Photoredox/nickel dual-catalyzed reductive C(sp2)–Si cross-coupling
Abstract
Nickel-catalyzed cross-electrophile coupling of carbon-electrophiles and silicon-electrophiles has recently emerged as a powerful tool for C–Si bond formations to synthesize highly valuable organosilanes. This reductive coupling strategy eliminates the manipulation of highly reactive organometallic reagents, thereby leading to a good functional group tolerance and a high step economy. However, the reported reductive C–Si couplings have to use stoichiometric amounts of Zn or Mn dust as a reductant, which somehow limits the synthetic application. Herein, we reported a novel cross-electrophile coupling of aryl halides with chlorosilanes enabled by dual photoredox/nickel catalysis. Instead of using metallic reductants, a mild and readily available α-silylamine is selected as a reliable organic reductant. Various vinyl chlorosilanes and chlorohydrosilanes were coupled smoothly. This new catalytic protocol offers an alternative approach for facile synthesis of organosilanes.
Keywords
INTRODUCTION
Organosilanes are a class of important building blocks in organic synthesis, which also have broad applications in material science and medicinal chemistry[1-5]. Due to the importance of these compounds, substantial efforts have been devoted to the development of efficient methodologies for the synthesis of organosilanes[6-8]. Transition metal-catalyzed C–Si coupling has been developed as a powerful synthetic tool for the preparation of organosilanes [Figure 1][9,10]. Traditional synthesis involves the reaction of silyl electrophiles Si–X (X = Cl, Br, I, etc.) with organometallic reagents C–M (M = Mg, Li, B, etc.)[11-14]
Over the past decade, transition metal-catalyzed cross-electrophile coupling between two electrophiles has received considerable attention[26-29]. This novel and convenient coupling strategy bypasses the pre-preparation and handling of highly reactive organometallic reagents, leading to a high atom- and step-economy and broad functional group tolerance. Cross-electrophile coupling has significantly progressed in organic synthesis for the construction of C–C bonds, while the successful applications of reductive strategy in C–Si coupling are rarely reported[30,31] [Figure 1A(iv)]. Duan et al. demonstrated the first nickel-catalyzed cross-electrophile coupling of vinyl/aryl electrophiles with chlorosilanes using Mn as a reductant[32]. Further endeavors have been devoted to the expansion of substrate types and exploration of new transformations by the researchers[33-38], Zhang and Oestreich[39], and others[40-43]. These attractive syntheses make an important contribution to the area of C–Si coupling. Despite the impressive advancements, the requirement of stoichiometric amounts of metal reductants (Mn or Zn) would hinder the practical application due to the substantial disposal of metal salts. Therefore, exploring a catalytic strategy that uses mild organic reductants instead of metallic reductants in C–Si coupling is meaningful.
Based on our previous studies on metallaphotoredox-catalyzed organic reductant-mediated cross-electrophile coupling reactions[44-48], we reported herein a photoredox/nickel dual-catalyzed reductive C–Si coupling of aryl halides and chlorosilanes by applying silylamine as a potential organic reductant [Figure 1B]. This novel catalytic platform obviates the necessity of metallic reductants and shows good tolerance for both chlorosilanes and chlorohydrosilanes, thus providing a complementary approach to synthesize organosilanes via reductive C–Si coupling.
EXPERIMENTAL
The reactions were set up in a N2-filled glovebox. An oven-dried vial equipped with a stir-bar was added aryl bromide 1a (0.20 mmol, 1.0 equiv.), Ir(ppy)3 (1.3 mg, 2.0 µmol, 0.010 equiv.), 5,5’-diMebpy (7.4 mg,
RESULTS AND DISCUSSION
Based on this design, we initiated our study of the reductive C–Si coupling with methyl 4-bromobenzoate
Optimization of the reaction conditionsa
![]() | ||
Entry | Deviation from the standard condition | Yield (%)b |
1 | None | 64 (62)c |
2 | 4-CzIPN as photocatalyst | 28 |
3 | HE as reductant | 0 |
4 | Et3N as reductant | 0 |
5 | TDAE as reductant | 0 |
6 | 4,4’-dtbbpy as ligand | 37 |
7 | without Ni catalyst | 0 |
8 | without light or photocatalyst | 0 |
With the optimal reaction conditions determined, we then investigated the scope generality of the reductive C–Si coupling. First, the substrate scope of the aryl electrophiles was studied by reactions with 2a [Figure 2]. Aryl electrophiles irrespective of aryl bromides, iodides, and triflates were valid carbon-electrophiles to undergo reductive C–Si coupling, affording the desired coupling products in moderate yields. Aryl halides with different electronic and steric natures of substituents have no significant influence on the reactivity. Not only electron-withdrawing groups (3-8) but also electron-donating groups (9-12) reacted smoothly. Aryl bromides bearing a para-, meta-, or sterically congested ortho-substituted ester group successfully engaged in the reaction to deliver the corresponding products (3-5). The reaction tolerated a variety of functional groups such as CN (6), amide (7), and chloride (8). Particularly noted, the coupling process could even tolerate a boron group, a synthetic versatile functionality for further cross-coupling (13). Besides phenyl bromides, 2-bromonaphthalene and 1-bromonaphthalene were proved to be suitable substrates (15-16). Gratifyingly, heteroaryl bromides such as 5-bromobenzo[d][1,3]dioxole (17) and 3-bromothiophene
Figure 2. Substrate scope of C–Si coupling with respect to aryl electrophiles. Reaction conditions: 1 (0.2 mmol), 2a (0.6 mmol, 3.0 equiv.). Isolated yields.
Furthermore, we turned our attention to investigating the substrate scope of chlorosilanes [Figure 3]. Vinyl chlorosilanes containing different substituents such as chloro(methyl)(phenyl)(vinyl)silane and chlorodiphenyl(vinyl)silane were tolerated well (19-20). Specially, chlorotri(vinyl)silane could be incorporated into the reaction to deliver tri(vinyl)phenylsilane in a good yield (21). Probably due to the enhanced coordination ability of vinylchlorosilane to the nickel catalyst, chlorosilanes without vinyl-substituent have failed to deliver any desired products. Chlorohydrosilanes are a kind of very challenging substrates in transition-metal-catalyzed silylation via selective Si–Cl cleavage as the bond dissociation energy of Si–Cl bond is higher than that of Si–H bond. Zhao et al. reported the first example of nickel-catalyzed reductive C–Si coupling of chlorohydrosilanes via selective Si–Cl bond cleavage in the aid of Mn as reductant[34]. To explore the feasibility of chlorohydrosilanes in our developed photoredox/nickel dual catalytic system, we further optimized the reaction conditions. To our delight, after slightly adjusting the ligand to 3,4,7,8-Me4Phen and using a mixed solvent system, the coupling of chlorodiphenylsilane with different aryl halides could afford triphenylsilanes in moderate yields (22-24).
Figure 3. Substrate scope of C–Si coupling with respect to chlorosilanes. Reaction conditions: 1a (0.2 mmol), 2 (0.6 mmol, 3.0 equiv.). Isolated yields. aMeCN/DMAc: 7:3 (0.1 M), 3,4,7,8-tetramethyl-1,10-phenanthroline (20 mol%) was used. DMAc: N,N-dimethylacetamide.
To demonstrate the scalability of this reaction, Hiyama cross-coupling of product 3 with 2-bromo-5-(trifluoromethyl)pyridine was attempted in the presence of 5 mol% Pd(PPh3)4 and 1.0 equiv. of CuI as a promotor, the reaction proceeded smoothly to get aryl-heteroaryl coupled product 25 in 63% isolated yield [Figure 4A]. In order to gain insight into the mechanism, several control experiments were conducted
Based on the above-performed experiments and previous reports[32,39], a plausible mechanism for the photoredox-assisted nickel-catalyzed reductive C–Si coupling was depicted in Figure 5. Ni0 undergoes an oxidative addition of aryl halide forms a ArNiIIX [Ered NiII/NiI = -1.17 V vs. saturated calomel electrode (SCE)][49], which is reduced by PC* (E1/2 IrIV/*IrIII = -1.73 V vs. SCE)[50] via a single-electron transfer (SET) to get a ArNiI complex, which further oxidized by chlorosilane to form ArNiIII, final reductive elimination of ArNiIII affords C–Si coupling product and generates the NiI, which undergoes a second SET with PC* and regenerates Ni0 catalyst[51-53]. On the other hand, the oxidative state of PC (E1/2 IrIV/IrIII = +0.77 V vs. SCE)[50] is reduced to its ground state by α-silylamine (E1/2ox = +0.71 V vs. SCE)[54] completing both catalytic cycles.
CONCLUSION
In summary, we have reported a synergistic photoredox/nickel catalytic protocol for reductive cross-coupling of aryl electrophiles and chlorosilanes. α-Silylamine is identified as a potent organic reductant to replace metal reductants for reductive C–Si coupling. The reaction tolerances a generally broad scope of chlorosilanes and (hetero)aryl halides. Further studies on mechanistic investigation and synthetic application are underway in this lab.
DECLARATIONS
Authors’ contributions
Performing the experiments and data analysis: Liu, J.
Directing the project and writing the manuscript: Yuan, W.
Availability of data and materials
The raw data supporting the findings of this study are available within this Article and its Supplementary Materials. Further data are available from the corresponding authors upon reasonable request.
Financial support and sponsorship
We thank the National Natural Science Foundation of China (22201087) and Guangdong Basic and Applied Basic Research Foundation (2022A1515012507) for the financial support.
Conflicts of interest
Both authors declared that there are no conflicts of interest.
Ethical approval and consent to participate
Not applicable.
Consent for publication
Not applicable.
Copyright
© The Author(s) 2025.
Supplementary Materials
REFERENCES
1. Ramesh, R.; Reddy, D. S. Quest for novel chemical entities through incorporation of silicon in drug scaffolds. J. Med. Chem. 2018, 61, 3779-98.
2. Sore, H. F.; Galloway, W. R. J. D.; Spring, D. R. Palladium-catalysed cross-coupling of organosilicon reagents. Chem. Soc. Rev. 2012, 41, 1845-66.
3. Franz, A. K.; Wilson, S. O. Organosilicon molecules with medicinal applications. J. Med. Chem. 2013, 56, 388-405.
4. Langkopf, E.; Schinzer, D. Uses of silicon-containing compounds in the synthesis of natural products. Chem. Rev. 1995, 95, 1375-408.
5. Hiyama, T.; Oestreich, M. Organosilicon chemistry: novel approaches and reactions. Wiley-VCH: Weinheim; 2019.
6. Li, B.; Dixneuf, P. H. Metal-catalyzed silylation of sp3C-H bonds. Chem. Soc. Rev. 2021, 50, 5062-85.
7. Du, X.; Huang, Z. Advances in base-metal-catalyzed alkene hydrosilylation. ACS. Catal. 2017, 7, 1227-43.
8. Richter, S. C.; Oestreich, M. Emerging strategies for C–H silylation. Trends. Chem. 2020, 2, 13-27.
10. Korch, K. M.; Watson, D. A. Cross-coupling of heteroatomic electrophiles. Chem. Rev. 2019, 119, 8192-228.
11. Murakami, K.; Hirano, K.; Yorimitsu, H.; Oshima, K. Silver-catalyzed transmetalation between chlorosilanes and aryl and alkenyl Grignard reagents for the synthesis of tetraorganosilanes. Angew. Chem. Int. Ed. Engl. 2008, 47, 5833-5.
12. Cinderella, A. P.; Vulovic, B.; Watson, D. A. Palladium-catalyzed cross-coupling of silyl electrophiles with alkylzinc halides: a silyl-negishi reaction. J. Am. Chem. Soc. 2017, 139, 7741-4.
13. Vulovic, B.; Cinderella, A. P.; Watson, D. A. Palladium-catalyzed cross-coupling of monochlorosilanes and grignard reagents. ACS. Catal. 2017, 7, 8113-7.
14. Naganawa, Y.; Guo, H.; Sakamoto, K.; Nakajima, Y. Nickel-catalyzed selective cross-coupling of chlorosilanes with organoaluminum reagents. ChemCatChem 2019, 11, 3756-9.
15. Chu, C. K.; Liang, Y.; Fu, G. C. Silicon-carbon bond formation via nickel-catalyzed cross-coupling of silicon nucleophiles with unactivated secondary and tertiary alkyl electrophiles. J. Am. Chem. Soc. 2016, 138, 6404-7.
16. Xue, W.; Qu, Z. W.; Grimme, S.; Oestreich, M. Copper-catalyzed cross-coupling of silicon pronucleophiles with unactivated alkyl electrophiles coupled with radical cyclization. J. Am. Chem. Soc. 2016, 138, 14222-5.
17. Xue, W.; Shishido, R.; Oestreich, M. Bench-stable stock solutions of silicon grignard reagents: application to iron- and cobalt-catalyzed radical C(sp3)-Si cross-coupling reactions. Angew. Chem. Int. Ed. Engl. 2018, 57, 12141-5.
18. Zarate, C.; Nakajima, M.; Martin, R. A mild and ligand-free Ni-catalyzed silylation via C-OMe cleavage. J. Am. Chem. Soc. 2017, 139, 1191-7.
19. Yamanoi, Y.; Taira, T.; Sato, J.; Nakamula, I.; Nishihara, H. Efficient preparation of monohydrosilanes using palladium-catalyzed Si-C bond formation. Org. Lett. 2007, 9, 4543-6.
20. Hamze, A.; Provot, O.; Alami, M.; Brion, J. D. Platinum oxide catalyzed silylation of aryl halides with triethylsilane: an efficient synthetic route to functionalized aryltriethylsilanes. Org. Lett. 2006, 8, 931-4.
21. Yuan, W.; Smirnov, P.; Oestreich, M. Custom hydrosilane synthesis based on monosilane. Chem 2018, 4, 1443-50.
22. Yuan, W.; Orecchia, P.; Oestreich, M. Palladium-catalyzed three-component reaction of dihydrosilanes and vinyl iodides in the presence of alcohols: rapid assembly of silyl ethers of tertiary silanes. Chem. Eur. J. 2018, 24, 19175-8.
23. Sun, H.; Cheng, Y.; Teng, H.; et al. 3-Alkyl-2-pyridyl directing group-enabled C2 selective C-H silylation of indoles and pyrroles via an iridium catalyst. J. Org. Chem. 2022, 87, 13346-51.
24. Liu, S.; Robert, F.; Landais, Y. Dual photoredox nickel-catalyzed silylation of aryl/heteroaryl bromides using hydrosilanes. Chem. Commun. 2023, 59, 11369-72.
25. Neil, B.; Lucien, F.; Fensterbank, L.; Chauvier, C. Transition-metal-free silylation of unactivated C(sp2)–H bonds with tert-butyl-substituted silyldiazenes. ACS. Catal. 2021, 11, 13085-90.
26. Weix, D. J. Methods and mechanisms for cross-electrophile coupling of Csp2 halides with alkyl electrophiles. Acc. Chem. Res. 2015, 48, 1767-75.
27. Gu, J.; Wang, X.; Xue, W.; Gong, H. Nickel-catalyzed reductive coupling of alkyl halides with other electrophiles: concept and mechanistic considerations. Org. Chem. Front. 2015, 2, 1411-21.
28. Yi, L.; Ji, T.; Chen, K.; Chen, X.; Rueping, M. Nickel-catalyzed reductive cross-couplings: new opportunities for carbon–carbon bond formations through photochemistry and electrochemistry. CCS. Chem. 2022, 4, 9-30.
29. Qian, P.; Guan, H.; Wang, Y. E.; et al. Catalytic enantioselective reductive domino alkyl arylation of acrylates via nickel/photoredox catalysis. Nat. Commun. 2021, 12, 6613.
30. Yang, Y.; Pang, X.; Shu, X. Transition-metal-catalyzed cross-coupling of chlorosilanes. Synthesis 2023, 55, 868-76.
31. Pang, X.; Shu, X. Z. Nickel-catalyzed reductive coupling of chlorosilanes. Chem. Eur. J. 2023, 29, e202203362.
32. Duan, J.; Wang, K.; Xu, G. L.; et al. Cross-electrophile C(sp2)-Si coupling of vinyl chlorosilanes. Angew. Chem. Int. Ed. Engl. 2020, 59, 23083-8.
33. Pan, Q. Q.; Qi, L.; Pang, X.; Shu, X. Z. Nickel-catalyzed cross-electrophile 1,2-silyl-arylation of 1,3-dienes with chlorosilanes and aryl bromides. Angew. Chem. Int. Ed. Engl. 2023, 62, e202215703.
34. Zhao, Z. Z.; Pang, X.; Wei, X. X.; Liu, X. Y.; Shu, X. Z. Nickel-catalyzed reductive C(sp2)-Si coupling of chlorohydrosilanes via Si-Cl cleavage. Angew. Chem. Int. Ed. Engl. 2022, 61, e202200215.
35. Qi, L.; Pan, Q. Q.; Wei, X. X.; Pang, X.; Liu, Z.; Shu, X. Z. Nickel-catalyzed reductive [4 + 1] sila-cycloaddition of 1,3-dienes with dichlorosilanes. J. Am. Chem. Soc. 2023, 145, 13008-14.
36. Duan, J.; Wang, Y.; Qi, L.; Guo, P.; Pang, X.; Shu, X. Z. Nickel-catalyzed cross-electrophile C(sp3)-Si coupling of unactivated alkyl bromides with vinyl chlorosilanes. Org. Lett. 2021, 23, 7855-9.
37. Qi, L.; Pang, X.; Yin, K.; Pan, Q.; Wei, X.; Shu, X. Mn-mediated reductive C(sp3)–Si coupling of activated secondary alkyl bromides with chlorosilanes. Chin. Chem. Lett. 2022, 33, 5061-4.
38. Pang, X.; Su, P. F.; Shu, X. Z. Reductive cross-coupling of unreactive electrophiles. Acc. Chem. Res. 2022, 55, 2491-509.
39. Zhang, L.; Oestreich, M. Nickel-catalyzed, reductive C(sp3)-Si cross-coupling of α-cyano alkyl electrophiles and chlorosilanes. Angew. Chem. Int. Ed. Engl. 2021, 60, 18587-90.
40. Xing, M.; Cui, H.; Zhang, C. Nickel-catalyzed reductive cross-coupling of alkyl bromides and chlorosilanes. Org. Lett. 2021, 23, 7645-9.
41. Sun, J.; Zhou, Y.; Gu, R.; Li, X.; Liu, A.; Zhang, X. Regioselective Ni-catalyzed reductive alkylsilylation of acrylonitrile with unactivated alkyl bromides and chlorosilanes. Nat. Commun. 2022, 13, 7093.
42. Xing, D.; Liu, J.; Cai, D.; Huang, B.; Jiang, H.; Huang, L. Cobalt-catalyzed cross-electrophile coupling of alkynyl sulfides with unactivated chlorosilanes. Nat. Commun. 2024, 15, 4502.
43. Li, C.; Yang, S.; Zeng, X. Cross-electrophile silylation of aryl carboxylic esters with hydrochlorosilanes by SiH-directed and Cr-catalyzed couplings. ACS. Catal. 2023, 13, 12062-73.
44. Zhao, H.; Yuan, W. Three-component reductive conjugate addition/aldol tandem reaction enabled by nickel/photoredox dual catalysis. Chem. Sci. 2023, 14, 1485-90.
45. Liu, J.; Chen, Y.; Zhao, H.; Yuan, W. Nickel/photoredox dual-catalyzed reductive cross-coupling of aryl halides and aldehydes. Org. Chem. Front. 2024, 11, 1205-10.
46. Xi, X.; Luo, Y.; Li, W.; et al. From esters to ketones via a photoredox-assisted reductive acyl cross-coupling strategy. Angew. Chem. Int. Ed. Engl. 2022, 61, e202114731.
47. Xi, X.; Chen, Y.; Yuan, W. Nickel-catalyzed three-component alkylacylation of alkenes enabled by a photoactive electron donor-acceptor complex. Org. Lett. 2022, 24, 3938-43.
48. Chen, Y.; Xi, X.; Yuan, W. Photoinduced nickel-catalyzed reductive acyl cross-coupling: facile access to all carbon quaternary aliphatic ketones. Org. Chem. Front. 2023, 10, 3669-75.
49. Shields, B. J.; Doyle, A. G. Direct C(sp3)-H cross coupling enabled by catalytic generation of chlorine radicals. J. Am. Chem. Soc. 2016, 138, 12719-22.
50. Prier, C. K.; Rankic, D. A.; MacMillan, D. W. Visible light photoredox catalysis with transition metal complexes: applications in organic synthesis. Chem. Rev. 2013, 113, 5322-63.
51. Zhang, P.; Le, C. C.; MacMillan, D. W. Silyl radical activation of alkyl halides in metallaphotoredox catalysis: a unique pathway for cross-electrophile coupling. J. Am. Chem. Soc. 2016, 138, 8084-7.
52. Cong, F.; Lv, X. Y.; Day, C. S.; Martin, R. Dual catalytic strategy for forging sp2-sp3 and sp3-sp3 architectures via β-scission of aliphatic alcohol derivatives. J. Am. Chem. Soc. 2020, 142, 20594-9.
53. Yi, J.; Badir, S. O.; Kammer, L. M.; Ribagorda, M.; Molander, G. A. Deaminative reductive arylation enabled by nickel/photoredox dual catalysis. Org. Lett. 2019, 21, 3346-51.
Cite This Article
How to Cite
Download Citation
Export Citation File:
Type of Import
Tips on Downloading Citation
Citation Manager File Format
Type of Import
Direct Import: When the Direct Import option is selected (the default state), a dialogue box will give you the option to Save or Open the downloaded citation data. Choosing Open will either launch your citation manager or give you a choice of applications with which to use the metadata. The Save option saves the file locally for later use.
Indirect Import: When the Indirect Import option is selected, the metadata is displayed and may be copied and pasted as needed.
About This Article
Copyright
Data & Comments
Data
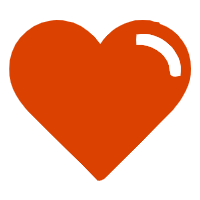
Comments
Comments must be written in English. Spam, offensive content, impersonation, and private information will not be permitted. If any comment is reported and identified as inappropriate content by OAE staff, the comment will be removed without notice. If you have any queries or need any help, please contact us at [email protected].