Chlorinated paraffin metabolism and its importance for understanding CP toxicity
Abstract
Chlorinated paraffins (CPs) are industrial chemicals with broad use as plasticizers and components in paints, cutting and drilling oil additives. The research interest in CPs has recently increased, not least due to the progress in analytical techniques and the globally reported large production volumes of CPs. The adverse effects on CPs in biota and in man are being reported in an increasing number of articles and the mechanism of toxicity is being discussed. Whether the metabolism of CPs could increase their toxicity is, however, still an unsolved question. In this Perspective paper, CP metabolism is discussed and arguments pointing to the important role of metabolic enhancement in CP toxicity are highlighted.
Keywords
SHORT COMMON BACKGROUND
How come a globally used industrial chemical, produced in extensive volumes during at least 50 years and today found in biological samples often in higher amounts than of other environmental compounds, has only recently become a commonly recognized environmental and human health concern? There are, of course, several answers to why chlorinated paraffins (CPs) have just lately sparked a marked activity in terms of regulatory measures[1,2] and an increase in scientific publications[3,4]. For one thing, these chlorinated straight-chain alkanes, generally divided into short-, medium- and long-chain CPs (SCCPs, MCCPs, LCCPs)[5], have created severe problems for analytical chemists, not least due to the vast number of isomers being present in each commercial CP product[6]. Recently, major advancements have been achieved in the chemical analysis of CPs, making it possible to quantitate CP levels in man and environment and to differentiate between different CP groups and isomers in biological samples[6]. Another fact that may have previously deterred the scientific community from showing interest in CPs is their moderate persistence compared to earlier studied persistent pollutants, such as polychlorinated biphenyls (PCBs) and polybrominated diphenyl ethers (PBDEs). In classical toxicological thinking, the metabolism of a persistent pollutant implies that the compound has decreased its harmful properties. Today, an increasing number of scientific studies present data on CP metabolism in different model systems, both animal and human[7-9]. If we consider the vast number of CP isomers present in commercial mixtures and the potential formation of various types of metabolites from these original compounds, it becomes evident that an immense array of CP metabolite structures could emerge, which considerably amplifies the complexity of the analysis. So, what will happen if the metabolism of CPs increases their toxicity rather than reducing it? The rest of this text will, therefore, focus on CP metabolism, as this could have important implications.
EARLY INDICATIONS OF CP METABOLISM
In the 1980s, I worked in a Swedish research team led by Drs Ingvar Brandt and Åke Bergman, which studied CP disposition in rodent and bird models with the aid of radioactively labeled CP compounds. We showed that CPs of low and medium chain length were retained for some time in the body and that this retention increased with the degree of chlorination[10,11]. Concomitantly, the CP carbon chain could be degraded with the formation of CO2, a degradation that was inverse to the degree of chlorination[11]. It was also noted that this carbon chain degradation had to be preceded by a dechlorination step, as the 14C-carbon was chlorinated, and that cytochrome 450 was involved in this degradation[12]. Additional studies reported biliary sulfur-containing CP metabolites, indicating phase II metabolism[13]. In short, it was concluded that CPs are metabolized and degraded in vivo in rodents and birds, and that phase I and II metabolites are indicated. Additionally, in bacteria, enzymatic degradation of CPs was shown in the 1980s by
RECENT PROGRESS IN RESEARCH ON CP EFFECTS
Since these early works, the CP literature has greatly expanded, and during the last 10 years, there has been an exponential increase in scientific CP articles. Apart from the vast increase in analytical-chemical articles, mirroring the advantages in chemical identification and quantification of CPs in different matrices, an increasing number of articles have also been focused on CP effects in various biological models, some also including speculations on mechanisms of toxicity. Already in the early CP papers, histologically based findings identified the liver and kidney as targets of toxicity[15,16]. In addition, short-chain CPs (60%Cl) were classified as potentially carcinogenic (group 2B) by IARC[17]. Today, an increased number of CP-related findings are known, including effects on thyroid hormone and hormonal systems[18-21], disruption of metabolic systems[22,23], developmental effects[24], and immunomodulatory effects[25]. Interestingly, a few papers have identified that certain CP effect parameters could be modified by co-exposure to other compounds, opening for the possibility of synergistic effects (PBDEs and CPs - effects on TH levels and P450 activities[26,27]; PAHs and CPs - perturbation of metabolic activities[28]).
NEW FINDINGS ON CP METABOLISM
Recently published data from studies on various biological levels give an improved scientific base for understanding CP metabolism. In bacteria, CPs could be degraded to chlorinated olefins by LinA2 enzymes via a HCl elimination reaction, and CPs could be divided into reactive and persistent congeners, possibly depending on the degree and position of chlorines on the CP molecule[29]. Mono- and dehydroxylated CP products were also formed in bacterial systems (LinB enzyme)[30]. In pumpkins and soybeans, plant biotransformation of CPs was observed, and dechlorination, chlorine rearrangement, and carbon chain decomposition were proposed as the major metabolic pathways for SCCPs[31,32]. In rice cell suspension, another plant-based system, the metabolism of SCCPs and MCCPs resulted in 25 and 40 metabolic products, respectively, including hydroxylated, dechlorinated, HCl-eliminated compounds, and conjugated products[33]. In vertebrates, studies were performed in chicken and human microsomes. In chicken microsomes, after incubations with several CPs, Huang et al. found an extensive metabolism resulting in mainly monohydroxylated metabolites and, in smaller amounts, dihydroxylated[34]. In another study on chicken liver microsomes, Lin et al. found only one CP metabolite, namely a monohydroxylated hexachlorodecane (after incubation of a heptachlorodecane)[35]. The latter authors also studied human liver microsomes in a similar model and using the same CP isomer. In this case, two metabolites were detected (monohydroxy-hexachlorodecane, and -heptachlorodecane, respectively). As compared to chicken microsomes, the observed average biotransformation rate of the heptachlorodecane was much faster for human liver microsomes[35]. Another study on human liver microsomes underlined results from the previous one and suggested that rapid metabolic processes take place upon incubation with CPs[36]. Several tentative CP metabolites, including alcohols, ketones, and carboxylic acids, were suggested. C-C bond cleavage was also shown, giving rise to shortened CP forms and, eventually, very short chain chlorinated paraffins. The ketone products may have longer metabolic half-life, and therefore, according to the authors, they could be considered suitable as exposure biomarkers.
Whereas previous indications of CP metabolism have been indicated by carbon chain degradation to CO2, sulfur-containing fecal biliary metabolites, and conclusions drawn from mass balance calculations in feeding experiments, the recent progress in CP metabolism studies in different experimental systems clearly manifests that CPs are subjected to metabolic transformation, and that both phase I and II reactions occur. Schematic figures to summarize possible basic metabolic steps starting from the parent CP molecule have been shown by Darnerud and Bergman[8] and by Chen et al.[3], and several different initial metabolic reactions are postulated: hydrolysis, to form primary or secondary alcohols; dehydrochlorination, with emission of HCl; reductive dechlorination, with loss of Cl; vicinal halogen reduction, with loss of Cl2; and oxidative dehalogenation, replacing a carbon chlorine with an aldehyde or a ketone [Figure 1]. However, most of the proposed CP metabolites await structural identification.
Figure 1. Proposed initial metabolic steps starting from a thought CP congener, 2,4,5,8,10,14-hexachlorotetradecane. The postulated metabolic reactions are: hydrolysis, to form primary (I a) or secondary alcohols (I b); dehydrochlorination, with emission of HCl (II); reductive dechlorination, with loss of Cl (III); vicinal halogen reduction, with loss of Cl2; and oxidative dehalogenation, replacing a carbon chlorine with an aldehyde (V a) or ketone (V b). This simplified figure does not show the subsequent metabolic reactions that will likely take place; however, a more detailed metabolic scheme has been proposed by Darnerud and Bergman[8].
IS CP METABOLISM A KEY TO UNDERSTANDING CP EFFECTS IN ANIMALS AND HUMANS?
While the extensive metabolism of CPs gives several metabolites with different chemical properties, it is still largely unknown how these transformation products interact with biological systems. There are several reports claiming that CPs exert their adverse effects through the production of reactive oxygen species (ROS) and perturbation of metabolic processes, e.g., fatty acid metabolism[37]. Notably, Wang et al. claim that CP metabolites are responsible for the production of ROS, with potential effects on enzyme activities, dysregulation of signaling pathways, and damage of cellular components and DNA, thereby resulting in various toxic effects[38-41]. Oxidative stress could also induce the formation of chlorinated fatty lipids, such as chloro fatty aldehydes and chlorohydrins, which have potent pro-inflammatory effects[42]. Thus, evidence is building for the role of chlorinated lipids in inflammatory disease, and CP metabolites may have a near resemblance to these lipids.
The carcinogenicity of CPs in animal models is well established and SCCPs are defined as carcinogenic according to IARC[17]. Assuming that CP binding to macromolecules is a prerequisite for tumor formation and likely for exerting other toxic effects, CPs must be metabolically activated, possibly via dehydrochlorination and subsequent steps as outlined in Figure 1 and earlier presented by Darnerud and Bergman[8]. Regarding a monochlorinated alkane, 1-chlorodecane, an interesting theoretical metabolism study suggested that the metabolites, 10-chloro-decan-5-ol and 1-chlorodecanol, might be more easily bioaccumulated, more carcinogenic, and induce more cardiovascular damage than the parent SCCP isomer[43].
When it comes to the hormonal effects of CPs, the effects on plasma thyroxine (T4) and thyroid-stimulating hormone (TSH) levels are documented, and mechanistically, CPs probably interact with ligand binding to the constitutive androstane receptor (CAR) in the liver and to the transport protein transthyretin (TTR)[18,19]. Another example of ligand binding is that of SCCPs to the peroxisome proliferator-activated receptor alpha (PPARα), studied by, e.g., Gong et al.[44]. If molecular docking to active ligand sites requires the parent CPs, the role of CP metabolism is more questionable, and metabolic transformation of the CP molecule would possibly decrease these hormonal effects. However, a new group of compounds has been found in commercial CP mixtures, namely chlorinated fatty acid methyl esters (CFAMEs). These compounds have been found to be ubiquitous in environmental matrices and have also been detected in blood samples from the general population[45]. CFAMEs can compete with T4 for binding TTR with higher potencies than the CPs and may lead to disruption of thyroid hormone homeostasis. Whether these CFAMEs could also be formed in vivo as metabolites from CPs is not yet shown but could be assumed according to the suggested metabolic scheme for CPs.
SUGGESTIONS IN FUTURE CP RESEARCH
In this paper, CP metabolites are suggested to be important in producing adverse biological effects following CP administration in animals and humans. However, data is still scarce, and the theory must be confirmed in future studies. The following questions are important to discuss and possibly clarify in future CP research:
● Are there metabolic differences between different CP isomers, and could the degree of chlorination, carbon length, and molecular Cl positions on the carbon chain explain these differences? It is notable that in the studies of Knobloch et al.[30], a difference in persistence to bacterial CP metabolizing enzymes is suggested, with two groups of CPs being either reactive or persistent to bacterial enzymatic dehalogenation.
● What types of different CP metabolites are formed and in which proportions are they formed? Could certain CP metabolites be defined as especially “toxic”, and what factors decide the formation of these potentially harmful metabolites?
● Could external factors, e.g., nutritional status and concomitant exposure to other substances, influence CP metabolism and, consequently, CP toxicity? Perhaps the perturbation of metabolic enzyme activities, e.g., by knock-down techniques, could be fruitful in future studies.
● Are there distinct species differences in CP metabolism, and if so, to what extent could metabolism results from animal studies be applicable to man?
The knowledge on the disposition of CPs in animals and humans has today reached an exciting level, where analytical techniques make it possible to evaluate levels of different CP isomers in biological samples and to begin to follow the formation of different CP metabolites. Studies on the potential correlation between toxic effects and specific CP metabolites could give important information in future risk assessments.
DECLARATIONS
Acknowledgements
I thank my former research team, under the lead of Prof. Åke Bergman and late Prof. Ingvar Brandt, for all the support and fun during the years. I also acknowledge the Swedish EPA for financial support during these years.
Author contribution
The author contributed solely to the article.
Availability of data and contents
Not applicable.
Formal support and sponsorship
Not applicable.
Ethical approval and consent to participate
Not applicable.
Consent for publication
Not applicable.
Copyright
© The Author(s) 2023.
REFERENCES
1. Candidate POPs chlorinated paraffins with carbon chain lengths in the rang C14-17 and chlorination levels at or exceeding 45 per cent Chlorine by Weight. Available from: https://chm.pops.int/TheConvention/POPsReviewCommittee/Meetings/POPRC17/MeetingDocuments/tabid/8918/Default.aspx [Last accessed on 10 Nov 2023].
2. Report on the conference of parties to the stockholm convention on persistent organic pollutants on the work of its eight meeting. Available from: https://chm.pops.int/theconvention/conferenceoftheparties/meetings/cop8/tabid/5309/default.aspx [Last accessed on 10 Nov 2023].
3. Chen W, Liu J, Hou X, Jiang G. A review on biological occurrence, bioaccumulation, transmission and metabolism of chlorinated paraffins. Crit Rev Env Sci Tec 2023:online ahead of print.
4. Mu YW, Cheng D, Zhang CL, Zhao XL, Zeng T. The potential health risks of short-chain chlorinated paraffin: a mini-review from a toxicological perspective. Sci Total Environ 2023;872:162187.
5. EFSA. Risk assessment of chlorinated paraffins in feed and food. Available from: https://www.efsa.europa.eu/en/efsajournal/pub/5991 [Last accessed on 10 Nov 2023].
6. Yuan B, Muir D, Macleod M. Methods for trace analysis of short-, medium-, and long-chain chlorinated paraffins: critical review and recommendations. Analytica Chimica Acta 2019;1074:16-32.
7. Chen L, Mai B, Luo X. Bioaccumulation and biotransformation of chlorinated paraffins. Toxics 2022;10:778.
8. Darnerud PO, Bergman Å. Critical review on disposition of chlorinated paraffins in animals and humans. Environ Int 2022;163:107195.
9. Yuan S, Wang M, Lv B, Wang J. Transformation pathways of chlorinated paraffins relevant for remediation: a mini-review. Environ Sci Pollut Res 2021;28:9020-8.
10. Biessmann A, Darnerud PO, Brandt I. Chlorinated paraffins: disposition of a highly chlorinated polychlorohexadecane in mice and quail. Arch Toxicol 1983;53:79-86.
11. Darnerud PO, Biessmann A, Brandt I. Metabolic fate of chlorinated paraffins: degree of chlorination of [1-14C]-chlorododecanes in relation to degradation and excretion in mice. Arch Toxicol 1982;50:217-26.
12. Darnerud PO. Chlorinated paraffins: effect of some microsomal enzyme inducers and inhibitors on the degradation of 1-14C-chlorododecanes to 14CO2 in mice. Acta Pharmacol Toxicol 1984;55:110-5.
13. Ahlman M, Bergman A, Darnerud PO, Egestad B, Sjövall J. Chlorinated paraffins: formation of sulphur-containing metabolites of polychlorohexadecane in rats. Xenobiotica 1986;16:225-32.
14. Omori T, Kimura T, Kodama T. Bacterial cometabolic degradation of chlorinated paraffins. Appl Microbiol Biotechnol 1987;25:553-7.
15. Bucher JR, Alison RH, Montgomery CA, et al. Comparative toxicity and carcinogenicity of two chlorinated paraffins in F344N rats and B6C3F1 mice. Fundam Appl Toxicol 1987;9:454-68.
16. Serrone DM, Birtley RD, Weigand W, Millischer R. Toxicology of chlorinated paraffins. Food Chem Toxicol 1987;25:553-62.
17. Chlorinated paraffins. IARC monogr eval carcinog risks hum. Available from: https://publications.iarc.fr/_publications/media/download/1716/0355080f19678d0a827e62ad130467568c603bb1.pdf [Last accessed on 10 Nov 2023].
18. Gong Y, Zhang H, Geng N, et al. Short-chain chlorinated paraffins (SCCPs) induced thyroid disruption by enhancement of hepatic thyroid hormone influx and degradation in male Sprague Dawley rats. Sci Total Environ 2018;625:657-66.
19. Sprengel J, Behnisch PA, Besselink H, Brouwer A, Vetter W. In vitro human cell-based TTR-TRβ CALUX assay indicates thyroid hormone transport disruption of short-chain, medium-chain, and long-chain chlorinated paraffins. Arch Toxicol 2021;95:1391-6.
20. Wyatt I, Coutts CT, Elcombe CR. The effect of chlorinated paraffins on hepatic enzymes and thyroid hormones. Toxicology 1993;77:81-90.
21. Zhang Q, Wang J, Zhu J, Liu J, Zhang J, Zhao M. Assessment of the endocrine-disrupting effects of short-chain chlorinated paraffins in in vitro models. Environ Int 2016;94:43-50.
22. Geng N, Luo Y, Cao R, et al. Effect of short-chain chlorinated paraffins on metabolic profiling of male SD rats. Sci Total Environ 2021;750:141404.
23. Geng N, Ren X, Gong Y, et al. Integration of metabolomics and transcriptomics reveals short-chain chlorinated paraffin-induced hepatotoxicity in male Sprague-Dawley rat. Environ Int 2019;133:105231.
24. Liu L, Li Y, Coelhan M, Chan HM, Ma W, Liu L. Relative developmental toxicity of short-chain chlorinated paraffins in Zebrafish (Danio rerio) embryos. Environ Pollut 2016;219:1122-30.
25. Wang X, Zhu J, Kong B, et al. C(9-13) chlorinated paraffins cause immunomodulatory effects in adult C57BL/6 mice. Sci Total Environ 2019;675:110-21.
26. Hallgren S, Darnerud PO. Polybrominated diphenyl ethers (PBDEs), polychlorinated biphenyls (PCBs) and chlorinated paraffins (CPs) in rats-testing interactions and mechanisms for thyroid hormone effects. Toxicology 2002;177:227-43.
27. Lundstedt-enkel K, Karlsson D, Darnerud PO. Interaction study with rats given two flame retardants: polybrominated diphenyl ethers (Bromkal 70-5 DE) and chlorinated paraffins (Cereclor 70L). J Chemometr 2010;24:710-8.
28. Wang F, Zhang H, Geng N, et al. A metabolomics strategy to assess the combined toxicity of polycyclic aromatic hydrocarbons (PAHs) and short-chain chlorinated paraffins (SCCPs). Environ Pollut 2018;234:572-80.
29. Heeb NV, Schalles S, Lehner S, et al. Biotransformation of short-chain chlorinated paraffins (SCCPs) with LinA2: A HCH and HBCD converting bacterial dehydrohalogenase. Chemosphere 2019;226:744-54.
30. Knobloch MC, Schinkel L, Schilling I, et al. Transformation of short-chain chlorinated paraffins by the bacterial haloalkane dehalogenase LinB - Formation of mono- and di-hydroxylated metabolites. Chemosphere 2021;262:128288.
31. Li Y, Hou X, Chen W, et al. Carbon chain decomposition of short chain chlorinated paraffins mediated by pumpkin and soybean seedlings. Environ Sci Technol 2019;53:6765-72.
32. Li Y, Hou X, Yu M, et al. Dechlorination and chlorine rearrangement of 1,2,5,5,6,9,10-heptachlorodecane mediated by the whole pumpkin seedlings. Environ Pollut 2017;224:524-31.
33. Chen W, Yu M, Zhang Q, et al. Metabolism of SCCPs and MCCPs in suspension rice cells based on paired mass distance (PMD) analysis. Environ Sci Technol 2020;54:9990-9.
34. Huang X, Xu K, Lyu L, Ding C, Zhao Y, Wang X. Identification and yield of metabolites of chlorinated paraffins incubated with chicken liver microsomes: Assessment of their potential to convert into metabolites. J Hazard Mater 2023;455:131640.
35. Lin L, Abdallah MAE, Chen LJ, Luo XJ, Mai BX, Harrad S. Comparative in vitro metabolism of short chain chlorinated paraffins (SCCPs) by human and chicken liver microsomes: first insight into heptachlorodecanes. Sci Total Environ 2022;851:158261.
36. He C, van Mourik L, Tang S, et al. In vitro biotransformation and evaluation of potential transformation products of chlorinated paraffins by high resolution accurate mass spectrometry. J Hazard Mater 2021;405:124245.
37. Ren X, Geng N, Zhang H, et al. Comparing the disrupting effects of short-, medium- and long-chain chlorinated paraffins on cell viability and metabolism. Sci Total Environ 2019;685:297-307.
38. Wang X, Zhu J, Xue Z, Jin X, Jin Y, Fu Z. The environmental distribution and toxicity of short-chain chlorinated paraffins and underlying mechanisms: Implications for further toxicological investigation. Sci Total Environ 2019;695:133834.
39. Geng N, Zhang H, Zhang B, et al. Effects of short-chain chlorinated paraffins exposure on the viability and metabolism of human hepatoma HepG2 cells. Environ Sci Technol 2015;49:3076-83.
40. Liang Y, Li J, Lin Q, et al. Research progress on signaling pathway-associated oxidative stress in endothelial cells. Oxid Med Cell Longev 2017;2017:7156941.
41. Chen JW, Ni BB, Li B, Yang YH, Jiang SD, Jiang LS. The responses of autophagy and apoptosis to oxidative stress in nucleus pulposus cells: implications for disc degeneration. Cell Physiol Biochem 2014;34:1175-89.
42. Spickett CM. Chlorinated lipids and fatty acids: an emerging role in pathology. Pharmacol Ther 2007;115:400-9.
43. Wang M, Gao Y, Li G, An T. Increased adverse effects during metabolic transformation of short-chain chlorinated paraffins by cytochrome P450: a theoretical insight into 1-chlorodecane. J Hazard Mater 2021;407:124391.
44. Gong Y, Zhang H, Geng N, et al. Short-chain chlorinated paraffins (SCCPs) disrupt hepatic fatty acid metabolism in liver of male rat via interacting with peroxisome proliferator-activated receptor α (PPARα). Ecotoxicol Environ Saf 2019;181:164-71.
Cite This Article
Export citation file: BibTeX | RIS
OAE Style
Darnerud PO. Chlorinated paraffin metabolism and its importance for understanding CP toxicity. J Environ Expo Assess 2024;3:2. http://dx.doi.org/10.20517/jeea.2023.35
AMA Style
Darnerud PO. Chlorinated paraffin metabolism and its importance for understanding CP toxicity. Journal of Environmental Exposure Assessment. 2024; 3(1): 2. http://dx.doi.org/10.20517/jeea.2023.35
Chicago/Turabian Style
Darnerud, Per Ola. 2024. "Chlorinated paraffin metabolism and its importance for understanding CP toxicity" Journal of Environmental Exposure Assessment. 3, no.1: 2. http://dx.doi.org/10.20517/jeea.2023.35
ACS Style
Darnerud, PO. Chlorinated paraffin metabolism and its importance for understanding CP toxicity. J. Environ. Expo. Assess. 2024, 3, 2. http://dx.doi.org/10.20517/jeea.2023.35
About This Article
Copyright
Data & Comments
Data
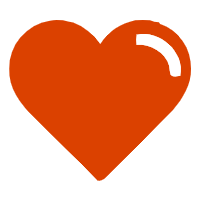

Comments
Comments must be written in English. Spam, offensive content, impersonation, and private information will not be permitted. If any comment is reported and identified as inappropriate content by OAE staff, the comment will be removed without notice. If you have any queries or need any help, please contact us at support@oaepublish.com.