Transformation products of antibiotics: overlooked drivers for enhancing the environmental spread of antibiotic resistance
Abstract
Humans have released thousands of antibiotics into the environment, which is a primary driver of the global dissemination of bacterial resistance. Parent compounds can generate various transformation products (TPs), many of which remain unidentified and lack comprehensive microbial risk assessments. The TPs formed during the process may exhibit structural similarities to the parent compounds, and they can induce antibiotic-like resistance transmission despite lacking the bactericidal/antibacterial properties of the parent compounds. However, assessments of antimicrobial resistance hazards predominantly emphasize parent compounds while largely neglecting their TPs. Here, we highlight that TPs warrant greater attention regarding chemical structure identification and the risk of resistance transmission. This perspective summarizes TPs’ potential, mechanisms, and challenges in triggering the risk of resistance transmission.
Keywords
INTRODUCTION
Driven by population growth and the rising demand for veterinary drugs, global antibiotic consumption has been steadily increasing[1]. The global annual consumption of antibiotics is estimated to be between 100,000 and 200,000 tons[2]. However, due to poor intestinal absorption or incomplete metabolism, many antibiotics are excreted in their unchanged form or produce some potentially toxic transformation products (TPs) that ultimately enter the environment[3,4]. Environmental residues of TPs may exhibit antibiotic-like effects, such as inhibiting the growth and activity of microorganisms, exerting toxic effects on plants and animals, and presenting a potential risk to ecosystem stability[5-7]. Furthermore, TPs can also disseminate antibiotic resistance genes (ARGs) through environmental media such as water, soil, and air, as well as within plants and animals, thus posing a significant exposure risk and human health threat[8]. The identification of the resistance transmission risk posed by TPs remains inadequate. This is primarily due to the fact that research on drug resistance has predominantly centered on parent antibiotics. Additionally, TPs’ complex and heterogeneous nature introduces considerable uncertainty when assessing their risks.
EFFECTS AND MOLECULAR MECHANISMS OF ANTIBIOTIC-INDUCED RESISTANCE TRANSMISSION
Antibiotics are widely used in medicine, agriculture, animal husbandry, etc. However, their extensive use is a major driver of antibiotic resistance and also causes exposure risks to humans[9]. Antibiotic resistance can arise either through the induction of gene mutations or via vertical gene transfer and horizontal gene transfer (HGT). Antibiotics can induce the overproduction of reactive oxygen species (ROS) in bacterial cells, damaging DNA. Errors during the repair process may introduce mutations that enable bacteria to acquire antibiotic resistance[10]. In particular, HGT can make bacterial resistance transfer between different strains or even between different communities, mainly including four ways: conjugation, transformation, transduction, and vesiduction [Figure 1][11]. Conjugation is through direct contact between bacteria; resistant bacteria pass ARGs to other bacteria through the plasmid, which is the HGT process with the highest frequency[12]. For example, tetracycline at subinhibitory concentrations (e.g., 3.9-250.0 ng/mL) promoted HGT frequency by 1.47- to 3.19-fold[13]. In addition, bacteria can promote antibiotic resistance transmission through antibiotic target changes, efflux pump enhancement, enzyme degradation processes, and metabolic pathway changes[14]. Pu et al. found that enhanced efflux pump activity, especially multi-drug efflux genes TolC, can promote drug tolerance in dormant bacterial cells[15]. Modifications in metabolic pathways allow bacteria to circumvent the mechanisms of antibiotic action and sustain their growth[16]. The alterations in these biological processes increase the risk of resistance transfer mainly indirectly. Since antibiotic TPs may exhibit characteristics of both parent compounds and other substances, the underlying mechanisms of the risk of transmission of resistance are complex and deserve comprehensive consideration.
MIGRATION AND TRANSFORMATION OF ANTIBIOTICS IN THE ENVIRONMENT
The primary sources of antibiotics in the environment include industrial wastewater, medical wastewater, livestock farming, and aquaculture[17]. However, the existing treatment processes cannot eliminate antibiotics, resulting in the presence of antibiotic residues. Antibiotics are redistributed across environmental media through various biochemical processes, including adsorption, migration, and degradation, posing exposure risks to humans[18]. Adsorption is a key process in the interaction of antibiotics with various environmental media, directly influencing their migration/degradation[19]. Sulfonamides remain at high levels due to electrostatic repulsion with negatively charged sediments, potentially becoming the source of groundwater contamination[20]. Additionally, antibiotics undergo various natural degradation reactions, such as abiotic degradation (hydrolysis and photolysis) and microbial degradation[21]. For example, β-lactam, amphenicol, and macrolide antibiotics are soluble in water and prone to hydrolyzed[22]. In addition to hydrolysis, most antibiotics can also undergo photolysis in surface water and on soil surfaces. Direct photodegradation refers to the breakdown of antibiotic molecules through electronic transitions induced by ultraviolet radiation[23]. Research showed that sulfamethoxazole and sulfadiazine exhibited good photodegradation effects in ultrapure water, with half-lives of 6.76 and 0.86 h, respectively[24]. Indirect photodegradation involves various natural photosensitizers in water bodies (such as humus, algal organic matter, etc.), which convert light energy into ROS and promote the degradation of antibiotics[20]. The efficiency of this process is influenced by the types and concentrations of photosensitizers, while different photosensitizers can promote or inhibit the degradation of antibiotics. Furthermore, the biodegradation of antibiotics plays an important role in aquatic environments, mainly relying on the activities of coexisting microorganisms. In the activated sludge, microorganisms can break down antibiotics into smaller molecules by enzymatic catalysis, such as N-oxidation and hydrolysis of macrolide rings[25]. The migration and transformation of antibiotics are also influenced by environmental factors such as water temperature, pH, light intensity, and water mobility[26]. For example, influenced by seasonal river flow, a significant increase in summer runoff results in a faster photolysis rate and lower antibiotic residues than in winter[27]. The classification of antibiotic TPs is determined by the specific transformation processes of their parent compounds, and environmental factors and water treatment processes significantly increase the complexity of the conversion process.
THE CONTRIBUTION OF OVERLOOKED ANTIBIOTIC TPS TO ANTIBIOTIC RESISTANCE DISSEMINATION
TPs are increasingly detected in various environmental media, forming through both natural processes and anthropogenic activities like wastewater treatment. They may accumulate over time, leading to long-term environmental exposure and posing significant risks of ecotoxicity and resistance gene induction
Main antibiotic TPs’ structural features, environmental concentration, transfer pathway, and resistance mechanism
Antibiotic class | TP name | Structural features | Environmental concentration | Transfer pathway | Mechanism of resistance |
Sulfonamides | N4-acetyl sulfamethoxazole | N-acetylation![]() | Surface water: ng/L~μg/L[29] Sediment: μg/kg[30] | Micro-biological degradation[30], chemical oxidation degradation[31] | Promote conjugation, enhance ROS, cell membrane permeability, secretion system, etc.[32] |
4-nitro sulfamethoxazole | Nitro substitution on the benzene ring![]() | WWTP effluent: ng/L~μg/L[31,33] | |||
Quinolones | CIP N-oxide | Oxidation of the piperazine ring![]() | Soil: μg/kg[34] Surface water: μg/L~mg/L[35] | Photocatalytic degradation[36], micro-biological degradation[37] | Enhance resistant mutations, random errors during DNA replication, activate SOS response, and increase intracellular ROS[38] |
Decarboxy ofloxacin | Decarboxylation of carboxylic group![]() | River sediment: μg/kg[39] | |||
Macrolides | Clathromycin | Hydroxylation at C14![]() | Surface water: ng/L~μg/L[40] Pharmaceutical wastewater: mg/L[41] | Photochemical degradation[42], adsorption and migration[43], micro-biological degradation[44] | Change the target of macrolide (i.e., ribosome) and upregulation of efflux pump[45] |
Tetracyclines | 4-epi-tetracycline | Epimerization at C4![]() | Livestock manure: mg/kg[46] | Micro-biological degradation[47], chemical oxidation degradation[48] | Enhance resistant mutations, enhance target protection, metabolic adaptations, and reduce membrane permeability[49] |
Anhydrotetracycline | Dehydration of C6 hydroxyl![]() | Compost product: μg/kg[46] |
Many studies have been conducted to investigate the ecotoxicity of antibiotic conversion products. Some of the TPs may have stronger toxic activity than the original antibiotics. For example, the accumulation of certain TPs (such as chlorinated or brominated products of sulfamethoxazole) is prone to bioaccumulation in aquatic environments due to poor hydrophilicity, resulting in death or stunted growth of aquatic species, thus disrupting the food chain[50]. Zhang et al. found that after biodegradation in the wastewater system, the TPs of ciprofloxacin (CIP) retain partial toxicity and antibacterial activity. Notably, TPs generated through decarboxylation, hydroxylation, and cleavage of the piperidinyl substituent exhibit higher toxicity compared to the parent CIP[51]. However, there are few studies on the transmission of TPs-related resistance genes, with most research focusing only on the induction of ARGs and their bactericidal/antibacterial effects, and even few studies on transmission, such as HGT, and it is easy to ignore the overall effect of maternal compounds and TPs. Fluoroquinolone degradation products can bind to DNA gyrase, interfere with DNA replication, and stimulate resistant mutations in E. coli[38]. Degradation products like tetracycline can enhance the expression of oxidative stress gene lexA, promote the production of ROS, and the membrane-associated efflux pump protein encoding otrC to induce bacterial resistance genes[52]. Changes in bacterial metabolism caused by TPs are consistent with the previously discussed mechanism that indirectly causes the spread of resistance. However, this aspect has not been extensively explored in the current study, and the risk of HGT is often ignored. Furthermore, in our investigation of sulfamethoxazole TPs, we observed that although these TPs do not retain the bactericidal properties of the parent compound, they significantly enhance conjugative HGT by over 70-fold. This enhancement is primarily driven by the induction of ROS, increased cell membrane permeability, and upregulation of bacterial secretion systems[32]. Due to the wide variety of TPs, the current lack of uniform standards for the characterization and management of TPs, and the research preference of researchers focusing on TPs toxicity, research on the risks associated with TPs-mediated resistance transmission remains limited. Future studies should highlight the influence of TPs on the development of bacterial resistance, especially in the spread of AGRs, and how to better use theoretical approaches to predict the toxicity and resistance of TPs. An in-depth study and comprehensive evaluation of the induction and transmission ability of TPs-related resistance genes, along with strengthening the identification and management of TPs, is an important task to reduce the environmental problems of antibiotics.
PERSPECTIVES
The existing literature has commonly suggested the worldwide occurrence of antibiotic TPs in multiple environmental matrices, while their ecological risks have been largely documented. In particular, most TPs possess molecular structures similar to the parent antibiotics and their modes of action may be similar in inducing antibiotic resistance. While standardized protocols for systematic TP identification are lacking, recent analytical advancements (e.g., non-targeted screening, high-resolution mass spectrometry, and stable isotope analysis) have enabled pragmatic identification of TPs and their ecological risks[53,54]. To better determine its molecular structure, and identify or predict its toxicity and drug resistance, it is essential to effectively integrate TPs into chemical management frameworks. This can be achieved by enhancing the identification of TPs’ structures and broadening the discussion on resistance transmission mechanisms. Several key topics warrant focused attention following:
1. The development of non-targeted analysis, high-resolution mass spectrometry, and stable isotope analysis is conducive to effectively predicting the potential molecular formula of TPs and even determining their chemical structure.
2. Integrating diverse antibiotic TPs into the chemical management framework is needed. Leveraging the accumulation of big data, machine learning technologies can ultimately be utilized in the toxicity assessment and prediction tools for TPs. How to better use theoretical methods to predict toxicity and resistance of TPs becomes the future direction.
3. Attention should be given to the risk of resistance transmission induced by the antibiotic-like effect, as TPs frequently lose their parent compounds’ bactericidal or bacteriostatic efficacy against bacteria while introducing additional toxic risks.
4. Laboratory-oriented strategies for mitigating resistance risks from TPs: Future research should focus on developing selective advanced oxidation processes (e.g., solar/chlorine and chlorination/bromination treatment[55,56]) and functional nanostructured adsorbents [e.g., metal-organic framework (MOFs)] for selective TP capture. Integrated technologies synergistically suppress HGT in multi-pollutant matrices, weakening environmental persistence and resistance-driven ecological threats.
DECLARATIONS
Authors’ contributions
Methodology, investigation, writing - original draft, writing - review and editing: Zhang, Y.
Methodology, investigation, writing - original draft: Jiang, J.
Conceptualization, methodology, investigation, writing - original draft, writing - review and editing, project administration, funding acquisition: Feng, M.; Ye, C.
Availability of data and materials
Not applicable.
Financial support and sponsorship
This research was supported by the National Key R&D Program of China (2023YFE0112100), the China Postdoctoral Science Foundation (2023M732947), and the Postdoctoral Fellowship Program of CPSF (GZC20231409).
Conflicts of interest
All authors declared that there are no conflicts of interest.
Ethical approval and consent to participate
Not applicable.
Consent for publication
Not applicable.
Copyright
© The Author(s) 2025.
REFERENCES
1. Klein, E. Y.; Van, B. T. P.; Martinez, E. M.; et al. Global increase and geographic convergence in antibiotic consumption between 2000 and 2015. Proc. Natl. Acad. Sci. U. S. A. 2018, 115, E3463-70.
2. Zhu, Y. G.; Johnson, T. A.; Su, J. Q.; et al. Diverse and abundant antibiotic resistance genes in Chinese swine farms. Proc. Natl. Acad. Sci. U. S. A. 2013, 110, 3435-40.
3. Sarmah, A. K.; Meyer, M. T.; Boxall, A. B. A global perspective on the use, sales, exposure pathways, occurrence, fate and effects of veterinary antibiotics (VAs) in the environment. Chemosphere 2006, 65, 725-59.
4. Li, S.; Zhu, Y.; Zhong, G.; Huang, Y.; Jones, K. C. Comprehensive assessment of environmental emissions, fate, and risks of veterinary antibiotics in China: an environmental fate modeling approach. Environ. Sci. Technol. 2024, 58, 5534-47.
5. Du, J.; Liu, Q.; Pan, Y.; Xu, S.; Li, H.; Tang, J. The research status, potential hazards and toxicological mechanisms of fluoroquinolone antibiotics in the environment. Antibiotics 2023, 12, 1058.
6. Li, W.; Liu, K.; Min, Z.; et al. Transformation of macrolide antibiotics during chlorination process: kinetics, degradation products, and comprehensive toxicity evaluation. Sci. Total. Environ. 2023, 858, 159800.
7. Hu, J.; Lyu, Y.; Liu, Y.; You, X.; Helbling, D. E.; Sun, W. Incorporating transformation products for an integrated assessment of antibiotic pollution and risks in surface water. Environ. Sci. Technol. 2025, 59, 2815-26.
8. Aminzare, M.; Mohammadi, M.; Hashemi, M.; et al. Residual antibiotics as an alarming health threat for human; a systematic study and meta-analysis in Iranian animal food products. J. Agr. Food. Res. 2024, 18, 101435.
9. Clark, M. D.; Halford, Z.; Herndon, C.; Middendorf, E. Evaluation of antibiotic initiation tools in end-of-life care. Am. J. Hosp. Palliat. Care. 2022, 39, 274-81.
10. Zhao, Q.; Guo, W.; Luo, H.; et al. Deciphering the transfers of antibiotic resistance genes under antibiotic exposure conditions: driven by functional modules and bacterial community. Water. Res. 2021, 205, 117672.
11. Acar Kirit, H.; Bollback, J. P.; Lagator, M. The role of the environment in horizontal gene transfer. Mol. Biol. Evol. 2022, 39, msac220.
12. Chi, W.; Zou, Y.; Qiu, T.; et al. Horizontal gene transfer plays a crucial role in the development of antibiotic resistance in an antibiotic-free shrimp farming system. J. Hazard. Mater. 2024, 476, 135150.
13. Zhou, H.; Lu, Z.; Liu, X.; et al. Environmentally relevant concentrations of tetracycline promote horizontal transfer of antimicrobial resistance genes via plasmid-mediated conjugation. Foods 2024, 13, 1787.
14. Goodarzi, Z.; Asad, S.; Mehrshad, M. Genome-resolved insight into the reservoir of antibiotic resistance genes in aquatic microbial community. Sci. Rep. 2022, 12, 21047.
15. Pu, Y.; Zhao, Z.; Li, Y.; et al. Enhanced efflux activity facilitates drug tolerance in dormant bacterial cells. Mol. Cell. 2016, 62, 284-94.
16. He, M.; Shi, J.; Liu, A.; Xu, Y. J.; Liu, Y. Antibiotic-induced gut microbiota dysbiosis altered host metabolism. Mol. Omics. 2023, 19, 330-9.
17. Zainab, S. M.; Junaid, M.; Xu, N.; Malik, R. N. Antibiotics and antibiotic resistant genes (ARGs) in groundwater: a global review on dissemination, sources, interactions, environmental and human health risks. Water. Res. 2020, 187, 116455.
18. Huang, S.; Yu, J.; Li, C.; et al. The effect review of various biological, physical and chemical methods on the removal of antibiotics. Water 2022, 14, 3138.
19. Zhang, T.; Yan, R.; Gui, Q.; Gao, Y.; Wang, Q.; Xu, S. Fine particulate matter as a key factor promoting the spread of antibiotics in river network. Sci. Total. Environ. 2024, 935, 173323.
20. Liu, X.; Lv, K.; Deng, C.; Yu, Z.; Shi, J.; Johnson, A. C. Persistence and migration of tetracycline, sulfonamide, fluoroquinolone, and macrolide antibiotics in streams using a simulated hydrodynamic system. Environ. Pollut. 2019, 252, 1532-8.
21. Bueno, I.; He, H.; Kinsley, A. C.; et al. Biodegradation, photolysis, and sorption of antibiotics in aquatic environments: a scoping review. Sci. Total. Environ. 2023, 897, 165301.
22. Mitchell, S. M.; Ullman, J. L.; Teel, A. L.; Watts, R. J. Hydrolysis of amphenicol and macrolide antibiotics: chloramphenicol, florfenicol, spiramycin, and tylosin. Chemosphere 2015, 134, 504-11.
23. Tyutereva, Y. E.; Snytnikova, O. A.; Sheven, D. G.; et al. Mechanism of UV photodegradation of fluoroquinolone antibiotic ciprofloxacin in aqueous solutions. Chemosphere 2024, 367, 143643.
24. Loureiro Dos Louros, V.; Silva, C. P.; Nadais, H.; Otero, M.; Esteves, V. I.; Lima, D. L. D. Photodegradation of sulfadiazine in different aquatic environments - evaluation of influencing factors. Environ. Res. 2020, 188, 109730.
25. Terzic, S.; Udikovic-Kolic, N.; Jurina, T.; et al. Biotransformation of macrolide antibiotics using enriched activated sludge culture: kinetics, transformation routes and ecotoxicological evaluation. J. Hazard. Mater. 2018, 349, 143-52.
26. Wang, L.; Wang, Y.; Wang, Y.; Wang, H.; Wu, H.; Gao, D. Efficient photocatalytic degradation of sulfonamides in wastewater using g-C3N4 heterostructures: a critical review. Environ. Technol. Innov. 2024, 36, 103854.
27. Luo, Y.; Xu, L.; Rysz, M.; Wang, Y.; Zhang, H.; Alvarez, P. J. Occurrence and transport of tetracycline, sulfonamide, quinolone, and macrolide antibiotics in the Haihe River Basin, China. Environ. Sci. Technol. 2011, 45, 1827-33.
28. Löffler, P.; Escher, B. I.; Baduel, C.; Virta, M. P.; Lai, F. Y. Antimicrobial transformation products in the aquatic environment: global occurrence, ecotoxicological risks, and potential of antibiotic resistance. Environ. Sci. Technol. 2023, 57, 9474-94.
29. Archundia, D.; Duwig, C.; Lehembre, F.; et al. Antibiotic pollution in the Katari subcatchment of the Titicaca Lake: major transformation products and occurrence of resistance genes. Sci. Total. Environ. 2017, 576, 671-82.
30. Chen, J.; Ke, Y.; Zhu, Y.; Chen, X.; Xie, S. Deciphering of sulfonamide biodegradation mechanism in wetland sediments: from microbial community and individual populations to pathway and functional genes. Water. Res. 2023, 240, 120132.
31. Hu, J.; Li, X.; Liu, F.; Fu, W.; Lin, L.; Li, B. Comparison of chemical and biological degradation of sulfonamides: solving the mystery of sulfonamide transformation. J. Hazard. Mater. 2022, 424, 127661.
32. Zhang, Y.; Rosado-García, F. M.; Peña, Y. P.; et al. Sulfonamide metabolites enhance resistance transmission via conjugative transfer pathways. J. Hazard. Mater. 2025, 491, 137932.
33. Xiao, Y.; Zhang, Q.; Yang, Y.; et al. Unraveling the pollution and discharge of aminophenyl sulfone compounds, sulfonamide antibiotics, and their acetylation products in municipal wastewater treatment plants. Environ. Sci. Technol. 2024, 58, 11695-706.
34. Han, J.; Xu, Y.; Xu, D.; et al. Mechanism of downward migration of quinolone antibiotics in antibiotics polluted natural soil replenishment water and its effect on soil microorganisms. Environ. Res. 2023, 218, 115032.
35. Tang, T.; Qian, K.; Shi, T.; et al. Photodegradation of quinestrol in waters and the transformation products by UV irradiation. Chemosphere 2012, 89, 1419-25.
36. Ma, B.; Zha, Y.; Yu, P.; et al. Hydrothermal nanoarchitectonics of Z-scheme g-C3N4/Bi2WO6 heterojunction with enhanced adsorption and photocatalytic activity for fluoroquinolone antibiotics removal: kinetics, mechanism and degradation pathway. J. Alloys. Compd. 2023, 952, 170061.
37. Jia, Y.; Khanal, S. K.; Shu, H.; Zhang, H.; Chen, G. H.; Lu, H. Ciprofloxacin degradation in anaerobic sulfate-reducing bacteria (SRB) sludge system: mechanism and pathways. Water. Res. 2018, 136, 64-74.
38. Wang, D.; Ning, Q.; Dong, J.; Brooks, B. W.; You, J. Predicting mixture toxicity and antibiotic resistance of fluoroquinolones and their photodegradation products in Escherichia coli. Environ. Pollut. 2020, 262, 114275.
39. Hu, J.; Lyu, Y.; Chen, H.; Li, S.; Sun, W. Suspect and nontarget screening reveal the underestimated risks of antibiotic transformation products in wastewater treatment plant effluents. Environ. Sci. Technol. 2023, 57, 17439-51.
40. Senta, I.; Kostanjevecki, P.; Krizman-Matasic, I.; Terzic, S.; Ahel, M. Occurrence and behavior of macrolide antibiotics in municipal wastewater treatment: possible importance of metabolites, synthesis byproducts, and transformation products. Environ. Sci. Technol. 2019, 53, 7463-72.
41. Senta, I.; Krizman-Matasic, I.; Terzic, S.; Ahel, M. Comprehensive determination of macrolide antibiotics, their synthesis intermediates and transformation products in wastewater effluents and ambient waters by liquid chromatography-tandem mass spectrometry. J. Chromatogr. A. 2017, 1509, 60-8.
42. Ge, L.; Cui, N.; Halsall, C.; Yang, Y.; Cao, S.; Zhang, P. Exploring the aqueous photodegradation of three ionisable macrolide antibiotics: kinetics, intermediates and photoinduced toxicity. J. Water. Process. Eng. 2024, 62, 105383.
43. Deng, X.; Jiang, Y.; Zhang, M.; Nan, Z.; Liang, X.; Wang, G. Sorption properties and mechanisms of erythromycin and ampicillin in loess soil: Roles of pH, ionic strength, and temperature. Chem. Eng. J. 2022, 434, 134694.
44. Yan, S.; Ding, N.; Yao, X.; et al. Effects of erythromycin and roxithromycin on river periphyton: structure, functions and metabolic pathways. Chemosphere 2023, 316, 137793.
45. do Rêgo, R. L.; Neves, F. P. G.; Miranda, F. M.; et al. CRISPR elements and their association with macrolide and aminoglycoside resistance genes in enterococci. Microb. Drug. Resist. 2025, 31, 75-9.
46. Wu, D.; Dai, S.; Feng, H.; Karunaratne, S. H. P. P.; Yang, M.; Zhang, Y. Persistence and potential risks of tetracyclines and their transformation products in two typical different animal manure composting treatments. Environ. Pollut. 2024, 341, 122904.
47. Li, C.; Awasthi, M. K.; Liu, J.; Yao, T. Veterinary tetracycline residues: environmental occurrence, ecotoxicity, and degradation mechanism. Environ. Res. 2025, 266, 120417.
48. Zhi, D.; Wang, J.; Zhou, Y.; et al. Development of ozonation and reactive electrochemical membrane coupled process: enhanced tetracycline mineralization and toxicity reduction. Chem. Eng. J. 2020, 383, 123149.
49. Guo, Z.; Zhang, T.; Yang, H.; et al. Unraveling tetracycline and its degradation product: Induction mechanisms of antibiotic resistance in Escherichia coli. Sci. Total. Environ. 2025, 970, 178959.
50. Yang, Y.; Lu, X.; Jiang, J.; et al. Degradation of sulfamethoxazole by UV, UV/H2O2 and UV/persulfate (PDS): formation of oxidation products and effect of bicarbonate. Water. Res. 2017, 118, 196-207.
51. Zhang, H.; Quan, H.; Yin, S.; Sun, L.; Lu, H. Unraveling the toxicity associated with ciprofloxacin biodegradation in biological wastewater treatment. Environ. Sci. Technol. 2022, 56, 15941-52.
52. Zheng, H.; Ji, Y.; Li, S.; Li, W.; Ma, J.; Niu, J. Ecotoxicity and resistance genes induction changing of antibiotic tetracycline degradation products dominated by differential free radicals. Environ. Res. 2023, 227, 115427.
53. Liu, X.; Köpke, J.; Akay, C.; Kümmel, S.; Imfeld, G. Sulfamethoxazole transformation by heat-activated persulfate: linking transformation products patterns with carbon and nitrogen isotope fractionation. Environ. Sci. Technol. 2025, 59, 5704-14.
54. Hofstetter, T. B.; Bakkour, R.; Buchner, D.; et al. Perspectives of compound-specific isotope analysis of organic contaminants for assessing environmental fate and managing chemical pollution. Nat. Water. 2024, 2, 14-30.
55. Xie, Y.; Chen, W.; Ye, Z.; Yan, J.; Yu, X.; Feng, M. Mechanistic insight into the environmental fate of highly concerned transformation products of aqueous micropollutants during the solar/chlorine treatment. Water. Res. 2025, 278, 123413.
Cite This Article

How to Cite
Download Citation
Export Citation File:
Type of Import
Tips on Downloading Citation
Citation Manager File Format
Type of Import
Direct Import: When the Direct Import option is selected (the default state), a dialogue box will give you the option to Save or Open the downloaded citation data. Choosing Open will either launch your citation manager or give you a choice of applications with which to use the metadata. The Save option saves the file locally for later use.
Indirect Import: When the Indirect Import option is selected, the metadata is displayed and may be copied and pasted as needed.
About This Article
Copyright
Data & Comments
Data
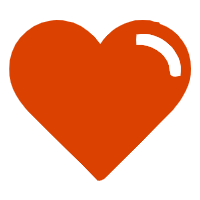
Comments
Comments must be written in English. Spam, offensive content, impersonation, and private information will not be permitted. If any comment is reported and identified as inappropriate content by OAE staff, the comment will be removed without notice. If you have any queries or need any help, please contact us at [email protected].