Fabry nephropathy: focus on podocyte damage and therapeutic target
Abstract
Fabry disease, a rare X-linked lysosomal storage disorder, is marked by a deficiency in the activity of the enzyme
Keywords
INTRODUCTION
Fabry disease (FD) is an inherited X-linked lysosomal storage disorder resulting from mutations in the GLA gene. This gene is responsible for the production of α-galactosidase A (α-GalA) and leads to a potentially fatal buildup of globotriaosylceramide (Gb3) and relevant glycosphingolipids in tissues that contain lysosomes. The estimated prevalence of this condition ranges from 1 in 40,000 to 1 in 117,000 individuals[1]. In newborn screenings, the prevalence is underestimated, ranging from 1 in 8,882 to 1 in 12,500[2]. High-risk and family screening studies have revealed a higher frequency of FD than previously expected. Therefore, the estimated prevalence varies depending on the type of screening, such as family, newborn, non-dialysis-dependent chronic kidney disease patients, dialysis patients, and kidney transplant recipients[3]. FD can be categorized into two phenotypes: the classic and the non-classic. Classic FD primarily occurs in males, with almost complete deficiency of α-Gal A activity, and complications such as kidney, heart, and cerebrovascular issues appear earlier, leading to a poor prognosis. In contrast, the late-onset FD retains residual enzymatic activity, often manifesting as single-organ involvement, typically affecting the kidneys or heart[4].
Kidney complications are a predominant factor of morbidity and mortality in individuals with FD. The primary clinical presentations of renal involvement include proteinuria, hypertension, and progressive chronic kidney disease (CKD). Given that FD is a progressive disorder, it is essential for patients to receive a definitive diagnosis promptly, so that they gain early monitoring, supportive care, and suitable treatment options, which can prevent the onset of irreversible and potentially fatal complications. Since 2001, enzyme replacement therapy (ERT) has been the primary treatment for FD. This treatment aims to stabilize the condition, prevent the progression of organ damage, and alleviate disease symptoms. In addition, a chaperone therapy known as migalastat (Galafold®, Amicus) was approved for use in certain FD patients in the European Union in 2016 and subsequently in the United States in 2018[5].
Podocytes are primary targets in Fabry nephropathy [Figure 1]. The accumulation of Gb3 causes injury to podocytes, which are essential components of glomerular cells, responsible for maintaining the integrity of the glomerular filtration barrier. In pediatric renal biopsies, the deposition of Gb3 within renal cells and the podocyte foot process effacement can be detected, preceding the clinical onset of pathological albuminuria. Previous studies have demonstrated that podocytes in Fabry nephropathy accumulate the most Gb3, leading to the early occurrence of (micro)albuminuria[6,7]. Given the restricted ability to regenerate, podocyte damage and loss are regarded as pivotal events in the pathophysiology of kidney disease, making them prime targets for therapeutic intervention. Inflammation also plays a significant pathogenetic role in FD. Accumulated Gb3 in lysosomes results in the accumulation of impaired organelles and protein aggregates, which initiate inflammation and oxidative stress. This initiates the synthesis of extracellular matrix proteins to facilitate tissue repair. However, if uncontrolled, this reparative response can evolve into a pathological state, causing excessive protein accumulation and renal fibrosis[8]. In this work, we offer a comprehensive review of Fabry nephropathy, with a particular focus on kidney podocyte lesions and treatment options.
Figure 1. (A) The typical pathological manifestations of glomerulus in Fabry disease under light microscope. Vacuole-like changes in the cytoplasm of podocytes and parietal epithelial cells were observed. (Periodic Acid-Schiff stain, ×400 times); (B) The typical pathological manifestations of glomerulus in Fabry disease under light microscope. A large number of Gb3 bodies were deposited in the cytoplasm of podocytes and parietal epithelial cells, and toluidine blue staining was observed. (×400 times); (C) Typical glomerular pathological manifestations of Fabry disease under electron microscope. A large number of “zebra bodies” formed by Gb3 deposition in the cytoplasm of podocytes and foot process segmental fusion.
CLINICAL FEATURES OF FABRY NEPHROPATHY
Kidney involvement occurs in 55% of Fabry disease patients[9]. Albuminuria and estimated glomerular filtration rate (eGFR) are the definitive indicators for tracking kidney conditions associated with FD
Clinical manifestation of Fabry nephropathy
Laboratory examination | Clinical manifestation |
Urinalysis | Microalbuminuria in early phases, then progress to moderate to severe proteinuria in adulthood |
Hematuria | |
Kidney function | Glomerular hyperfiltration is an early marker, then a progressive decrease in GFR |
Urine microscopy | Mulberry cells with characteristic “Maltese cross bodies”, podocyturia |
Tubular dysfunction | α1-microglobulin, N-acetyl-β-glucosaminidase, and alanine aminopeptidase were elevated in early phases, isosthenuria, distal renal tubular acidosis |
Ultrasound findings | Renal cysts, mainly parapelvic ones |
PATHOLOGICAL FEATURES AND PATHOGENESIS
Kidney biopsy offered crucial insights that were not accessible from standard evaluations of renal function and proteinuria levels, underscoring the significance of renal biopsy as an initial assessment tool for all individuals with Fabry disease, regardless of the severity of clinical presentation. Kidney biopsies can identify and measure Gb3 deposits in individuals with a 30-300 mg/g albumin-to-creatinine ratio and normal renal function. For women without signs of FD-related kidney disease, substantial renal deposits could indicate the need to initiate specific treatment[14]. Fogo et al. developed a standardized biopsy scoring system for disease-specific lesions and general progression lesions in kidney biopsies, revealing a range of histological changes in the early stages of Fabry nephropathy. This underscores the importance of kidney biopsies for baseline assessments, even in cases with minimal clinical manifestations[15]. The initial damage in Fabry disease stems from the accumulation of glycolipids in nearly all types of cells within the glomerulus, with particularly large accumulations in podocytes. These deposits are not confined to the glomerular cells but are also found within the tubular cells and the endothelium of the peritubular capillaries[16]. The progression of kidney disease is primarily characterized by podocyte damage, persistent proteinuria, and permanent damage to the small blood vessels within the kidneys, leading to progressive glomerulosclerosis, capillary wall thickening, tubular atrophy, interstitial fibrosis, and arterial and arteriolar sclerosis. Several studies showed glomerulosclerosis and segmental sclerosis levels are sensitive indicators for the kidney progression of patients with FD[13,17].
Gb3 deposits in podocytes have drawn particular attention from researchers. On light microscopy, vacuoles were frequently observed in podocytes. Electron microscopy revealed that the Gb3 accumulations within the cells manifested as distinctive osmiophilic inclusions in the podocytes’ cytoplasm, exhibiting a pattern of alternating light and dark concentric rings. These osmiophilic myelin bodies had a diameter ranging from 1 to 3 nm and presented a "zebra-like" pattern [Figure 1]. Effacement of the segmental foot processes was also found in the podocytes. Using quantitative morphometric electron microscopic studies, it was indicated that the proportion of podocyte cytoplasm filled with Gb3 deposits rises with age but stabilizes after the age of 27. This implies that there exists a critical level of Gb3 accumulation beyond which podocytes may sustain injury and loss[18]. Indeed, podocyturia (loss of podocytes through the urine) in Fabry nephropathy has been generally examined and is considered an indicator of early kidney involvement in FD[19].
The accumulation of Gb3 can lead to its conversion into globotriaosylsphingosine (lyso-Gb3), a process likely facilitated by the non-specific action of lysosomal acid ceramidase that cleaves the fatty acid from Gb3. Elevated lyso-Gb3 levels in the plasma of FD patients suggest that it is a more reliable biomarker for tracking disease progression compared to plasma Gb3 levels[20]. In cultured podocytes, Lyso-Gb3 triggered the activation of Notch1, leading to an increase in the production of tumor growth factor β1 (TGF-β1), which facilitates the development of fibrosis and sustained inflammation by the synthesis of extracellular matrix in renal cells via epithelial-to-mesenchymal transition[21]. Another study found that human podocytes respond to lyso-Gb3 by initially increasing the expression of genes for the αvβ3 integrin and uPAR. The αvβ3/UPAR system plays a pivotal role in the detachment of podocytes and the subsequent release of these cells in the urine, a process known as podocyturia[22]. Analyses utilizing transcriptome connectivity mapping and SILAC quantitative proteomics showed the deposits of α-synuclein (SNCA) as a critical factor in podocyte damage. Suppressing SNCA through genetic and pharmacological interventions may enhance the lysosomal structure and functionality in Fabry podocytes[23].
CURRENT TREATMENT
Enzyme replacement therapy
Since 2001, ERT has been available as a specific treatment option to compensate forαGalA deficiency[24] [Table 2]. At present, three formulations of recombinant human αGalA are accessible: agalsidase α(Replagal®), agalsidase β(Fabrazyme®), and a biosimilar of agalsidase β (JR-051). Agalsidase α is manufactured using a human cell line and administered at a dosage of 0.2 mg/kg, with an infusion duration of 40 min. Agalsidase β is derived from Chinese hamster ovary cells, with a suggested dosage of
Potential effects of enzymatic and supportive therapy on podocytes
Treatments | Effects on podocytes |
Enzyme replacement therapy | A reduction in Gb3 levels within podocytes |
Chaperone therapy | Reduced Gb3 deposits on podocytes |
SNCA inhibitor | Improve lysosome podocyte structure and function |
ACEI/ARB | Decrease progressive kidney disease by alleviating podocyte injury |
SGLT2 inhibitors | Alleviate podocyte damage by targeting the pathogenetic mechanisms, such as oxidative stress and inflammation |
Data from the Fabry Outcome Survey (FOS) indicated that early initiation of ERT with agalsidase α can slow renal function deterioration and improve symptoms, and it also underscored the sustained efficacy and safety of ERT in FD patients[27]. Sustained administration of agalsidase β has been demonstrated to effectively clear Gb3 from mesangial and glomerular endothelial cells[28]. A dose-dependent clearance of Gb3 in podocytes was also reported[28]. Research by Nowak et al. indicated that the licensed dosage of Agalsidase β outperforms Agalsidase α in lowering Lyso-Gb3 levels among patients with classic FD[29]. However,
Chaperone therapy
Migalastat, a pharmaceutical chaperone, has gained approval as the first oral medication for FD since 2016. It is specifically indicated for patients with amenable mutations, predominantly those with attenuated, late-onset forms of the disease who retain significant residual enzyme activity. Individuals with these genetic variants rarely have severe renal disease, resulting in minimal or absent podocyte impairment[31]. Migalastat is a derivative of 1-deoxygalactonojirimycin and acts as a structural stabilizer for the terminal galactose of Gb3, enhancing susceptible mutant forms of the α-Gal A enzyme. Additionally, this compound increases and stabilizes the lysosomal activity[32], promoting the transport of susceptible mutant α-Gal A from the endoplasmic reticulum to lysosomes. The enhancement of kidney and heart function, coupled with increased α-Gal A enzyme activity and reduced Gb3 deposits after chaperone therapy, indicated that migalastat could be a practical therapeutic choice and a secure substitute for ERT among FD patients[33]. Moreover, the capability of migalastat to penetrate the blood-brain barrier is promising, and its efficacy on neurological symptoms remains to be confirmed in upcoming studies.
Developing treatments
Substrate reduction therapies function by decreasing the synthesis of accumulated substrate caused by the lack of α-Gal A activity. A principal benefit of these treatments is their effectiveness regardless of the specific genetic mutation causing the enzymatic deficiency. Notably, they can be used as a standalone treatment or in conjunction with ERT[34].
Pegunigalsidase alfa represents a pegylated variant of the α-Gal A, produced through plant cell culture techniques, and is recently approved in the European Union and United States for the treatment of FD. In terms of efficacy outcomes, kidney biopsies from patients administered pegunigalsidase alfa showed a marked decrease in Gb3 levels, and their renal function was stable[35]. Multiple phase-3 clinical trials are either actively in progress, such as the BALANCE study [NCT02795676], or have recently concluded, including the BRIGHT study [NCT03180840] and the BRIDGE study [NCT03018730].
Gene therapy operates on the principle of introducing DNA that encodes the α-Gal A protein into the patient’s cells. Introducing a therapeutic gene, along with subsequent endogenous cellular expression, will facilitate the production of the enzyme that was originally scarce, potentially altering the course of FD fundamentally[36]. Currently, phase 1/2 clinical trials are exploring the use of in-vivo gene therapy with various biological agents, including adeno-associated virus vectors and non-viral vectors.
Research has shown that although ERT can reduce Gb3 accumulation in podocytes, its effect on podocyte damage repair is not obvious[23]. In addition, the CRISPR/Cas9-mediated knockout of alpha galactose glucoside enzyme of Sertoli cell model confirmed that although ERT can reverse the accumulation of Gb3, it does not solve the problem of lysosome dysfunction[37]. Further transcriptome and quantitative proteomic analysis found that SNCA accumulation is the key factor in podocyte damage[23]. Genetic and pharmacological inhibition of SNCA can improve the structure and function of lysosomal podocytes in Fabry disease, demonstrating an effect that surpasses that of ERT[23,38].
In addition, it has been suggested that podocyte injury in Fabry disease is related to the dysregulation of the autophagy pathway. In podocytes, upregulation of the autophagy marker microtubule-associated protein 1 light chain 3 (LC3-II) was observed, which may be related to defects in mTOR and AKT signaling pathways[39]. mTOR is a key negative regulator of autophagy formation, and AKT is its upstream regulator, both of which show reduced activity in Fabry disease[39]. Reduced mTOR phosphorylation results in a rise in autophagosomes. However, the continuous overactivation of autophagy can cause podocyte injury. Li et al. proposed that a negative feedback loop might exist, where the surge in autophagosomes could reactivate mTOR[40]. This reactivation would suppress further autophagosome creation and promote lysosomal regeneration, possibly serving as a cellular defense against autophagy-induced cell death[40]. Accumulation of Gb3 impairing mitochondrial function can disrupt mTA1 activity, causing spontaneous effects and energy deficits. Therefore, mTOR-dependent pathways are pivotal in regulating autophagic-lysosomal fusion and mitochondrial function. Disruption of the lysosome-autophagy-mitochondria axis is a significant factor in the organ damage associated with FD[41]. Additionally, this interaction may also offer a new direction for treating podocyte damage in Fabry disease[39].
Supportive therapy
Controlling proteinuria is crucial for maintaining renal function in FD patients. Medications such as angiotensin-converting enzyme inhibitors (ACEi) or angiotensin receptor blockers (ARBs) have been shown to slow the progression of renal disease in FD by reducing damage to podocytes[42,43]. Warnock et al. demonstrated individuals who sustained a UP/Cr ratio < 0.5 g/g or a 50% reduction from their initial UP/Cr ratio through the use of an ACEi or ARBs experienced a less decline in eGFR (-3.6 mL/min), in contrast to those who failed to reach these criteria (-7.0 mL/min)[43].
Initially developed to reduce blood glucose levels, SGLT2 inhibitors have become increasingly recognized for their role in CKD management. Recently, SGLT2 inhibitors have gained approval for treating CKD progression in patients with proteinuria. Battaglia et al. suggested that SGLT2 inhibitors can mitigate damage to podocytes by targeting the pathogenetic mechanisms like oxidative stress and inflammation that ERT or chaperone therapy alone might not fully resolve[44]. This study is the first to assess the effect of Dapaglifozin in FD patients with albuminuria and renal function progression.
PODOCYTE REGENERATION
Professor Romagnani from Italy, as early as 2013 after a series of research results, accurate account of the inherent in the human renal unit cells, especially the glomerular cells and renal tubular epithelial cells of progenitor cell source, positioning and differentiation problem[45]. During the embryonic stage, these renal progenitor cells are located in the renal utricle and S-shaped bodies and retain the ability to differentiate into both glomerular and tubular epithelial cells. During subsequent development, the number of RPCs decreases, and at the adult stage, RPCs only account for about 2% of the innate cells in the kidney. As RPCs of podocyte, only exists in the bowman’s capsule wall, at the same time expressing cells and associated RPCs differentiation markers.
Podocyte regeneration for repair and functional recovery after kidney damage is of crucial importance. In the last decade, notable progress has been made in the study of podocyte regeneration. For example, it has been shown that by enhancing the differentiation capacity of renal precursor cells, podocyte regeneration can be enhanced, leading to improved prognosis in chronic kidney disease[46]. In addition, some studies have also discussed the effects of specific drugs such as BIO (GSK3 inhibitor) on cell regeneration[47].
In Fabry disease, the loss of αGalA activity leads to the accumulation of metabolic substrates in podocytes and other cells, which may affect the normal function and regeneration ability of podocytes. Therefore, therapeutic strategies targeting podocyte regeneration may have some potential for the treatment of renal involvement in FD. However, the specific mechanism of the interaction between FD and podocyte regeneration is not fully understood at present, and further studies are needed to elucidate it.
As research on kidney damage markers advances in both theory and methodology, the evaluation of tissue damage using non-invasive biomarkers has gained significant attention in recent years. Small molecules, such as those found in blood and urine tests, are explored, including urine concentrations of dissolved Gb3 in Sertoli cells or the detection of glomerular progenitor cells in urine. At the ERA - EDTA 2024 academic conference, a study by Ugalde-Altamirano et al. from Spain reported that RPCs detected in urine could serve as early non-invasive markers for evaluating kidney damage in Fabry patients[48]. The study investigated the correlation between RPCs and Gb3 accumulation in relation to the severity of renal tissue injury. The research involved Fabry disease patients, healthy controls, and chronic kidney disease (CKD) patients without Fabry disease. RPCs were isolated from urine using specific markers and flow cytometry, and their association with renal function indicators and the level of proteinuria was analyzed. The study found that labeled RPCs were difficult to detect in the urine of healthy individuals, while their numbers significantly increased in the urine of Fabry disease patients and those with biopsy-proven CKD. Additionally, the number of RPCs correlated with the degree of proteinuria. This research identified, for the first time, glomerular-specific progenitor cells with Gb3 accumulation in the urine of Fabry patients, indicating their potential as biomarkers for early kidney injury in this disease. The findings suggest that therapeutic strategies aimed at podocyte regeneration may emerge as a new direction for future research in Fabry disease, potentially improving renal prognosis for patients.
CONCLUSION
FD is a lysosomal storage disease that can affect multiple organs and presents diverse and non-specific clinical manifestations. Among the histological features of renal involvement, podocyte lesions are the most severely affected. Early ERT can delay disease progression and improve prognosis. The implementation of a new dynamic monitoring mechanism for podocyte injury, along with the expanded concept of cell repair and regeneration therapy, combined with comprehensive interdisciplinary symptom management, offers an optimistic outlook for the prognosis of patients with renal impairment due to FD.
DECLARATION
Authors’ contributions
Concept and design of the review,and drafting: Zhang D
Literature collection: Xie K
Revising the manuscript: Zhang J
All authors read and approved the final version of the manuscript.
Availability of data and materials
Not applicable.
Financial support and sponsorship
None.
Conflicts of interest
All authors declared that there are no conflicts of interest.
Ethical approval and consent to participate
Not applicable.
Consent for publication
Not applicable.
Copyright
The Author(s) 2024.
REFERENCES
1. Vardarli I, Rischpler C, Herrmann K, Weidemann F. Diagnosis and screening of patients with Fabry disease. Ther Clin Risk Manag 2020;16:551-8.
2. Linhart A, Germain DP, Olivotto I, et al. An expert consensus document on the management of cardiovascular manifestations of Fabry disease. Eur J Heart Fail 2020;22:1076-96.
3. Battaglia Y, Fiorini F, Azzini C, et al. Deficiency in the screening process of Fabry disease: analysis of chronic kidney patients not on dialysis. Front Med 2021;8:640876.
4. Germain DP, Altarescu G, Barriales-Villa R, et al. An expert consensus on practical clinical recommendations and guidance for patients with classic Fabry disease. Mol Genet Metab 2022;137:49-61.
5. Germain DP, Fan JQ. Pharmacological chaperone therapy by active-site-specific chaperones in Fabry disease: in vitro and preclinical studies. Int J Clin Pharmacol Ther 2009;47 (Suppl 1):S111-7.
6. Najafian B, Svarstad E, Bostad L, et al. Progressive podocyte injury and globotriaosylceramide (GL-3) accumulation in young patients with Fabry disease. Kidney Int 2011;79:663-70.
7. Tøndel C, Bostad L, Hirth A, Svarstad E. Renal biopsy findings in children and adolescents with Fabry disease and minimal albuminuria. Am J Kidney Dis 2008;51:767-76.
8. Kurdi H, Lavalle L, Moon JCC, Hughes D. Inflammation in Fabry disease: stages, molecular pathways, and therapeutic implications. Front Cardiovasc Med 2024;11:1420067.
9. Mehta A, Clarke JT, Giugliani R, et al. FOS Investigators. Natural course of Fabry disease: changing pattern of causes of death in FOS - Fabry outcome survey. J Med Genet 2009;46:548-52.
10. Wanner C, Oliveira JP, Ortiz A, et al. Prognostic indicators of renal disease progression in adults with Fabry disease: natural history data from the Fabry registry. Clin J Am Soc Nephrol 2010;5:2220-8.
11. Echevarria L, Benistan K, Toussaint A, et al. X-chromosome inactivation in female patients with Fabry disease. Clin Genet 2016;89:44-54.
12. Řeboun M, Sikora J, Magner M, et al. Pitfalls of X-chromosome inactivation testing in females with Fabry disease. Am J Med Genet A 2022;188:1979-89.
13. Zhang D, Zhang J, Liang S, Wang J, Liu Z. Clinic-pathologic features and renal outcome of Fabry disease: data from a Chinese cohort. Am J Nephrol 2018;48:137-46.
14. Svarstad E, Marti HP. The changing landscape of Fabry disease. Clin J Am Soc Nephrol 2020;15:569-76.
15. Fogo AB, Bostad L, Svarstad E, et al. all members of the International Study Group of Fabry Nephropathy (ISGFN). Scoring system for renal pathology in Fabry disease: report of the International Study Group of Fabry Nephropathy (ISGFN). Nephrol Dial Transplant 2010;25:2168-77.
16. Tøndel C, Bostad L, Larsen KK, et al. Agalsidase benefits renal histology in young patients with Fabry disease. J Am Soc Nephrol 2013;24:137-48.
17. Valbuena C, Carvalho E, Bustorff M, et al. Kidney biopsy findings in heterozygous Fabry disease females with early nephropathy. Virchows Arch 2008;453:329-38.
18. Najafian B, Tøndel C, Svarstad E, Gubler MC, Oliveira JP, Mauer M. Accumulation of globotriaosylceramide in podocytes in Fabry nephropathy is associated with progressive podocyte loss. J Am Soc Nephrol 2020;31:865-75.
19. Trimarchi H, Canzonieri R, Costales-Collaguazo C, et al. Early decrease in the podocalyxin to synaptopodin ratio in urinary Fabry podocytes. Clin Kidney J 2019;12:53-60.
20. Nikolaenko V, Warnock DG, Mills K, Heywood WE. Elucidating the toxic effect and disease mechanisms associated with Lyso-Gb3 in Fabry disease. Hum Mol Genet 2023;32:2464-72.
21. Matafora V, Cuccurullo M, Beneduci A, et al. Early markers of Fabry disease revealed by proteomics. Mol Biosyst 2015;11:1543-51.
22. Trimarchi H, Ortiz A, Sánchez-Niño MD. Lyso-Gb3 increases αvβ3 integrin gene expression in cultured human podocytes in Fabry nephropathy. J Clin Med 2020;9:3659.
23. Braun F, Abed A, Sellung D, et al. Accumulation of α-synuclein mediates podocyte injury in Fabry nephropathy. J Clin Invest 2023;133:e157782.
25. El Dib R, Gomaa H, Carvalho RP, et al. Enzyme replacement therapy for anderson-Fabry disease. Cochrane Database Syst Rev 2016;7:CD006663.
26. Cybulla M, Nicholls K, Feriozzi S, et al. FOS Study Group. Renoprotective effect of agalsidase alfa: a long-term follow-up of patients with Fabry disease. J Clin Med 2022;11:4810.
27. Beck M, Ramaswami U, Hernberg-Ståhl E, et al. Twenty years of the Fabry outcome survey (FOS): insights, achievements, and lessons learned from a global patient registry. Orphanet J Rare Dis 2022;17:238.
28. Tøndel C, Thurberg BL, DasMahapatra P, et al. Clinical relevance of globotriaosylceramide accumulation in Fabry disease and the effect of agalsidase beta in affected tissues. Mol Genet Metab 2022;137:328-41.
29. Nowak A, Dormond O, Monzambani V, Huynh-Do U, Barbey F. Agalsidase-β should be proposed as first line therapy in classic male Fabry patients with undetectable α-galactosidase A activity. Mol Genet Metab 2022;137:173-8.
30. Arends M, Biegstraaten M, Wanner C, et al. Agalsidase alfa versus agalsidase beta for the treatment of Fabry disease: an international cohort study. J Med Genet 2018;55:351-8.
31. Weidemann F, Jovanovic A, Herrmann K, Vardarli I. Chaperone therapy in Fabry disease. Int J Mol Sci 2022;23:1887.
32. Yam GH, Zuber C, Roth J. A synthetic chaperone corrects the trafficking defect and disease phenotype in a protein misfolding disorder. FASEB J 2005;19:12-8.
33. Müntze J, Gensler D, Maniuc O, et al. Oral chaperone therapy migalastat for treating Fabry disease: enzymatic response and serum biomarker changes after 1 year. Clin Pharmacol Ther 2019;105:1224-33.
34. Coutinho MF, Santos JI, Alves S. Less is more: substrate reduction therapy for lysosomal storage disorders. Int J Mol Sci 2016;17:1065.
35. Schiffmann R, Goker-Alpan O, Holida M, et al. Pegunigalsidase alfa, a novel PEGylated enzyme replacement therapy for Fabry disease, provides sustained plasma concentrations and favorable pharmacodynamics: a-year phase 1/2 clinical trial. J Inherit Metab Dis 2019;42:534-44.
36. Domm JM, Wootton SK, Medin JA, West ML. Gene therapy for Fabry disease: progress, challenges, and outlooks on gene-editing. Mol Genet Metab 2021;134:117-31.
37. Song HY, Chiang HC, Tseng WL, et al. Using CRISPR/Cas9-mediated GLA gene knockout as an in vitro drug screening model for Fabry disease. Int J Mol Sci 2016;17:2089.
38. Germain DP. Reconceptualizing podocyte damage in Fabry disease: new findings identify α-synuclein as a putative therapeutic target. Kidney Int 2024;105:237-9.
39. Liebau MC, Braun F, Höpker K, et al. Dysregulated autophagy contributes to podocyte damage in Fabry’s disease. PLoS One 2013;8:e63506.
40. Li P, Xi Y, Zhang Y, et al. GLA mutations suppress autophagy and stimulate lysosome generation in Fabry disease. Cells 2024;13:437.
41. Tuttolomondo A, Simonetta I, Riolo R, et al. Pathogenesis and molecular mechanisms of Anderson-Fabry disease and possible new molecular addressed therapeutic strategies. Int J Mol Sci 2021;22:10088.
42. Warnock DG, Ortiz A, Mauer M, et al. Fabry Registry. Renal outcomes of agalsidase beta treatment for Fabry disease: role of proteinuria and timing of treatment initiation. Nephrol Dial Transplant 2012;27:1042-9.
43. Warnock DG, Thomas CP, Vujkovac B, et al. Antiproteinuric therapy and Fabry nephropathy: factors associated with preserved kidney function during agalsidase-beta therapy. J Med Genet 2015;52:860-6.
44. Battaglia Y, Bulighin F, Zerbinati L, Vitturi N, Marchi G, Carraro G. Dapaglifozin on albuminuria in chronic kidney disease patients with Fabry disease: the DEFY study design and protocol. J Clin Med 2023;12:3689.
45. Romagnani P, Lasagni L, Remuzzi G. Renal progenitors: an evolutionary conserved strategy for kidney regeneration. Nat Rev Nephrol 2013;9:137-46.
46. Huang B, Zeng Z, Kim S, et al. Long-term expandable mouse and human-induced nephron progenitor cells enable kidney organoid maturation and modeling of plasticity and disease. Cell Stem Cell 2024;31:921-39.e17.
47. Lasagni L, Angelotti ML, Ronconi E, et al. Podocyte regeneration driven by renal progenitors determines glomerular disease remission and can be pharmacologically enhanced. Stem Cell Reports 2015;5:248-63.
Cite This Article
How to Cite
Download Citation
Export Citation File:
Type of Import
Tips on Downloading Citation
Citation Manager File Format
Type of Import
Direct Import: When the Direct Import option is selected (the default state), a dialogue box will give you the option to Save or Open the downloaded citation data. Choosing Open will either launch your citation manager or give you a choice of applications with which to use the metadata. The Save option saves the file locally for later use.
Indirect Import: When the Indirect Import option is selected, the metadata is displayed and may be copied and pasted as needed.
About This Article
Special Issue
Copyright
Data & Comments
Data
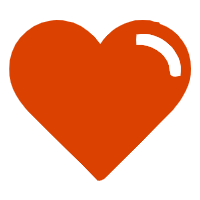
Comments
Comments must be written in English. Spam, offensive content, impersonation, and private information will not be permitted. If any comment is reported and identified as inappropriate content by OAE staff, the comment will be removed without notice. If you have any queries or need any help, please contact us at [email protected].