Polybrominated diphenyl ethers and Dechlorane Plus in soil from four different industry parks: identification, emission characteristics, spatial distribution, and health risk assessment
Abstract
This study investigated the surface soil of four typical industrial parks involved in crude oil extraction, petroleum refining, and downstream petrochemical manufacturing processes to clarify the emission patterns of polybrominated diphenyl ethers (PBDEs) and Dechlorane Plus (DP) in various industrial areas, and reveal their impact on the surrounding environment. The concentrations of PBDEs and DPs in soil from a flame retardant manufacturing park were 4.13 × 103 and 1.74 × 103 ng/g, respectively, far exceeding those in three petrochemical parks. Among the 20 PBDE congeners analyzed, BDE209, a Deca-BDE congener, consistently exhibited the highest concentrations across all four sites. However, the relative compositions of syn-DP and anti-DP differed between the flame retardant manufacturing park and the petrochemical parks: the fanti at the flame retardant manufacturing park was significantly lower than those in the three petrochemical parks. The horizontal spatial distributions of PBDEs and DPs revealed the presence of point source emissions and demonstrated that chemical emissions from the parks influenced surrounding areas. A prolonged emission history contributed to the cumulative concentrations and distinctive composition profiles of these chemicals in soil. Dermal contact-based non-carcinogenic risk assessments indicated that the hazard indices for PBDEs in soil were below 1, suggesting an acceptable health risk. Among PBDE congeners, Tri-BDEs contributed most significantly to non-carcinogenic risks, despite BDE209 being the most abundant. Non-carcinogenic risks associated with DPs were negligible across all four parks.
Keywords
INTRODUCTION
Brominated flame retardants (BFRs) are compounds that directly bromine organic molecules or add bromine to olefins to achieve flame retardancy. BFRs are classified into three subgroups based on their mode of incorporation into polymers: additive and reactive BFRs, and brominated monomers[1]. Polybrominated diphenyl ethers (PBDEs), the most widely used BFRs by volume and a predominant additive type, have attracted significant scientific attention. These chemicals have been widely used in a variety of consumer products, such as electronics, furniture, textiles, and plastics, due to their flame-retardant properties[2]. They have 209 congeners based on the number and position of bromine atom substitutions, and there are three commercial products, i.e., Penta-BDEs, Octa-BDEs, and Deca-BDE[3].
As a kind of additive flame retardant, PBDEs are not chemically bonded with the host materials, and consequently, they have a propensity to be released into the environment during manufacturing, use, and disposal[4-7]. As a typical persistent organic pollutant, PBDEs are persistent in the environment matrices, such as air, dust, water, soil, and sediment[8-10] and can bioaccumulate in organisms, including humans[6,11]. Numerous studies have shown that PBDEs can negatively impact the endocrine system, neurodevelopment, reproductive health, and immune function[12,13]. Consequently, PBDEs have been formally listed as priority-controlled substances under the Stockholm Convention on Persistent Organic Pollutants, prompting the implementation of comprehensive regulatory restrictions on their production and use across major global jurisdictions, including the European Union, the United States, and China[14]. China has completely banned the production, processing, use, and import/export of PBDEs as of December 31, 2023. Notwithstanding these control measures, the legacy of widespread industrial deployment preceding regulatory phase-out necessitates ongoing quantitative characterization of PBDE reservoirs in soil, particularly given their demonstrated soil persistence.
Dechlorane Plus (DP), consisting of syn-DP and anti-DP, was developed as a replacement for mirex and has been in use since the 1960s[15]. Later, DP emerged as a new class of halogenated flame retardant that was mass-produced and widely employed in products such as computer connectors, cable coatings, and electrical wires for fire prevention, owing to its low density, low cost, and excellent photochemical and thermal stability[16-18]. Similar to PBDEs, DP is environmentally persistent and can bioaccumulate in organisms[19]. As its usage expanded, DP has been frequently detected in the air, soil, water, plants, animals, and even human tissues[20-23]. Recent studies have shown that DP may act as a potential endocrine disruptor, exhibiting antagonistic activity against thyroid hormone receptors (TRs) in cells and possibly disrupting thyroid function by binding to TRs and acting as an antagonist[24,25]. Currently, DP is listed in Annex A (Elimination) of the Stockholm Convention, requiring parties to eliminate its production and use.
However, it should be noted that prohibiting PBDEs and DPs will only have positive effects on newly manufactured products. Older or currently used products containing these chemicals may still release them into the environment. Therefore, PBDEs and DPs remain among the halogenated flame retardants that require particular attention. Industrial emissions are major sources of environmental pollution, and certain activities - such as flame retardant production, electronic waste dismantling, and operations within petrochemical industrial parks - warrant special scrutiny. E-waste dismantling plants, recognized as primary sources of flame retardant emissions, have been extensively documented for their high levels of release[26-28]. Additionally, during production processes within petrochemical industrial parks, large amounts of flame retardants are used[1,29], contributing further to environmental contamination. As potential emission sources, these facilities release flame retardants that persist in the surrounding environment and may pose health risks to nearby residents. Human exposure to flame retardant chemicals occurs mainly through three vectors: the ambient domestic environment, foodborne particulate matter, and direct dermal contact with contaminated consumer goods. Pediatric populations exhibit heightened vulnerability due to distinct behavioral patterns and physiological susceptibilities, demonstrating significantly elevated transdermal pollutant uptake rates compared to adults. This differential exposure poses substantial risks to the development of critical organ systems during sensitive growth periods.
However, information on the levels and compositional patterns of PBDEs and DPs in petrochemical industrial parks and surrounding areas remains very limited. In particular, no data are available on whether emission characteristics of these chemicals differ across various sectors of the petrochemical industry, including upstream (oil extraction), midstream (petroleum refining), and downstream (chemical synthesis) activities. The present study hypothesized that the emission characteristics of PBDEs and DPs vary between different regions within the flame retardant manufacturing sector and across the petrochemical industry chain from upstream to downstream. Therefore, the present study aimed to: (1) identify the target substances in three types of petrochemical industries and compare them with those found in a flame retardant manufacturing park; (2) investigate the chemical composition profiles in soils from the four parks; (3) reveal the spatial distribution patterns and the impact on surrounding areas; and (4) assess human health risks from chemical exposure through oral ingestion and dermal contact. This study addresses a gap in the research on flame retardant emissions from petrochemical enterprises and provides important information for the management and control of these target chemicals in both petrochemical industries and flame retardant manufacturing areas.
MATERIALS AND METHODS
Reagents and materials
Empty solid-phase extraction (SPE) cartridges were purchased from CNW Technologies GmbH (Berlin, Germany). PBDE standards, comprising twenty congeners (BDE17, 28, 47, 66, 71, 85, 99, 100, 138,153, 154, 183, 190, 196, 197, 203, 206, 207, 208, and 209) were obtained from AccuStandard (New Haven, CT, USA). DPs (syn-DP and anti-DP) were also purchased AccuStandard. The recovery standard 13C-PCB141 and the internal standard 13C-PCB208 were supplied by the same company. High-performance liquid chromatography-grade solvents, including ethyl acetate, dichloromethane, n-hexane, and iso-octane, were obtained from CNW (Düsseldorf, Germany).
Study areas and sample collection
Soil samples were collected from various locations across China using the grid point method[30], including three petrochemical industries (upstream, midstream, and downstream sectors) and a flame retardant production park. The samples were taken from Caofeidian District, Tangshan, Hebei Province, in October 2022. A total of 65 soil samples were collected from various functional zones, including oil extraction sites, a steel plant, chemical industrial parks, and residential areas. In July 2023, 50 soil samples were collected from the areas surrounding a petrochemical park in Jieyang, Guangdong Province, which includes schools, residential areas, and salt flats. This petrochemical park mainly focuses on petroleum refining. In May 2021, 16 samples were collected from a petrochemical park in Lanzhou, Gansu Province, with an additional 26 samples from the surrounding area. Two nearby residential areas were also included in the sampling. Additionally, in December 2020, 40 soil samples were collected from a flame retardant manufacturing park in Weifang, Shandong Province, including production areas (n = 15) and an adjacent office building (n = 1), as well as the surrounding area (n = 24). In total, 197 samples were obtained, with the sampling site details provided in Supplementary Tables 1-4. Each sample was collected using a five-point sampling method, transported to the laboratory in an icebox, and lyophilized. The resulting powders were sieved and stored in brown vials at -20 °C until analysis.
Sample pretreatment protocols
Each soil sample (0.5 g) was extracted with a 1:2:2 (v/v) ethyl acetate/dichloromethane/n-hexane mixture after spiking with 13C-PCB141 surrogate standards. The extraction procedure involved 10 min of ultrasonication, followed by 1 min of vortex mixing and centrifugation at 2,500 rpm for 10 min. This extraction process was repeated three times. The combined extracts were concentrated using rotary evaporation and then loaded onto a Florisil SPE cartridge (1 g) that had been pre-conditioned sequentially with ethyl acetate, dichloromethane, and n-hexane. Target compounds (PBDEs and DPs) were then eluted with 10 mL of a 1:1 (v/v) ethyl acetate/dichloromethane mixture. Finally, the eluate was concentrated, reconstituted in 50 μL of isooctane containing the internal standard 13C-PCB208, and stored at -20 °C until instrumental analysis.
Instrumental analysis
PBDEs and DPs were determined by a 7890B gas chromatograph coupled with a 5977B mass spectrometer (Agilent, USA). Chemical separation was accomplished with a DB-5 HT capillary column (15 m × 0.25 mm × 0.1 μm, Agilent, USA). The oven temperature program began at 80 °C (hold time: 0.5 min), then increased at a rate of 20 °C/min to 310 °C, followed by a final isothermal hold for 3 min. A 1 μL sample injection was made, with the injector maintained at 280 °C. High-purity helium was used as the carrier gas, delivered at a constant flow rate of 1.0 mL/min. The analysis was performed in negative chemical ionization (NCI) mode using selected ion monitoring (SIM), with the monitored ions listed in Supplementary Table 5.
Quality assurance and quality control
To ensure data quality, each analytical batch included ten soil samples, one matrix-spiked sample, and one procedural blank. The standard deviation was confirmed to be < 10%. Quality control was conducted by analyzing matrix-spiked samples containing known concentrations of target standards, with all reported concentrations being recovery-corrected. Method validation demonstrated excellent linearity for PBDEs and DPs (R2 > 0.99) and satisfactory matrix spike recoveries (71%-125%). The limits of detection (LOD) and quantification (LOQ), defined as 3× and 10× the signal-to-noise ratio, respectively, are provided in Supplementary Table 5.
Health risk assessment by dermal contact
To evaluate non-carcinogenic risks, hazard quotients (HQs) were calculated using the reference dose (RfD) and the estimated daily uptake (EDU) of each target chemical. The hazard index (HI), which represents the cumulative risk from multiple chemicals, was derived by summing the individual HQs. Both HQ and HI values below 1 indicate acceptable non-carcinogenic risk levels, while values above 1 warrant further attention. This study assessed the exposure pathways for children and adults through oral ingestion and dermal contact. The exposure assessment model developed by the U.S. Environmental Protection Agency was applied. The EDU, HQ, and HI values are calculated as follows[31]:
where C is concentration of the chemical in soil (mg/kg); EF is exposure frequency (days/year); RfD (mg/kg/day) is the reference dose; ED is exposure duration (years); IR is soil ingestion per year (mg/day); BW is body weight (kg); CF is conversion factor (dimensionless); SA is exposed skin surface area (cm2); AF is skin adherence factor (mg/cm2/day); Ba (%) is absorption factor of the chemical by dermal contact or oral ingestion (%); AT is average time for non-cancer risk (day); EDU (mg/kg/day) is the estimated daily uptake of the chemical, considering the absorption factor. All the parameters are listed in Supplementary Tables 6 and 7.
Statistical analysis
Analytes with concentrations below the LOD were recorded as “not detected” (n.d.). For detected analytes, concentrations were estimated as half the LOQ when the detection frequency exceeded 50%, or one-quarter of the LOQ otherwise[32]. All statistical analyses were performed using SPSS (IBM, USA), with P < 0.05 considered statistically significant and 0.05 ≤ P < 0.10 considered marginally significant. Non-parametric methods were employed due to the non-normal distribution of the data. The Mann-Whitney U test was used to assess differences in analyte concentrations between the park and surrounding areas. Contour plots were obtained using Surfer (Golden Software, USA).
RESULTS AND DISCUSSION
Occurrence of PBDEs and DPs
The detection frequencies of the target PBDEs and DPs in this study are presented in Supplementary Tables 8-11. PBDEs and DPs were detected at varying levels across all four areas. Notably, within the Weifang flame retardant manufacturing park, all 20 PBDE congeners and both DP compounds demonstrated 100% detection frequencies both inside and outside the park [Supplementary Table 8]. In contrast to the other three petrochemical parks studied, soils in the Lanzhou petrochemical park exhibited significantly higher detection frequencies of both PBDEs and DPs, compared to the surrounding areas outside the park
Among the 20 PBDE congeners, BDE209 had the highest detection frequencies of 86.2%-100% in all four sampling regions. Comparatively, BDE47 and BDE100 showed relatively low detection frequencies. For example, the detection frequencies of BDE47 were only 3.08% in Tangshan and 22.0% in Jieyang soils. For the two DP isomers, the detection frequency of anti-DP was generally higher than that of syn-DP, except in the Jieyang petrochemical park, where anti-DP and syn-DP had equal detection frequencies of 48.0%.
Levels of PBDEs and DPs in different industrial areas
The concentrations of PBDEs and DPs in soils from the four industrial parks are listed in Table 1. The concentration ranges of ∑PBDEs (the total sum of PBDEs) in the Tangshan, Jieyang, Lanzhou, and Weifang industrial parks were 0.48-165, 0-44.4, 0.06-359, and 262-1.48 × 105 ng/g dw, respectively, and of ∑2DPs were 0-4.37, 0-2.23, 0-56.4, and 10.1-6.52 × 104 ng/g dw, respectively. The results showed that PBDEs and DPs were detected in all four park regions, although there were some specific locations where they were not found. The highest concentrations were detected in Weifang, a BFR manufacturing park [Table 1].
Concentrations (ng/g dw) of PBDEs and DPs in soils from the four industry parks and surrounding areas
Compounds | LPP | BFRP | TPP | JPP | ||||||||
Min | Median | Max | Min | Median | Max | Min | Median | Max | Min | Median | Max | |
PBDEs | ||||||||||||
BDE17 | n.d. | n.d. | 2.84 | 0.05 | 0.94 | 497 | n.d. | n.d. | 5.94 | n.d. | n.d. | 1.14 |
BDE28 | n.d. | n.d. | 9.72 | 1.29 | 25.6 | 2.10 × 103 | n.d. | n.d. | 6.34 | n.d. | n.d. | 2.38 |
BDE47 | n.d. | 0.11 | 1.62 | 0.25 | 6.24 | 1.26 × 103 | n.d. | n.d. | 1.26 | n.d. | n.d. | 1.93 |
BDE66 | n.d. | 0.11 | 0.85 | 0.14 | 2.50 | 1.53 × 103 | n.d. | 0.07 | 0.84 | n.d. | n.d. | 1.60 |
BDE71 | n.d. | 0.76 | 18.7 | 0.07 | 15.1 | 648 | n.d. | 0.24 | 62.6 | n.d. | n.d. | 2.01 |
BDE85 | n.d. | 0.08 | 2.96 | 0.14 | 6.76 | 390 | n.d. | 0.10 | 21.8 | n.d. | n.d. | 1.70 |
BDE99 | n.d. | n.d. | 3.06 | 0.14 | 14.9 | 559 | n.d. | 0.17 | 2.87 | n.d. | n.d. | 1.82 |
BDE100 | n.d. | 0.37 | 78.9 | 0.16 | 1.33 | 1.32 × 103 | n.d. | n.d. | 6.33 | n.d. | n.d. | 1.87 |
BDE138 | n.d. | 0.12 | 11.2 | 0.14 | 3.17 | 3.01 × 103 | n.d. | n.d. | 0.84 | n.d. | n.d. | 2.21 |
BDE153 | n.d. | 5.92 | 198 | 1.86 | 73.7 | 7.20 × 103 | n.d. | 0.07 | 1.71 | n.d. | n.d. | 2.37 |
BDE154 | n.d. | 0.13 | 3.77 | 0.27 | 20.8 | 738 | n.d. | 0.04 | 2.33 | n.d. | n.d. | 1.93 |
BDE183 | n.d. | n.d. | 6.48 | 2.47 | 33.0 | 4.59 × 103 | n.d. | n.d. | 1.30 | n.d. | n.d. | 2.29 |
BDE190 | n.d. | n.d. | 8.07 | 0.21 | 10.4 | 6.15 × 103 | n.d. | n.d. | 0.59 | n.d. | n.d. | 2.29 |
BDE196 | n.d. | 0.23 | 5.70 | 0.29 | 21.1 | 3.17 × 103 | n.d. | 0.02 | 1.12 | n.d. | n.d. | 2.30 |
BDE197 | n.d. | 1.06 | 8.80 | 0.77 | 38.6 | 1.18 × 104 | n.d. | 0.11 | 3.62 | n.d. | n.d. | 2.91 |
BDE203 | n.d. | 0.60 | 15.0 | 0.57 | 77.7 | 6.29 × 103 | n.d. | 0.07 | 1.43 | n.d. | n.d. | 3.01 |
BDE206 | n.d. | 0.66 | 14.8 | 2.81 | 181 | 2.51 × 104 | n.d. | n.d. | 29.3 | n.d. | 0.15 | 2.67 |
BDE207 | n.d. | 2.72 | 17.5 | 3.57 | 186 | 6.14 × 104 | n.d. | 0.20 | 7.49 | n.d. | 0.15 | 2.71 |
BDE208 | n.d. | 0.98 | 22.9 | 6.44 | 179 | 1.28 × 104 | n.d. | 0.25 | 8.39 | n.d. | n.d. | 1.72 |
BDE209 | n.d. | 5.36 | 145 | 225 | 3.60 × 103 | 7.16 × 104 | n.d. | 1.30 | 142 | n.d. | 0.45 | 32.4 |
∑PBDEs | 0.06 | 30.4 | 359 | 262 | 5.20 × 103 | 1.48 × 105 | 0.48 | 5.32 | 166 | n.d. | 2.04 | 44.4 |
DP | ||||||||||||
syn-DP | n.d. | 0.22 | 19.2 | 10.0 | 569 | 6.17 × 104 | n.d. | n.d. | 2.58 | n.d. | n.d. | 0.59 |
anti-DP | n.d. | 2.28 | 41.7 | 0.05 | 11.9 | 5.03 × 103 | n.d. | n.d. | 3.38 | n.d. | n.d. | 1.64 |
∑DP | n.d. | 2.59 | 56.4 | 10.1 | 644 | 6.52 × 104 | n.d. | n.d. | 4.37 | n.d. | 0.02 | 2.23 |
The median PBDE concentration in the BFR manufacturing park was 5.20 × 103 ng/g dw, approximately two orders of magnitude higher than the median levels measured in the three petrochemical parks [Table 1]. Furthermore, the median concentration of PBDEs within the BFR manufacturing park was 4.13 × 104 ng/g dw, significantly higher than the median concentration outside the park, which was 2.15 × 103 ng/g dw (P < 0.05) [Supplementary Table 8]. This difference was expected, as two factories in the BFR manufacturing park produce Deca-BDE, with annual production capacities of 6,000 and 2,500 tons/year. Among the target 20 congeners, BDE209, the most common BFR in China, exhibited the highest median concentration (3.60 × 103 ng/g dw), two orders of magnitude higher than the second most abundant congener, BDE207 (186 ng/g dw). High concentrations of BDE209 are typical in flame retardant production areas. Consistent with our findings, BDE209, with a mean concentration of 1.80 × 103 ng/g dw, was identified as the dominant congener in soils from an e-waste recycling facility in eastern China[33]. In comparison, the median concentration of DPs in the BFR manufacturing park was 643.6 ng/g dw, significantly lower than that of PBDEs, but still much higher than the concentrations found in the three petrochemical industry parks. Similar to PBDEs, DP concentrations in the BFR manufacturing park showed a median value of 1.74 × 104 ng/g dw, approximately two orders of magnitude higher than in surrounding areas (101 ng/g dw).
The median concentration of PBDEs in the Lanzhou petrochemical park was 30.4 ng/g dw, which is similar to the concentrations detected in surface soils near a chemical plant (26.0 ng/g, dw) and a power plant
The concentrations of PBDEs and DPs in the vicinity of the Tangshan and Jieyang petrochemical parks are lower than those around the Lanzhou petrochemical park [Supplementary Tables 10 and 11]. Specifically, the median concentration of PBDEs outside the Tangshan petrochemical park is 5.32 ng/g dw, which is slightly higher than that outside the Jieyang petrochemical park. Both values, however, are lower than the median concentration of 12.0 ng/g dw outside the Lanzhou petrochemical park. Notably, in the Jieyang petrochemical park, the median concentrations of PBDEs and DPs measured as low as 2.04 and 0.02 ng/g dw, respectively, far lower than the concentrations detected in the BFR manufacturing and Lanzhou petrochemical parks. This discrepancy is primarily due to the fact that the Jieyang petrochemical park began operation only in 2023. These results highlight the substantial impact of historical chemical emissions on their accumulation in the environment. Therefore, continuous monitoring is necessary to determine whether the Jieyang petrochemical park will have any future emissions of flame retardants that could affect the surrounding environment.
Compared to other regions, the data from the BFR manufacturing park were similar to the results from Laizhou Bay. The median concentration of ∑23PBDEs in the soil collected by Li et al. outside a factory in Laizhou Bay was 2.2 × 103 ng/g, while the concentration inside the factory was 2.80 × 104 ng/g[36]. According to a report by Deng et al., the average concentration of ∑8PBDEs in soil samples collected from a PBDE manufacturing site in Laizhou Bay in 2016 was 1.48 × 105 ng/g[37]. Similar high concentrations of PBDEs were also observed in an e-waste dismantling park in Guiyu, Guangdong Province. The concentration ranges of PBDEs in soil, sediment, dust, ash, and burnt e-wastes were 140-3.9 × 105 ng/g[8]. Such high PBDE concentrations are also commonly found in e-waste dismantling areas in South China, where contamination levels in e-waste recycling zones range from 227 to 1.60 × 105 ng/g and from 530 to
Spatial distribution of PBDEs and DPs in industrial parks and surrounding areas
To identify potential sources and assess the environmental impacts of PBDEs and DPs, their spatial distributions were investigated [Figure 1]. In the BFR manufacturing park, high concentrations of PBDEs were mainly detected within the park itself. A significant amount of these chemicals was also found in the nearby soil as a result of the manufacture of flame retardants. Additionally, due to the predominance of northerly winds during winter in Weifang City, higher concentrations were observed in the areas located downwind of the BFR manufacturing park. Similar effects of wind direction on the spatial distribution of PBDEs and other chemicals have been reported in the literature[40].
Figure 1. The spatial distributions of PBDEs and DPs in parks and their surrounding areas. (A and B) Lanzhou petrochemical park; (C and D) BFR manufacturing park; (E and F) Tangshan petrochemical park; (G and H) Jieyang petrochemical park. (A, C, E, and G) for PBDEs; (B, D, F, and H) for DPs. PBDEs: Polybrominated diphenyl ethers; DPs: Dechlorane Plus; BFR: brominated flame retardant.
In the petrochemical parks, high concentrations of PBDEs and DPs were detected in Lanzhou [Figure 1A and B]. Spatial distribution analysis revealed that the elevated chemical concentrations were predominantly localized within the petrochemical plant boundaries. Two areas with high concentrations were identified: the petrochemical production area and the raw material stacking area. This outcome is expected given the unique nature of the petrochemical industry, which involves the use of equipment that contains substantial amounts of flame retardants to prevent fires. As a result, these flame retardants are released from the equipment into the air, eventually settling on the soil. As reported by Webster et al., BDE209, the primary component of PBDEs, can transfer to dust and soil through physical processes such as wear or weathering[41].
Compared to the other two parks, the Tangshan and Jieyang petrochemical parks had lower levels of PBDEs and DPs. These chemicals were detected both in the steel factory and the oil-producing region in Tangshan, indicating that heavy industries are potential sources of PBDE and DP emissions. Similar findings were reported by Odabasi et al., who identified iron-steel plants, petrochemical plants, ship-breaking, and the petroleum refinery as major sources of some persistent organic pollutants, including PBDEs and polychlorinated biphenyls, in samples collected from the Aliaga industrial region in Turkey[42]. Surprisingly, PBDEs and DPs were also detected in the soil samples near the upper left corner of a toll station at a highway intersection, which may be attributed to the dense traffic and longer vehicle stopping times, resulting in increased exhaust emissions. Therefore, vehicle emissions could also be a contributing source of these chemicals[43].
For the Jieyang petrochemical park, there were no significant detections of PBDEs and DPs around the park. Instead, elevated concentrations of target compounds were identified in residential areas to the northwest of the site. To the best of our knowledge, human activities in the residential area may also contribute to the emission of flame retardants. Research has shown that indoor electronic products and waste incineration in residential areas can release flame retardants[44]. The sampling area is close to the ocean and is susceptible to southeast winds in summer, which causes the chemicals to diffuse from the residential areas to the northwest. Therefore, residential activities significantly influence the presence of these target substances and climate conditions also affect their spatial distribution. Given that the petrochemical park was recently established in Jieyang, it is unlikely to be the main source of PBDEs and DPs in this region.
Composition characteristics of PBDEs and DPs in different industrial areas
In this study, the median concentrations of most compounds were below their respective LODs. Given the significant environmental impact of pollutant emissions on surrounding areas, we employed mean concentrations to characterize the compositional distribution of these compounds. The composition profiles of the target analytes in each individual park area are shown in Figure 2.
Figure 2. Composition profiles of (A) PBDEs and (B) DPs in soil samples from four industrial parks and their surrounding areas. PBDEs: Polybrominated diphenyl ethers; DPs: Dechlorane Plus; LPP: Lanzhou petrochemical park; LPS: surrounding area of Lanzhou petrochemical park; BFRP: BFR manufacturing park; BFRS: surrounding area of BFR manufacturing park.
The relative abundance of BDE209 was highest in the BFR manufacturing park, which is expected given that BDE209 constitutes 91.6% to 96.8% of technical Deca-BDE. Furthermore, technical Deca-BDE is the primary PBDE product in China, while commercial Penta- and Octa-BDEs are more commonly produced in European and American countries. However, the relative abundance of BDE209 within the BFR manufacturing park was 55%, which is lower than the 81% observed in the surrounding area. This discrepancy seems unusual. We hypothesize that it is primarily due to the decades-long history of flame retardant production and emissions in this park, which likely led to the debromination of BDE209 into lower-brominated congeners. In contrast, the surrounding areas are more affected by the recent emission of BDE209. This hypothesis is supported by another study conducted in the same park, which found that the relative abundance of BDE209 in core soil samples decreased from 59.9% to 17.9% with increasing depth[45]. Additionally, previous research has shown that the factory unintentionally produces lower-brominated congeners, such as Nona-BDEs (BDE206, BDE207, BDE208), through photodegradation during PBDE production[46]. The relative abundance of Nona-BDEs within the BFR manufacturing park is 25%, significantly higher than the 10% found outside the park, further supporting the idea that the higher concentration of BDE209 within the park is prone to degradation.
For the petrochemical parks, there were different composition characteristics. In the Tangshan and Jieyang petrochemical parks, BDE209 was found in the highest proportions, with Nona-BDEs being the second most abundant congeners. In contrast, the relative abundances of BDE209 inside and outside the Lanzhou petrochemical park were 32% and 30%, respectively. Additionally, BDE153 exhibited relative abundances of 27% inside the park and 28% outside. This difference is largely due to the long history of the Lanzhou petrochemical park, which started production in 1959, a time when the use of technical Penta-BDEs was not yet prohibited. Consequently, BDE153 has accumulated significantly in the soil samples of both the park and its surrounding areas.
As for DPs, the relative abundance of anti-DP is higher than that of syn-DP in the three petrochemical parks. Specifically, the relative abundances of anti-DP within and around the Lanzhou petrochemical park are 79% and 91%, respectively. In the Tangshan and Jieyang petrochemical parks, the relative abundances of anti-DP are 74% and 64%, respectively. This is mainly due to the commercial production of DP, which follows a 1:3 ratio of syn-DP to anti-DP[47]. However, in the BFR manufacturing park and its surrounding areas, syn-DP is more prevalent than anti-DP, with relative abundances of 98% and 92%, respectively. This phenomenon may result from the greater photodegradation stability of syn-DP isomers or the isomerization of anti-DP to syn-DP, potentially driven by the park’s prolonged history of chemical production[48]. A similar pattern of higher syn-DP levels than anti-DP was also observed in soil near an e-waste dismantling plant in Pakistan[39]. However, the exact cause of this phenomenon remains unclear and requires further investigation.
Health risk assessment
Figure 3 presents the daily exposure levels and associated health risks from oral intake and dermal contact pathways for both children and adults across the four chemical industrial parks, with complete numerical data available in Supplementary Tables 12-15. The daily exposure levels of PBDEs and DPs through oral intake for adults are ranked from highest to lowest as follows: Weifang > Lanzhou > Tangshan > Jieyang, but the daily exposure for children in Jieyang was higher than that in Tangshan [Supplementary Table 12]. The HI values for PBDEs in the flame retardant manufacturing park were comparatively higher compared to the three petrochemical industrial parks for both children and adults. The highest HI value, 0.149, was observed for children exposed to dermal contact in the flame retardant manufacturing park, followed by the Lanzhou petrochemical park area (1.02 × 10-3). For adults, all HI values for PBDEs in the four areas were below 1, suggesting that the associated risks of PBDE exposure are acceptable. Similar results were observed for DPs. Our findings are consistent with studies reporting elevated health risks from environmental exposure to flame retardants in e-waste dismantling areas, where children were found to be more vulnerable than adults[49]. Previous studies have also highlighted dermal contact as the dominant exposure pathway for workers in these facilities[50]. Additionally, a study conducted in a plastic manufacturing plant in eastern China confirmed that dermal exposure posed a greater risk compared to other exposure pathways[51].
Figure 3. Non-carcinogenic risks of PBDEs and DPs in four industrial areas through (A) oral intake and (B) dermal contact for both children and adults. PBDEs: Polybrominated diphenyl ethers; DPs: Dechlorane Plus; LPP: Lanzhou petrochemical park; LPS: surrounding area of the Lanzhou petrochemical park; BFRP: BFR manufacturing park; BFRS: surrounding area of the BFR manufacturing park.
The contribution of each individual compound to the HI is determined solely by its concentration and RfD, regardless of whether the exposure scenario involves adults or children, as all other parameters cancel out proportionally. Additionally, since the risk posed by DPs is two orders of magnitude lower than that of PBDEs, only PBDEs are considered in the analysis. Among the congeners, tetra-BDEs and hexa-BDEs generally contributed more to the HI in the three petrochemical industrial parks, with the highest contribution of 53.1% observed in the Tangshan petrochemical park [Figure 4]. In the flame retardant manufacturing park area, tri- to hepta-BDEs had comparable contribution, although hepta-BDEs showed the highest. Despite the significantly higher daily exposure level of BDE209 compared to low-brominated congeners, it accounted for only 1.5%-7.5% of the total risk, mainly attributed to its higher RfD value.
CONCLUSIONS
This study identified and quantified PBDEs and DPs in the soil of four industrial parks. PBDE concentrations consistently exceeded DP levels across all study areas, with significantly higher concentrations observed within the industrial parks compared to surrounding zones. Notably, PBDE levels in the flame retardant manufacturing park were 2-3 orders of magnitude greater than those in the three petrochemical parks. The compositional profiles of PBDEs across the studied parks were consistent and primarily dominated by Deca-BDE. Its concentration in the BFR manufacturing park was significantly higher than in the three petrochemical parks. In contrast, distinct compositional differences were observed for DPs: syn-DP was the predominant isomer in the BFR manufacturing park, while anti-DP was more prevalent in the three petrochemical parks. Spatial distribution analysis revealed point-source pollution patterns within the petrochemical industrial parks and the BFR manufacturing park, although emission sources were also found in the surrounding areas. This study confirmed the distinct emission profiles of PBDEs and DPs in both the BFR manufacturing park and petrochemical parks. Considering the temporal variation in sampling across different sites, the potential impact of this timespan on the concentrations and compositions of these chemicals cannot be excluded. Risk assessments showed that both PBDEs and DPs posed acceptable non-carcinogenic risks through oral and dermal exposure pathways. However, in the flame retardant manufacturing park, children showed higher HI values than adults. Although BDE209 had the highest concentration, low-brominated congeners contributed more to the total non-carcinogenic risks. This study bridges a critical knowledge gap regarding fugitive emission profiling of BFRs across petrochemical production complexes, providing foundational data to inform sector-specific mitigation frameworks for prioritized contaminants.
DECLARATIONS
Authors’ contributions
Methodology, data analysis, and draft preparation: Yang, C.
Data analysis and draft preparation: Long, C.
Methodology and data analysis: Huang, Z.
Sampling and methodology: Ren, H.
Design, reviewing and editing: Yu, Y.
Availability of data and materials
Supplementary data associated with this article can be found in Supplementary Materials. Further data are available from the corresponding author upon reasonable request.
Financial support and sponsorship
The study was supported by the National Natural Science Foundation of China (41991311), the Key R&D Plans of Guangzhou Science and Technology (202206010190), Guangdong Provincial Key R&D Program (2022-GDUT-A0007), and the Guangdong-Hong Kong-Macao Joint Laboratory for Contaminants Exposure and Health (2020B1212030008).
Conflicts of interest
All authors declared that there are no conflicts of interest.
Ethical approval and consent to participate
Not applicable.
Consent for publication
Not applicable.
Copyright
© The Author(s) 2025.
Supplementary Materials
REFERENCES
1. Alaee, M.; Arias, P.; Sjödin, A.; Bergman, A. An overview of commercially used brominated flame retardants, their applications, their use patterns in different countries/regions and possible modes of release. Environ. Int. 2003, 29, 683-9.
2. Zhang, Y.; Xi, B.; Tan, W. Release, transformation, and risk factors of polybrominated diphenyl ethers from landfills to the surrounding environments: a review. Environ. Int. 2021, 157, 106780.
3. Chen, Z.; Luo, X.; Zeng, Y.; Tan, S.; Guo, J.; Xu, Z. Polybrominated diphenyl ethers in indoor air from two typical E-waste recycling workshops in Southern China: emission, size-distribution, gas-particle partitioning, and exposure assessment. J. Hazard. Mater. 2021, 402, 123667.
4. Beach, M. W.; Kearns, K. L.; Davis, J. W.; et al. Stability assessment of a polymeric brominated flame retardant in polystyrene foams under application-relevant conditions. Environ. Sci. Technol. 2021, 55, 3050-8.
5. Charitopoulou, M. A.; Kalogiannis, K. G.; Lappas, A. A.; Achilias, D. S. Novel trends in the thermo-chemical recycling of plastics from WEEE containing brominated flame retardants. Environ. Sci. Pollut. Res. Int. 2021, 28, 59190-213.
6. Estill, C. F.; Mayer, A. C.; Chen, I. C.; et al. Biomarkers of organophosphate and polybrominated diphenyl ether (PBDE) flame retardants of American workers and associations with inhalation and dermal exposures. Environ. Sci. Technol. 2024, 58, 8417-31.
7. Liu, L.; Zhen, X.; Wang, X.; Zhang, D.; Sun, L.; Tang, J. Spatio-temporal variations and input patterns on the legacy and novel brominated flame retardants (BFRs) in coastal rivers of North China. Environ. Pollut. 2021, 283, 117093.
8. Labunska, I.; Harrad, S.; Santillo, D.; Johnston, P.; Brigden, K. Levels and distribution of polybrominated diphenyl ethers in soil, sediment and dust samples collected from various electronic waste recycling sites within Guiyu town, Southern China. Environ. Sci. Process. Impacts. 2013, 15, 503-11.
9. Han, W.; Fan, T.; Xu, B.; et al. Passive sampling of polybrominated diphenyl ethers in indoor and outdoor air in Shanghai, China: seasonal variations, sources, and inhalation exposure. Environ. Sci. Pollut. Res. Int. 2016, 23, 5771-81.
10. Ohoro, C. R.; Adeniji, A. O.; Okoh, A. I.; Okoh, O. O. Polybrominated diphenyl ethers in the environmental systems: a review. J. Environ. Health. Sci. Eng. 2021, 19, 1229-47.
11. Sun, R. X.; Pan, C. G.; Peng, F. J.; et al. Evidence of polybrominated diphenyl ethers (PBDEs) and alternative halogenated flame retardants (AHFRs) in wild fish species from the remote tropical marine environment, south China sea. Environ. Pollut. 2024, 361, 124885.
12. Gomes, J.; Begum, M.; Kumarathasan, P. Polybrominated diphenyl ether (PBDE) exposure and adverse maternal and infant health outcomes: systematic review. Chemosphere 2024, 347, 140367.
13. Lin, Y.; Yang, L.; Xie, M.; Li, H.; Zhang, Q. Exposure to polybrominated diphenyl ethers and thyroid disease: a systematic review and meta-analysis of epidemiological studies. Curr. Epidemiol. Rep. 2024, 11, 20-31.
14. Wang, N.; Lai, C.; Xu, F.; et al. A review of polybrominated diphenyl ethers and novel brominated flame retardants in Chinese aquatic environment: source, occurrence, distribution, and ecological risk assessment. Sci. Total. Environ. 2023, 904, 166180.
15. Xian, Q.; Siddique, S.; Li, T.; Feng, Y.; Takser, L.; Zhu, J. Sources and environmental behavior of Dechlorane Plus - a review. Environ. Int. 2011, 37, 1273-84.
16. Bao, J.; Ren, H.; Han, J.; Yang, X.; Li, Y.; Jin, J. Levels, tissue distribution and isomer stereoselectivity of Dechlorane Plus in humans: a critical review. Sci. Total. Environ. 2023, 903, 166156.
17. Feo, M. L.; Barón, E.; Eljarrat, E.; Barceló, D. Dechlorane Plus and related compounds in aquatic and terrestrial biota: a review. Anal. Bioanal. Chem. 2012, 404, 2625-37.
18. Qiu, X.; Marvin, C. H.; Hites, R. A. Dechlorane Plus and other flame retardants in a sediment core from Lake Ontario. Environ. Sci. Technol. 2007, 41, 6014-9.
19. Zafar, M. I.; Kali, S.; Ali, M.; et al. Dechlorane Plus as an emerging environmental pollutant in Asia: a review. Environ. Sci. Pollut. Res. Int. 2020, 27, 42369-89.
20. Chen, K.; Zheng, J.; Yan, X.; et al. Dechlorane Plus in paired hair and serum samples from e-waste workers: correlation and differences. Chemosphere 2015, 123, 43-7.
21. Abdel Malak, I.; Cariou, R.; Guiffard, I.; et al. Assessment of Dechlorane Plus and related compounds in foodstuffs and estimates of daily intake from Lebanese population. Chemosphere 2019, 235, 492-7.
22. Yadav, I. C.; Devi, N. L.; Kumar, A.; Li, J.; Zhang, G. Airborne brominated, chlorinated and organophosphate ester flame retardants inside the buildings of the Indian state of Bihar: exploration of source and human exposure. Ecotoxicol. Environ. Saf. 2020, 191, 110212.
23. Zhang, H.; Bayen, S.; Kelly, B. C. Multi-residue analysis of legacy POPs and emerging organic contaminants in Singapore’s coastal waters using gas chromatography-triple quadrupole tandem mass spectrometry. Sci. Total. Environ. 2015, 523, 219-32.
24. Mortensen, Å. K.; Verreault, J.; François, A.; et al. Flame retardants and their associations with thyroid hormone-related variables in northern fulmars from the Faroe Islands. Sci. Total. Environ. 2022, 806, 150506.
25. Zhu, J.; Zhao, L.; Guo, L. Dechloranes exhibit binding potency and activity to thyroid hormone receptors. J. Environ. Sci. 2022, 112, 16-24.
26. Lan, Y.; Liu, Y.; Cai, Y.; et al. Eight novel brominated flame retardants in indoor and outdoor dust samples from the E-waste recycling industrial park: Implications for human exposure. Environ. Res. 2023, 238, 117172.
27. Li, N.; Chen, X. W.; Deng, W. J.; Giesy, J. P.; Zheng, H. L. PBDEs and Dechlorane Plus in the environment of Guiyu, Southeast China: a historical location for E-waste recycling (2004, 2014). Chemosphere 2018, 199, 603-11.
28. Zhang, K.; Schnoor, J. L.; Zeng, E. Y. E-waste recycling: where does it go from here? Environ. Sci. Technol. 2012, 46, 10861-7.
29. Kirk–Othmer encyclopedia of chemical technology. New York: Wiley. 2001. https://onlinelibrary.wiley.com/doi/book/10.1002/0471238961. (accessed 29 Apr 2025).
30. Ministry of Ecology and Environment of the People’s Republic of China. Technical guidelines for monitoring during risk control and remediation of soil contamination of land for construction. 2019. https://www.mee.gov.cn/ywgz/fgbz/bz/bzwb/trhj/201912/W020191224560850148092.pdf. (accessed 29 Apr 2025).
31. Yang, J.; Huang, D.; Zhang, L.; et al. Multiple-life-stage probabilistic risk assessment for the exposure of Chinese population to PBDEs and risk managements. Sci. Total. Environ. 2018, 643, 1178-90.
32. GB 17378.2-2007. The specification for marine monitoring - Part 2: data processing and quality control of analysis. https://webstore.ansi.org/standards/spc/GB173782007-1682981. (accessed 29 Apr 2025)
33. Ma, J.; Addink, R.; Yun, S.; Cheng, J.; Wang, W.; Kannan, K. Polybrominated dibenzo-p-dioxins/dibenzofurans and polybrominated diphenyl ethers in soil, vegetation, workshop-floor dust, and electronic shredder residue from an electronic waste recycling facility and in soils from a chemical industrial complex in Eastern China. Environ. Sci. Technol. 2009, 43, 7350-6.
34. Zhang, K.; Wei, Y. L.; Zeng, E. Y. A review of environmental and human exposure to persistent organic pollutants in the Pearl River Delta, South China. Sci. Total. Environ. 2013, 463-4, 1093-110.
35. Yu, Z.; Lu, S.; Gao, S.; et al. Levels and isomer profiles of Dechlorane Plus in the surface soils from e-waste recycling areas and industrial areas in South China. Environ. Pollut. 2010, 158, 2920-5.
36. Li, W. L.; Liu, L. Y.; Zhang, Z. F.; et al. Brominated flame retardants in the surrounding soil of two manufacturing plants in China: occurrence, composition profiles and spatial distribution. Environ. Pollut. 2016, 213, 1-7.
37. Deng, C.; Chen, Y.; Li, J.; Li, Y.; Li, H. Environmental pollution of polybrominated diphenyl ethers from industrial plants in China: a preliminary investigation. Environ. Sci. Pollut. Res. Int. 2016, 23, 7012-21.
38. Wang, J.; Ma, Y. J.; Chen, S. J.; Tian, M.; Luo, X. J.; Mai, B. X. Brominated flame retardants in house dust from e-waste recycling and urban areas in South China: implications on human exposure. Environ. Int. 2010, 36, 535-41.
39. Iqbal, M.; Syed, J. H.; Breivik, K.; et al. E-waste driven pollution in Pakistan: the first evidence of environmental and human exposure to flame retardants (FRs) in Karachi City. Environ. Sci. Technol. 2017, 51, 13895-905.
40. Wu, Z.; Han, W.; Xie, M.; Han, M.; Li, Y.; Wang, Y. Occurrence and distribution of polybrominated diphenyl ethers in soils from an e-waste recycling area in northern China. Ecotoxicol. Environ. Saf. 2019, 167, 467-75.
41. Webster, T. F.; Harrad, S.; Millette, J. R.; et al. Identifying transfer mechanisms and sources of decabromodiphenyl ether (BDE 209) in indoor environments using environmental forensic microscopy. Environ. Sci. Technol. 2009, 43, 3067-72.
42. Odabasi, M.; Falay, E. O.; Tuna, G.; et al. Biomonitoring the spatial and historical variations of persistent organic pollutants (POPs) in an industrial region. Environ. Sci. Technol. 2015, 49, 2105-14.
43. Wang, L. C.; Lee, W. J.; Lee, W. S.; Chang-Chien, G. P. Emission estimation and congener-specific characterization of polybrominated diphenyl ethers from various stationary and mobile sources. Environ. Pollut. 2010, 158, 3108-15.
44. Al-Harbi, M.; Al-Enzi, E.; Al-Mutairi, H.; Whalen, J. K. Human health risks from brominated flame retardants and polycyclic aromatic hydrocarbons in indoor dust. Chemosphere 2021, 282, 131005.
45. Bai, C.; Ge, X.; Huang, Z.; et al. Polybrominated diphenyl ethers and their alternatives in soil cores from a typical flame-retardant production park: vertical distribution and potential influencing factors. Environ. Pollut. 2024, 359, 124597.
46. Stapleton, H. M.; Dodder, N. G. Photodegradation of decabromodiphenyl ether in house dust by natural sunlight. Environ. Toxicol. Chem. 2008, 27, 306-12.
47. Zhu, J.; Feng, Y. L.; Shoeib, M. Detection of Dechlorane Plus in residential indoor dust in the city of Ottawa, Canada. Environ. Sci. Technol. 2007, 41, 7694-8.
48. Mwangi, J. K.; Lee, W. J.; Wang, L. C.; et al. Persistent organic pollutants in the Antarctic coastal environment and their bioaccumulation in penguins. Environ. Pollut. 2016, 216, 924-34.
49. Yu, Y.; Li, C.; Zhang, X.; et al. Route-specific daily uptake of organochlorine pesticides in food, dust, and air by Shanghai residents, China. Environ. Int. 2012, 50, 31-7.
50. Ohajinwa, C. M.; van, B. P. M.; Osibanjo, O.; et al. Health risks of polybrominated diphenyl ethers (PBDEs) and metals at informal electronic waste recycling sites. Int. J. Environ. Res. Public. Health. 2019, 16, 906.
Cite This Article

How to Cite
Download Citation
Export Citation File:
Type of Import
Tips on Downloading Citation
Citation Manager File Format
Type of Import
Direct Import: When the Direct Import option is selected (the default state), a dialogue box will give you the option to Save or Open the downloaded citation data. Choosing Open will either launch your citation manager or give you a choice of applications with which to use the metadata. The Save option saves the file locally for later use.
Indirect Import: When the Indirect Import option is selected, the metadata is displayed and may be copied and pasted as needed.
About This Article
Special Issue
Copyright
Data & Comments
Data
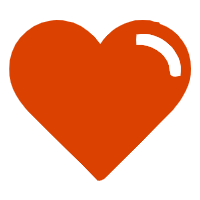
Comments
Comments must be written in English. Spam, offensive content, impersonation, and private information will not be permitted. If any comment is reported and identified as inappropriate content by OAE staff, the comment will be removed without notice. If you have any queries or need any help, please contact us at [email protected].