Mechanisms of action and resistance to anti-HER2 antibody-drug conjugates in breast cancer
Abstract
Human epidermal growth factor 2 (HER2)-positive breast cancer (BC) represents nearly 20% of all breast tumors. Historically, these patients had a high rate of relapse and dismal prognosis. The advent of HER2-targeting monoclonal antibodies such as trastuzumab followed by pertuzumab had improved the prognosis of HER2-positive metastatic BC. More recently, antibody-drug conjugates (ADCs) are now reshaping the treatment paradigm of solid tumors, especially breast cancer. Tratsuzumab emtansine (T-DM1) was one of the first ADC developed in oncology and was approved for the management of HER2-positive metastatic BC. In a head-to-head comparison, trastuzumab deruxtecan (T-DXd) defeated T-DM1 as a second-line treatment. The efficacy of ADCs is counterbalanced by the appearance of acquired resistance to these agents. In this paper, we summarize the mechanisms of action and resistance of
Keywords
INTRODUCTION
Breast cancer (BC) is the most common cancer in women worldwide and the second leading reason of cancer-related mortality. It accounts for 287,000 new diagnosed cases and approximately 43,000 deaths in the United States in 2022[1]. Human epidermal growth factor 2 (HER2)-positive BC represents nearly 20% of newly diagnosed patients. The HER2-neu gene encodes an oncoprotein that belongs to the epidermal growth factor receptor (EGFR) family consisting of four members[2-4]. This subtype of tumors has historically been linked to an aggressive phenotype, a higher rate of relapse, and a worse prognosis compared to other tumor subtypes if untreated[5]. This worse prognosis of HER2-positive BC has dramatically ameliorated with the development of anti-HER2 monoclonal antibodies (mAb). Trastuzumab, in combination with chemotherapy, was the first mAb approved in 1998 in HER2-positive metastatic BC[6]. Trastuzumab was also approved as a neoadjuvant and adjuvant treatment in combination with chemotherapy. Pertuzumab is another HER2-targeting recombinant humanized mAb. The combination of chemotherapy, trastuzumab, and pertuzumab represents the gold standard in first-line setting in metastatic HER2-positive BC based on the CLEOPATRA study[7]. The combination regimen has been then approved as a neoadjuvant treatment based on the NeoSphere phase II trial results[8]. Tyrosine kinase inhibitors (TKIs) targeting HER2, such as tucatinib, lapatinib, and neratinib, were also incorporated into the therapeutic arsenal of patients with metastatic BC[9]. The activity of all these drugs was limited by the development of resistance to HER2-targeting agents, and patients presented with disease progression. Antibody-drug conjugates (ADCs) are an emerging category of treatment developed in order to prevent or delay the emergence of resistance to treatment and to ameliorate efficacy in patients with metastatic BC. The first approved ADC was trastuzumab emtansine (T-DM1) as a second-line treatment in this subgroup of patients according to the randomized phase III EMILIA study in 2013[10,11]. Then came trastuzumab deruxtecan (T-DXd), a new-generation ADC, to replace T-DM1 in the second-line setting according to the results of a first randomized DESTINY-Breast03 phase III trial comparing T-DXd vs. T-DM1[12,13]. Table 1 summarizes the approvals of T-DM1 and T-DXd. Once again, the clinical efficacy of ADC is counterbalanced by the occurrence of acquired resistance. In this review, we focus on the structure of ADC, the mechanism of action and the clinical efficacy of T-DXd and T-DM1. We also discuss the mechanisms of resistance to these agents, and the strategies to overcome such resistance.
Current approvals of T-DM1 and T-DXd
Drug | Study name | Date of approval | Indication |
T-DM1 | EMILIA | February 2013 | HER2+ MBC previously treated with taxane and trastuzumab |
T-DM1 | KATHERINE | May 2019 | Adjuvant treatment in HER2+ EBC with residual disease after NAC with taxane and trastuzumab |
T-DXd | DESTINY-Breast01 | December 2019 | HER2+ MBC after two treatment lines |
T-DXd | DESTINY-Gastric01 | January 2021 | HER2+ MGC or GEJ after a prior trastuzumab-based treatment |
T-DXd | DESTINY-Breast03 | May 2022 | HER2+ MBC in second-line treatment |
T-DXd | DESTINY-Breast04 | August 2022 | HER2-low MBC after prior systemic treatment |
T-DXd | DESTINY-Lung02 | August 2022 | HER2-mutant metastatic NSCLC |
STRUCTURE OF ADC
ADCs are composed of humanized mAbs binding to an antigen specific to the tumor and a payload (cytotoxic drug) via a linker that could be cleavable or non-cleavable[14]. The antibodies incorporated in ADCs are fully humanized and most commonly IgG1-based because of their easier fabrication and stronger immunogenic characteristics in comparison with other IgG subtypes[15]. The antibody contains two fragments that bind to antigens, known as Fabs, and a constant fragment (Fc). Fabs mediate the identification of the antigen, while Fc is responsible for the interaction between the antibody and the effector immune cells[16]. An optimal antibody might present high affinity of binding to the correspondent target with low immunogenicity and good internalization with a relatively long plasma half-life[17]. Activation of immune cells, activation of the complement complexes, induction of complement-dependent cytotoxicity (CDC), activation of antibody-dependent cell-mediated cytotoxicity (ADCC), and antibody-dependent cell-mediated phagocytosis (ADCP) represent the main immune effector function of the antibody[18].
The payload (cytotoxic drug) is very potent and represents the most important element of the ADC. Cytotoxic payloads are divided into several categories such as anti-tubulin (maystatine analogs, auristatin analogs), topoisomerase I inhibitors (deruxtecan, govitecan), DNA-damaging agents (calicheamicins, duocarmazine, pyrrolobenzodiazepines), and RNA polymerase II inhibitor (amanitin). These payloads had an unfavorable therapeutic index if given intravenously as non-conjugated drugs[19].
The antibodies are connected to the cytotoxic payloads via linkers that lead to the stabilization of the ADCs and affect the pharmacokinetic characteristics of these drugs. These linkers might be cleavable, a process linked to factors specific to the tumors, such as pH changes (acid pH), reduction-oxidation conditions, or lysosomal enzymes. They can also be non-cleavable and require more processing to release cytotoxic payloads. In the case of non-cleavable linkers, the whole proteolytic degradation of the antibody happens in the lysosomes, a mechanism that might influence the payload. However, cleavable linkers presented lower plasma stability and shorter half-lives compared to non-cleavable linkers[20-22].
Drug-to-antibody ratio (DAR) is an important indicator and characteristic of ADCs. It refers to the number of drug molecules linked to the antibody. In general, the potency of ADCs is positively correlated to DAR. High DAR could be associated with quick clearance of the drug and, probably, higher toxicity. In contrast, lower DAR could be associated with decreased activity of ADC and increased therapeutic index[23,24].
MECHANISM OF ACTION AND CLINICAL ACTIVITY
T-DM1
As previously mentioned, T-DM1 is the first anti-HER2 ADC approved for the management of metastatic BC. It is composed of trastuzumab as HER2-targeting IgG1 monoclonal antibody and linked to emtansine (also known as DM1), a microtubule-inhibitory agent derivative of maytansine, via a non-cleavable linker. T-DM1 has a DAR of 3.5[21]. The mechanism of action of T-DM1 is complex. It is based on selective liberation of emtansine to HER2-positive tumor cells, inhibition of HER2 signaling by the trastuzumab, and shedding of the HER2 extracellular domain. T-DM1 can also evoke ADCC, similar to trastuzumab[25-27]. Another mechanism of action of T-DM1 that has been reported by Hunter et al. was the mitotic catastrophe that inhibits tumor growth with prolonged treatment of HER2-positive xenografts with T-DM1. It is characterized by the presence of a high proportion of tumor cells with aberrant mitotic figures[27]. The clinical activity of T-DM1 was evaluated in the EMILIA phase III study. It showed the superiority of T-DM1 over capecitabine combined with lapatinib in second-line treatment. T-DM1 had statistically significant higher median PFS (9.6 vs. 6.4 months; P < 0.001) and median OS (30.9 vs. 25.1 months; P < 0.001). In 2013, the FDA approved T-DM1 as a second-line treatment for the management of patients with HER2-positive metastatic BC, according to the results of this study[10,11]. However, T-DM1 failed to be positioned as a first-line treatment in the MARIANNE phase III study that randomized T-DM1 alone or with pertuzumab vs. taxane and trastuzumab. T-DM1 was not superior to control group[28]. Moreover, the incorporation of
T-DXd, DS8201
T-DXd is a third-generation ADC composed of trastuzumab, linked to deruxtecan, a potent topoisomerase I inhibitor and is an exatecan derivantive, via a cleavable linker[32]. T-DXd is characterized by multiple features such as its potent payload, short systemic half-life avoiding systemic exposure, a DAR of 8, and the bystander effect[33]. The phase II DESTINY-Breast01 clinical trial investigated the dosage of 5.4 mg/kg of
Major ongoing trials as monotherapy in BC
Study | Agents | Phase | No. of patients | Patients | Primary endpoint |
NCT05113251 (DESTINY-Breast11) | ddAC THP vs. T-DXd or T-DXd followed by THP | III | 900 | High-risk HER2+ early-stage BC | Rate of pCR |
NCT05704829 (ADAPTHER2-IV) | T-DXd vs. CT + T + P | II | 402 | Neoadjuvant in low-intermediate risk HER2+ BC | Rate of pCR dDFS |
NCT05900206 (ARIADNE) | T-DXd vs. standard of care | II | 370 | Neoadjuvant treatment HER2+ BC | Rate of pCR |
NCT04622319 (DESTINY-Breast05) | T-DXd vs. T-DM1 | III | 1,600 | High-risk HER2+ BC with residual disease in breast or axillary lymph nodes following neoadjuvant therapy | IDFS |
NCT04784715 (DESTINY-Breast09) | T-DXd vs. T-DXd + P vs. THP | III | 1,157 | HER2+ first-line MBC | PFS |
NCT04739761 (DESTINY-Breast12) | T-DXd | IIIb/IV | 506 | Previously treated HER2+ MBC with or without BM | ORR PFS |
NCT05950945 (DESTINY-Breast15) | T-DXd | IIIb | 250 | MBC HER2-low or HER2 IHC 0 | TTNT |
NCT05744375 (TRANSCENDER) | T-DXd | II | 41 | First-line treatment MBC resistant to T + P | ORR |
NCT06048718 (TUXEDO-4) | T-DXd | II | 27 | HER2-low MBC with newly diagnosed or progressing BM | ORR |
MECHANISMS OF RESISTANCE TO ANTI-HER2 ADC
Due to the complexity of the structure and mechanism of action of ADCs, which involve multiple processes, resistance can occur at any stage. It could be related to the expression of an antigen and its identification, or to internalization of the drug and its degradation, or to the release of payload and finally regulation of the apoptosis. To be noted that the large part of data discussed below had been obtained in preclinical models.
Antigen-related resistance
The most important mechanisms of resistance to HER2-targeting ADCs are related to the exposed antigen. After a long exposure to treatment, the level of expression of the receptor may decrease or structural alterations can occur. After a long treatment with T-DM1 and a high selective pressure, cells showing increased HER2 expression are cleared while those showing decreased HER2 receptor expression are subjected to clonal evolution[41]. Loganzo et al. reported the results of an in vitro experience of trastuzumab-maytansinoid (TM-ADC), which is an ADC similar to T-DM1, and BC cells. Two different lines of cells were used: MBA MB-361-DYT2 parental cells, and JIMT1 tumoral cells resistant to first-line treatment with trastuzumab. JIMT1 cells exhibited resistance to TM-ADC and presented responses to other chemotherapies that include anti-tubulin, demonstrating that decreased HER2 expression after months of selective pressure could contribute to drug resistance[42].
Moreover, the role of tumor heterogeneity in antigen expression and efficacy of ADCs was also reported in the KRISTINE trial. The presence of HER2-negative area in 10% of the cases by FISH or the detection of ERBB2 amplification between 5% and 50% of tumor cells defined HER2 heterogeneity. The pathologic complete response rate was 0% in the 10% of patients presenting tumor heterogeneity[43]. Furthermore, in the KRISTINE trial, patients with high levels of HER2 heterogeneity before T-DM1 had lower survival outcomes in comparison with those with low heterogeneity[43]. Similar results were found in the ZEPHIT trial that showed shorter times to treatment failure in patients with high levels of HER2 heterogeneity on HER2-PET CT scans[44]. Additionally, the antigen ectodomain may present truncated forms of HER2. It was reported as one of the potential resistance mechanisms but has not yet been proven in ADC. It has been initially proven with trastuzumab; tumors with truncated P95HER2 exhibited resistance to trastuzumab, whereas tumors with normal receptors were responsive to trastuzumab[45]. Heregulin, also known as neuregulin (NRG), which is a ligand for ERBB3 and ERBB4 receptors, could be another potential mechanism that might modulate the sensitivity to ADCs. NRG is a member of the epidermal growth factor (EGF) group, altering the sensitivity to T-DM1 and stimulating the heterodimerization of HER2 with HER3 and HER4[46]. Moreover, it seems that HER3 overexpression might be associated with resistance to HER2-targeting drugs such as T-DM1 and trastuzumab[47]. The inhibition of HER3 reversed acquired resistance to trastuzumab in in vitro xenografts derived from HER2-overexpressed gastric cancer[48].
Payload-related resistance
Another suggested mechanism of resistance to ADC is payload-related resistance that was initially observed in patients with non-Hodgkin lymphomas[49]. Takegawa et al. demonstrated that cells with resistance to
Intrinsic mechanisms of resistance to ADCs
One of the intrinsic mechanisms of resistance is the failure of internalization of HER2 by endocytosis. Numerous pathways of internalization were reported, such as clathrin-mediated endocytosis mediated by clathrin, the most common way of endocytosis of ADCs, endocytosis mediated by caveolae, and endocytosis independent of clathrin-caveolin[51]. Sung et al. generated multiple in-vitro models of resistance to
After the internalization of ADCs and their target molecules via endocytosis, they are driven to lysosomes, and cytotoxic agents are released after chemical and enzymatic cleavage. An impaired lysosomal function can contribute to resistance to ADCs. In fact, Ríos-Luci et al. isolated three resistant HER2-positive clones following treatment with T-DM1. These clones had the same expression of HER2, normal internalization and trafficking pathways. However, they presented increased pH levels in the lysosomes, along with altered proteolysis, resulting in the accumulation of T-DM1 and subsequently limiting its antitumor activity[57]. Moreover, defective liberation of antineoplastic drugs from lysozymes or defective traffic to cytoplasm might be implicated in the resistance to ADCs. In vitro models showed that the silencing or loss of SLC46A3, a gene encoding for a protein that is a direct transporter of maytansine-based catabolites outside the lysosomes, led to the accumulation of catabolites in the lysosomes and subsequent acquired T-DM1 resistance[58]. Regarding T-DXd, the DAISY multicenter phase II trial was the first study investigating the mechanisms of action of T-DXd as well as the mechanism of resistance that was unclear. The DAISY trial enrolled patients with metastatic BC into three cohorts: HER2-positive cohort (n = 72 patients), HER2-low
Drug efflux pumps were also reported as a mechanism of resistance to treatment by enhancing treatment ejection from tumor cells and could contribute to the occurrence of cancers with multidrug-resistant (MDR) phenotypes[60]. It could also be considered as a mechanism of resistance to ADC. The most frequent phenotype is the MDR1-mediated efflux. For example, maytansinoids are substrates of ABC transporters such as MDR1[61]. Moreover, in vitro data showed that the inhibition of MDR1 led to the reversal of the resistance to T-DM1[62].
Signaling pathways activation
Another potential mechanism that might contribute to resistance to ADCs is the activation of signaling pathways. The most common pathway involved is the PI3k/AKT/mTOR pathway, which is responsible for tumor growth, metabolism, and survival. It has been reported that the activation of the PI3K pathway can contribute to reduced response to ADCs, decreased activity of the cytotoxic drug, and longer survival of the cells in patients treated with trastuzumab who have PTEN deletions or PIK3CA mutations[63]. To date, available data from the EMILIA trial show that patients with PIK3CA mutation or PTEN deletion and receiving T-DM1 had the same survival outcomes compared to those without genetic alterations[64]. Moreover, Wu et al. reported that the overexpression of Wnt3 favors higher expression of β-catenin, increased cell proliferation rate, cell invasiveness and resistance to trastuzumab, indicating that the activation of the Wnt/β-catenin pathway might be involved in resistance to ADCs[65]. Another potential mechanism of resistance is the overexpression of the trophoblast cell-surface antigen 2 (TROP-2), which is a transmembrane glycoprotein that could be implicated in the akt phosphorylation in different tumoral cells, and in the activation of several signaling pathways such as PI3K/Akt, MAPK/ERK, and Wnt/ β-catenin pathways[66]. Recently, Gion et al. reported a retrospective analysis from the PHERGain trial of the relationship between TROP-2 expression and pathological complete response (pCR). Patients with TROP-2 expression had significantly lower pCR (P = 0.01). These findings suggest that TROP-2 could be a potential biomarker of resistance[67]. Moreover, the Topoisomerase II Alpha (TOP2A) gene is located at chromosome 17 and near the HER2/neu gene. It encodes for topoisomerase II alpha protein. Interestingly, it has been reported that 35% of patients with BC harboring HER2 amplification present with co-amplification of the TOP2A gene[68]. The topoisomerase II alpha protein is the target of anthracyclines, notably doxorubicin and epirubicin[69]. The role of TOP2A in the resistance to anti-HER2 targeted therapies is unclear. However, it could be intriguing to develop agents that target HER2 and TOP2A.
OVERCOMING RESISTANCE TO ADC
Multiple strategies are currently under evaluation in order to prevent or reverse resistance to anti-HER2 ADCs that remains a significant challenge, and to ameliorate their clinical activity in metastatic BC. These strategies might be the use of other ADCs, more specifically, next-generation ADCs with different target or payload or DAR, or combination with other agents such as tyrosine kinase inhibitors (TKIs), immune checkpoint inhibitors (ICIs), and synthetic DNA-damaging agents. Tables 3 and 4 summarize major ongoing clinical trials evaluating T-DM1 and T-DXd combined with other drugs. Moreover, Durbin et al. reported in preclinical models that the reduction of antigen/antibody complex recycling to the membrane could be a possible target for pharmacological intervention[70].
Ongoing studies evaluating T-DM1 combined with other drugs in BC
Trial | Agents | Phase | Number of patients | Patients | Primary endpoint |
NCT05673928 (TUCATEMEB) | Tucatinib in combination with T-DM1 | II | 30 | Metastatic HER2-positive cancers | Intracranial antitumor activity |
NCT04873362 (Astefania) | T-DM1 + placebo or atezolizumab | III | 1,700 | HER2-positive BC with high risk of relapse in the adjuvant setting | IDFS |
NCT04457596 (CompassHER2 RD) | T-DM1 + placebo or tucatinib | III | 1,031 | HER2-positive BC with residual disease following neoadjuvant HER2-based treatment | IDFS |
NCT05560308 | T-DM1 + pyrotinib maleate | II | 50 | HER2+ MBC after progression on TKI | ORR |
Ongoing studies evaluating T-DXd associated with other agents in BC
Study | Agents | Phase | Number of patients | Patients | Primary endpoint |
NCT04539938 (HER2CLIMB-04) | Tucatinib + T-DXd | II | 70 | HER2-positive MBC progressing on trastuzumab + taxanes | ORR |
NCT05633979 | T-DXd + valemetostat | Ib | 37 | HER2-low/0 MBC | Safety MTD ORR RDE |
NCT05795101 (TRUDI) | T-DXd + durvalumab | II | 63 | Previously untreated with HER2+/low Inflammatory BC | pCR |
NCT05372614 | T-DXd + neratinib | I | 18 | Metastatic HER2-altered tumors | DLTs TEAEs |
NCT04538742 (DESTINY-Breast07) | T-DXd + durvalumab or tucatinib or pertuzumab or paclitaxel | I/II | 245 | Previously untreated HER2-positive MBC | AEs SAEs |
NCT04042701 | pembrolizumab + T-DXd | I | 115 | HER2-positive tumors after progression on T-DM1 or HER2-low MBC | DLTs ORR |
NCT04585958 | Olaparib + T-DXd | I | 55 | Metastatic HER2-altered tumors | MTD, RP2D AEs |
Next-generation ADCs
Regarding next-generation ADCs, the previously discussed DESTINY-Breast02 phase III trial is the first randomized study proving that an ADC (T-DXd) can overcome the resistance to another one (T-DM1)[35].
Trastuzumab duocarmazine, formerly named SYD985, is a HER2-targeting monoclonal antibody coupled to a duocarmycin (alkylating agent) via a C linker. The DAR of SYD985 is 2.6. It has been investigated in a phase I study enrolling patients with locally advanced or metastatic solid tumors with HER2 immunohistochemistry of 1+ or more. The study included patients with heavily pretreated metastatic BC. The dose-limiting toxicity was 2.4 mg/kg (related to pneumonitis) and the recommended phase 2 dose was 1.2 mg/kg. In the dose-expansion cohort, the ORR was 33% and 32% in patients with HER2-positive and HER2-low metastatic BC, respectively. Regarding safety profile, grade 3 or more AEs were observed in 35% of participants[71]. The TULIP phase III trial randomized patients with HER2-positive metastatic BC who failed at least two previous lines of treatment to either SYD985 or treatment of physician’s choice. Previous treatment with T-DM1 was allowed. The study met its primary endpoint of PFS. SYD985 significantly prolonged PFS in comparison with control group (7.0 vs. 4.9 months, HR: 0.64; P = 0.002). However, the final analysis of OS did not show a survival advantage with SYD985 (HR: 0.87; 95% CI, 0.68-1.12; P = 0.236) and both arms showed similar ORR. The rate of interstitial lung disease/pneumonitis was 7.6% (22/288 patients), including two grade 5 events (grade 1-2 in 5.2% of patients). To be noted that 21.2% of patients presented grade 3 or more ocular toxicities in the SYD985 group that led to treatment withdrawal in 20.8% of patients[72]. The ISPY-P1.01 is a phase I trial evaluating SYD985 in combination with paclitaxel in metastatic BC (NCT04602117).
Trastuzumab rezetecan (SHR-A1811) is a new-generation HER2-directed ADC composed of trastuzumab linked to a novel topoisomerase I inhibitor payload (SHR9265) via a stable and cleavable linker. It has been studied in a phase I first-in-human study including pretreated patients with HER2-mutated/expressing advanced solid tumors. The entire cohort has an ORR of 61.6% (154/250). Interestingly, patients with HER2-positive BC had an ORR of 81.5% (88/108 patients), while patients with HER2-low disease had an ORR of 55.8% (43/77). Moreover, patients with HER2-positive BC and previously treated with T-DM1 had an ORR of 82.4% (14/17). Fifteen patients had previously received an anti-HER2 ADC other than T-DM1, including T-DXd. The ORR was 60% in these patients (9/15)[73]. A166 is another HER2-directed ADC composed of humanized HER2-targeting antibody linked to a cytotoxic drug (Duostatin-5, a microtubule inhibitor agent) via a stable cleavable linker. In the cohort of HER2-positive patients, A166 was associated with an ORR of 70%. In addition, median PFS ranged between 9.4 and 12.3 months following the administered doses[74].
Disitamab vedotin (RC48) is a humanized anti-HER2 ADC conjugated with monomethyl auristatin E (MMAE) via a cleavable linker with a bystander effect on cancer cells. The DAR for RC48 is 4.1. In a preclinical model of HER2-positive BC metastatic to the lungs, tumor cells showed resistance to T-DM1 and T-DXd and were moderately sensitive to disitamab vedotin. In the mouse model, the three drugs inhibited the development of lung metastases, and T-DXd and disitamab vedotin were more efficacious than
XMT-1522 is another novel HER2-targeting ADC and was compared to T-DM1 in vitro and in vivo in gastric cancer and BC. Le Joncour et al. found that XMT-1522 showed efficacy in BC and gastric cancer cells expressing HER2 and xenograft models both resistant to T-DM1[77]. Moreover, ARX788 is an anti-HER2 ADC consisting of anti-HER2 mAb conjugated to amberstatin (AS269) cytotoxic payload. In vitro and
Bispecific or biparatopic mAbs are a promising solution to overcome or prevent resistance to ADCs. Li et al. engineered in vitro models of HER2-targeting bivalent biparatopic ADCs that demonstrated efficacy against T-DM1 resistant tumors as well as HER2-low expressing cancers. The benefit is due to the targeting of two non-overlapping epitopes on HER2 inducing HER2 receptor clustering and consequently robust internalization, lysosomal trafficking, and degradation[82]. Moreover, Zanidatamab zodovotin (ZW49) is a bispecific ADC targeting two non-overlapping HER2 antigens at the same time and linked to an auristatin payload via a C linker. Its DAR is 2. It has been evaluated in HER2-positive solid tumors in a phase I dose-escalation trial. Two dose-limiting toxicities have been reported at the dosage of 1.75 and 2.5 mg/kg. It should be noted that treatment-related keratitis was observed in 43% of patients. The DCR in the cohort of 2.5 mg/kg was 50% in patients with HER2-positive metastatic BC[83]. BIO-201 is a novel bispecific ADC that targets TROP-2 and HER2 conjugated with a topoisomerase I inhibitor via a cleavable linker. Preclinical data suggest that BIO-201 is effective in different types of cancer co-expressing TROP-2/HER2 or either of the targets[84].
As previously mentioned, HER3 overexpression can lead to resistance to anti-HER2 drugs. HER3 inhibition using HER3-targeting ADC such as patritumab deruxtecan could be an interesting area to investigate. Patritumab deruxtecan (HER3-DXd) is a HER3-targeting ADC coupled to TOPO1 inhibitor via a C linker. The DAR is 8. It has been evaluated in the U31402-A-J101 phase I/II trial in patients with HER3-expressing metastatic BC. Interestingly, in the subgroup of patients with HER2-positive BC (n = 14), HER3-DXd was associated with remarkable clinical activity with ORR of 42.9%, DCR of 92.9%, and median PFS of 11.0 months[85]. Zenocutuzumab is a bispecific antibody targeting HER2 and HER3 simultaneously. It has been evaluated in patients with cancers harboring NRG1 fusion. In a phase II trial, responses to zenocutuzumab were observed in 34% of patients (35% in patients with NSCLC, 39% in patients with pancreatic adenocarcinoma, and 50% in patients with BC)[86].
Another strategy to overcome resistance to ADC and increase sensitivity to these agents is the development of non-internalizing ADCs that could target the tumor microenvironment (TME). In fact, the TME, mainly composed of extracellular matrix, abnormal blood vessels and stromal cells such as cancer-associated fibroblasts, plays a crucial role in the growth of tumor cells, neoangiogenesis, and the removal of metabolic waste[87]. The TME is associated with some antigens that include enzymes, transcription factors, and checkpoint molecules[88]. Several novel ADCs are under clinical development and evaluation in hematological malignancies and solid tumors targeting CD74, CCR7, and CD276[89,90].
Combination strategies with ADC
Multiple combinations are currently under investigation to overcome acquired resistance to HER2-targeting ADCs and improve the outcomes after failure of these drugs. The most evaluated and promising associations are ADCs with TKI, ICIs, and DNA-damaging agents such as PolyADP-Ribose Polymerase-1 (PARP) inhibitors. The HER2CLIMB-02 is the first phase III study to compare tucatinib combined with
Regarding the combination with ICIs, the KATE2 phase II randomized trial was the first study to evaluate the combination of T-DM1 with atezolizumab, a programmed death ligand-1 (PD-L1), in patients with previously treated HER2-positive metastatic BC. The trial did not meet its primary endpoint of PFS in the intention-to-treat population (8.2 vs. 6.8 months; HR: 0.82, 95%CI: 0.55-1.23; P = 0.33), showing that the addition of atezolizumab to T-DM1 did not improve PFS and was associated with more adverse events[92]. The subsequent KATE3, randomized phase III trial is comparing T-DM1 in combination with atezolizumab vs. T-DM1 alone in patients with previously treated HER2-positive and PD-L1-positive metastatic BC (NCT04740918)[93]. Moreover, the ASTEFANIA phase III trial is also evaluating T-DM1 and atezolizumab or placebo in patients with HER2-positive BC at a high risk of recurrence following neoadjuvant chemotherapy (NCT04873362)[94]. The combination of T-DXd with nivolumab has also been evaluated in a phase 1b trial in patients with HER2-positive or HER2-low metastatic BC. In the HER2-positive metastatic BC, the combination was associated with antitumor activity consistent with prior results of T-DXd alone, raising the question of the utility of adding ICIs to ADCs, which requires more follow-up and additional studies[95].
CONCLUSION
As previously mentioned, the HER2-targeting landscape has dramatically changed the treatment landscape of patients with HER2-positive BC as well as HER2-low metastatic BC. To date, two drugs are approved by the FDA and EMA for patients with HER2-expressing breast cancer. T-DM1 is the first approved ADC and is currently indicated as adjuvant treatment in patients with HER2-positive BC with residual disease following neo-adjuvant trastuzumab-base chemotherapy. T-DXd is the second HER2-targeting approved ADC in HER2-positive and HER2-low metastatic BC. It defeated T-DM1 in a head-to-head comparison in second-line settings in patients with HER2-positive metastatic BC and sparked the debate on the efficacy of T-DM1 as monotherapy in subsequent therapeutic lines. The impressive clinical efficacy of T-DXd is counterbalanced by the emergence of resistance to this agent and the absence of clinical data and standardized consensus for the treatment of patients after progression on T-DXd. The preferred available regimens could be T-DM1 or the combination of capecitabine, tucatinib, and trastuzumab following the results of the HER2CLIMB phase III trial that showed statistically longer median OS in comparison with capecitabine and trastuzumab[96]. The combination of T-DM1 and tucatinib could be another option. Moreover, ongoing clinical trials are assessing T-DXd as a potential first-line treatment, comparing it with the current standard of care and exploring its use in the adjuvant setting. If approved for these indications, it will further complicate the treatment landscape for patients with HER2-positive breast cancer. Investigating next-generation ADCs is crucial, with a need for more efforts to elucidate their mechanisms of action, pharmacodynamic and pharmacokinetic profiles, and the molecular drivers of resistance, which remain poorly understood. Another pertinent concern is whether an ADC can effectively reverse the resistance to another ADC. While this has been demonstrated with
DECLARATIONS
Authors’ contributions
Conceptualization and writing original draft: Saleh K, Khoury R, Khalife N
Writing review and editing: Chahine C, Ibrahim R, Tikriti Z
Supervising, writing-review, and editing: Le Cesne A
All authors have read and agreed to the published version of the manuscript.
Availability of data and materials
Not applicable.
Financial support and sponsorship
None.
Conflicts of interest
All authors declared that there are no conflicts of interest.
Ethical approval and consent to participate
Not applicable.
Consent for publication
Not applicable.
Copyright
© The Author(s) 2024
REFERENCES
2. Pauletti G, Godolphin W, Press MF, Slamon DJ. Detection and quantitation of HER-2/neu gene amplification in human breast cancer archival material using fluorescence in situ hybridization. Oncogene 1996;13:63-72.
3. Yarden Y, Sliwkowski MX. Untangling the ErbB signalling network. Nat Rev Mol Cell Biol 2001;2:127-37.
4. Spector NL, Blackwell KL. Understanding the mechanisms behind trastuzumab therapy for human epidermal growth factor receptor 2-positive breast cancer. J Clin Oncol 2009;27:5838-47.
5. Choong GM, Cullen GD, O’Sullivan CC. Evolving standards of care and new challenges in the management of HER2-positive breast cancer. CA Cancer J Clin 2020;70:355-74.
6. Slamon DJ, Leyland-Jones B, Shak S, et al. Use of chemotherapy plus a monoclonal antibody against HER2 for metastatic breast cancer that overexpresses HER2. N Engl J Med 2001;344:783-92.
7. Swain SM, Baselga J, Kim SB, et al; CLEOPATRA Study Group. Pertuzumab, trastuzumab, and docetaxel in HER2-positive metastatic breast cancer. N Engl J Med 2015;372:724-34.
8. Gianni L, Pienkowski T, Im YH, et al. Efficacy and safety of neoadjuvant pertuzumab and trastuzumab in women with locally advanced, inflammatory, or early HER2-positive breast cancer (NeoSphere): a randomised multicentre, open-label, phase 2 trial. Lancet Oncol 2012;13:25-32.
9. Guidi L, Pellizzari G, Tarantino P, Valenza C, Curigliano G. Resistance to antibody-drug conjugates targeting HER2 in breast cancer: molecular landscape and future challenges. Cancers 2023;15:1130.
10. Verma S, Miles D, Gianni L, et al; EMILIA Study Group. Trastuzumab emtansine for HER2-positive advanced breast cancer. N Engl J Med 2012;367:1783-91.
11. Diéras V, Miles D, Verma S, et al. Trastuzumab emtansine versus capecitabine plus lapatinib in patients with previously treated HER2-positive advanced breast cancer (EMILIA): a descriptive analysis of final overall survival results from a randomised, open-label, phase 3 trial. Lancet Oncol 2017;18:732-42.
12. Cortés J, Kim SB, Chung WP, et al; DESTINY-Breast03 Trial Investigators. Trastuzumab deruxtecan versus trastuzumab emtansine for breast cancer. N Engl J Med 2022;386:1143-54.
13. Hurvitz SA, Hegg R, Chung WP, et al. Trastuzumab deruxtecan versus trastuzumab emtansine in patients with HER2-positive metastatic breast cancer: updated results from DESTINY-Breast03, a randomised, open-label, phase 3 trial. Lancet 2023;401:105-17.
15. Criscitiello C, Morganti S, Curigliano G. Antibody-drug conjugates in solid tumors: a look into novel targets. J Hematol Oncol 2021;14:20.
16. Hoffmann RM, Coumbe BGT, Josephs DH, et al. Antibody structure and engineering considerations for the design and function of Antibody Drug Conjugates (ADCs). Oncoimmunology 2018;7:e1395127.
17. De Cecco M, Galbraith DN, McDermott LL. What makes a good antibody-drug conjugate? Expert Opin Biol Ther 2021;21:841-7.
18. Chang HL, Schwettmann B, McArthur HL, Chan IS. Antibody-drug conjugates in breast cancer: overcoming resistance and boosting immune response. J Clin Invest 2023;133:e172156.
19. Diamantis N, Banerji U. Antibody-drug conjugates - an emerging class of cancer treatment. Br J Cancer 2016;114:362-7.
21. Drago JZ, Modi S, Chandarlapaty S. Unlocking the potential of antibody-drug conjugates for cancer therapy. Nat Rev Clin Oncol 2021;18:327-44.
22. Lu J, Jiang F, Lu A, Zhang G. Linkers having a crucial role in antibody-drug conjugates. Int J Mol Sci 2016;17:561.
23. Khoury R, Saleh K, Khalife N, et al. Mechanisms of resistance to antibody-drug conjugates. Int J Mol Sci 2023;24:9674.
24. Sun X, Ponte JF, Yoder NC, et al. Effects of drug-antibody ratio on pharmacokinetics, biodistribution, efficacy, and tolerability of antibody-maytansinoid conjugates. Bioconjug Chem 2017;28:1371-81.
25. Junttila TT, Li G, Parsons K, Phillips GL, Sliwkowski MX. Trastuzumab-DM1 (T-DM1) retains all the mechanisms of action of trastuzumab and efficiently inhibits growth of lapatinib insensitive breast cancer. Breast Cancer Res Treat 2011;128:347-56.
26. Lewis Phillips GDL, Li G, Dugger DL, et al. Targeting HER2-positive breast cancer with trastuzumab-DM1, an antibody-cytotoxic drug conjugate. Cancer Res 2008;68:9280-90.
27. Hunter FW, Barker HR, Lipert B, et al. Mechanisms of resistance to trastuzumab emtansine (T-DM1) in HER2-positive breast cancer. Br J Cancer 2020;122:603-12.
28. Perez EA, Barrios C, Eiermann W, et al. Trastuzumab emtansine with or without pertuzumab versus trastuzumab plus taxane for human epidermal growth factor receptor 2-positive, advanced breast cancer: primary results from the phase III MARIANNE study. J Clin Oncol 2017;35:141-8.
29. Hurvitz SA, Martin M, Symmans WF, et al. Neoadjuvant trastuzumab, pertuzumab, and chemotherapy versus trastuzumab emtansine plus pertuzumab in patients with HER2-positive breast cancer (KRISTINE): a randomised, open-label, multicentre, phase 3 trial. Lancet Oncol 2018;19:115-26.
30. Hurvitz SA, Martin M, Jung KH, et al. Neoadjuvant trastuzumab emtansine and pertuzumab in human epidermal growth factor receptor 2-positive breast cancer: three-year outcomes from the phase III KRISTINE study. J Clin Oncol 2019;37:2206-16.
31. von Minckwitz G, Huang CS, Mano MS, et al; KATHERINE Investigators. Trastuzumab emtansine for residual invasive HER2-positive breast cancer. N Engl J Med 2019;380:617-28.
32. Ogitani Y, Aida T, Hagihara K, et al. DS-8201a, a novel HER2-targeting ADC with a novel DNA topoisomerase I inhibitor, demonstrates a promising antitumor efficacy with differentiation from T-DM1. Clin Cancer Res 2016;22:5097-108.
33. Yver A, Agatsuma T, Soria JC. The art of innovation: clinical development of trastuzumab deruxtecan and redefining how antibody-drug conjugates target HER2-positive cancers. Ann Oncol 2020;31:430-4.
34. Modi S, Saura C, Yamashita T, et al; DESTINY-Breast01 Investigators. Trastuzumab deruxtecan in previously treated HER2-positive breast cancer. N Engl J Med 2020;382:610-21.
35. André F, Hee Park Y, Kim SB, et al. Trastuzumab deruxtecan versus treatment of physician’s choice in patients with HER2-positive metastatic breast cancer (DESTINY-Breast02): a randomised, open-label, multicentre, phase 3 trial. Lancet 2023;401:1773-85.
36. Modi S, Jacot W, Yamashita T, et al; DESTINY-Breast04 Trial Investigators. Trastuzumab deruxtecan in previously treated HER2-low advanced breast cancer. N Engl J Med 2022;387:9-20.
37. Bartsch R, Berghoff AS, Furtner J, et al. Trastuzumab deruxtecan in HER2-positive breast cancer with brain metastases: a single-arm, phase 2 trial. Nat Med 2022;28:1840-7.
38. Pérez-García JM, Vaz Batista M, Cortez P, et al. Trastuzumab deruxtecan in patients with central nervous system involvement from HER2-positive breast cancer: The DEBBRAH trial. Neuro Oncol 2023;25:157-66.
39. Jerusalem G, Park YH, Yamashita T, et al. Trastuzumab deruxtecan in HER2-positive metastatic breast cancer patients with brain metastases: a DESTINY-Breast01 subgroup analysis. Cancer Discov 2022;12:2754-62.
40. Jacobson A. Trastuzumab deruxtecan improves progression-free survival and intracranial response in patients with HER2-positive metastatic breast cancer and brain metastases. Oncologist 2022;27:S3-4.
41. Venetis K, Crimini E, Sajjadi E, et al. HER2 Low, Ultra-low, and novel complementary biomarkers: expanding the spectrum of HER2 positivity in breast cancer. Front Mol Biosci 2022;9:834651.
42. Loganzo F, Tan X, Sung M, et al. Tumor cells chronically treated with a trastuzumab-maytansinoid antibody-drug conjugate develop varied resistance mechanisms but respond to alternate treatments. Mol Cancer Ther 2015;14:952-63.
43. Filho OM, Viale G, Stein S, et al. Impact of HER2 heterogeneity on treatment response of early-stage HER2-positive breast cancer: phase II neoadjuvant clinical trial of T-DM1 combined with pertuzumab. Cancer Discov 2021;11:2474-87.
44. Gebhart G, Lamberts LE, Wimana Z, et al. Molecular imaging as a tool to investigate heterogeneity of advanced HER2-positive breast cancer and to predict patient outcome under trastuzumab emtansine (T-DM1): the ZEPHIR trial. Ann Oncol 2016;27:619-24.
45. Scaltriti M, Rojo F, Ocaña A, et al. Expression of p95HER2, a truncated form of the HER2 receptor, and response to anti-HER2 therapies in breast cancer. J Natl Cancer Inst 2007;99:628-38.
46. Phillips GD, Fields CT, Li G, et al. Dual targeting of HER2-positive cancer with trastuzumab emtansine and pertuzumab: critical role for neuregulin blockade in antitumor response to combination therapy. Clin Cancer Res 2014;20:456-68.
47. Schwarz LJ, Hutchinson KE, Rexer BN, et al. An ERBB1-3 neutralizing antibody mixture with high activity against drug-resistant HER2+ breast cancers with ERBB ligand overexpression. J Natl Cancer Inst 2017;109:djx065.
48. Zhang M, Li B, Liao H, et al. Targeting HER3 or MEK overcomes acquired Trastuzumab resistance in HER2-positive gastric cancer-derived xenograft. Cell Death Discov 2022;8:478.
49. Yu SF, Zheng B, Go M, et al. A novel anti-CD22 anthracycline-based antibody-drug conjugate (ADC) that overcomes resistance to auristatin-based ADCs. Clin Cancer Res 2015;21:3298-306.
50. Takegawa N, Nonagase Y, Yonesaka K, et al. DS-8201a, a new HER2-targeting antibody-drug conjugate incorporating a novel DNA topoisomerase I inhibitor, overcomes HER2-positive gastric cancer T-DM1 resistance. Int J Cancer 2017;141:1682-9.
51. Kalim M, Chen J, Wang S, et al. Intracellular trafficking of new anticancer therapeutics: antibody-drug conjugates. Drug Des Devel Ther 2017;11:2265-76.
52. Sung M, Tan X, Lu B, et al. Caveolae-mediated endocytosis as a novel mechanism of resistance to trastuzumab emtansine (T-DM1). Mol Cancer Ther 2018;17:243-53.
53. Mayor S, Pagano RE. Pathways of clathrin-independent endocytosis. Nat Rev Mol Cell Biol 2007;8:603-12.
54. Indira Chandran V, Månsson AS, Barbachowska M, et al. Hypoxia attenuates trastuzumab uptake and trastuzumab-emtansine
55. Pereira PMR, Sharma SK, Carter LM, et al. Caveolin-1 mediates cellular distribution of HER2 and affects trastuzumab binding and therapeutic efficacy. Nat Commun 2018;9:5137.
56. Baldassarre T, Truesdell P, Craig AW. Endophilin A2 promotes HER2 internalization and sensitivity to trastuzumab-based therapy in HER2-positive breast cancers. Breast Cancer Res 2017;19:110.
57. Ríos-Luci C, García-Alonso S, Díaz-Rodríguez E, et al. Resistance to the antibody-drug conjugate T-DM1 is based in a reduction in lysosomal proteolytic activity. Cancer Res 2017;77:4639-51.
58. Hamblett KJ, Jacob AP, Gurgel JL, et al. SLC46A3 is required to transport catabolites of noncleavable antibody maytansine conjugates from the lysosome to the cytoplasm. Cancer Res 2015;75:5329-40.
59. Mosele F, Deluche E, Lusque A, et al. Trastuzumab deruxtecan in metastatic breast cancer with variable HER2 expression: the phase 2 DAISY trial. Nat Med 2023;29:2110-20.
60. Yu M, Ocana A, Tannock IF. Reversal of ATP-binding cassette drug transporter activity to modulate chemoresistance: why has it failed to provide clinical benefit? Cancer Metastasis Rev 2013;32:211-27.
61. Lambert JM, Chari RV. Ado-trastuzumab Emtansine (T-DM1): an antibody-drug conjugate (ADC) for HER2-positive breast cancer. J Med Chem 2014;57:6949-64.
62. Li G, Guo J, Shen BQ, et al. Mechanisms of acquired resistance to trastuzumab emtansine in breast cancer cells. Mol Cancer Ther 2018;17:1441-53.
63. Berns K, Horlings HM, Hennessy BT, et al. A functional genetic approach identifies the PI3K pathway as a major determinant of trastuzumab resistance in breast cancer. Cancer Cell 2007;12:395-402.
64. Baselga J, Lewis Phillips GD, Verma S, et al. Relationship between tumor biomarkers and efficacy in EMILIA, a phase III study of trastuzumab emtansine in HER2-positive metastatic breast cancer. Clin Cancer Res 2016;22:3755-63.
65. Wu Y, Ginther C, Kim J, et al. Expression of Wnt3 activates Wnt/β-catenin pathway and promotes EMT-like phenotype in trastuzumab-resistant HER2-overexpressing breast cancer cells. Mol Cancer Res 2012;10:1597-606.
66. Yao L, Chen J, Ma W. Decoding TROP2 in breast cancer: significance, clinical implications, and therapeutic advancements. Front Oncol 2023;13:1292211.
67. Gion M, García-Mosquera JJ, Pérez-García JM, et al. Correlation between trophoblast cell-surface antigen-2 (Trop-2) expression and pathological complete response in patients with HER2-positive early breast cancer treated with neoadjuvant docetaxel, carboplatin, trastuzumab, and pertuzumab. Breast Cancer Res Treat 2024;205:589-98.
68. Arthi M, Arun Kumar K, D’Cruze L, S R, Joseph LD, Dev B. The significance of topoisomerase II alpha in invasive breast carcinoma. Cureus 2021;13:e18733.
69. Fountzilas G, Valavanis C, Kotoula V, et al. HER2 and TOP2A in high-risk early breast cancer patients treated with adjuvant epirubicin-based dose-dense sequential chemotherapy. J Transl Med 2012;10:10.
70. Durbin KR, Phipps C, Liao X. Mechanistic modeling of antibody-drug conjugate internalization at the cellular level reveals inefficient processing steps. Mol Cancer Ther 2018;17:1341-51.
71. Banerji U, van Herpen CML, Saura C, et al. Trastuzumab duocarmazine in locally advanced and metastatic solid tumours and HER2-expressing breast cancer: a phase 1 dose-escalation and dose-expansion study. Lancet Oncol 2019;20:1124-35.
72. Aftimos P, Turner N, O'shaughnessy J, et al. 386MO Trastuzumab duocarmazine versus physician’s choice therapy in pre-treated HER2-positive metastatic breast cancer: Final results of the phase III TULIP trial. Ann Oncol 2023;34:S340-1.
73. Yao H, Yan M, Tong Z, et al. Abstract CT175: Safety, tolerability, pharmacokinetics, and antitumor activity of SHR-A1811 in HER2-expressing/mutated advanced solid tumors: a global phase 1, multi-center, first-in-human study. Cancer Res 2023;83:CT175.
74. Zhang J, Liu R, Gao S, et al. Phase I study of A166, an antibody-drug conjugate in advanced HER2-expressing solid tumours. NPJ Breast Cancer 2023;9:28.
75. Pourjamal N, Yazdi N, Halme A, et al. Comparison of trastuzumab emtansine, trastuzumab deruxtecan, and disitamab vedotin in a multiresistant HER2-positive breast cancer lung metastasis model. Clin Exp Metastasis 2024;41:91-102.
76. Wang J, Liu Y, Zhang Q, et al. RC48-ADC, a HER2-targeting antibody-drug conjugate, in patients with HER2-positive and HER2-low expressing advanced or metastatic breast cancer: a pooled analysis of two studies. J Clin Oncol 2021;39:1022.
77. Le Joncour V, Martins A, Puhka M, et al. A novel anti-HER2 antibody-drug conjugate XMT-1522 for HER2-positive breast and gastric cancers resistant to trastuzumab emtansine. Mol Cancer Ther 2019;18:1721-30.
78. Skidmore L, Sakamuri S, Knudsen NA, et al. ARX788, a site-specific anti-HER2 antibody-drug conjugate, demonstrates potent and selective activity in HER2-low and T-DM1-resistant breast and gastric cancers. Mol Cancer Ther 2020;19:1833-43.
79. Barok M, Le Joncour V, Martins A, et al. ARX788, a novel anti-HER2 antibody-drug conjugate, shows anti-tumor effects in preclinical models of trastuzumab emtansine-resistant HER2-positive breast cancer and gastric cancer. Cancer Lett 2020;473:156-63.
80. Hu X, Zhang J, Ji D, et al. Abstract P1-18-16: A phase 1 study of ARX788, a HER2-targeting antibody-drug conjugate, in patients with metastatic HER2-positive breast cancer. Cancer Res 2020;80:P1-18-16.
81. ACE-breast-02 pivotal phase 3 study of Ambrx’s ARX788 for the treatment of HER2 positive metastatic breast cancer achieves positive results. Available from: https://ir.ambrx.com/news/news-details/2023/ACE-Breast-02-Pivotal-Phase-3-Study-of-Ambrxs-ARX788-for-the-Treatment-of-HER2-Positive-Metastatic-Breast-Cancer-Achieves-Positive-Results/default.aspx. [Last accessed on 11 May 2024].
82. Li JY, Perry SR, Muniz-Medina V, et al. A biparatopic HER2-targeting antibody-drug conjugate induces tumor regression in primary models refractory to or ineligible for HER2-targeted therapy. Cancer Cell 2016;29:117-29.
83. Jhaveri K, Han H, Dotan E, et al. 460MO Preliminary results from a phase I study using the bispecific, human epidermal growth factor 2 (HER2)-targeting antibody-drug conjugate (ADC) zanidatamab zovodotin (ZW49) in solid cancers. Ann Oncol 2022;33:S749-50.
84. Zhong H, Xiong Y, Huang H, et al. Preclinical antitumor activity of an anti-HER2/Trop-2 bispecific antibody-drug conjugate with a new DNA topoisomerase I inhibitor. J Clin Oncol 2023;41:e15013.
85. Krop IE, Masuda N, Mukohara T, et al. Patritumab deruxtecan (HER3-DXd), a human epidermal growth factor receptor 3-directed antibody-drug conjugate, in patients with previously treated human epidermal growth factor receptor 3-expressing metastatic breast cancer: a multicenter, phase I/II trial. J Clin Oncol 2023;41:5550-60.
86. Schram AM, Goto K, Kim D, et al. Efficacy and safety of zenocutuzumab, a HER2 x HER3 bispecific antibody, across advanced NRG1 fusion (NRG1+) cancers. J Clin Oncol 2022;40:105.
87. de Visser KE, Joyce JA. The evolving tumor microenvironment: from cancer initiation to metastatic outgrowth. Cancer Cell 2023;41:374-403.
89. Ziogas DC, Theocharopoulos C, Lialios PP, et al. Beyond CTLA-4 and PD-1 inhibition: novel immune checkpoint molecules for melanoma treatment. Cancers 2023;15:2718.
90. Ceci C, Lacal PM, Graziani G. Antibody-drug conjugates: resurgent anticancer agents with multi-targeted therapeutic potential. Pharmacol Ther 2022;236:108106.
91. Goodman A. HER2CLIMB-02: Tucatinib plus T-DM1 extends progression-free survival vs T-DM1 alone in advanced HER2-positive breast cancer - the ASCO post. 2023. Available from: https://ascopost.com/issues/december-25-2023/tucatinib-plus-t-dm1-extends-progression-free-survival-vs-t-dm1-alone-in-advanced-her2-positive-breast-cancer/. [Last accessed on 11 May 2024].
92. Emens LA, Esteva FJ, Beresford M, et al. Trastuzumab emtansine plus atezolizumab versus trastuzumab emtansine plus placebo in previously treated, HER2-positive advanced breast cancer (KATE2): a phase 2, multicentre, randomised, double-blind trial. Lancet Oncol 2020;21:1283-95.
93. Loi S, Schneeweiss A, Song E, et al. 329TiP KATE3: a phase III study of trastuzumab emtansine (T-DM1) in combination with atezolizumab or placebo in patients with previously treated HER2-positive and PD-L1-positive locally advanced or metastatic breast cancer. Ann Oncol 2021;32:S509.
94. Hurvitz SA, Bachelot T, Bianchini G, et al. ASTEFANIA: adjuvant ado-trastuzumab emtansine and atezolizumab for high-risk, HER2-positive breast cancer. Future Oncol 2022;18:3563-72.
95. Hamilton E, Shapiro CL, Petrylak D, et al. Abstract PD3-07: trastuzumab deruxtecan (T-DXd; DS-8201) with nivolumab in patients with HER2-expressing, advanced breast cancer: a 2-part, phase 1b, multicenter, open-label study. Cancer Res 2021;81:PD3-07.
Cite This Article

How to Cite
Saleh, K.; Khoury R.; Khalife N.; Chahine C.; Ibrahim R.; Tikriti Z.; Le Cesne A. Mechanisms of action and resistance to anti-HER2 antibody-drug conjugates in breast cancer. Cancer. Drug. Resist. 2024, 7, 22. http://dx.doi.org/10.20517/cdr.2024.06
Download Citation
Export Citation File:
Type of Import
Tips on Downloading Citation
Citation Manager File Format
Type of Import
Direct Import: When the Direct Import option is selected (the default state), a dialogue box will give you the option to Save or Open the downloaded citation data. Choosing Open will either launch your citation manager or give you a choice of applications with which to use the metadata. The Save option saves the file locally for later use.
Indirect Import: When the Indirect Import option is selected, the metadata is displayed and may be copied and pasted as needed.
About This Article
Copyright
Data & Comments
Data
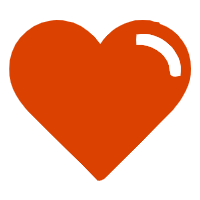
Comments
Comments must be written in English. Spam, offensive content, impersonation, and private information will not be permitted. If any comment is reported and identified as inappropriate content by OAE staff, the comment will be removed without notice. If you have any queries or need any help, please contact us at support@oaepublish.com.