015;97:5-12.
18. Rajmohan KVS, Ramya C, Viswanathan MR, Varjani S. Plastic pollutants: effective waste management for pollution control and abatement. Curr Opin Environ Sci Health 2019;12:72-84.
19. Geyer R, Jambeck JR, Law KL. Production, use, and fate of all plastics ever made. Sci Adv 2017;3:e1700782.
20. Malli A, Corella-Puertas E, Hajjar C, Boulay AM. Transport mechanisms and fate of microplastics in estuarine compartments: a review. Mar Pollut Bull 2022;177:113553.
21. Zettler ER, Mincer TJ, Amaral-Zettler LA. Life in the “plastisphere”: microbial communities on plastic marine debris. Environ Sci Technol 2013;47:7137-46.
22. Cooper DA, Corcoran PL. Effects of mechanical and chemical processes on the degradation of plastic beach debris on the island of Kauai, Hawaii. Mar Pollut Bull 2010;60:650-4.
23. Nelms SE, Coombes C, Foster LC. et al. Marine anthropogenic litter on British beaches: a 10-year nationwide assessment using citizen science data. Sci Total Environ 2017;579:1399-409.
24. Zheng Y, Yanful EK, Bassi AS. A review of plastic waste biodegradation. Crit Rev Biotechnol 2005;25:243-50.
25. Barnes DKA, Galgani F, Thompson RC, Barlaz M. Accumulation and fragmentation of plastic debris in global environments. Philos Trans R Soc Lond B Biol Sci 2009;364:1985-98.
26. Li J, Liu H, Chen JP. Microplastics in freshwater systems: a review on occurrence, environmental effects, and methods for microplastics detection. Water Res 2018;137:362-74.
27. Mahdavimanesh M, Noghrehabadi AR, Behbahaninejad M, Ahmadi G, Dehghanian M. Lagrangian particle tracking: model development. Life Sci J 2013;10:34-41. Available from: https://citeseerx.ist.psu.edu/document?repid=rep1&type=pdf&doi=66a90cb17c5aaa3816e504848f152b94c2e5a315. [Last accessed on 16 May 2024].
28. Alosairi Y, Al-Salem SM, Al Ragum A. Three-dimensional numerical modelling of transport, fate and distribution of microplastics in the northwestern Arabian/Persian Gulf. Mar Pollut Bull 2020;161:111723.
29. Rangel-Buitrago N, Vergara-Cortés H, Barría-Herrera J, Contreras-López M, Agredano R. Marine debris occurrence along Las Salinas beach, Viña Del Mar (Chile): magnitudes, impacts and management. Ocean Coast Manage 2019;178:104842.
30. Hardesty BD, Harari J, Isobe A, et al. Using numerical model simulations to improve the understanding of micro-plastic distribution and pathways in the marine environment. Front Mar Sci 2017;4:30.
31. Moher D, Liberati A, Tetzlaff J, Altman DG; PRISMA Group. Preferred reporting items for systematic reviews and meta-analyses: the PRISMA statement. Ann Intern Med 2009;151:264-9.
32. OECD Library. Global plastics outlook. Available from: https://www.oecd-ilibrary.org/environment/data/global-plastic-outlook_c0821f81-en. [Last accessed on 16 May 2024].
33. Koutnik VS, Leonard J, Alkidim S, et al. Distribution of microplastics in soil and freshwater environments: global analysis and framework for transport modeling. Environ Pollut 2021;274:116552.
34. Horton AA, Walton A, Spurgeon DJ, Lahive E, Svendsen C. Microplastics in freshwater and terrestrial environments: evaluating the current understanding to identify the knowledge gaps and future research priorities. Sci Total Environ 2017;586:127-41.
35. Prata JC, Silva ALP, da Costa JP, et al. Solutions and integrated strategies for the control and mitigation of plastic and microplastic pollution. Int J Environ Res Public Health 2019;16:2411.
37. Weithmann N, Möller JN, Löder MGJ, Piehl S, Laforsch C, Freitag R. Organic fertilizer as a vehicle for the entry of microplastic into the environment. Sci Adv 2018;4:eaap8060.
38. Alomar C, Estarellas F, Deudero S. Microplastics in the Mediterranean Sea: deposition in coastal shallow sediments, spatial variation and preferential grain size. Mar Environ Res 2016;115:1-10.
39. Murphy F, Ewins C, Carbonnier F, Quinn B. Wastewater treatment works (WwTW) as a source of microplastics in the aquatic environment. Environ Sci Technol 2016;50:5800-8.
40. Cole M, Lindeque P, Halsband C, Galloway TS. Microplastics as contaminants in the marine environment: a review. Mar Pollut Bull 2011;62:2588-97.
41. Leslie HA, Brandsma SH, van Velzen MJM, Vethaak AD. Microplastics en route: field measurements in the Dutch river delta and Amsterdam canals, wastewater treatment plants, North Sea sediments and biota. Environ Int 2017;101:133-42.
42. Eklund B, Hansson T, Bengtsson H, Eriksson Wiklund AK. Pollutant concentrations and toxic effects on the red alga Ceramium tenuicorne of sediments from natural harbors and small boat harbors on the west coast of Sweden. Arch Environ Contam Toxicol 2016;70:583-94.
43. De Witte B, Devriese L, Bekaert K, et al. Quality assessment of the blue mussel (Mytilus edulis): comparison between commercial and wild types. Mar Pollut Bull 2014;85:146-55.
44. Gauquie J, Devriese L, Robbens J, De Witte B. A qualitative screening and quantitative measurement of organic contaminants on different types of marine plastic debris. Chemosphere 2015;138:348-56.
45. Auta HS, Emenike CU, Fauziah SH. Distribution and importance of microplastics in the marine environment: a review of the sources, fate, effects, and potential solutions. Environ Int 2017;102:165-76.
46. Carr SA, Liu J, Tesoro AG. Transport and fate of microplastic particles in wastewater treatment plants. Water Res 2016;91:174-82.
47. Karbalaei S, Hanachi P, Walker TR, Cole M. Occurrence, sources, human health impacts and mitigation of microplastic pollution. Environ Sci Pollut Res Int 2018;25:36046-63.
48. Hou H, Wang J, Yuan M, et al. Estimating the mitigation potential of the Chinese service sector using embodied carbon emissions accounting. Environ Impact Assess Rev 2021;86:106510.
49. Obbard RW, Sadri S, Wong YQ, Khitun AA, Baker I, Thompson RC. Global warming releases microplastic legacy frozen in Arctic Sea ice. Earths Future 2014;2:315-20.
50. He D, Luo Y, Lu S, Liu M, Song Y, Lei L. Microplastics in soils: analytical methods, pollution characteristics and ecological risks. TrAC Trend Anal Chem 2018;109:163-72.
51. Rillig MC, Ingraffia R, de Souza Machado AA. Microplastic incorporation into soil in agroecosystems. Front Plant Sci 2017;8:1805.
52. Wang W, Ge J, Yu X, Li H. Environmental fate and impacts of microplastics in soil ecosystems: progress and perspective. Sci Total Environ 2020;708:134841.
53. Nizzetto L, Futter M, Langaas S. Are agricultural soils dumps for microplastics of urban origin? Environ Sci Technol 2016;50:10777-9.
54. Browne MA, Galloway T, Thompson R. Microplastic - an emerging contaminant of potential concern? Integr Environ Assess Manag 2007;3:559-61.
55. Dubaish F, Liebezeit G. Suspended microplastics and black carbon particles in the Jade system, southern North Sea. Water Air Soil Poll 2013;224:1352.
56. Mahon AM, O’Connell B, Healy MG, et al. Microplastics in sewage sludge: effects of treatment. Environ Sci Technol 2017;51:810-8.
57. Scarascia-Mugnozza G, Sica C, Russo G. Plastic materials in European agriculture: actual use and perspectives. J Agric Eng 2011;42:15-28.
58. Kasirajan S, Ngouajio M. Polyethylene and biodegradable mulches for agricultural applications: a review. Agron Sustain Dev 2012;32:501-29.
59. Shiu RF, Chen LY, Lee HJ, Gong GC, Lee C. New insights into the role of marine plastic-gels in microplastic transfer from water to the atmosphere via bubble bursting. Water Res 2022;222:118856.
60. Yuan W, Christie-Oleza JA, Xu EG, et al. Environmental fate of microplastics in the world’s third-largest river: basin-wide investigation and microplastic community analysis. Water Res 2022;210:118002.
61. Yuan Z, Nag R, Cummins E. Ranking of potential hazards from microplastics polymers in the marine environment. J Hazard Mater 2022;429:128399.
62. Kurniawan TA, Haider A, Ahmad HM, et al. Source, occurrence, distribution, fate, and implications of microplastic pollutants in freshwater on environment: a critical review and way forward. Chemosphere 2023;325:138367.
63. Wong JKH, Lee KK, Tang KHD, Yap PS. Microplastics in the freshwater and terrestrial environments: prevalence, fates, impacts and sustainable solutions. Sci Total Environ 2020;719:137512.
64. Bejgarn S, MacLeod M, Bogdal C, Breitholtz M. Toxicity of leachate from weathering plastics: an exploratory screening study with Nitocra spinipes. Chemosphere 2015;132:114-9.
65. Teuten EL, Saquing JM, Knappe DRU, et al. Transport and release of chemicals from plastics to the environment and to wildlife. Philos Trans R Soc Lond B Biol Sci 2009;364:2027-45.
66. Jambeck JR, Geyer R, Wilcox C, et al. Marine pollution. Plastic waste inputs from land into the ocean. Science 2015;347:768-71.
67. Obbard RW. Microplastics in polar regions: the role of long range transport. Curr Opin Environ Sci Health 2018;1:24-9.
69. Dris R, Imhof H, Sanchez W, et al. Beyond the ocean: contamination of freshwater ecosystems with (micro-) plastic particles. Environ Chem 2015;12:539-50.
70. Horton AA, Dixon SJ. Microplastics: an introduction to environmental transport processes. WIREs Water 2018;5:e1268.
71. Boucher J, Friot D. Primary microplastics in the oceans: a global evaluation of sources. 2017. Available from: https://portals.iucn.org/library/sites/library/files/documents/2017-002-En.pdf. [Last accessed on 16 May 2024].
72. Abbasi S, Keshavarzi B, Moore F, et al. Distribution and potential health impacts of microplastics and microrubbers in air and street dusts from Asaluyeh County, Iran. Environ Pollut 2019;244:153-64.
73. Chae Y, An YJ. Effects of micro-and nanoplastics on aquatic ecosystems: current research trends and perspectives. Mar Pollut Bull 2017;124:624-32.
74. Pelley AJ, Tufenkji N. Effect of particle size and natural organic matter on the migration of nano- and microscale latex particles in saturated porous media. J Colloid Interface Sci 2008;321:74-83.
75. Bradford SA, Bettahar M. Concentration dependent transport of colloids in saturated porous media. J Contam Hydrol 2006;82:99-117.
76. Liu M, Lu S, Song Y, et al. Microplastic and mesoplastic pollution in farmland soils in suburbs of Shanghai, China. Environ Pollut 2018;242:855-62.
77. Ali MU, Lin S, Yousaf B, et al. Environmental emission, fate and transformation of microplastics in biotic and abiotic compartments: global status, recent advances and future perspectives. Sci Total Environ 2021;791:148422.
78. Dris R, Gasperi J, Saad M, Mirande C, Tassin B. Synthetic fibers in atmospheric fallout: a source of microplastics in the environment? Mar Pollut Bull 2016;104:290-3.
79. Critchell K, Lambrechts J. Modelling accumulation of marine plastics in the coastal zone; what are the dominant physical processes? Estuar Coast Shelf Sci 2016;171:111-22.
80. Filella M. Questions of size and numbers in environmental research on microplastics: methodological and conceptual aspects. Environ Chem 2015;12:527-38.
81. Ryan PG. Does size and buoyancy affect the long-distance transport of floating debris? Environ Res Lett 2015;10:084019.
82. Shimanaga M, Yanagi K. The Ryukyu Trench may function as a “depocenter” for anthropogenic marine litter. J Oceanogr 2016;72:895-903.
84. Alimi OS, Budarz JF, Hernandez LM, Tufenkji N. Microplastics and nanoplastics in aquatic environments: aggregation, deposition, and enhanced contaminant transport. Environ Sci Technol 2018;52:1704-24.
85. Hidalgo-Ruz V, Gutow L, Thompson RC, Thiel M. Microplastics in the marine environment: a review of the methods used for identification and quantification. Environ Sci Technol 2012;46:3060-75.
86. Law KL, Morét-Fergusonet S, Maximenko NA, et al. Plastic accumulation in the North Atlantic subtropical gyre. Science 2010;329:1185-8.
87. Zhang Y, Gao T, Kang S, Sillanpää M. Importance of atmospheric transport for microplastics deposited in remote areas. Environ Pollut 2019;254:112953.
88. Haque F, Fan C. Fate and impacts of microplastics in the environment: hydrosphere, pedosphere, and atmosphere. Environments 2023;10:70.
89. Hamid F, Bhatti MS, Anuar N, Anuar N, Mohan P, Periathamby A. Worldwide distribution and abundance of microplastic: how dire is the situation? Waste Manag Res 2018;36:873-97.
90. Angnunavuri PN, Attiogbe F, Mensah B. Consideration of emerging environmental contaminants in africa: review of occurrence, formation, fate, and toxicity of plastic particles. Sci Afr 2020;9:e00546.
91. Yoshida M. Situation of municipal solid waste management in African Cities - An interpretation of the information provided by the first ACCP meeting. 2018. Available from: http://www.gwopa.org/sites/default/files/2023-06/8_Discussion_Paper_2.pdf. [Last accessed on 16 May 2024].
92. Lebreton L, Andrady A. Future scenarios of global plastic waste generation and disposal. Palgrave Commun 2019;5:6.
93. Tackling plastic waste and pollution for human health and marine biodiversity - A call for global action. 2019. Available from: https://www.fauna-flora.org/wp-content/uploads/2023/05/Tearfund-FFI_2020-Tackling-plastic-waste.pdf. [Last accessed on 16 May 2024].
94. Wilson DC, Rodic L, Modak P, et al. Global waste management outlook. 2015. Available from: https://www.uncclearn.org/wp-content/uploads/library/unep23092015.pdf. [Last accessed on 16 May 2024].
95. Free CM. Jensen OP, Mason SA, Eriksen M, Williamson N, Boldgiv B. High-levels of microplastic pollution in a large, remote, mountain lake. Mar Pollut Bull 2014;85:156-63.
96. Haave M, Henriksen T. Sources and fate of microplastics in Urban Systems. In: Rocha-Santos T, Costa MF, Mouneyrac C, editors. Handbook of microplastics in the environment. Springer, Cham. 2022. pp. 849-75.
97. Chaukura N, Kefeni KK, Chikurunhe I, et al. Microplastics in the aquatic environment - the occurrence, sources, ecological impacts, fate, and remediation challenges. Pollutants 2021;1:95-118.
98. UNEP. Marine litter: a global challenge. 2009. Available from: https://wedocs.unep.org/bitstream/handle/20.500.11822/7787/-Marine%20Litter_%20A%20Global%20Challenge%20(2009)-2009845.pdf?sequence=3&%3BisAllowed=. [Last accessed on 16 May 2024].
99. Al-Thawadi S. Microplastics and nanoplastics in aquatic environments: challenges and threats to aquatic organisms. Arab J Sci Eng 2020;45:4419-40.
100. Mattsson K, Jocic S, Doverbratt I, Hansson LA. Chapter 13 - Nanoplastics in the aquatic environment. In: Microplastic contamination in aquatic environments. 2018. pp. 379-99.
Cite This Article
Export citation file: BibTeX | EndNote | RIS
OAE Style
Belioka MP, Achilias DS. How plastic waste management affects the accumulation of microplastics in waters: a review for transport mechanisms and routes of microplastics in aquatic environments and a timeline for their fate and occurrence (past, present, and future). Water Emerg Contam Nanoplastics 2024;3:14. http://dx.doi.org/10.20517/wecn.2024.09
AMA Style
Belioka MP, Achilias DS. How plastic waste management affects the accumulation of microplastics in waters: a review for transport mechanisms and routes of microplastics in aquatic environments and a timeline for their fate and occurrence (past, present, and future). Water Emerging Contaminants & Nanoplastics. 2024; 3(3): 14. http://dx.doi.org/10.20517/wecn.2024.09
Chicago/Turabian Style
Maria-Paraskevi Belioka, Dimitrios S. Achilias. 2024. "How plastic waste management affects the accumulation of microplastics in waters: a review for transport mechanisms and routes of microplastics in aquatic environments and a timeline for their fate and occurrence (past, present, and future)" Water Emerging Contaminants & Nanoplastics. 3, no.3: 14. http://dx.doi.org/10.20517/wecn.2024.09
ACS Style
Belioka, M.P.; Achilias DS. How plastic waste management affects the accumulation of microplastics in waters: a review for transport mechanisms and routes of microplastics in aquatic environments and a timeline for their fate and occurrence (past, present, and future). Water. Emerg. Contam. Nanoplastics. 2024, 3, 14. http://dx.doi.org/10.20517/wecn.2024.09
About This Article
Special Issue
Copyright
Data & Comments
Data
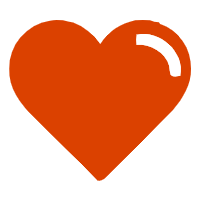
Comments
Comments must be written in English. Spam, offensive content, impersonation, and private information will not be permitted. If any comment is reported and identified as inappropriate content by OAE staff, the comment will be removed without notice. If you have any queries or need any help, please contact us at support@oaepublish.com.