Ultrafast S-scheme interfacial electron transport enhances CO2 photoreduction
Keywords
The increasing gap between CO2 emissions from human activities and the carbon capture and sequestration capabilities of natural ecosystems has intensified the focus on achieving global net-zero carbon emissions[1-3]. Photocatalytic CO2 reduction, which transforms CO2 into high-value-added fuels or chemicals, presents a promising strategy for reaching carbon neutrality[4-6]. However, challenges associated with photocatalysts, such as limitations in light absorption, rapid recombination of photogenerated carriers, and kinetic barriers, significantly hinder CO2 photoconversion efficiency[7]. As a result, the development of efficient photocatalysts to improve CO2 reduction efficiency has become a key research priority in the field of photocatalysis[8,9].
Among various photocatalyst design strategies, S-scheme heterojunctions composed of reduction and oxidation semiconductors have gained significant attention[10,11]. Compared with single-component photocatalysts and type-II heterojunctions, S-scheme heterojunctions not only facilitate efficient separation of photogenerated carriers but also enhance redox capacity, providing a distinct advantage for CO2 photoreduction[12-14]. However, low-quality interfaces in these heterojunctions often impede efficient interfacial electron transport, thereby limiting overall photocatalytic efficiency.
To address this issue, Deng et al. recently developed an S-scheme In2O3/Nb2O5 heterojunction photocatalyst, denoted as INx[15]. In this designation, “I” stands for In2O3, “N” represents Nb2O5, and “x” indicates the weight percentage of Nb2O5 relative to In2O3. This catalyst was prepared using a one-step high-temperature calcination process after electrospinning the precursor into fibers [Figure 1A]. Unlike conventional two- or multi-step synthesis strategies, this “one-pot” approach allowed for the simultaneous synthesis of In2O3 and Nb2O5, ensuring optimal contact between the two components. Transmission electron microscopy (TEM) images revealed that the close contact between In2O3 and Nb2O5 created an extensive two-phase interface, significantly enhancing carrier transport efficiency [Figure 1B-D]. This design strategy created a high-quality carrier transport channel, facilitating efficient interfacial charge transfer.
Figure 1. (A) Schematic illustration and design concept of the study; (B) Field emission scanning electron microscopy image and energy-dispersive X-ray spectrum; (C) TEM image; and (D) high-resolution TEM images of the In2O3/Nb2O5 heterojunctions (IN10). This figure is quoted with permission from Deng et al.[15]. OP: Oxidation photocatalysts; RP: reduction photocatalysts; fs-TAS: femtosecond transient absorption spectroscopy.
Femtosecond transient absorption spectroscopy indicated that the ground-state bleach (GSB) signal of In2O3 is attributed to photogenerated electrons[Figure 2], as confirmed by using AgNO3 as an electron scavenger. In pristine In2O3, photogenerated electrons undergo interband diffusion and shallow trap state capture
Figure 2. Charge carrier dynamic decay for (A) pure In2O3 in Ar; (B) IN5 in Ar; (C) IN10 in Ar; and (D) IN10 in CO2; schematic illustrations of the decay pathways of photogenerated electrons in (E) pure In2O3; (F) In2O3/Nb2O5 heterojunctions in Ar; and (G) In2O3/Nb2O5 heterojunctions in CO2. This figure is quoted with permission from Deng et al.[15].
In contrast, the GSB signal of Nb2O5 was attributed to photogenerated holes[Figure 3], as verified by using lactic acid as a hole scavenger. In Nb2O5, photogenerated holes participate in recombination with both intrinsic photogenerated electrons and electrons transferred from In2O3 [Figure 3A and E]. Under an argon atmosphere, the half-life of photogenerated holes in Nb2O5 within the In2O3/Nb2O5 heterojunction is shorter than that in pristine Nb2O5 due to rapid hole consumption by ultrafast interfacial electron transfer
Figure 3. Charge carrier dynamic decay for (A) pure Nb2O5 in Ar; (B) IN20 in Ar; (C) IN10 in Ar; and (D) IN10 in CO2; schematic illustrations of the decay pathways of photogenerated holes in (E) pure Nb2O5; (F) In2O3/Nb2O5 heterojunctions in Ar; and (G) In2O3/Nb2O5 heterojunctions in CO2. This figure is quoted with permission from Deng et al.[15].
Photocatalytic CO2 reduction experiments demonstrated that the primary product across all samples was CO, exhibiting nearly 100% selectivity [Figure 4A]. Pure In2O3 and Nb2O5 displayed poor photocatalytic performance due to the rapid recombination of photogenerated carriers inherent to single-phase photocatalysts. However, the formation of S-scheme heterojunctions significantly enhanced CO yield, achieving a maximum CO2-to-CO conversion activity of 0.21 mmol·gactive sites-1·h-1 for IN10. Furthermore, the significance of close interfacial contact between the two phases in promoting ultrafast interfacial electron transfer within the S-scheme heterojunction was validated by comparing the CO2 photoreduction activities of IN10, In2O3/Nb2O5 nanohybrids synthesized via the conventional impregnation-calcination method, and physical mixtures of In2O3 and Nb2O5 [Figure 4B]. Additionally, isotopically labeled experiments revealed three mass spectral signals at m/z = 13, 16, and 29, corresponding to the fragments of 13C, O, and 13CO, respectively [Figure 4C]. These findings confirm the absence of interference from exogenous carbon sources in the origin of the products.
Figure 4. (A) Production yields and CO selectivity over In2O3, INx, and Nb2O5; (B) Comparison of CO2 photoreduction performance among various In2O3/Nb2O5 composites; (C) Total ion chromatography and corresponding mass spectra of products from the photocatalytic reduction of 13CO2 over IN10. RT denotes retention time. This figure is quoted with permission from Deng et al.[15].
In summary, this work has significantly enhanced CO2 photoreduction efficiency by developing an S-scheme In2O3/Nb2O5 heterojunction photocatalyst with high-quality interfacial transport channels. It underscores the critical role of high-quality heterojunction interfaces in achieving ultrafast carrier transport and provides valuable insights for the design of future heterojunction photocatalysts.
DECLARATIONS
Authors’ contributions
Wrote the draft manuscript: Yang J
Revised and rewrote the manuscript: Bie C
Availability of data and materials
Not applicable.
Financial support and sponsorship
This work was supported by the National Natural Science Foundation of China (22202187), the National Postdoctoral Program for Innovative Talents (BX2021275), the Project funded by China Postdoctoral Science Foundation (2022M712957), and the Postdoctoral Funding Program of Hubei Province. Chuanbiao Bie acknowledges financial support from the China Scholarship Council.
Conflicts of interest
All authors declared that there are no conflicts of interest.
Ethical approval and consent to participate
Not applicable.
Consent for publication
Not applicable.
Copyright
© The Author(s) 2024.
REFERENCES
1. Tang J, Weiss E, Shao Z. Advances in cutting-edge electrode engineering toward CO2 electrolysis at high current density and selectivity: a mini-review. Carbon Neutralization 2022;1:140-58.
2. Bie C, Meng Z, He B, Cheng B, Liu G, Zhu B. Exploring photogenerated charge carrier transfer in semiconductor/metal junctions using Kelvin probe force microscopy. J Mater Sci Technol 2024;173:11-9.
3. Yin Y, Kang X, Han B. Two-dimensional materials: synthesis and applications in the electro-reduction of carbon dioxide. Chem Synth 2022;2:19.
4. Xie F, Bie C, Sun J, Zhang Z, Zhu B. A DFT study on Pt single atom loaded COF for efficient photocatalytic CO2 reduction. J Mater Sci Technol 2024;170:87-94.
5. Wu D, Tian C, Zhou J, et al. Morphology and structure of lead-free CuSb-based double perovskites for photocatalytic CO2 reduction. Carbon Neutralization 2022;1:298-305.
6. Bie C, Zhang L, Yu J. Graphene oxide-based photocatalysts for CO2 reduction. In: Yu J, Zhang L, Kuang P, editors. Graphene oxide-metal oxide and other graphene oxide-based composites in photocatalysis and electrocatalysis. Elsevier; 2022. pp. 93-134.
7. Hiragond CB, Powar NS, Kim H, In S. Unlocking solar energy: photocatalysts design for tuning the CO2 conversion into high-value (C2+) solar fuels. EnergyChem 2024;6:100130.
8. Vuong H, Nguyen D, Phuong LP, Minh PPD, Ho BN, Nguyen HA. Nitrogen-rich graphitic carbon nitride (g-C3N5): emerging low-bandgap materials for photocatalysis. Carbon Neutralization 2023;2:425-57.
9. Li Y, Zhang D, Qiao W, et al. Nanostructured heterogeneous photocatalyst materials for green synthesis of valuable chemicals. Chem Synth 2022;2:9.
10. Bie C, Yu J. Application of S -scheme heterojunction photocatalyst. In: Wang X, Anpo M, Fu X, editors. UV-visible photocatalysis for clean energy production and pollution remediation. Wiley; 2023. pp. 41-58.
11. Meng K, Zhang J, Cheng B, et al. Plasmonic near-infrared-response S-scheme ZnO/CuInS2 photocatalyst for H2O2 production coupled with glycerin oxidation. Adv Mater 2024;36:e2406460.
12. Li F, Yue X, Liao Y, Qiao L, Lv K, Xiang Q. Understanding the unique S-scheme charge migration in triazine/heptazine crystalline carbon nitride homojunction. Nat Commun 2023;14:3901.
Cite This Article

How to Cite
Yang, J.; Bie, C. Ultrafast S-scheme interfacial electron transport enhances CO2 photoreduction. Chem. Synth. 2024, 4, 68. http://dx.doi.org/10.20517/cs.2024.105
Download Citation
Export Citation File:
Type of Import
Tips on Downloading Citation
Citation Manager File Format
Type of Import
Direct Import: When the Direct Import option is selected (the default state), a dialogue box will give you the option to Save or Open the downloaded citation data. Choosing Open will either launch your citation manager or give you a choice of applications with which to use the metadata. The Save option saves the file locally for later use.
Indirect Import: When the Indirect Import option is selected, the metadata is displayed and may be copied and pasted as needed.
About This Article
Copyright
Author Biographies
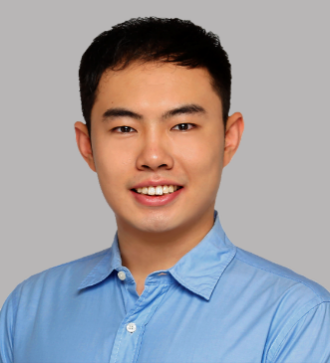
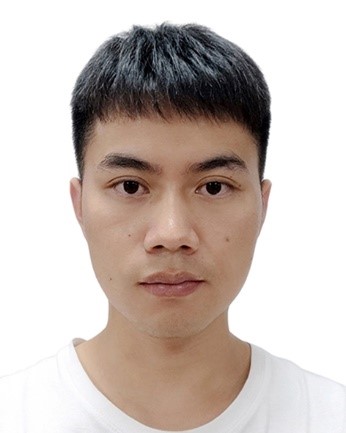
Data & Comments
Data
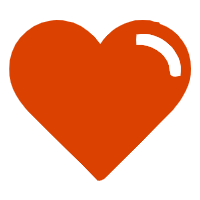
Comments
Comments must be written in English. Spam, offensive content, impersonation, and private information will not be permitted. If any comment is reported and identified as inappropriate content by OAE staff, the comment will be removed without notice. If you have any queries or need any help, please contact us at support@oaepublish.com.