The role of circRNAs and miRNAs in drug resistance and targeted therapy responses in breast cancer
Abstract
MicroRNAs (miRNAs) are small non-coding RNAs comprising 19-24 nucleotides that indirectly control gene expression. In contrast to other non-coding RNAs (ncRNAs), circular RNAs (circRNAs) are defined by their covalently closed loops, forming covalent bonds between the 3’ and 5’ ends. circRNAs regulate gene expression by interacting with miRNAs at transcriptional or post-transcriptional levels. Accordingly, circRNAs and miRNAs control many biological events related to cancer, including cell proliferation, metabolism, cell cycle, and apoptosis. Both circRNAs and miRNAs are involved in the pathogenesis of diseases, such as breast cancer. This review focuses on the latest discoveries on dysregulated circRNAs and miRNAs related to breast cancer, highlighting their potential as biomarkers for clinical diagnosis, prognosis, and chemotherapy response.
Keywords
INTRODUCTION
Breast cancer (BC) is the most common cancer in women worldwide and the leading cause of cancer-related death. In 2022, nearly 2.3 million new cases of BC were diagnosed globally[1]. There are many factors that affect the incidence of BC, including age, early menarche, late menopause, hormone-replacement therapy, lifestyle factors (e.g., alcohol intake and physical inactivity), BRCA1 and BRCA2 mutations, and so on. The signaling pathways recognized to be involved in BC are steroid hormone signaling, human epidermal growth factor receptor 2 (HER2/ERBB2) signaling pathway, cyclin D1/cyclin-dependent kinase
miRNAs are 19-24-nucleotide-long non-coding RNAs (ncRNAs) that are processed from hairpin precursors[5]. These small non-coding RNAs (snRNAs) are pivotal in regulating gene expression by operating at the post-transcriptional level to impact protein synthesis[6,7]. They mediate the silencing process of approximately 30% of coding genes after transcription[8]. miRNAs widely participate in regulating important biological processes such as proliferation, apoptosis, differentiation, immune response, and maintenance of cell or tissue specificity[9,10]. miRNAs can be used as biomarkers for cancer diagnosis and the prediction of curative effects[11,12].
circRNAs, a subclass of ncRNAs, constitute a covalently closed circular structure in eukaryotes. They are transcribed from protein-coding genes and undergo an unconventional precursor messenger RNA
This review highlights the latest discoveries related to circRNAs and miRNAs associated with BC, emphasizing their potential as biomarkers for clinical diagnosis, prognosis, and response to chemotherapy. By comprehensively exploring the roles of circRNAs and miRNAs in BC, we aim to deepen our understanding of this disease and provide insights for improving diagnostic and therapeutic strategies.
BIOGENESIS AND FUNCTIONAL MECHANISM OF MIRNAS AND CIRCRNAS
miRNA molecule belongs to a kind of snRNA encoded by eukaryotic genomic DNA, which is evolutionarily highly conserved. The biosynthesis of miRNA is catalyzed by multiple enzymes in different cell compartments, which is a complicated process. Initially, miRNA is transcribed from DNA into primary miRNA (pri-miRNA) transcripts by RNA polymerase II or III in the cell nucleus. Afterwards, pri-miRNA undergoes cleavage by the microprocessor complex, comprising RNAse III Drosha and its cofactor DiGeorge syndrome critical region 8 (DGCR8), resulting in the formation of a precursor miRNA
Figure 1. Biogenesis and functional mechanism of miRNA. MiRNA is transcribed from DNA into primary miRNA (pri-miRNA) transcripts by RNA polymerase II or III in the cell nucleus. Subsequently, pri-miRNA is cleaved into precursor miRNA (pre-miRNA). Under the regulation of RanGTP protein, pre-miRNA is transported to the cytoplasm through the shuttle protein exportin 5. After entering the cytoplasm, Dicer RNase catalyzes the cleavage of pre-miRNA into mature double-stranded miRNA. One of the miRNA single strands binds to RISC (also known as miRISC). RISC: RNA-induced silencing complex.
Circular RNA (circRNA) is a non-coding RNA characterized by a covalently closed loop structure devoid of a 5’ cap and a 3’ polyadenylated tail. First, circRNA is transcribed from genomic DNA by RNA polymerase II or III, similar to linear mRNA transcripts. Subsequently, during the splicing process, the downstream 5’ splice site of the precursor mRNA (pre-mRNA) is back-spliced with the upstream 3’ splice site to form a circular molecule[26-28]. CircRNAs can significantly contribute to the progression of diverse diseases by exerting various biological effects[29-31]. In the context of known tumor development, circRNA exhibits multiple functions, including: (A) acting as a sponge for competing endogenous RNA (ceRNA) or miRNA; (B) acting as sponges for RNA-binding proteins (RBPs); (C) participating in protein translation; (D) regulating transcription and splicing; and (E) interacting with RNA-binding proteins [Figure 2][32-36].
Figure 2. Biogenesis and functional mechanism of circRNA. First, circRNA is transcribed from genomic DNA by RNA polymerase II or III. Subsequently, during the splicing process, the downstream 5’ splice site of the precursor mRNA (pre-mRNA) is back-spliced with the upstream 3’ splice site to form a circular molecule.
MIRNAS AND CIRCRNAS AS DIAGNOSTIC MARKERS IN BC
BC is an epithelial malignancy arising from the terminal ductal lobular unit of the breast, which is generally categorized into non-invasive or invasive carcinoma based on histology. Non-invasive carcinoma is further divided into ductal and lobular carcinoma in situ. Invasive BC penetrates surrounding breast tissue and primarily includes invasive lobular carcinoma (ILC) and invasive ductal carcinoma (IDC)[2]. BCs in different tissue types and molecular subtypes differ in pathology, genomic profiles, metastatic organ chemotaxis, and responses to treatment. But in any case, early diagnosis of BC is the most effective way to reduce mortality. Identifying biomarkers for the early detection of BC is crucial.
Since miRNAs and circRNAs control many biological events related to cancer, they are often associated with the early diagnosis of the disease. Studies have shown that some circRNAs and miRNAs exhibit differential expression patterns in BC compared to normal tissues, and these circRNAs and miRNAs may be involved in the occurrence and development of BC by regulating cancer-related genes. Similarly, certain circRNAs and miRNAs can also be detected in serum exosomes, and their expression levels may be associated with clinical features of BC [Figure 3]. Recent research has found that five plasma miRNAs
Figure 3. CircRNAs and miRNAs in the exosomes of breast cancer patients. Some circRNAs and miRNAs can be detected in the serum exosomes of breast cancer patients, and analysis of exosomes in serum can provide information about the disease status.
The continuous discovery in research also indicates that miRNA and circRNA expression influence proliferation, invasion, metastasis, and epithelial-mesenchymal transition (EMT) in BC. Compared to healthy controls, the expression levels of miR-144-3p, miR-23a-3p, miR-148a-3p, miR-130a-5p, and miR-152-3p were lower in BC patients, which was associated with clinical stage and lymph node metastasis[41]. Overexpression of circRHOT1 and circ_0076611 enhanced the proliferative ability of triple-negative breast cancer (TNBC) cells and promoted the proliferation, invasion, metastasis, and EMT of BC[42-44]. In addition, miRNA and circRNA combined with other diagnostic markers can improve the sensitivity of BC diagnosis. MiR-1910-3p in serum exosomes may be used as an effective diagnostic marker for BC diagnosis, and when combined with CA153, it can improve the sensitivity of BC diagnosis[45]. A combination of two miRNAs, miR-4710 and miR-629-3p, along with three clinicopathologic factors, lymphovascular invasion, ultrasound findings, and T stage, showed an AUC of 0.86, which may help diagnose ALN metastasis prior to surgery in a less-invasive manner than SLNB[46].
However, there is still controversy about the application of miRNA in diagnosis. Several studies have found that serum miR-21 expression is abnormally elevated in BC. By comparing miR-21 expression levels in the plasma of 252 BC patients, 82 benign breast tumors, and 127 healthy controls, plasma miR-21 levels were found elevated in BC patients, which were significantly decreased after surgery compared with
MIRNAS AND CIRCRNAS AS PROGNOSTIC AND PREDICTIVE BIOMARKERS IN BC
Studies on circRNA and miRNA in BC tissue and serum samples have shown that the expression patterns of these molecules are associated with tumor malignancy and poor prognosis. Analysis of circRNA and miRNA in serum samples can provide a non-invasive method for the prognostic assessment of BC patients.
Several studies have evaluated the relationship between miRNA expression and BC survival or prognostic prediction. High levels of miR-421, miR-128-1, and miR-128-2 were significantly associated with adverse clinical features and cancer recurrence, and can serve as potential prognostic markers for BC[50]. High expression of miR-205, miR-133a, miR-21, miR-155, and miR-92b-3p were correlated with poor overall survival in BC patients[51]. Conversely, high expression of some miRNAs can improve the overall survival of BC patients. For example, high expression of miR-451a and miR-367 can improve progression-free survival and overall survival in BC patients[52,53]. In addition, miRNA expression may also predict the effects of certain chemotherapeutic drugs and antitumor immune responses. A combination of eight serum miRNAs, (miR-3160-5p, miR-5698, miR-4710, miR-4483, miR-575, miR-8089, miR-296-3p, and miR-4755-3p) was established as biomarkers to predict the responsiveness to eribulin and the emergence of new distant metastases in metastatic BC patients[54]. Bioinformatic analysis of human BC databases revealed that high serum and tumor miR-155 levels were correlated with favorable antitumor immune profiles and better patient outcomes, which may be a favorable prognostic marker for BC patients[55]. Although the above-mentioned miRNAs as predictive markers have not yet been used in clinical applications, they provide sufficient evidence for the use of miRNAs as prognostic markers for BC.
Similar to miRNA, there is also a relationship between circRNA expression and BC survival or prognosis. Zhong et al. collected serum samples from 45 BC patients and 45 normal individuals for qRT-PCR analysis and found that the expression of circRASSF2 was significantly increased in the BC group[56]. CircRASSF2 acts as a sponge for miR-1205 and regulates the expression of HOXA1, thereby promoting BC proliferation, migration, and invasion. BC patients with high circRASSF2 expression have lower overall survival and progression-free survival compared to those with low circRASSF2 expression. The expression of circCDYL is upregulated in tumor tissue and serum of BC patients, and its upregulation is associated with a higher tumor burden, shorter survival, and poor response to clinical treatment[57].
MIRNAS, CIRCRNAS, AND DRUG RESISTANCE IN BC
In the treatment of malignant tumors, chemotherapy is one of the commonly used methods and has a broad prospect for future development. However, due to the emergence of drug resistance, the therapeutic effect of chemotherapy often fails to reach expectations. The mechanisms leading to drug resistance include changes in the cell cycle, enhanced DNA repair, functional changes in the cell death mechanism, increased drug efflux, and EMT. With the advancement of DNA and RNA microarray and sequencing technologies, extensive studies have demonstrated that miRNA and circRNA play a vital role in drug resistance. A comprehensive understanding of the role of miRNA and circRNA in the molecular mechanisms leading to drug resistance will help to develop better strategies for cancer treatment.
The cell cycle encompasses the entirety of processes that a cell undergoes from the conclusion of one division to the conclusion of the subsequent division, and consists of four consecutive phases: G0/G1 (Gap 0/1), S (synthesis), G2 (Gap 2), and M (mitosis)[58]. Disruption of the cell cycle is a well-known hallmark of cancer, and its abnormal activation is associated with drug resistance. Quite a few miRNAs have been found to regulate genes involved in the cell cycle, leading to drug resistance. Bao et al. found low expression of miR-93 in paclitaxel (PTX)-resistant BC samples compared to PTX-responsive patients[59]. This is because miR-93 inhibits the pRB/E2F1 pathway and AKT phosphorylation by directly targeting E2F1 and CCND1, which inhibits cell proliferation and cell cycle progression to enhance the therapeutic effect of PTX in vivo. In addition, E2F1 has been identified as a direct target of miR-302b, which enhances sensitivity to cisplatin by regulating the E2F1/ATM axis[60].
At present, most chemotherapeutic agents induce direct or indirect DNA damage via double-strand breaks (DSB). DNA damage can be repaired through homologous recombination (HR) or non-homologous
Cell death is crucial in multiple physiological functions of the human body. Programmed cell death mainly includes three forms: apoptosis, autophagy, and programmatic necrosis[64]. Since miRNAs and circRNAs can regulate cell death, the combination of drugs and miRNAs/circRNAs has been widely studied in anticancer therapy. It was reported that miR-21-5p can downregulate the expression of programmed cell death 4 (PDCD4), which leads to paclitaxel resistance[65]. miR-512-3p directly targets the 3’UTR of Livin, which enhances the antitumor effect of epirubicin, gemcitabine, and docetaxel[66]. In addition, the low expression of circ_0006528 can alleviate ADM resistance and reduce the proliferation, migration, and autoplastic apoptosis of BC cells[67]. Circ-ABCB10 mediated PTX resistance and apoptosis in BC cells via the let-7a-5p/DUSP7 axis[68]. Therefore, identifying miRNAs and circRNAs that regulate pro-apoptotic or anti-apoptotic proteins to promote apoptosis and reduce resistance to anticancer drugs in cancer cells is a prospective study.
Another mechanism of chemotherapy resistance involves adenosine triphosphate (ATP)-dependent efflux pumps that reduce intracellular drug concentrations. ATP binding cassettes (ABCs) are a class of transmembrane transporters, such as BC resistance protein (BCRP), multidrug resistance-associated protein 1 (MRP1), and P-glycoprotein (P-gp)[69]. There have been some studies on the regulation of various ABC transporters in acquired chemotherapy-resistant cells by miRNA and circRNA. MiR-451 can downregulate the expression of BCRP, MRP1, and P-gp, thereby reversing the resistance of BC cells to ADM[70]. Knockdown of circ-CHI3L1.2 downregulated the expression levels of P-GP, MRP1, and glutathione
BC is composed of heterogeneous cell populations, which are mainly divided into two types of cell populations: BC stem cells (BCSC) and differentiated cells[58]. EMT is the most important pathway involved in BCSC phenotypic regulation. EMT is characterized by the loss of epithelial phenotype and the gain of mesenchymal characteristics, and it is a key factor for the development of resistance to multiple chemotherapeutic drugs. In recent years, more and more reports have shown that miRNAs and circRNAs are involved in drug resistance by regulating EMT and stem cell properties. Research found that
MIRNAS, CIRCRNAS, AND RESPONSE TO ENDOCRINE THERAPY IN BC
Endocrine therapy is one of the commonly used treatments for BC patients and is suitable for hormone receptor-positive BC patients[76-78]. However, there are significant differences in the response of various patients to endocrine therapy. Recent studies have indicated that the expression of miRNA and circRNA may be closely associated with the response of BC patients to endocrine therapy. Some miRNAs and circRNAs were found to be correlated with the expression levels of endocrine receptors (ER and PR), which play a crucial role in endocrine therapy. miR-342 expression was positively correlated with ERα mRNA in tissue samples, and its introduction into ER-dependent cells enhanced BC sensitivity to tamoxifen[79]. circPVT1 promotes the expression of ESR1 and ERα through miR-181a-2-3p[80]. Antisense oligonucleotides (ASOs) targeting circPVT1 inhibited the growth of ERα-positive BC cells and tumors and sensitized tamoxifen-resistant ERα-positive BC cells to tamoxifen treatment. In addition, some miRNAs and circRNAs may also affect the sensitivity of BC cells to hormones by regulating signaling pathways or target genes related to endocrine therapy. miR-519a is a novel oncogenic miR in ER-positive BC cells that enhances cell viability, promotes cell cycle progression, and confers resistance to tamoxifen-induced apoptosis[81].
MIRNAS, CIRCRNAS, AND RESPONSE TO TARGETED THERAPY IN BC
Targeted therapy is a cancer treatment method that targets specific genes or proteins. Trastuzumab is the first humanized mAb developed for HER2 and has achieved remarkable success in the treatment of HER2-positive BC[84]. However, it has been observed that some BC patients are insensitive to HER2 treatment or change from susceptible to resistant. Increased expression of miR-221 can be observed in trastuzumab-resistant cells[85]. Circ-BGN is overexpressed in trastuzumab-resistant BC tissues, and its downregulation reduces cell viability, especially restoring sensitivity to trastuzumab[86]. These findings indicate that the expression of miRNA and circRNA has an impact on the efficacy of trastuzumab. In addition, overexpression of circCDYL2 stabilized growth factor receptor-bound protein 7 (GRB7) by preventing its ubiquitination degradation, thereby making BC cells resistant to trastuzumab[87]. Therefore, an in-depth exploration of the mechanisms of miRNA and circRNA in the response to targeted therapies could be helpful for BC-targeted therapies.
CONCLUSION
BC ranks first in global incidence and mortality among female cancers. Identifying key biomarkers in the progression of BC can address the urgent need to enhance personalized treatment and improve the cure rate. MiRNA is abnormally expressed in many cancers, and can be utilized for the diagnosis and classification of BC. MiRNA data are easy to obtain, sensitive, and reliable, which can inspire research on the occurrence and progression of BC. With the advancement of miRNA chip and deep sequencing technologies, new miRNA biomarkers have been identified [Figure 4]. By interacting with miRNAs and other molecular effectors, circRNAs modulate gene expression at various levels, contributing to the intricate network of dysregulated signaling pathways in cancer. In BC, aberrant circRNA expression profiles have been implicated in tumorigenesis, progression, and metastasis [Figure 4]. Importantly, several dysregulated circRNAs show potential as diagnostic and prognostic markers, highlighting their valuable utility as clinical tools for BC management.
Despite significant progress in understanding the role of miRNA and circRNA in BC, challenges and opportunities remain. First, due to the large differences in the number of enrolled samples, current research on miRNA and circRNA as biomarkers still faces obstacles related to inconsistency and irreproducibility. Unifying the requirements for patient inclusion and the number of selected cases will be the key to solving this problem. Second, due to the limited sensitivity of current technologies and the specific objectives of researchers, discrepancies exist in studies assessing the association between miRNA and circRNA and their target genes. It is necessary to combine extensive multicenter studies with basic research to better characterize specific miRNAs and circRNAs and their related pathways. Finally, current therapeutic research on miRNA and circRNA is mainly at the preclinical stage, and miRNA and circRNA mimics or antagonists are mainly used to promote or inhibit their roles in BC. Addressing issues such as poor transfection efficiency and off-target effect is crucial.
Exploring the potential of miRNAs and circRNAs as diagnostic and prognostic biomarkers for BC represents a paradigm shift in the field of oncology, offering possibilities for more precise and personalized diagnostic and therapeutic strategies for BC. By leveraging the unique expression profiles of these
DECLARATIONS
Acknowledgments
We thank Figdraw (https://www.home-for-researchers.com/) for the preparation of the graphic abstract, Figure 1, and Figure 2. Figure 3 was partly generated using Servier.
Medical Art, provided by Servier, licensed under a Creative Commons Attribution 3.0. unported license.
Authors’ contributions
Conceptualization and design of the study: Zhang M (Meilan Zhang), Guo Z, Wang H, Tan W, Wang S
Original drafting: Zhang M (Meilan Zhang), Zheng Z, Zhang M (Mengli Zhang), Wang S
Preparation of figures: Liu R, Wang S
Review and editing: Zhang M (Meilan Zhang), Guo Z, Wang H, Tan W
All authors have read and agreed to the published version of the manuscript.
Availability of data and materials
Not applicable.
Financial support and sponsorship
This work was supported by the Science and Technology Project of Guangzhou City (No. 202201010029).
Conflicts of interest
All authors declared that there are no conflicts of interest.
Ethical approval and consent to participate
Not applicable.
Consent for publication
Not applicable.
Copyright
© The Author(s) 2024.
REFERENCES
1. Bray F, Laversanne M, Sung H, et al. Global cancer statistics 2022: GLOBOCAN estimates of incidence and mortality worldwide for 36 cancers in 185 countries. CA Cancer J Clin 2024;74:229-63.
2. Nolan E, Lindeman GJ, Visvader JE. Deciphering breast cancer: from biology to the clinic. Cell 2023;186:1708-28.
3. Wang J, Li B, Luo M, et al. Progression from ductal carcinoma in situ to invasive breast cancer: molecular features and clinical significance. Signal Transduct Target Ther 2024;9:83.
4. Purrahman D, Mahmoudian-Sani MR, Saki N, Wojdasiewicz P, Kurkowska-Jastrzębska I, Poniatowski ŁA. Involvement of progranulin (PGRN) in the pathogenesis and prognosis of breast cancer. Cytokine 2022;151:155803.
5. He B, Zhao Z, Cai Q, et al. miRNA-based biomarkers, therapies, and resistance in Cancer. Int J Biol Sci 2020;16:2628-47.
7. Syed R, Davey MG, Richard V, Miller N, Kerin MJ. Biological implications of MicroRNAs as regulators and biomarkers of therapeutic toxicities in breast cancer. Int J Mol Sci 2023;24:12694.
8. Hill M, Tran N. miRNA interplay: mechanisms and consequences in cancer. Dis Model Mech 2021;14:dmm047662.
9. Vishnoi A, Rani S. miRNA biogenesis and regulation of diseases: an updated overview. Methods Mol Biol 2023;2595:1-12.
10. Saliminejad K, Khorram Khorshid HR, Soleymani Fard S, Ghaffari SH. An overview of microRNAs: biology, functions, therapeutics, and analysis methods. J Cell Physiol 2019;234:5451-65.
12. Zeng Y, Du W, Huang Z, et al. Hsa_circ_0060467 promotes breast cancer liver metastasis by complexing with eIF4A3 and sponging miR-1205. Cell Death Discov 2023;9:153.
13. Memczak S, Jens M, Elefsinioti A, et al. Circular RNAs are a large class of animal RNAs with regulatory potency. Nature 2013;495:333-8.
14. Ashwal-Fluss R, Meyer M, Pamudurti NR, et al. circRNA biogenesis competes with pre-mRNA splicing. Mol Cell 2014;56:55-66.
15. Yarmishyn AA, Ishola AA, Chen CY, et al. Circular RNAs modulate cancer hallmark and molecular pathways to support cancer progression and metastasis. Cancers 2022;14:862.
16. Li Y, Wang Z, Yang J, et al. CircTRIM1 encodes TRIM1-269aa to promote chemoresistance and metastasis of TNBC via enhancing CaM-dependent MARCKS translocation and PI3K/AKT/mTOR activation. Mol Cancer 2024;23:102.
17. Song X, Wang X, Chen X, Yu Z, Zhou Y. SRSF1 inhibits ferroptosis and reduces cisplatin chemosensitivity of triple-negative breast cancer cells through the circSEPT9/GCH1 axis. J Proteomics 2024;292:105055.
18. Wang H, Wang X, Shen W, et al. CircRNA (circ)_0007823 contributes to triple-negative breast cancer progression and cisplatin resistance via the miR-182-5p/FOXO1 pathway. Biochem Genet 2024:1-13.
20. Matsuyama H, Suzuki HI. Systems and synthetic microRNA biology: from biogenesis to disease pathogenesis. Int J Mol Sci 2019;21:132.
21. Pietrykowska H, Sierocka I, Zielezinski A, et al. Biogenesis, conservation, and function of miRNA in liverworts. J Exp Bot 2022;73:4528-45.
22. Tafrihi M, Hasheminasab E. MiRNAs: Biology, biogenesis, their web-based tools, and databases. Microrna 2019;8:4-27.
23. Do DN, Dudemaine PL, Mathur M, Suravajhala P, Zhao X, Ibeagha-Awemu EM. miRNA regulatory functions in farm animal diseases, and biomarker potentials for effective therapies. Int J Mol Sci 2021;22:3080.
24. Kilikevicius A, Meister G, Corey DR. Reexamining assumptions about miRNA-guided gene silencing. Nucleic Acids Res 2022;50:617-34.
25. Pu M, Chen J, Tao Z, et al. Regulatory network of miRNA on its target: coordination between transcriptional and post-transcriptional regulation of gene expression. Cell Mol Life Sci 2019;76:441-51.
26. Yang L, Wilusz JE, Chen LL. Biogenesis and regulatory roles of circular RNAs. Annu Rev Cell Dev Biol 2022;38:263-89.
27. Chen LL. The expanding regulatory mechanisms and cellular functions of circular RNAs. Nat Rev Mol Cell Biol 2020;21:475-90.
28. Chen LL. The biogenesis and emerging roles of circular RNAs. Nat Rev Mol Cell Biol 2016;17:205-11.
29. Huang X, Song C, Zhang J, Zhu L, Tang H. Circular RNAs in breast cancer diagnosis, treatment and prognosis. Oncol Res 2023;32:241-9.
30. Zhu Y, Huang G, Li S, et al. CircSMARCA5: a key circular RNA in various human diseases. Front Genet 2022;13:921306.
31. Wang Z, Yang L, Wu P, et al. The circROBO1/KLF5/FUS feedback loop regulates the liver metastasis of breast cancer by inhibiting the selective autophagy of afadin. Mol Cancer 2022;21:29.
32. Li Z, Ruan Y, Zhang H, Shen Y, Li T, Xiao B. Tumor-suppressive circular RNAs: mechanisms underlying their suppression of tumor occurrence and use as therapeutic targets. Cancer Sci 2019;110:3630-8.
33. Li X, Yang L, Chen LL. The biogenesis, functions, and challenges of circular RNAs. Mol Cell 2018;71:428-42.
34. Wu S, Lu J, Zhu H, et al. A novel axis of circKIF4A-miR-637-STAT3 promotes brain metastasis in triple-negative breast cancer. Cancer Lett 2024;581:216508.
35. Zhang M, Bai X, Zeng X, Liu J, Liu F, Zhang Z. circRNA-miRNA-mRNA in breast cancer. Clin Chim Acta 2021;523:120-30.
36. Huang A, Zheng H, Wu Z, Chen M, Huang Y. Circular RNA-protein interactions: functions, mechanisms, and identification. Theranostics 2020;10:3503-17.
37. Li M, Zou X, Xia T, et al. A five-miRNA panel in plasma was identified for breast cancer diagnosis. Cancer Med 2019;8:7006-17.
38. Wang YW, Xu Y, Wang YY, et al. Elevated circRNAs circ_0000745, circ_0001531 and circ_0001640 in human whole blood: potential novel diagnostic biomarkers for breast cancer. Exp Mol Pathol 2021;121:104661.
39. Kurozumi S, Seki N, Narusawa E, et al. Identification of microRNAs associated with histological grade in early-stage invasive breast cancer. Int J Mol Sci 2023;25:35.
40. Verma VK, Beevi SS, Nair RA, et al. MicroRNA signatures differentiate types, grades, and stages of breast invasive ductal carcinoma (IDC): miRNA-target interacting signaling pathways. Cell Commun Signal 2024;22:100.
41. Li X, Zou W, Wang Y, et al. Plasma-based microRNA signatures in early diagnosis of breast cancer. Mol Genet Genomic Med 2020;8:e1092.
42. Jiang W, Yu Y, Ou J, Li Y, Zhu N. Exosomal circRNA RHOT1 promotes breast cancer progression by targeting miR-204-5p/ PRMT5 axis. Cancer Cell Int 2023;23:260.
43. Turco C, Esposito G, Iaiza A, et al. MALAT1-dependent hsa_circ_0076611 regulates translation rate in triple-negative breast cancer. Commun Biol 2022;5:598.
44. Yang SJ, Wang DD, Zhong SL, et al. Tumor-derived exosomal circPSMA1 facilitates the tumorigenesis, metastasis, and migration in triple-negative breast cancer (TNBC) through miR-637/Akt1/β-catenin (cyclin D1) axis. Cell Death Dis 2021;12:420.
45. Wang B, Mao JH, Wang BY, et al. Exosomal miR-1910-3p promotes proliferation, metastasis, and autophagy of breast cancer cells by targeting MTMR3 and activating the NF-κB signaling pathway. Cancer Lett 2020;489:87-99.
46. Shiino S, Matsuzaki J, Shimomura A, et al. Serum miRNA-based prediction of axillary lymph node metastasis in breast cancer. Clin Cancer Res 2019;25:1817-27.
47. Wang H, Tan Z, Hu H, et al. microRNA-21 promotes breast cancer proliferation and metastasis by targeting LZTFL1. BMC Cancer 2019;19:738.
48. Yuan X, Qian N, Ling S, et al. Breast cancer exosomes contribute to pre-metastatic niche formation and promote bone metastasis of tumor cells. Theranostics 2021;11:1429-45.
49. Alunni-Fabbroni M, Majunke L, Trapp EK, et al; SUCCESS Study Group. Whole blood microRNAs as potential biomarkers in post-operative early breast cancer patients. BMC Cancer 2018;18:141.
50. Bao S, Hu T, Liu J, et al. Genomic instability-derived plasma extracellular vesicle-microRNA signature as a minimally invasive predictor of risk and unfavorable prognosis in breast cancer. J Nanobiotechnology 2021;19:22.
51. Raghu A, Magendhra Rao AKD, Rajkumar T, Mani S. Prognostic implications of microRNA-155, -133a, -21 and -205 in breast cancer patients’ plasma. Microrna 2021;10:206-18.
52. Liu B, Pan J, Fu C. Correlation of microRNA-367 in the clinicopathologic features and prognosis of breast cancer patients. Medicine 2021;100:e26103.
53. Záveský L, Jandáková E, Weinberger V, et al. Small non-coding RNA profiling in breast cancer: plasma U6 snRNA, miR-451a and miR-548b-5p as novel diagnostic and prognostic biomarkers. Mol Biol Rep 2022;49:1955-71.
54. Satomi-Tsushita N, Shimomura A, Matsuzaki J, et al. Serum microRNA-based prediction of responsiveness to eribulin in metastatic breast cancer. PLoS One 2019;14:e0222024.
55. Wang J, Wang Q, Guan Y, et al. Breast cancer cell-derived microRNA-155 suppresses tumor progression via enhancing immune cell recruitment and antitumor function. J Clin Invest 2022;132:e157248.
56. Zhong W, Bao L, Yuan Y, Meng Y. CircRASSF2 acts as a prognostic factor and promotes breast cancer progression by modulating miR-1205/HOXA1 axis. Bioengineered 2021;12:3014-28.
57. Liang G, Ling Y, Mehrpour M, et al. Autophagy-associated circRNA circCDYL augments autophagy and promotes breast cancer progression. Mol Cancer 2020;19:65.
58. Garrido-Cano I, Pattanayak B, Adam-Artigues A, et al. MicroRNAs as a clue to overcome breast cancer treatment resistance. Cancer Metastasis Rev 2022;41:77-105.
59. Bao C, Chen J, Chen D, et al. MiR-93 suppresses tumorigenesis and enhances chemosensitivity of breast cancer via dual targeting E2F1 and CCND1. Cell Death Dis 2020;11:618.
60. Cataldo A, Cheung DG, Balsari A, et al. miR-302b enhances breast cancer cell sensitivity to cisplatin by regulating E2F1 and the cellular DNA damage response. Oncotarget 2016;7:786-97.
61. Papaspyropoulos A, Hazapis O, Lagopati N, et al. The role of circular RNAs in DNA damage response and repair. Cancers 2021;13:5352.
62. Lu X, Liu R, Wang M, et al. MicroRNA-140 impedes DNA repair by targeting FEN1 and enhances chemotherapeutic response in breast cancer. Oncogene 2020;39:234-47.
63. Lin S, Yu L, Song X, et al. Intrinsic adriamycin resistance in p53-mutated breast cancer is related to the miR-30c/FANCF/REV1-mediated DNA damage response. Cell Death Dis 2019;10:666.
64. Strasser A, Vaux DL. Cell death in the origin and treatment of cancer. Mol Cell 2020;78:1045-54.
65. Tao L, Wu YQ, Zhang SP. MiR-21-5p enhances the progression and paclitaxel resistance in drug-resistant breast cancer cell lines by targeting PDCD4. Neoplasma 2019;66:746-55.
66. Duan WJ, Bi PD, Ma Y, Liu NQ, Zhen X. MiR-512-3p regulates malignant tumor behavior and multi-drug resistance in breast cancer cells via targeting Livin. Neoplasma 2020;67:102-10.
67. Hao J, Du X, Lv F, Shi Q. Knockdown of circ_0006528 suppresses cell proliferation, migration, invasion, and adriamycin chemoresistance via regulating the miR-1236-3p/CHD4 axis in breast cancer. J Surg Res 2021;260:104-15.
68. Yang W, Gong P, Yang Y, Yang C, Yang B, Ren L. Circ-ABCB10 contributes to paclitaxel resistance in breast cancer through let-7a-5p/DUSP7 axis. Cancer Manag Res 2020;12:2327-37.
69. Chen Z, Shi T, Zhang L, et al. Mammalian drug efflux transporters of the ATP binding cassette (ABC) family in multidrug resistance: a review of the past decade. Cancer Lett 2016;370:153-64.
70. Chen Y, Li X, Shi L, et al. Combination of 7-O-geranylquercetin and microRNA-451 enhances antitumor effect of Adriamycin by reserving P-gp-mediated drug resistance in breast cancer. Aging 2022;14:7156-69.
71. Zhang Z, Zhou Q, Luo F, et al. Circular RNA circ-CHI3L1.2 modulates cisplatin resistance of osteosarcoma cells via the miR-340-5p/LPAATβ axis. Hum Cell 2021;34:1558-68.
72. Liang H, Lin Z, Lin H, Zhao L, Huang W. circRNA_103615 contributes to tumor progression and cisplatin resistance in NSCLC by regulating ABCB1. Exp Ther Med 2021;22:934.
73. Lee JW, Guan W, Han S, Hong DK, Kim LS, Kim H. MicroRNA-708-3p mediates metastasis and chemoresistance through inhibition of epithelial-to-mesenchymal transition in breast cancer. Cancer Sci 2018;109:1404-13.
74. Wang G, Dong Y, Liu H, et al. Loss of miR-873 contributes to gemcitabine resistance in triple-negative breast cancer via targeting ZEB1. Oncol Lett 2019;18:3837-44.
75. Liu YY, Zhang LY, Du WZ. Circular RNA circ-PVT1 contributes to paclitaxel resistance of gastric cancer cells through the regulation of ZEB1 expression by sponging miR-124-3p. Biosci Rep 2019;39:BSR20193045.
76. Jhaveri K, Marmé F. Current and emerging treatment approaches for hormone receptor-positive/human epidermal growth factor receptor 2-negative metastatic breast cancer. Cancer Treat Rev 2024;123:102670.
77. Nakagawa T, Hayashi K, Ogawa A, et al. Bone marrow carcinomatosis in a stage IV breast cancer patient treated by letrozole as first-line endocrine therapy. Case Rep Oncol 2022;15:436-41.
78. Boscolo Bielo L, Trapani D, Nicolò E, et al. The evolving landscape of metastatic HER2-positive, hormone receptor-positive Breast Cancer. Cancer Treat Rev 2024;128:102761.
79. He YJ, Wu JZ, Ji MH, et al. miR-342 is associated with estrogen receptor-α expression and response to tamoxifen in breast cancer. Exp Ther Med 2013;5:813-8.
80. Yi J, Wang L, Hu GS, et al. CircPVT1 promotes ER-positive breast tumorigenesis and drug resistance by targeting ESR1 and MAVS. EMBO J 2023;42:e112408.
81. Ward A, Shukla K, Balwierz A, et al. MicroRNA-519a is a novel oncomir conferring tamoxifen resistance by targeting a network of tumour-suppressor genes in ER+ breast cancer. J Pathol 2014;233:368-79.
82. Zheng L, Meng X, Li X, et al. miR-125a-3p inhibits ERα transactivation and overrides tamoxifen resistance by targeting CDK3 in estrogen receptor-positive breast cancer. FASEB J 2018;32:588-600.
83. Chen J, Shi P, Zhang J, et al. CircRNA_0044556 diminishes the sensitivity of triple-negative breast cancer cells to adriamycin by sponging miR-145 and regulating NRAS. Mol Med Rep 2022;25:51.
84. Misir S, Yaman SO, Petrović N, Sumer C, Hepokur C, Aliyazicioglu Y. circRNAs in drug resistance of breast cancer. Oncol Res 2022;30:157-72.
85. Ye X, Bai W, Zhu H, et al. MiR-221 promotes trastuzumab-resistance and metastasis in HER2-positive breast cancers by targeting PTEN. BMB Rep 2014;47:268-73.
86. Wang S, Wang Y, Li Q, Li X, Feng X. A novel circular RNA confers trastuzumab resistance in human epidermal growth factor receptor 2-positive breast cancer through regulating ferroptosis. Environ Toxicol 2022;37:1597-607.
Cite This Article

How to Cite
Zhang, M.; Zheng Z.; Wang S.; Liu R.; Zhang M.; Guo Z.; Wang H.; Tan W. The role of circRNAs and miRNAs in drug resistance and targeted therapy responses in breast cancer. Cancer. Drug. Resist. 2024, 7, 30. http://dx.doi.org/10.20517/cdr.2024.62
Download Citation
Export Citation File:
Type of Import
Tips on Downloading Citation
Citation Manager File Format
Type of Import
Direct Import: When the Direct Import option is selected (the default state), a dialogue box will give you the option to Save or Open the downloaded citation data. Choosing Open will either launch your citation manager or give you a choice of applications with which to use the metadata. The Save option saves the file locally for later use.
Indirect Import: When the Indirect Import option is selected, the metadata is displayed and may be copied and pasted as needed.
About This Article
Special Issue
Copyright
Data & Comments
Data
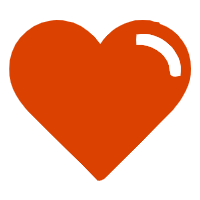
Comments
Comments must be written in English. Spam, offensive content, impersonation, and private information will not be permitted. If any comment is reported and identified as inappropriate content by OAE staff, the comment will be removed without notice. If you have any queries or need any help, please contact us at support@oaepublish.com.