Metabolism, sex, and what lies beyond the scalpel in MASLD
Abstract
The discovery of the one-carbon metabolism-homocysteine-metabolic dysfunction-associated steatotic liver disease (OCM-Hcy-MASLD) axis has renewed our understanding of MASLD-related primary liver cancer (PLC). Based on Suzuki et al.’s mathematical modeling findings of diminished cystathionine β-synthase (CBS) and phosphatidylethanolamine N-methyltransferase (PEMT) expression in MASLD, this commentary analyzes recent findings regarding sex-specific variations in this axis and their implications for surgical management. We highlight how the integration of OCM-Hcy pathway modulation with precise surgical interventions could enhance perioperative outcomes and long-term prognosis. The emerging evidence suggests that targeted metabolic interventions, particularly those accounting for sex differences, may complement traditional surgical approaches by addressing the systemic nature of MASLD-related PLC. This paradigm shift from purely surgical resection toward comprehensive metabolic regulation marks a significant advance in precision medicine for hepatobiliary surgery, potentially improving both perioperative safety and oncological outcomes.
Keywords
INTRODUCTION
Metabolic dysfunction-associated steatotic liver disease (MASLD, formerly NAFLD) currently exceeds a 30% global prevalence, making it a primary contributor to the contemporary burden of liver disease[1]. In the ongoing quest to elucidate MASLD pathogenesis, the emerging centrality of the one-carbon metabolism (OCM) pathway and its regulatory product, homocysteine (Hcy), has become increasingly apparent. Through mathematical modeling, Suzuki et al. substantiated that diminished expression of two key OCM enzymes - cystathionine β-synthase (CBS) and phosphatidylethanolamine N-methyltransferase (PEMT) - correlates significantly with the progression of hepatic fibrosis in individuals with MASLD[2]. Their work further indicates that strategic OCM cofactor supplementation may effectively modulate hepatic Hcy levels, while highlighting the pivotal role of sex-related factors in both disease progression and therapeutic response. Collectively, these findings point toward a new frontier in the stratified treatment of MASLD.
Moreover, given that MASLD has been identified as an independent risk factor for primary liver cancer (PLC), including hepatocellular carcinoma (HCC) and cholangiocarcinoma (CCA)[3,4], the elucidation of this metabolic pathway holds profound implications for the field of hepatobiliary surgery. The bidirectional interplay between metabolic derangement and tumorigenesis is reshaping traditional surgical strategies. The liver’s role as a central metabolic hub, coupled with the systemic nature of PLC, compels a critical reappraisal of the limitations inherent in surgical resection alone. In the era of precision medicine, integrating metabolic characteristics into preoperative assessment and implementing targeted metabolic interventions during the perioperative period may fundamentally enhance both surgical outcomes and patient prognosis.
Accordingly, this paper endeavors to systematically analyze the findings of Suzuki et al., sketch the molecular landscape of the OCM-Hcy-MASLD axis, underscore the significance of sex-based modulation within this continuum, and examine its transformative potential for PLC surgery[2]. By highlighting these insights, we strive to extend the reach of precision medicine into both the mechanistic understanding of hepatic metabolic disorders and the evolving surgical strategies that address them.
ADVANCES IN CLINICS AND MECHANISM
The intersection of OCM and hepatic pathology was first documented in 1974 when Gaull et al. observed hepatocellular enlargement and microvesicular steatosis in liver biopsies from patients with severe hyperhomocysteinemia due to CBS deficiency[5]. This association was mechanically confirmed by
Subsequent mechanistic investigations have elucidated four primary pathogenic pathways through which OCM perturbation and Hcy elevation promote liver injury: oxidative stress induction[7], endoplasmic reticulum stress activation[8], reduced nitric oxide bioavailability[9], and protein homocysteinylation-mediated cellular dysfunction[10]. These molecular mechanisms collectively contribute to hepatic inflammation and fibrosis progression, highlighting the multifaceted role of the OCM-Hcy axis in MASLD pathogenesis.
The clinical relevance of this pathway was further validated by Gulsen et al., who demonstrated a robust correlation between serum Hcy levels and MASLD severity[11]. Their findings established Hcy as an independent risk factor for hepatic inflammation and fibrosis progression, providing a strong rationale for therapeutic targeting of the OCM-Hcy axis in MASLD treatment. This clinical correlation has profound implications for surgical decision making, as elevated Hcy levels may serve as a biomarker for disease severity and surgical risk stratification.
Recent advances[12,18] have substantiated the causal relationship within the OCM-Hcy-MASLD axis [Table 1], elucidating the regulatory effects of OCM cofactors on Hcy homeostasis and MASLD progression. This emerging molecular landscape not only provides multiple therapeutic targets but also highlights the promising therapeutic potential of OCM cofactor intervention strategies. The intricate molecular mechanisms underlying the OCM-Hcy-MASLD axis have been progressively unveiled.
Mechanistic studies of OCM-Hcy-MASLD axis
Authors | Year of publication | Country | Sample source | Related conclusion |
Ai et al.[12] | China | 2017 | C57BL/6J mice | ER stress and PERK-eIF2α pathway mediate Hcy-induced MASLD progression via SREBP-1c activation and de novo lipogenesis |
Liang et al.[13] | China | 2019 | C57BL/6J mice and human HepG2 cells | Orphan nuclear receptor NR4A1 suppresses Hcy-induced MASLD through H3K27 acetylation, with its agonist CsnB as a potential therapeutic target |
Yan et al.[14] | China | 2020 | C57BL/6J mice and Human plasma | Hcy induces MASLD via HIF1α-ERO1α-dependent oxidative stress pathway, while adipocyte-specific HIF1α deletion ameliorates this pathological process |
Tripathi et al.[15] | Singapore | 2022 | C57BL/6J mice, primate models and human serum and liver tissue | Hcy promotes MASH progression through STX17 homocysteinylation and ubiquitination-mediated autophagy dysfunction, which can be improved by vitamin B12 and folate supplementation |
Bagherieh et al.[16] | Iran | 2023 | Human HepG2 cells | Folate ameliorates palmitate-induced inflammation in HepG2 cells by reducing Hcy levels, ROS production, and NF-κB pathway activation |
Wang et al.[17] | China | 2023 | Macrophage-specific PDHA1 gene knockout mice | Macrophage-specific PDHA1 deletion exacerbates Hcy-induced MASLD through enhanced hepatocyte apoptosis |
Xiang et al.[18] | China | 2023 | C57BL/6J mice | Hcy activates NLRP3 inflammasome via MDM2-mediated HSF1 K372 ubiquitination, leading to MASLD and insulin resistance |
SEX-SPECIFIC REGULATION MATTERS
Although a growing body of literature now identifies the OCM-Hcy-MASLD axis as a key mediator of hepatic injury, the sex-specific intricacies of these interlinked mechanisms have often been overlooked. Most studies to date have not stratified participants by sex or age, limiting the ability to capture how hormonal subpopulations might respond differently to OCM-targeted interventions.
Indeed, estrogens and androgens distinctly regulate key steps within the OCM-Hcy axis, exerting divergent effects on critical enzymes[19]. These divergent influences, in turn, translate into differing metabolic landscapes for men and women, shaping both the natural history of MASLD. A prominent meta-analysis by Balakrishnan et al. underscores this sexual dimorphism, revealing that while women have a 19% lower risk of MASLD (formerly termed NAFLD), they exhibit a 37% higher risk of developing advanced fibrosis compared with men[20]. Notably, menopause at approximately age 50 modifies the effect of sex on disease aggressiveness, with postmenopausal women facing elevated odds of MASH (metabolic dysfunction-associated steatohepatitis, previously known as NASH) and advanced fibrosis. Emerging genetic evidence suggests that this disparity is amplified by PNPLA3 variant interaction - the rs738409-CG polymorphism shows a 40% higher penetrance of fibrosis in men[21]. This was further supported by mechanistic findings from Vilar-Gomez et al., who found that PNPLA3 risk variants disrupt hepatic methionine metabolism[22].
These findings collectively suggest that hormonal fluctuations exert profound effects on hepatic metabolism and disease progression. Women may benefit from protective hormonal profiles during their premenopausal years; yet, once this protective window closes, the subsequent rise in fibrotic burden may be heightened by metabolic perturbations that were previously attenuated. Considering sex, age, and reproductive status (e.g., menarche and menopause) is therefore emerging as a pivotal element in designing precision medicine approaches for MASLD[23].
Against this backdrop, Suzuki et al. [Figure 1] take a bold step forward by pairing traditional clinical insights with a sophisticated mathematical approach, offering more than a descriptive account of Hcy dysregulation[2]. Their simulations of distinct OCM cofactor regimens in men and women deliver practical guidance on how vitamins B6, B12, folate, and betaine might be harnessed synergistically for maximum Hcy-lowering impact. While prior studies have confirmed the importance of OCM cofactors in reducing liver injury, the novelty of Suzuki et al.’s model lies in its capacity to capture subtle sex differences that could otherwise remain obscured[2]. Specifically, the data suggest that although folate alone confers a potent Hcy-lowering effect in both sexes, combining all available cofactors consistently yields the greatest reduction, hinting at untapped therapeutic synergies. This approach powerfully illustrates how theory-driven modeling can accelerate translational research, refining our understanding of MASLD phenotypes and illuminating personalized interventions.
Figure 1. OCM Cofactor Mediated Hcy Regulation[2]: This figure delineates Hcy metabolic regulation, comprising two core cycles (folate cycle and methionine cycle), two exit pathways (transsulfuration and polyamine pathways), with OCM cofactors and sex hormones demonstrating tissue-specific regulatory effects on Hcy levels. Yellow box: Folate cycle. MTHFR catalyzes THF reduction to 5mTHF, which serves as a methyl donor for Hcy methylation. BHMT/BHMT2 mediates 5mTHF regeneration to THF, completing the cyclical process. Red box: Methionine cycle. Met undergoes ATP-dependent conversion to SAM. Subsequent demethylation by GnMT generates SAH, which is hydrolyzed to Hcy. BHMT/BHMT2-mediated methylation regenerates SAM using folate-derived methyl groups. Red arrows: Exit pathways. (1) Transsulfuration: CBS-mediated conversion of Hcy to cysteine permanently removes it from core cycles; (2) Polyamine pathway: SAM decarboxylation yields spermidine/spermine, with final hydroxymethylmethionine exiting the system. Blue box: OCM cofactor regulation. (1) Folate: THF precursor and CBS allosteric activator; (2) B12: Mediates Hcy remethylation; (3) Betaine: Enhances BHMT/BHMT2 efficiency and CBS activation; (4) B6: Essential cofactor for CBS catalytic activity. Purple box: Sex hormone modulation. Estrogen upregulates betaine biosynthesis and CBS activation (Hcy-lowering). Androgen inhibits transsulfuration enzyme expression/activity (Hcy-accumulating). OCM: One-carbon metabolism; THF: tetrahydrofolate; MTHFR: methylenetetrahydrofolate reductase; 5mTHF: 5-methyltetrahydrofolate; -Me: methyl; Met: methionine; SAM: S-adenosylmethionine; GnMT: glycine N-methyltransferase; SAH: S-adenosylhomocysteine; CBS: cystathionine beta-synthase; Hcy: homocysteine; BHMT: betaine-homocysteine methyltransferase.
BRIDGING THE MECHANISMS AND BEDSIDE PROTOCOLS: LIMITATIONS AND OPPORTUNITIES
Despite the promising insights derived from foundational research, several pivotal challenges must be addressed before OCM-targeted interventions can be fully integrated into clinical practice.
First, much of the current understanding of the OCM-Hcy-MASLD axis remains anchored in cellular and animal models, with only limited human validation. Given the complex interplay among genetic susceptibilities, environmental influences, and metabolic shifts in human MASLD, bridging this translational gap is paramount. Large-scale, longitudinal clinical trials that incorporate diverse patient populations will be critical for confirming the therapeutic potential and safety profile of OCM cofactor supplementation.
Second, although emerging data underscore the heterogeneity in Hcy metabolism - particularly between males, females, and distinct age groups - research to date has only begun to explore how these differences might inform individualized treatment. Suzuki et al.’s findings highlight sex-specific responses to OCM cofactor supplementation, but additional factors, such as menopausal status and genetic polymorphisms, could further refine intervention outcomes[2]. A comprehensive understanding of these demographic nuances is indispensable for moving from a one-size-fits-all paradigm toward precision-based approaches.
Third, existing studies often focus on isolated enzymes or substrates in the OCM pathway, an approach that risks oversimplifying the broader metabolic interactions within the liver’s intricate network. Of particular translational relevance, emerging evidence reveals interconnected metabolic networks extending beyond classical OCM components. Recent clinicopathological studies have identified significant polyamine pathway dysregulation in MASLD progression, manifested through elevated ornithine decarboxylase (ODC1) expression and putrescine accumulation in both preclinical models and human biopsies[24]. Notably, this pathway’s perturbation not only exacerbates lipotoxic stress responses but also demonstrates a strong correlation with histopathological severity indices, implying potential mechanistic convergence with the OCM-Hcy axis that warrants systematic exploration. Given that MASLD is, by nature, a multifaceted disease involving dysregulated lipid, glucose, and amino acid metabolism, these findings collectively reinforce the essentiality of a systems biology perspective. To fully unravel the complex crosstalk among these pathways, multifactorial models - particularly those integrating transcriptomic, proteomic, and metabolomic datasets - must be prioritized. Such integrated approaches may yield a more holistic roadmap of OCM-Hcy perturbations and their synergistic contributions to disease progression.
Finally, the pathway from bench to bedside faces logistical difficulties related to the clinical implementation of OCM-targeted therapies. Standardized protocols for quantifying tissue-specific Hcy levels and assessing OCM enzyme activities are lacking, complicating both diagnostic precision and treatment monitoring. The optimal dosing strategy, combination of cofactors, and timing of intervention (including perioperative administration) are similarly undefined. Addressing these gaps will likely require well-designed, controlled trials that incorporate biomarker-driven end points and evaluate real-world efficacy in combination with surgery or other standard-of-care therapies.
Moving forward, robust, multicenter collaborations will be pivotal for generating consensus on clinical endpoints, bridging methodological discrepancies, and establishing universally accepted treatment algorithms. Integration of big data analytics, coupled with machine learning techniques, may further refine patient stratification and illuminate novel, sex-specific targets amenable to OCM-based interventions. Ultimately, these interdisciplinary efforts stand to elevate MASLD management, opening new avenues not only for preventing advanced disease but also for mitigating the oncogenic potential associated with MASLD over the long term.
BEYOND THE SCALPEL: METABOLIC AND PRECISION MEDICINE INSIGHTS
In recent years, PLC has come to be recognized as a systemic metabolic disease rather than a strictly localized malignancy. From an anatomical perspective, the liver functions as the central hub of whole-body metabolism, and recurrence or metastasis - rather than merely the primary tumor load - remains a leading cause of mortality in PLC[25]. Concurrently, tumorigenesis in PLC is fundamentally driven by metabolic reprogramming[26], signifying that no extent of local surgical excision can wholly resolve this global metabolic disorder. Given that MASLD is an established risk factor for PLC, and in light of the promising implications of the OCM-Hcy-MASLD axis, there is a need for a comprehensive treatment paradigm that addresses the metabolic underpinnings of disease. However, it is important to note that the current evidence on the role of OCM in hepatocarcinogenesis is still limited, and further research is needed to fully understand its impact on PLC development and progression.
Revisiting oncological priorities in MASLD-related PLC
MASLD-associated hepatic dysfunction presents unique surgical challenges, particularly regarding compromised hepatic reserve and altered regenerative capacity. Current evidence suggests R0 resection should be balanced with functional preservation[27], especially given the increased perioperative risks in metabolically compromised patients. Emerging data indicate that preoperative metabolic optimization may improve surgical tolerance, though specific protocols require further validation.
Incorporating the OCM-Hcy pathway into full-cycle intervention
The OCM-Hcy axis represents one of several metabolic pathways implicated in MASLD progression. While Suzuki et al.’s modeling provides theoretical support for cofactor supplementation, clinical translation requires rigorous validation[2]. Current evidence supports considering metabolic status during surgical planning rather than implementing specific OCM-targeted protocols.
Refining perioperative management in metabolically at-risk patients
Recent studies have emphasized the need for comprehensive metabolic assessment in patients with MASLD undergoing hepatectomy, and the study by Lopez-Pascual et al. has created a new way to develop perioperative management strategies for patients with MASLD from the perspective of nanomaterials[28]. Sex-specific metabolic changes merit consideration but should not override established perioperative protocols.
Anticipating future directions for MASLD-related PLC management
Therapeutic interventions targeting metabolic pathways, such as the OCM-Hcy-MASLD axis, may reduce perioperative risks. For patients with MASLD-related PLC, leveraging this axis through targeted pharmacotherapy - e.g., Hcy-lowering agents and metabolic regulators - could disrupt tumor-promoting processes while simultaneously improving hepatic reserve. Moreover, integrating these regimens with minimally invasive surgical approaches has the potential to minimize postoperative morbidity, especially in individuals burdened by obesity or other metabolic derangements.
CONCLUSION
In conclusion, the discovery of the OCM-Hcy-MASLD axis and its sex-specific characteristics has opened new avenues for improving perioperative management and long-term prognosis. Looking ahead, the integration of multi-omics data - including metabolomics, epigenomics, and microbiomics - coupled with the establishment of an OCM-Hcy axis-centered biomarker monitoring system and the combination of precision surgery with individualized metabolic interventions, holds promise for fundamentally enhancing outcomes in patients with MASLD-related PLC. This paradigm shift not only represents an evolution from mere surgical resection to comprehensive metabolic regulation but also marks a significant step forward in hepatobiliary surgery toward the era of precision medicine.
DECLARATIONS
Authors’ contributions
Conceptualized the commentary, conducted the literature review, and contributed to the writing and editing of the manuscript: Li ZL, Tang ZH
Assisted in the literature: Chen JL, Tang Y, Qin DL
Availability of data and materials
Not applicable.
Financial support and sponsorship
None.
Conflicts of interest
Tang ZH is a Junior Editorial Board member of the journal Metabolism and Target Organ Damage. Tang ZH was not involved in any steps of editorial processing, notably including reviewers’ selection, manuscript handling and decision making. The other authors declared that there are no conflicts of interest.
Ethical approval and consent to participate
Not applicable.
Consent for publication
Not applicable.
Copyright
© The Author(s) 2025.
REFERENCES
1. Li Z, Chen J, Tang Y, Qin D, Tang Z. MASLD: an emerging factor in the pathophysiology and clinical management of ICC. Hepatoma Res. 2024;10:41.
2. Suzuki A, Henao R, Reed MC, et al. Lower hepatic CBS and PEMT expression in advanced NAFLD: inferencing strategies to lower homocysteine with a mathematical model. Metab Target Organ Damage. 2024;4:21.
3. Lugari S, Baldelli E, Lonardo A. Metabolic primary liver cancer in adults: risk factors and pathogenic mechanisms. Metab Target Organ Damage. 2023;3:5.
4. Llovet JM, Willoughby CE, Singal AG, et al. Nonalcoholic steatohepatitis-related hepatocellular carcinoma: pathogenesis and treatment. Nat Rev Gastroenterol Hepatol. 2023;20:487-503.
5. Gaull G, Sturman JA, Schaffner F. Homocystinuria due to cystathionine synthase deficiency: enzymatic and ultrastructural studies. J Pediatr. 1974;84:381-90.
6. Torres L, García-Trevijano ER, Rodríguez JA, et al. Induction of TIMP-1 expression in rat hepatic stellate cells and hepatocytes: a new role for homocysteine in liver fibrosis. Biochim Biophys Acta. 1999;1455:12-22.
8. Werstuck GH, Lentz SR, Dayal S, et al. Homocysteine-induced endoplasmic reticulum stress causes dysregulation of the cholesterol and triglyceride biosynthetic pathways. J Clin Invest. 2001;107:1263-73.
9. Upchurch GR Jr, Welch GN, Fabian AJ, et al. Homocyst(e)ine decreases bioavailable nitric oxide by a mechanism involving glutathione peroxidase. J Biol Chem. 1997;272:17012-7.
10. Jakubowski H. Metabolism of homocysteine thiolactone in human cell cultures: possible mechanism for pathological consequences of elevated homocysteine levels. J Biol Chem. 1997;272:1935-42.
11. Gulsen M, Yesilova Z, Bagci S, et al. Elevated plasma homocysteine concentrations as a predictor of steatohepatitis in patients with non-alcoholic fatty liver disease. J Gastroenterol Hepatol. 2005;20:1448-55.
12. Ai Y, Sun Z, Peng C, Liu L, Xiao X, Li J. Homocysteine induces hepatic steatosis involving ER stress response in high methionine diet-fed mice. Nutrients. 2017;9:346.
13. Liang H, Xie X, Song X, et al. Orphan nuclear receptor NR4A1 suppresses hyperhomocysteinemia-induced hepatic steatosis in vitro and in vivo. FEBS Lett. 2019;593:1061-71.
14. Yan Y, Wu X, Wang P, et al. Homocysteine promotes hepatic steatosis by activating the adipocyte lipolysis in a HIF1α-ERO1α-dependent oxidative stress manner. Redox Biol. 2020;37:101742.
15. Tripathi M, Singh BK, Zhou J, et al. Vitamin B12 and folate decrease inflammation and fibrosis in NASH by preventing syntaxin 17 homocysteinylation. J Hepatol. 2022;77:1246-55.
16. Bagherieh M, Kheirollahi A, Zamani-Garmsiri F, Emamgholipour S, Meshkani R. Folic acid ameliorates palmitate-induced inflammation through decreasing homocysteine and inhibiting NF-κB pathway in HepG2 cells. Arch Physiol Biochem. 2023;129:893-900.
17. Wang Meng, Sun Yue, Yang Anning, et al. Effect of macrophage PDHA1 gene knockout on apoptosis of hepatocytes in mice with non-alcoholic fatty liver disease. Chin J Pathophysiol. 2023;39:123-30.
18. Xiang W, Yang Y, Weng L, et al. Hyperhomocysteinemia activates NLRP3 inflammasome to cause hepatic steatosis and insulin resistance via MDM2-mediated ubiquitination of HSF1. Int Immunopharmacol. 2023;118:110085.
19. Kim R, Nijhout HF, Reed MC. One-carbon metabolism during the menstrual cycle and pregnancy. PLoS Comput Biol. 2021;17:e1009708.
20. Balakrishnan M, Patel P, Dunn-Valadez S, et al. Women have a lower risk of nonalcoholic fatty liver disease but a higher risk of progression vs men: a systematic review and meta-analysis. Clin Gastroenterol Hepatol. 2021;19:61-71.e15.
21. Cherubini A, Rosso C, Della Torre S. Sex-specific effects of PNPLA3 I148M. Liver Int. 2025;45:e16088.
22. Vilar-Gomez E, Pirola CJ, Sookoian S, et al. Impact of the association between PNPLA3 genetic variation and dietary intake on the risk of significant fibrosis in patients with NAFLD. Am J Gastroenterol. 2021;116:994-1006.
23. Lonardo A, Nascimbeni F, Ballestri S, et al. Sex differences in nonalcoholic fatty liver disease: state of the art and identification of research gaps. Hepatology. 2019;70:1457-69.
24. Núñez-Sánchez MÁ, Martínez-Sánchez MA, Sierra-Cruz M, et al. Increased hepatic putrescine levels as a new potential factor related to the progression of metabolic dysfunction-associated steatotic liver disease. J Pathol. 2024;264:101-11.
25. Satriano L, Lewinska M, Rodrigues PM, Banales JM, Andersen JB. Metabolic rearrangements in primary liver cancers: cause and consequences. Nat Rev Gastroenterol Hepatol. 2019;16:748-66.
26. Lu M, Wu Y, Xia M, Zhang Y. The role of metabolic reprogramming in liver cancer and its clinical perspectives. Front Oncol. 2024;14:1454161.
27. Mejia JC, Pasko J. Primary liver cancers: intrahepatic cholangiocarcinoma and hepatocellular carcinoma. Surg Clin North Am. 2020;100:535-49.
Cite This Article

How to Cite
Li, Z.L.; Tang, Y.; Qin, D.L.; Chen, J.L.; Tang, Z.H. Metabolism, sex, and what lies beyond the scalpel in MASLD. Metab. Target. Organ. Damage. 2025, 5, 19. http://dx.doi.org/10.20517/mtod.2025.05
Download Citation
Export Citation File:
Type of Import
Tips on Downloading Citation
Citation Manager File Format
Type of Import
Direct Import: When the Direct Import option is selected (the default state), a dialogue box will give you the option to Save or Open the downloaded citation data. Choosing Open will either launch your citation manager or give you a choice of applications with which to use the metadata. The Save option saves the file locally for later use.
Indirect Import: When the Indirect Import option is selected, the metadata is displayed and may be copied and pasted as needed.
About This Article
Copyright
Data & Comments
Data
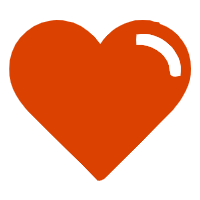
Comments
Comments must be written in English. Spam, offensive content, impersonation, and private information will not be permitted. If any comment is reported and identified as inappropriate content by OAE staff, the comment will be removed without notice. If you have any queries or need any help, please contact us at [email protected].