Revolutionizing fiber batteries with polymer gel electrolyte: a groundbreaking innovation in wearable energy
Keywords
The advent of flexible and wearable electronic devices heralds a transformative era in consumer electronics and personalized health monitoring. As these devices become increasingly integrated into our daily lives, there is a pressing need for the development of energy storage or conversion systems with the merits of high performance, small size, and adaptability to frequent deformation during usage. Among these power sources (such as batteries, supercapacitors, fuel cells, and solar cells)[1,2], batteries, with lithium-ion batteries being a prime example, have been known for several decades as devices that convert stored chemical energy directly into electricity[3,4]. To date, they are among the most widely used power supplies for electronic devices and have become an integral part of modern electronics. However, for the booming smart textiles, integrating batteries into textiles remains a critical challenge. Traditional rigid batteries are rigid and unsafe; they are not suitable for the inherently soft and flexible nature of smart textile technology[5].
Fiber lithium-ion batteries (FLBs) stand out for their unique benefits, particularly in the context of wearable technology and energy textiles. Their compatibility with the textile industry is a key advantage, as they can be seamlessly integrated into fabrics as functional yarns, enhancing the versatility of energy textiles. Additionally, these batteries offer improved air permeability compared to conventional planar batteries, which are often impermeable and resemble leather. This feature addresses a significant limitation in current battery design. Furthermore, the inherent flexibility and miniaturization potential of fiber-shaped batteries enable the creation of diverse, flexible power sources that can be tailored to various applications, providing unmatched adaptability and innovation potential in the field of energy storage. Through an optimized scalable industrial process, meters of FLBs have been produced in a continuous way[6]. From the perspective of practical application, there are still great requirements and challenges for batteries, which mainly include: (1) the difficulty in controlling the flexibility to ensure wear comfort while achieving stable electrochemical properties, especially during battery deformation; (2) and increased safety hazard, as these devices are subjected to more frequent dynamic deformation during use, raising the possibility of mechanical damage.
To enhance the safety profile and facilitate practical deployment of FLBs, researchers have explored the use of gel electrolytes as a substitute for traditional liquid electrolytes[5,7-10]. However, this substitution has been met with challenges. With an absence of chemical bonding to current collectors, gel electrolytes exhibit limited infiltration into the electrode materials, which leads to a suboptimal gel electrolyte-electrode interface. This issue significantly impacts the energy density of FLBs, often resulting in values well below the desired threshold, typically less than 10 Wh·kg-1.
The challenge for achieving reliable electrochemical performance lies in the inconsistent interface contact between the polymer gel electrolytes and the fiber electrodes, particularly under dynamic deformation. To address these challenging issues, building on their previous achievements on the assembly of FLBs[11-13], Lu
As shown in Figure 1, the small active particles are firstly deposited onto thin fiber current collectors and followed by larger ones, which results in the formation of a layered network of inner small and outer large channels among the particles. By rotating multiple cathode and anode fibers in conjunction with separators and introducing a monomer solution into these aligned and interconnected channels, robust and efficient gel electrolyte-electrode interfaces were produced after subsequent polymerization.
Figure 1. (A) Schematic illustration; (B) cross-sectional SEM image and (C) EDS elemental mapping of an FLB. The Active particles and networked channels on the cathode and anode fiber surface can be observed[14]. Credit: Springer Nature. SEM: Scanning electron microscopy; EDS: energy-dispersive X-ray spectroscopy; FLB: fiber lithium-ion battery.
Such aligned channels have been shown to markedly expedite the permeation of electrolytes, a critical factor in optimizing energy storage device performance. Specifically, the internal micro-architecture, composed of smaller particles, adeptly facilitates electrolyte collection along the sinuous profiles of fibers, thereby diminishing interfacial impedance and hastening ion intercalation during high-rate discharge. In stark contrast, the larger particles on the exterior surface exhibit enhanced reversibility in the lithium-ion intercalation-deintercalation process. This characteristic is paramount for achieving elevated Coulombic efficiency and a notable increase in specific capacity, thereby reinforcing the role of particle size stratification in the advancement of electrochemical properties.
Concurrently, the larger particles on the outer layers enhance the battery’s Coulombic efficiency and contribute to a high specific capacity output. As a result, The FLBs showed a high energy density of up to about 128 Wh·kg-1 and maintained high capacity even under a high production rate of 3,600 m·h-1. It is also critical to highlight that, in addition to the impressive electrochemical properties, their devices also demonstrated great stability and safety under various test conditions. Owing to the stable electrolyte-electrode interface, the FLB demonstrated remarkable flexibility and stability, withstanding an impressive 100,000 bending cycles. It also exhibited resilience in extreme conditions, such as operating temperatures ranging from 0 to 80 °C, underwater environments, exposure to ultraviolet light, and even functioning in a vacuum at -0.08 MPa. These robust characteristics show a great performance improvement [Table 1], and indicate that FLBs possess considerable potential for a variety of demanding applications, including firefighting and space exploration.
Performance comparison of different FLBs
Fiber battery | Specific capacity | Energy density | Stability assessment | Ref. |
FLBs (2021) | Between 160 and 180 mAh·g-1 at 0.1 C | 85.69 Wh·kg-1 | 93% capacity retention at 1 Crate; > 80% capacity at 100,000 bending cycles; strong environmental adaptability (-20 to 60 °C) | [6] |
FLBs (2022) | 86 mAh·g-1 at 50 mA·g-1 | 550 mWh·m-2 | Stable performance after 10,000 bending cycles; stable performance after bending, knotting, and twisting heat resistant (300 °C); good environmental adaptability (workable after puncture) | [11] |
FLBs (2023) | 14.97 mAh | 62 Wh·kg-1 | 85.8% capacity retention after 10,000 bending cycles | [15] |
FLBs (2023) | Linear specific capacity, 0.26 mWh·cm-1 | 106.85 mWh·cm-3 | 94.8%/1 C/1,000 bending cycles, good durability | [16] |
FLBs (2024) | 170 mAh·g-1 | 128 Wh·kg-1 | Stable performance after 100,000 deformation cycles; stable performance in 40-80 °C environments | [14] |
In summary, this work by Lu et al. provides an unprecedented strategy for closely and stably interfacing polymer gel electrolytes with electrode fibers, offering advantages such as higher power and energy densities, improved productivity and significantly enhanced safety for FLBs[14]. Their groundbreaking discovery propels the field of wearable battery technology forward, which opens up new possibilities for flexible electronics, biomedical engineering, space exploration, and wearable technology, marking a significant stride in the evolution of portable and adaptable energy solutions.
Despite significant advancements in FLBs, further enhancement in battery performance, flexibility, and assembly remains crucial for various application fields, such as wearable medical equipment or aerospace flexible energy systems. Building on this foundation, several in-depth strategies for electrolyte improvement have been proposed and thoroughly discussed:
(1) High performance is fundamental for battery applications; thus, developing lightweight, and electrochemically superior active materials is vital.
(2) Fibers are the building blocks of textiles to provide a soft and comfortable feel. Despite this, fiber-shaped batteries often lack the softness of natural fibers, exhibiting a texture more similar to plastic threads.
(3) Fabric is the key medium for FLB applications, yet seamlessly incorporating FLBs into breathable energy textiles presents a notable challenge under current technological constraints.
DECLARATIONS
Authors’ contributions
Wrote and revised the manuscript: Wang H, Yan W, Zhu M
Availability of data and materials
Not applicable.
Financial support and sponsorship
This work is financially supported by the Fundamental Research Funds for the Central Universities (Grant No. 2232024D-02), Science and Technology Commission of Shanghai Municipality (Grant No. 20JC1414900), the National Natural Science Foundation of China (Grant No. 52127805 and Grant No. 52202167), the National Key Research and Development Program of China (Grant No. 2021YFA1201300), and the Chenguang Program of Shanghai Education Development Foundation and Shanghai Municipal Education Commission (23CGA41).
Conflicts of interest
All author declared that there are no conflicts of interest.
Ethical approval and consent to participate
Not applicable.
Consent for publication
Not applicable.
Copyright
© The Author(s) 2024.
REFERENCES
1. Chen C, Feng J, Li J, Guo Y, Shi X, Peng H. Functional fiber materials to smart fiber devices. Chem Rev 2023;123:613-62.
2. Dang C, Wang Z, Hughes-Riley T, et al. Fibres-threads of intelligence-enable a new generation of wearable systems. Chem Soc Rev 2024;53:8790-846.
3. Mizushima K, Jones PC, Wiseman PJ, Goodenough JB. LixCoO2 (0 < x < -1): a new cathode material for batteries of high energy density. Mater Res Bull 1980;15:783-9.
5. Sumboja A, Liu J, Zheng WG, Zong Y, Zhang H, Liu Z. Electrochemical energy storage devices for wearable technology: a rationale for materials selection and cell design. Chem Soc Rev 2018;47:5919-45.
6. He J, Lu C, Jiang H, et al. Scalable production of high-performing woven lithium-ion fibre batteries. Nature 2021;597:57-63.
7. Shi C, Takeuchi S, Alexander GV, et al. High sulfur loading and capacity retention in bilayer garnet sulfurized‐polyacrylonitrile/lithium‐metal batteries with gel polymer electrolytes. Adv Energy Mater 2023;13:2301656.
8. Ren J, Zhang Y, Bai W, et al. Elastic and wearable wire-shaped lithium-ion battery with high electrochemical performance. Angew Chem Int Ed Engl 2014;53:7864-9.
9. Rao J, Liu N, Zhang Z, et al. All-fiber-based quasi-solid-state lithium-ion battery towards wearable electronic devices with outstanding flexibility and self-healing ability. Nano Energy 2018;51:425-33.
10. Khudiyev T, Grena B, Loke G, et al. Thermally drawn rechargeable battery fiber enables pervasive power. Mater Today 2022;52:80-9.
11. Liao M, Wang C, Hong Y, et al. Industrial scale production of fibre batteries by a solution-extrusion method. Nat Nanotechnol 2022;17:372-7.
12. Zeng K, Shi X, Tang C, Liu T, Peng H. Design, fabrication and assembly considerations for electronic systems made of fibre devices. Nat Rev Mater 2023;8:552-61.
13. Zhang Y, Wang L, Guo Z, Xu Y, Wang Y, Peng H. High-performance lithium-air battery with a coaxial-fiber architecture. Angew Chem Int Ed Engl 2016;55:4487-91.
14. Lu C, Jiang H, Cheng X, et al. High-performance fibre battery with polymer gel electrolyte. Nature 2024;629:86-91.
15. Huang X, Wang C, Li C, et al. Braided fiber current collectors for high-energy-density fiber lithium-ion batteries. Angew Chem Int Ed Engl 2023;62:e202303616.
Cite This Article

How to Cite
Wang, H.; Yan W.; Zhu M. Revolutionizing fiber batteries with polymer gel electrolyte: a groundbreaking innovation in wearable energy. Chem. Synth. 2024, 4, 57. http://dx.doi.org/10.20517/cs.2024.67
Download Citation
Export Citation File:
Type of Import
Tips on Downloading Citation
Citation Manager File Format
Type of Import
Direct Import: When the Direct Import option is selected (the default state), a dialogue box will give you the option to Save or Open the downloaded citation data. Choosing Open will either launch your citation manager or give you a choice of applications with which to use the metadata. The Save option saves the file locally for later use.
Indirect Import: When the Indirect Import option is selected, the metadata is displayed and may be copied and pasted as needed.
About This Article
Copyright
Data & Comments
Data
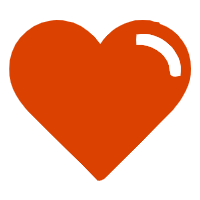
Comments
Comments must be written in English. Spam, offensive content, impersonation, and private information will not be permitted. If any comment is reported and identified as inappropriate content by OAE staff, the comment will be removed without notice. If you have any queries or need any help, please contact us at support@oaepublish.com.