Advancing CAR T-cell therapy for chronic lymphocytic leukemia: exploring resistance mechanisms and the innovative strategies to overcome them
Abstract
Chimeric antigen receptor (CAR) T-cell therapy has ushered in substantial advancements in the management of various B-cell malignancies. However, its integration into chronic lymphocytic leukemia (CLL) treatment has been challenging, attributed largely to the development of very effective chemo-free alternatives. Additionally, CAR
Keywords
INTRODUCTION
The successful development of genetically engineered chimeric antigen receptor (CAR) T-cell therapy has led to remarkable progress in the treatment of hematological malignancies[1,2]. Trials utilizing CAR T-cell therapy have demonstrated impressive overall response rates (ORR) of more than 80% in the treatment of relapsed/refractory (r/r) B-cell acute lymphoblastic leukemia (B-ALL), diffuse large B-cell lymphoma (DLBCL), and mantle cell lymphoma (MCL) and provide a potential for cure in 30%-40% of patients with DLBCL[3]. However, there has been less enthusiasm for CAR T-cells in chronic lymphocytic leukemia (CLL) than in other non-Hodgkin lymphomas (NHL) for several reasons. For one, the clinical course of CLL is generally insidious, and asymptomatic patients may go years without needing therapy. The median age for diagnosis is 70 years, and CAR T-cell therapy may not be appropriate for some elderly patients. However, real-world analysis of CAR T-cell therapy in older patients showed acceptable efficacy and safety even in individuals aged 75 years or older[4]. When treatment is indicated, effective, chemotherapy-free options such as Bruton tyrosine kinase (BTK) and B-cell lymphoma-2 (BCL2) inhibitors are preferred[5,6]. Additionally, CAR T-cell therapy has demonstrated less reliable ORR in larger studies ranging from 43%-79%, with much lower complete responses (CR)[7-9]. Nevertheless, treatment options remain limited in patients who are refractory to both BTKi and BCL2 inhibitors, particularly in those who have high-risk cytogenetics such as complex karyotype, unmuted immunoglobulin heavy chain gene mutation (IGHV), 17p deletion, and TP53 mutation[10,11]. In this setting, CAR T-cell therapy shows great promise, particularly in the challenging multi-agent refractory, high-risk population. Encouraging data from the TRANSCEND CLL 004 trial[9] using the CD19 CAR T-cell product, lisocabtagene maraleucel (liso-cel), has resulted in the first Food and Drug Administration (FDA) approval for CAR T-cell therapy in CLL. In March 2024, liso-cel was approved for the treatment of CLL patients who are relapsed or refractory to at least two prior lines including BTK and BCL2 inhibitors. This marks a significant milestone in the use of CAR T-cells in CLL, yet ongoing research is needed to further improve patient outcomes. This review will discuss current data using CAR T-cell therapy in CLL, possible resistance mechanisms, and ongoing strategies to augment their safety and efficacy.
THE EVOLUTION OF CAR T-CELLS
CARs are receptor proteins that are engineered on immune cells, most commonly T-cells, allowing them to target specific antigens. CARs can be constructed on a patient’s own T-cells (autologous CARs) or on healthy donor T-cells (allogeneic CARs). CARs contain an extracellular domain with an antigen-specific single-chain variable fragment (scFv), a transmembrane domain, and an intracellular signaling domain[2]. First-generation CARs that harbored only a CD3ζ chain were limited in promoting T-cell activation and expansion[12,13]. The second-generation CARs also included a costimulatory domain, most notably CD28 or 4-1BB, which enhanced CAR T-cell expansion, cytokine production, and cell survival[14]. Second-generation CAR T-cells are the predominant CARs used currently in all the FDA-approved products. Third-generation CARs include multiple costimulatory domains, potentially leading to even better expansion and persistence. Lastly, fourth-generation CARs have a transgenic “payload”, also known as T-cells Redirected towards Universal Cytokine Killing (TRUCKs), which include additional anti-tumor activity such as inducible cytokine production, such as IL-12, IL-15, IL-18, and IL-23 or stimulatory ligands to influence the local tumor microenvironment (TME) and reduce systemic toxicity[15-20]. Figure 1 illustrates the four different generations of CAR T-cells. To date, the FDA has approved 4 CD19-directed CAR T-cell products for B-cell malignancies, including axicabtagene ciloleucel (axi-cel) and brexucabtagene autoleucel (brex-cel) both harboring a CD28 costimulatory domain, and liso-cel and tisagenlecleucel (tisa-cel) harboring a 4-1BB costimulatory domain. In addition to variances in the CAR structure, manufacturing differences may also be important in different types of lymphoma. For example, although brex-cel and axi-cel are the same product, brex-cel undergoes an additional T-cell selection step during manufacturing. This step aims to remove contamination from circulating leukemic cell involvement, which is more frequently seen in MCL and CLL than other NHL types[21]. Additionally, the liso-cel product uniquely processes and manufactures CD4:CD8 CAR T-cells in a 1:1 ratio. Consequently, despite potentially heterogeneous starting materials, this approach minimizes variability in cell frequencies and enhances T-cell purity through T-cell-specific activation and expansion[9]. As CAR constructs evolve and manufacturing processes refine, CAR T-cell therapy is poised to advance in both safety and effectiveness, ultimately becoming more widely accessible.
Figure 1. Structure of the 4 generations of CAR T-cells. First-generation (1G) CARs contain an antigen recognition domain, scFv with a variable heavy and light chain connected by a linker, a transmembrane domain, and a signaling domain (CD3ζ). Second-generation (2G) CARs are similar to 1G with the addition of a costimulatory domain such as CD28 or 4-1BB. Third-generation (3G) CARs are the same as the 2G with the addition of a second costimulatory domain. Fourth-generation (4G) CARs, also known as “armored” CARs, also produce NFATs, which release a transgenic product (such as the cytokine IL-12) that can further regulate the CAR T-cell function and influence the tumor microenvironment. CAR: Chimeric antigen receptor; scFv: single-chain variable fragment; NFATs: nuclear factor of activated T-cells; IL: interleukin.
CURRENT EFFICACY AND SAFETY OF CAR T-CELLS IN CLL
One of the earliest reports of successful CAR T-cells use in B-cell malignancies was reported in 2 r/r CLL patients who achieved a CR with tisa-cel[22]. In a recent update, these patients still have ongoing minimal residual disease (MRD) negative remissions a decade later with persistent circulating CAR T-cells[23]. Since that initial account, however, the use of several CD19 CAR T-cell products has been reported in CLL with varying success rates. In some studies, response rates were particularly high. For instance, Turtle et al. presented a study involving 24 CLL patients who had previously been treated with ibrutinib exposure and subsequently received CD19 CAR-T cell therapy[24]. Almost all the patients had high-risk disease with a median of 5 prior lines of therapy, 96% with complex karyotype and/or del17p and 5 patients with Richter’s transformation. The ORR by imaging criteria was 75%, and 88% of patients with bone marrow disease had clearance of their CLL cells[24]. Cappell et al. reported findings on the long-term outcomes of using the
Subsequent studies have depicted lower response rates; however, they repeatedly illustrate that patients who achieve a CR sustain long-term durable responses. Outcomes in 42 CLL patients randomized to receive low (5 × 107 cells/kg) vs. high-dose (5 × 108 cells/kg) CD19 CAR T-cells[8] demonstrated CR and ORR of 28% and 44% at 4 weeks, respectively. The progression-free survival (PFS) and overall survival (OS) were prolonged in those who achieved a CR (40.2 months PFS, not reached OS) vs. those who have not achieved a CR
Lastly, the use of CAR T-cell therapy in patients with Richter Transformation (RT) is not well defined, mostly due to the rarity of RT[29]. A multi-institutional retrospective study was recently reported, which included 62 patients with RT who received CD19 CAR T-cells[30]. The ORR was 65% and CR was 47% after a median follow-up of 24.1 months, with a median PFS of 4.7 months and OS 8.5 months. However, the median duration of response was not reached among those who achieved a CR and only 2.3 months among those with a PR[30]. There is currently an ongoing Phase 2 study using brex-cel in rare B-cell malignancies including r/r RT (ZUMA-25; NCT05537766). Despite varying response rates, these studies highlight CAR T-cell therapy’s potential as a viable treatment option, even offering long-term durable remissions for some patients with limited alternatives.
PROPOSED RESISTANCE MECHANISMS IN CLL
The reason for lower responses observed with CAR T-cell therapy in CLL compared to other B-cell malignancies is not completely understood, but two predominant hypotheses proposed include impaired
Figure 2. Mechanisms of impaired T-cells and immunosuppressive environment in CLL. (1) T-cells from patients with CLL demonstrate a diminished CD4 to CD8 ratio and increased numbers of Tregs. (2) Tregs, in turn, further cause increased IL-2 production, reducing Th1 differentiation. (3) Increased expression of inhibitory cytokines such as IL-4 causes upregulation of the anti-apoptotic signal, BCL2. (4) T-cells have impaired synapse formation with APCs from impaired actin polymerization. (5) Increased expression of T-cell exhaustion markers such as PD-1. (6) An immunosuppressive environment composed of multiple inhibitory cells further diminishes T-cell function and supports CLL cell survival. CLL: Chronic lymphocytic leukemia; Treg: T-regulatory cell; IL: interleukin; Th1: T-helper type 1; BCL2:
Figure 3. Resistance mechanisms. (A) Tumor-specific mechanisms are mostly due to selective pressure and include (1) proliferation of existing antigen-negative clones; (2) development of new genetic mutations within the antigen gene locus, loss of heterogeneity, or alternative splicing; (3) lineage switching, whereas the primary neoplastic cell becomes another type of cell (i.e., from lymphoid to myeloid); and (4) trogocytosis where the antigen of interest transfers to the CAR T-cell, leading to CAR T-cells killing each other (“fratricide”), and proliferation of the antigen-negative neoplastic cells; (B) CAR T-specific mechanisms include having CAR T-cells that have exhaustion phenotypes (i.e., expression of PD-1, LAG-3), which leads to impaired expansion, cytotoxicity, and persistence; (C) TME-specific mechanisms are due to immunosuppressive cells, inhibitory cytokines, and vesicles that protect CLL cells and cause impaired CAR T-cell function. CAR: Chimeric antigen receptor; PD-1: programmed death-1; LAG-3: lymphocyte-activation gene 3; TME: tumor microenvironment; CLL: chronic lymphocytic leukemia; LOH: loss of heterogeneity; PD-L1: program death ligand 1; CAF: cancer-associated fibroblast; MSCs: mesenchymal stromal cells; NLCs: nurse-like cells; TAMs: tumor-associated macrophages; MDSCs: monocyte-derived suppressor cells.
Tumor-specific resistance mechanisms
Studies in other B-cell malignancies have shed light on antigen escape as an important mechanism of CAR T-cell resistance[31-34]. In both ELIANA and ZUMA-1 trials, relapse rates without CD19 expression were approximately 25% in B-ALL and 28% in DLBCL[35,36]. CD19 loss is thought to be caused by selective pressure and allows the tumor to evade detection of the circulating CD19-specific CAR T-cells. Multiple studies have demonstrated the expansion of CD19-negative pre-existing clones in samples collected before CAR T-cell infusion that subsequently become the dominant clone after relapse[36-38]. Additionally, genetic mutations, loss of heterozygosity, and alternative splicing of CD19 have been implicated in relapse[32,38,39] [Figure 3]. Lineage switching, wherein a clonally related malignancy of a different cell type emerges, has also been observed. An early example of this was described in a patient with Richter transformation who received CAR T-cells and later developed plasmablastic lymphoma with identical clonal IGH gene rearrangement and TP53 mutation[40]. Trogocytosis, another intriguing mechanism, involves the transfer of the CD19 antigen to the CAR-T cell, thereby reducing antigen density on the tumors and ultimately leading to self-killing or fratricide of the CAR T-cells[41]. Although many of these mechanisms have yet to be described in CLL, understanding potential pathways of antigen escape will inform strategies for overcoming resistance.
Product-specific resistance stemming from exhausted T-cells in CLL
In patients with CLL, their T-cells display an exhausted phenotype from continual antigen stimulation, resulting in poor expansion, persistence, and function[42-44]. Early studies have demonstrated elevated CD8+ expression and altered CD4:CD8 ratio[45], leading to an activated phenotype with a shift toward a terminally differentiated effector-memory subtype[46]. Both CD4+ and CD8+ T-cells in CLL patients express high levels of interleukin-4 (IL-4)[47], upregulating the anti-apoptotic signal, BCL-2[48]. Additionally, CLL patients have increased regulatory T-cells (Tregs) that have decreased responses to tumor antigens. Tregs also lead to increased IL-2 secretion, decreasing T-helper cell type 1 (Th1) differentiation[49,50]. Riches et al. demonstrated high expression of T-cell exhaustion markers CD244, CD160, and programmed death-1
Exhausted T-cells collected for CAR T-cell manufacturing can ultimately lead to inadequate CAR T-cell proliferation, cell killing, and persistence, all crucial factors in resistance. Mueller et al. studied the kinetics of tisa-cel CAR T-cells in 61 patients with B-ALL and 42 patients with CLL[54]. In the CLL cohort, those with a CR (n = 8 patients) had a longer persistence of 352 days vs. 176 days in those with PR (n = 7) and 28.5 days in those who had progressive disease (n = 22)[54]. Nevertheless, expansion was higher in the patients who achieved a PR than CR. This observation suggests that more than just antigen recognition and T-cell expansion is needed to induce a CR, and that T-cell persistence also plays a pivotal role. Hoffmann et al. demonstrated diminished expansion of CD4+ naïve T-cells, and higher PD-1 expression in CAR T-cells from CLL patients compared to normal controls[55]. Patients with CLL that have increased expression of exhaustion markers such as PD-1, T-cell immunoglobulin and mucin domain 3 (TIM-3), and lymphocyte-activation gene 3 (LAG-3)[56] show diminished response to CAR T-cell therapy.
The type of costimulatory domain may also be important. While the CD28 costimulatory domain enhances an early proliferative response, it may also augment early exhaustion, whereas the 4-1BB costimulatory domain may lead to more durable persistence[57,58]. Higher disease volume or tumor burden also predicted poor outcomes in both B-ALL[54] and NHL[59,60]. In CLL, extramedullary disease, such as in the liver, spleen, and lymph nodes, was demonstrated to resolve more slowly than CLL in blood[61]. The underlying reason for this is uncertain, but it may be due to an immunosuppressive TME within bulky tumors that leads to increased interferon signaling, immune dysregulation, and CAR T-cell exhaustion[62].
CLL patients exhibit an immunosuppressive tumor microenvironment
The TME is increasingly becoming a fascinating area of research and is recognized as an important influence in CAR T-cell resistance. CLL patients have an immunosuppressive TME characterized by cancer-associated fibroblasts (CAFs)[63], tumor-associated macrophages (TAMs)[64], blood-derived nurse-like cells (NLC)[65], monocytic myeloid derived suppressor cells (M-MDSCs)[66], and abundance of CLL-derived extracellular vesicles enriched with co-inhibitory signals such as PD-1[67] [Figure 2].
Paggetti et al. demonstrated that proteins and microRNAs from exosomes in CLL patients trigger an inflammatory response within the recipient stromal cells, akin to CAFs[63], prompting heightened proliferation, migration, and the release of inflammatory cytokines, thereby fostering an immunosuppressive TME[63]. Burger et al. further demonstrated that CLL cells are shielded by blood-derived NLCs, which differentiate into large fibroblast-like cells expressing stromal cell markers and stromal cell-derived factor-1 (SDF-1). CLL cells attach to these NLCs and downregulate receptors for SDF-1 (CXCR4) and are protected from apoptosis[65]. Additionally, CLL cells also induce MDSCs converted from healthy monocytes, which secrete indoleamine 2.3-dioxygenase (IDO) and limit T-cell function and proliferation, and promote Tregs[66]. IDO has also been found to inhibit CAR T-cells, but its expression seems to be downregulated with lymphodepleting chemotherapy[68]. Studies have also demonstrated that the TME plays a crucial role in determining CAR T-response. Extracellular vesicles, specifically PD-L1+ vesicles, in patients with CLL induce CAR T-cell exhaustion[67]. Additionally, immunosuppressive myeloid cells not only have a critical role in the suppression of CAR T-cell effector functions, but can also potentiate CRS and neurotoxicity[64]. The development of newer generations of CAR T-cells incorporates strategies to overcome the immunosuppressive TME.
STRATEGIES FOR OVERCOMING RESISTANCE
Despite the notable achievements observed with CAR T-cell therapy, ongoing studies are exploring diverse approaches to enhance their anti-tumor effects. These include combining other agents with CAR T-cells, developing novel CAR T-cell constructs targeting different antigens, using dual-targeting CAR T-cells, and incorporating “armored” CAR T-cells to modify the immunosuppressive TME. Furthermore, allogeneic CAR T- and natural killer (NK)-cells are being pursued to address the challenge of poor T-cell fitness associated with CLL. Figure 4 provides an overview of these strategies. A recent comprehensive list of all ongoing CAR trials in CLL was recently published[69].
Figure 4. Strategies to overcome resistance. (A) New combinations with CAR T-cell infusions include ibrutinib, lenalidomide, checkpoint inhibitors, and dasatinib with promising mechanisms of improving CAR T-cell function, expansion, durability, and safety; (B) Novel targets including new antigens of interest, and dual targeting CAR T-cell methods. Dual targeting methods include bispecific CAR
Combination therapies with CAR T-cells
Ibrutinib
The combination of ibrutinib and CAR T-cell therapy has emerged as an exciting area of research, offering potential improvements in both efficacy and safety[70,71]. Ibrutinib inhibits both BTK, inducing apoptosis in B-cells, and IL-2 inducible T-cell kinase (ITK)[72]. This dual effect leads to markedly increased activated CD4+ and CD8+ T-cells with enhanced persistence, not observed with other selective BTK inhibitors, like acalabrutinib. BTK inhibitors also reduce PD-1 and CTLA-4 expression, leading to dampening of the immunosuppressive signals in CLL[72].
Multiple clinical trials in CLL have demonstrated synergy with the combination of ibrutinib and CAR T-cell therapy. In a study of 19 heavily pretreated patients, 89% of whom had high-risk cytogenetics, ibrutinib was administered 2 weeks prior to leukapheresis and continued for 3 months post-CAR T-cell infusion. They found an ORR of 86%, with 59% achieving undetectable MRD in the marrow by IGH sequencing. They also observed lower CRS severity compared to historical rates and reduced levels of CRS-associated cytokines[7]. However, they did report 1 death from likely cardiac arrhythmia in the ibrutinib cohort. In a study by Gill
Lenalidomide
Lenalidomide, an immunomodulatory agent, is also under investigation as a combinational agent. Studies show that lenalidomide can repair defective synapse formation between T-cells and antigens in CLL patients[52]. A recent preclinical study evaluating lenalidomide combined with CD23-specific CAR-T cells demonstrated that lenalidomide preserved CAR T-cell immune synapses and enhanced migration to leukemic sites, delaying disease progression[77]. Additionally, co-administration of lenalidomide with CD19 and CD20 CAR T-cells in vivo results in diminished tumor expansion, infiltration of cytotoxic T-cells into the tumor, and interferon-γ production[78].
Checkpoint inhibitors
The application of checkpoint inhibitors (CPIs) in CLL has led to disappointing results, with a response only seen in Richter transformation, and only in less than half of cases[79]. Nevertheless, given the increased expression of exhaustion markers on CLL CAR T-cells, there is a strong rationale for adding CPIs as combinational agents. In CLL-like mouse models, PD-L1 blockade helped to restore aberrant and exhausted T-cell phenotypes as well as CD8 T-cell cytotoxicity and immune synapse formation[80]. Preliminary safety studies combining CPIs with the three approved CD19 CAR T-cell agents in LBCLs have all demonstrated favorable safety profiles with signals of improved efficacy[81-83].
Dasatinib
Dasatinib is a tyrosine kinase inhibitor that has gained interest for its unique ability to serve as an “off switch” for CRS developed from CAR T-cell therapy[84]. It interferes with lymphocyte-specific protein tyrosine kinase (LCK) and, through a series of events, diminishes CAR CD3ζ activation and downstream gene expression[84]. In mouse models, it was shown to turn off both CD8+ and CD4+ CAR T-cells immediately and could be reversed upon discontinuation of dasatinib without affecting T-cell viability. Interestingly, dasatinib can also enhance CAR T-cell transduction, expansion, and possible function when added during the manufacturing process[85,86].
Overall, these studies suggest that the synergistic effect of CAR T-cell therapy with other therapies, most notably ibrutinib, may enhance efficacy, reduce immune suppression, and improve safety. Longer term data in larger studies will be needed to fully understand their applications in CLL.
New targets and dual targeting CAR T-cells
In addition to combinational strategies, innovative CAR T-cell constructs are under investigation, including the targeting of novel antigens to combat antigen escape. In addition, dual-targeting CAR T-cells such as bispecific CARs, tandem CARs, and sequential CAR T-cell therapy are of great interest [Figure 4][87]. Triple-targeting CAR T-cells are also being investigated. Careful antigen selection is crucial to minimize off-target binding and adverse effects. Promising antigen targets include CD20, receptor tyrosine kinase-like orphan receptor 1 (ROR1), kappa (κ) or lambda (λ) light chains, Fcµ receptor (FcµR), CD23, B-cell activating factor-receptor (BAFF-R), and B-cell maturation antigen (BCMA).
CD20
CD20, found in over 90% of B-cell lymphomas, remains consistently expressed even after CD20-directed therapy and is therefore a common target for lymphoma treatment[88]. Nevertheless, akin to the CD19 target, it raises concerns regarding B-cell aplasia and agranulocytosis[88]. Early studies with third-generation CD20-targeting CAR T-cells showed promising results in low-grade B-cell lymphomas, with some patients still in remission at 24 months[89]. However, challenges arise in CLL due to modest CD20 expression and often exposure to prior CD20-directed therapies[90]. Nevertheless, CD20-targeted CAR T-cells can exhibit detectability and CAR T-cell activation even in cells with low antigen expression, holding promise for patients with CLL who have shown resistance to CD20 monoclonal antibodies[91].
Dual-targeting CAR T-cells, which simultaneously target CD19 and CD20, are also being explored. Innovations in CAR T-cell development have given rise to bispecific[92] and tandem[93] CAR designs, demonstrating their ability to induce cell killing against either CD19- or CD20-positive cells, helping prevent antigen escape as the CAR remains primed for activation by the other antigen if one is downregulated or lost. In a study of patients with r/r NHL, including three with CLL, dual CD19-CD20 CAR T-cells demonstrated an 88% ORR and a 64% CR rate. Among the CLL patients, two achieved CR, while one achieved PR. The duration of response for those in CR has yet to be determined[94]. However, further research is needed to thoroughly delineate the efficacy and safety profiles of these innovative CAR products in CLL. Several dual CAR T-cell trials are currently under investigation, with a notable trial on CD19-CD20-CD22 trispecific CAR T-cells (NCT05418088).
ROR1
ROR1 is another interesting antigen found predominantly in CLL and MCL cells[95]. While ROR1 is highly expressed in undifferentiated embryonic stem cells, it is not found in other adult tissue types except for a low expression in adipose tissue[96]. Additionally, higher levels of ROR1 on CLL cells are associated with more aggressive disease, and its expression remains stable across progression and treatment[97]. Therefore, it presents an attractive prospect for achieving highly targeted effects, thereby mitigating adverse events and reducing immunosuppression. Hudecek et al. reported the first ROR1-targeted CAR-T cell with cytotoxicity in CLL and MCL cells[96]. More recently, Prussak et al. reported on a ROR1-specific CAR T-cell that effectively and selectively killed ROR1-containing lymphoid cancer cells in mice[98].
κ/λ
CLL cells, like plasma cells in multiple myeloma, frequently exhibit clonal expression of either the κ or λ light chains, offering a potential targeted intervention that leaves half of normal B-cells. Both κ and λ
BAFF-R and BCMA
Two other potential targets in CLL cells are BAFF-R and BCMA, both activated by the protein BAFF, which is expressed by immunosuppressive cells in the TME in CLL and promotes disease progression and chemotherapy resistance[101]. BAFF-R signaling is essential for B-cell survival and is expressed on malignant B-cells. BAFF-R CAR T-cells have demonstrated anti-tumor activity in NHL including CLL in preclinical models[102,103] and can overcome CD19 antigen loss in B-cell malignancies[104]. There are currently ongoing Phase 1 studies of BAFF-R targeting CAR T-cells in r/r B-ALL (NCT04690595) and B-NHL (NCT05370430). A dual BAFF-R/CD19 CAR T-cell is under development to further curb antigen evasion[105]. Another promising target is BCMA, which is also elevated in CLL patients and correlates with more aggressive disease[106]. BCMA is typically prevalent in advanced-stage B-cells and plasma cells and has already been successfully targeted in multiple myeloma, leading to the FDA approval of two BCMA-CAR
Fcµ receptor and CD23
Another studied target is FcµR, also recognized as TOSO or FAIM3[109]. This receptor is consistently highly expressed in CLL cells and minimally expressed in healthy B-cells and other tissues. Mouse studies have shown promising results with FcµR CAR T-cells effectively eliminating autologous CLL cells, while preserving healthy B-cells[109]. CD23 CAR T-cells have also shown promising results in preclinical models when combined with lenalidomide[77]. However, comprehensive data are needed to fully understand the implications of these and other targets under investigation in CLL.
Altering the immunosuppressive TME, and the rise of the next generation of autologous CAR T-cells
Modifying the immunosuppressive TME in CLL could greatly enhance the CAR T-cells function. Augmenting pro-inflammatory cytokines is one approach. Early attempts to enhance adoptive immunotherapy were based on ex vivo culture and exogenous administration of cytokines IL-2 and IL-15, which augmented T-cell activity; however, these strategies were also associated with worse systemic toxicity[110]. In order to limit toxicity but still get the immunomodulatory effects of IL-2, a new strategy combines SYNCAR-001, a CD19-targeting CAR T-cell that expresses an engineered IL-2 receptor, and STK-009, a pegylated IL-2 cytokine that selectively binds only CAR T-cells[111]. This combination is being studied in a Phase 1 trial for CD19+ hematological malignancies, including CLL (NCT05665062). Additionally, exciting 4th generation armored CAR-T cells show promise in preclinical studies[20], necessitating further clinical assessment in CLL.
Allogenic CARs provide an exciting “off the shelf” alternative
Preserving high-quality T-cells suitable for CAR T-cell manufacturing in CLL patients is challenging due to T-cell exhaustion from prolonged antigen exposure and usually years of prior treatments. Furthermore, CAR T-cell manufacturing may take weeks, posing obstacles for rapidly progressive disease. Developing “off the shelf” allogenic CAR T-cells from healthy donor cells bypasses these challenges and dissolves the risk of leukemic cell contamination. Initially, there were concerns about graft-versus-host disease (GVHD) using allogenic CAR T-cells; however, gene-editing technologies such as zinc-finger nuclease, transcription activator-like effector nuclease (TALEN), or clustered regularly interspaced short palindromic repeats (CRISPRs) offer solutions by disrupting the TCR genes and reducing GVHD risk[112].
Brudno et al. documented an early instance of allogeneic CAR T-cells in humans. In their study, 20 patients with B-cell malignancies, including 5 CLL patients who relapsed after an allogenic transplant, received CD19 allogeneic CAR T-cells. Only one CLL patient achieved a sustained CR that persisted beyond 30 months of follow-up. The safety profile was similar to autologous CAR T-cell studies, with no instances of GVHD[113]. The CD19 allogeneic CAR T-cell FT819, which is an induced pluripotent stem cell (iPSC) line that can serve as a renewable source for mass production, in combination with IL-2[114], is currently under study in a phase 1 trial throughout the US (NCT04629729). In another promising Phase 1 trial, called ARDENT, a new “hypoimmune” CD19-directed allogeneic CAR T-cell therapy was designed to reduce immune recognition by depletion of HLA I/II to prevent adaptive immune rejection and overexpression of CD47 to thwart innate immune rejection[115]. This trial is currently recruiting patients with r/r NHL and CLL within Australia and the US (NCT05878184).
CAR NK cells have similar components to CAR T-cells, namely an antigen (scFvs)-recognition domain, an activation domain (usually CD3ζ), and costimulatory domains (CD18, 4-1BB, or 2B4)[116]. NK cells offer advantages such as HLA-independent cytotoxicity and no rejection reactions, making them suitable for allogeneic products sourced from healthy donor blood or cord blood[117,118]. Challenges in using NK CARs include difficulties with transfection, expansion, and durability, which have been addressed through various methods[119]. For instance, Liu et al. successfully engineered cord blood (CB) CAR NK-cells with expression of IL-15 and an inducible caspase 9 safety switch[117]. In a phase 1 study, this CB-CAR NK cell construct showed promising results, yielding a CR in 3 of the 5 patients in the study with CLL[120]. The expansion phase of this study, called the ANCHOR trial, is ongoing (NCT03774654). These readily available CAR options have the potential to emerge as more convenient alternatives to autologous CAR T-cells. Numerous other trials are underway, investigating innovative new CAR T and NK-cell products for the treatment of NHL, including CLL.
CONCLUSIONS
CAR T-cell therapy presents a compelling approach for addressing the treatment challenges in high-risk and multiply refractory patients with CLL. However, challenges persist in CLL due to underlying T-cell defects and lower response rates. Nevertheless, recent trials have shown promising results, leading to the first FDA approval of liso-cel for CLL patients who have failed BTK and BCL2 inhibitors. This “one and done” therapeutic approach holds the potential to offer a long-term remission for some patients, yielding prolonged responses exceeding a decade, as evidenced in some cases. While resistance mechanisms, such as antigen escape and T-cell exhaustion, pose hurdles, ongoing research aims to overcome these barriers through combination therapies, novel targets, and strategies to alter the immunosuppressive TME. Ongoing investigations into combinational agents and the development of the next generation of CAR constructs including allogenic and armored versions, offer a promising trajectory, heralding a new therapeutic era in the realm of CLL treatment.
DECLARATIONS
Author’s contributions
Wrote the manuscript and designed the figures: Borogovac A
Provided valuable insight and edited the manuscript/figures: Siddiqi T
Availability of data and materials
Not applicable.
Financial support and sponsorship
None.
Conflicts of interest
Siddiqi T received research funding from Bristol Myers Squibb; served on advisory boards for AbbVie, BeiGene, Bristol Myers Squibb, AstraZeneca, and Gilead; participated in the data safety monitoring board for BeiGene; contributed to speakers’ bureaus for Bristol Myers Squibb, AstraZeneca, and BeiGene; and served on the steering committee for Bristol Myers Squibb. The other author declared that there are no conflicts of interest.
Ethical approval and consent to participate
Not applicable.
Consent for publication
Not applicable.
Copyright
© The Author(s) 2024.
REFERENCES
1. Hartmann J, Schüßler-Lenz M, Bondanza A, Buchholz CJ. Clinical development of CAR T cells - challenges and opportunities in translating innovative treatment concepts. EMBO Mol Med 2017;9:1183-97.
3. Neelapu SS, Jacobson CA, Ghobadi A, et al. Five-year follow-up of ZUMA-1 supports the curative potential of axicabtagene ciloleucel in refractory large B-cell lymphoma. Blood 2023;141:2307-15.
4. Chihara D, Liao L, Tkacz J, et al. Real-world experience of CAR T-cell therapy in older patients with relapsed/refractory diffuse large B-cell lymphoma. Blood 2023;142:1047-55.
5. Shanafelt TD, Wang XV, Hanson CA, et al. Long-term outcomes for ibrutinib-rituximab and chemoimmunotherapy in CLL: updated results of the E1912 trial. Blood 2022;140:112-20.
6. Fischer K, Al-Sawaf O, Fink AM, et al. Venetoclax and obinutuzumab in chronic lymphocytic leukemia. Blood. 2017;129(19):2702-2705. Blood 2017;130:232.
7. Gauthier J, Hirayama AV, Purushe J, et al. Feasibility and efficacy of CD19-targeted CAR T cells with concurrent ibrutinib for CLL after ibrutinib failure. Blood 2020;135:1650-60.
8. Frey NV, Gill S, Hexner EO, et al. Long-term outcomes from a randomized dose optimization study of chimeric antigen receptor modified T cells in relapsed chronic lymphocytic leukemia. J Clin Oncol 2020;38:2862-71.
9. Siddiqi T, Maloney DG, Kenderian SS, et al. Lisocabtagene maraleucel in chronic lymphocytic leukaemia and small lymphocytic lymphoma (TRANSCEND CLL 004): a multicentre, open-label, single-arm, phase 1-2 study. Lancet 2023;402:641-54.
10. Döhner H, Stilgenbauer S, Benner A, et al. Genomic aberrations and survival in chronic lymphocytic leukemia. N Engl J Med 2000;343:1910-6.
11. Stilgenbauer S, Schnaiter A, Paschka P, et al. Gene mutations and treatment outcome in chronic lymphocytic leukemia: results from the CLL8 trial. Blood 2014;123:3247-54.
12. Brocker T, Peter A, Traunecker A, Karjalainen K. New simplified molecular design for functional T cell receptor. Eur J Immunol 1993;23:1435-9.
13. Brocker T, Karjalainen K. Signals through T cell receptor-zeta chain alone are insufficient to prime resting T lymphocytes. J Exp Med 1995;181:1653-9.
14. Maher J, Brentjens RJ, Gunset G, Rivière I, Sadelain M. Human T-lymphocyte cytotoxicity and proliferation directed by a single chimeric TCRzeta /CD28 receptor. Nat Biotechnol 2002;20:70-5.
15. Hoyos V, Savoldo B, Quintarelli C, et al. Engineering CD19-specific T lymphocytes with interleukin-15 and a suicide gene to enhance their anti-lymphoma/leukemia effects and safety. Leukemia 2010;24:1160-70.
16. Chmielewski M, Abken H. CAR T Cells Releasing IL-18 convert to T-Bethigh FoxO1low effectors that exhibit augmented activity against advanced solid tumors. Cell Rep 2017;21:3205-19.
17. Bell M, Gottschalk S. Engineered cytokine signaling to improve CAR T cell effector function. Front Immunol 2021;12:684642.
18. Lanitis E, Rota G, Kosti P, et al. Optimized gene engineering of murine CAR-T cells reveals the beneficial effects of IL-15 coexpression. J Exp Med 2021;218:e20192203.
19. Pegram HJ, Purdon TJ, van Leeuwen DG, et al. IL-12-secreting CD19-targeted cord blood-derived T cells for the immunotherapy of B-cell acute lymphoblastic leukemia. Leukemia 2015;29:415-22.
20. Chmielewski M, Abken H. TRUCKs: the fourth generation of CARs. Expert Opin Biol Ther 2015;15:1145-54.
21. Wang M, Munoz J, Goy A, et al. KTE-X19 CAR T-cell therapy in relapsed or refractory mantle-cell lymphoma. N Engl J Med 2020;382:1331-42.
22. Porter DL, Levine BL, Kalos M, Bagg A, June CH. Chimeric antigen receptor-modified T cells in chronic lymphoid leukemia. N Engl J Med 2011;365:725-33.
23. Melenhorst JJ, Chen GM, Wang M, et al. Decade-long leukaemia remissions with persistence of CD4+ CAR T cells. Nature 2022;602:503-9.
24. Turtle CJ, Hay KA, Hanafi LA, et al. Durable molecular remissions in chronic lymphocytic leukemia treated with CD19-specific chimeric antigen receptor-modified T cells after failure of ibrutinib. J Clin Oncol 2017;35:3010-20.
25. Cappell KM, Sherry RM, Yang JC, et al. Long-term follow-up of anti-CD19 chimeric antigen receptor T-cell therapy. J Clin Oncol 2020;38:3805-15.
26. Siddiqi T, Maloney DG, Kenderian SS, et al. Lisocabtagene maraleucel (liso-cel) in R/R CLL/SLL: 24-month median follow-up of TRANSCEND CLL 004. Blood 2023;142:330.
27. Kharfan-Dabaja MA, Yassine F, Gadd ME, Qin H. Driving out chronic lymphocytic leukemia with CAR T cells. Transplant Cell Ther 2022;28:5-17.
28. Vitale C, Griggio V, Perutelli F, Coscia M. CAR-modified cellular therapies in chronic lymphocytic leukemia: is the uphill road getting less steep? Hemasphere 2023;7:e988.
29. Hampel PJ, Rabe KG, Wang Y, et al. Incidence of richter transformation in patients with chronic lymphocytic leukemia (CLL)/small lymphocytic lymphoma (SLL): a cohort study evaluating different therapeutic eras. Blood 2023;142:3271.
30. Kittai AS, Bond DA, Huang Y, et al. Anti-CD19 chimeric antigen receptor T-cell therapy for Richter’s transformation: an international multicenter retrospective study. Blood 2023;142:108.
31. Shalabi H, Kraft IL, Wang HW, et al. Sequential loss of tumor surface antigens following chimeric antigen receptor T-cell therapies in diffuse large B-cell lymphoma. Haematologica 2018;103:e215-8.
32. Sotillo E, Barrett DM, Black KL, et al. Convergence of acquired mutations and alternative splicing of CD19 enables resistance to CART-19 immunotherapy. Cancer Discov 2015;5:1282-95.
33. Gardner R, Wu D, Cherian S, et al. Acquisition of a CD19-negative myeloid phenotype allows immune escape of MLL-rearranged B-ALL from CD19 CAR-T-cell therapy. Blood 2016;127:2406-10.
34. Wang J, Hu Y, Huang H. Acute lymphoblastic leukemia relapse after CD19-targeted chimeric antigen receptor T cell therapy. J Leukoc Biol 2017;102:1347-56.
35. Maude SL, Laetsch TW, Buechner J, et al. Tisagenlecleucel in children and young adults with B-cell lymphoblastic leukemia. N Engl J Med 2018;378:439-48.
36. Plaks V, Rossi JM, Chou J, et al. CD19 target evasion as a mechanism of relapse in large B-cell lymphoma treated with axicabtagene ciloleucel. Blood 2021;138:1081-5.
37. Fischer J, Paret C, El Malki K, et al. CD19 isoforms enabling resistance to CART-19 immunotherapy are expressed in B-ALL patients at initial diagnosis. J Immunother 2017;40:187-95.
38. Rabilloud T, Potier D, Pankaew S, Nozais M, Loosveld M, Payet-Bornet D. Single-cell profiling identifies pre-existing CD19-negative subclones in a B-ALL patient with CD19-negative relapse after CAR-T therapy. Nat Commun 2021;12:865.
39. Zhang Z, Chen X, Tian Y, et al. Point mutation in CD19 facilitates immune escape of B cell lymphoma from CAR-T cell therapy. J Immunother Cancer 2020;8:e001150.
40. Evans AG, Rothberg PG, Burack WR, et al. Evolution to plasmablastic lymphoma evades CD19-directed chimeric antigen receptor T cells. Br J Haematol 2015;171:205-9.
41. Hamieh M, Dobrin A, Cabriolu A, et al. CAR T cell trogocytosis and cooperative killing regulate tumour antigen escape. Nature 2019;568:112-6.
42. Riches JC, Ramsay AG, Gribben JG. Immune reconstitution in chronic lymphocytic leukemia. Curr Hematol Malig Rep 2012;7:13-20.
43. Riches JC, Ramsay AG, Gribben JG. Immune dysfunction in chronic lymphocytic leukemia: the role for immunotherapy. Curr Pharm Des 2012;18:3389-98.
44. Blank CU, Haining WN, Held W, et al. Defining ‘T cell exhaustion’. Nat Rev Immunol 2019;19:665-74.
45. Herrmann F, Lochner A, Philippen H, Jauer B, Rühl H. Imbalance of T cell subpopulations in patients with chronic lymphocytic leukaemia of the B cell type. Clin Exp Immunol 1982;49:157-62.
46. Van den Hove LE, Vandenberghe P, Van Gool SW, et al. Peripheral blood lymphocyte subset shifts in patients with untreated hematological tumors: evidence for systemic activation of the T cell compartment. Leuk Res 1998;22:175-84.
47. Mu X, Kay NE, Gosland MP, Jennings CD. Analysis of blood T-cell cytokine expression in B-chronic lymphocytic leukaemia: evidence for increased levels of cytoplasmic IL-4 in resting and activated CD8 T cells. Br J Haematol 1997;96:733-5.
48. Dancescu M, Rubio-Trujillo M, Biron G, Bron D, Delespesse G, Sarfati M. Interleukin 4 protects chronic lymphocytic leukemic B cells from death by apoptosis and upregulates Bcl-2 expression. J Exp Med 1992;176:1319-26.
49. D’Arena G, Laurenti L, Minervini MM, et al. Regulatory T-cell number is increased in chronic lymphocytic leukemia patients and correlates with progressive disease. Leuk Res 2011;35:363-8.
50. Lindqvist CA, Christiansson LH, Simonsson B, Enblad G, Olsson-Strömberg U, Loskog AS. T regulatory cells control T-cell proliferation partly by the release of soluble CD25 in patients with B-cell malignancies. Immunology 2010;131:371-6.
51. Riches JC, Davies JK, McClanahan F, et al. T cells from CLL patients exhibit features of T-cell exhaustion but retain capacity for cytokine production. Blood 2013;121:1612-21.
52. Ramsay AG, Clear AJ, Fatah R, Gribben JG. Multiple inhibitory ligands induce impaired T-cell immunologic synapse function in chronic lymphocytic leukemia that can be blocked with lenalidomide: establishing a reversible immune evasion mechanism in human cancer. Blood 2012;120:1412-21.
53. Ramsay AG, Johnson AJ, Lee AM, et al. Chronic lymphocytic leukemia T cells show impaired immunological synapse formation that can be reversed with an immunomodulating drug. J Clin Invest 2008;118:2427-37.
54. Mueller KT, Maude SL, Porter DL, et al. Cellular kinetics of CTL019 in relapsed/refractory B-cell acute lymphoblastic leukemia and chronic lymphocytic leukemia. Blood 2017;130:2317-25.
55. Hoffmann JM, Schubert ML, Wang L, et al. Differences in expansion potential of naive chimeric antigen receptor T cells from healthy donors and untreated chronic lymphocytic leukemia patients. Front Immunol 2017;8:1956.
56. Fraietta JA, Lacey SF, Orlando EJ, et al. Determinants of response and resistance to CD19 chimeric antigen receptor (CAR) T cell therapy of chronic lymphocytic leukemia. Nat Med 2018;24:563-71.
57. Ying Z, He T, Wang X, et al. Parallel comparison of 4-1BB or CD28 co-stimulated CD19-targeted CAR-T cells for B cell non-Hodgkin’s lymphoma. Mol Ther Oncolytics 2019;15:60-8.
58. Long AH, Haso WM, Shern JF, et al. 4-1BB costimulation ameliorates T cell exhaustion induced by tonic signaling of chimeric antigen receptors. Nat Med 2015;21:581-90.
59. Vercellino L, Di Blasi R, Kanoun S, et al. Predictive factors of early progression after CAR T-cell therapy in relapsed/refractory diffuse large B-cell lymphoma. Blood Adv 2020;4:5607-15.
60. Sesques P, Tordo J, Ferrant E, et al. Prognostic impact of 18F-FDG PET/CT in patients with aggressive B-cell lymphoma treated with anti-CD19 chimeric antigen receptor T cells. Clin Nucl Med 2021;46:627-34.
61. Porter DL, Hwang WT, Frey NV, et al. Chimeric antigen receptor T cells persist and induce sustained remissions in relapsed refractory chronic lymphocytic leukemia. Sci Transl Med 2015;7:303ra139.
62. Jain MD, Zhao H, Wang X, et al. Tumor interferon signaling and suppressive myeloid cells are associated with CAR T-cell failure in large B-cell lymphoma. Blood 2021;137:2621-33.
63. Paggetti J, Haderk F, Seiffert M, et al. Exosomes released by chronic lymphocytic leukemia cells induce the transition of stromal cells into cancer-associated fibroblasts. Blood 2015;126:1106-17.
64. Sterner RM, Kenderian SS. Myeloid cell and cytokine interactions with chimeric antigen receptor-T-cell therapy: implication for future therapies. Curr Opin Hematol 2020;27:41-8.
65. Burger JA, Tsukada N, Burger M, Zvaifler NJ, Dell’Aquila M, Kipps TJ. Blood-derived nurse-like cells protect chronic lymphocytic leukemia B cells from spontaneous apoptosis through stromal cell-derived factor-1. Blood 2000;96:2655-63.
66. Jitschin R, Braun M, Büttner M, et al. CLL-cells induce IDOhi CD14+HLA-DRlo myeloid-derived suppressor cells that inhibit T-cell responses and promote TRegs. Blood 2014;124:750-60.
67. Cox MJ, Lucien F, Sakemura R, et al. Leukemic extracellular vesicles induce chimeric antigen receptor T cell dysfunction in chronic lymphocytic leukemia. Mol Ther 2021;29:1529-40.
68. Ninomiya S, Narala N, Huye L, et al. Tumor indoleamine 2,3-dioxygenase (IDO) inhibits CD19-CAR T cells and is downregulated by lymphodepleting drugs. Blood 2015;125:3905-16.
69. Borogovac A, Siddiqi T. Transforming CLL management with immunotherapy: investigating the potential of CAR T-cells and bispecific antibodies. Semin Hematol 2024:S0037-1963(24)00003-9.
70. Fraietta JA, Beckwith KA, Patel PR, et al. Ibrutinib enhances chimeric antigen receptor T-cell engraftment and efficacy in leukemia. Blood 2016;127:1117-27.
71. Ruella M, Kenderian SS, Shestova O, et al. Kinase inhibitor ibrutinib to prevent cytokine-release syndrome after anti-CD19 chimeric antigen receptor T cells for B-cell neoplasms. Leukemia 2017;31:246-8.
72. Long M, Beckwith K, Do P, et al. Ibrutinib treatment improves T cell number and function in CLL patients. J Clin Invest 2017;127:3052-64.
73. Gill S, Vides V, Frey NV, et al. Anti-CD19 CAR T cells in combination with ibrutinib for the treatment of chronic lymphocytic leukemia. Blood Adv. 2022;6(21):5774-5785. Blood Adv 2023;7:6567.
74. Siddiqi T, Soumerai JD, Dorritie KA, et al. Phase 1 TRANSCEND CLL 004 study of lisocabtagene maraleucel in patients with relapsed/refractory CLL or SLL. Blood 2022;139:1794-806.
75. Wierda WG, Dorritie KA, Munoz J, et al. Transcend CLL 004: phase 1 cohort of lisocabtagene maraleucel (LISO-CEL) combined with ibrutinib (IBR) for patients (PTS) with r/r CLL/SLL. Hematol Oncol 2021;39:hon.86_2879.
76. Thorpe J, Rytlewski J, Gillenwater H, et al. 449 Concurrent ibrutinib enhances T cell function in patients with chronic lymphocytic leukemia (CLL) treated with lisocabtagene maraleucel (liso-cel), a chimeric antigen receptor (CAR) T cell therapy. J Immunother Cancer 2021;9:A477.
77. Tettamanti S, Rotiroti MC, Giordano Attianese GMP, et al. Lenalidomide enhances CD23.CAR T cell therapy in chronic lymphocytic leukemia. Leuk Lymphoma 2022;63:1566-79.
78. Otáhal P, Průková D, Král V, et al. Lenalidomide enhances antitumor functions of chimeric antigen receptor modified T cells. Oncoimmunology 2016;5:e1115940.
79. Ding W, LaPlant BR, Call TG, et al. Pembrolizumab in patients with CLL and Richter transformation or with relapsed CLL. Blood 2017;129:3419-27.
80. McClanahan F, Hanna B, Miller S, et al. PD-L1 checkpoint blockade prevents immune dysfunction and leukemia development in a mouse model of chronic lymphocytic leukemia. Blood 2015;126:203-11.
81. Siddiqi T, Abramson J, Lee H, et al. Safety of lisocabtagene maraleucel given with durvalumab in patients with relapsed/refractory aggressive B-cell non hodgkin lymphoma: first results from the platform study. Hematol Oncol 2019;37:171-2.
82. Jacobson CA, Locke FL, Miklos DB, et al. End of phase 1 results from zuma-6: axicabtagene ciloleucel (Axi-Cel) in combination with atezolizumab for the treatment of patients with refractory diffuse large B cell lymphoma. Blood 2018;132:4192.
83. Jaeger U, Worel N, McGuirk JP, et al. Safety and efficacy of tisagenlecleucel plus pembrolizumab in patients with r/r DLBCL: phase 1b PORTIA study results. Blood Adv 2023;7:2283-6.
84. Mestermann K, Giavridis T, Weber J, et al. The tyrosine kinase inhibitor dasatinib acts as a pharmacologic on/off switch for CAR T cells. Sci Transl Med 2019;11:eaau5907.
85. Braun AH, Frank AM, Ho N, Buchholz CJ. Dasatinib is a potent enhancer for CAR T cell generation by CD3-targeted lentiviral vectors. Mol Ther Methods Clin Dev 2023;28:90-8.
86. Zhang H, Hu Y, Shao M, et al. Dasatinib enhances anti-leukemia efficacy of chimeric antigen receptor T cells by inhibiting cell differentiation and exhaustion. J Hematol Oncol 2021;14:113.
87. Yang X, Wei J, Zhou J. Overcoming resistance to anti-CD19 CAR T-cell therapy in B-cell malignancies. Hematol Oncol 2022;40:821-34.
88. Tan Su Yin E, Hu YX, Huang H. The breakthrough and the future: CD20 chimeric antigen receptor T-cell therapy for hematologic malignancies. ImmunoMedicine 2022;2:e1039.
89. Till BG, Jensen MC, Wang J, et al. CD20-specific adoptive immunotherapy for lymphoma using a chimeric antigen receptor with both CD28 and 4-1BB domains: pilot clinical trial results. Blood 2012;119:3940-50.
90. Almasri NM, Duque RE, Iturraspe J, Everett E, Braylan RC. Reduced expression of CD20 antigen as a characteristic marker for chronic lymphocytic leukemia. Am J Hematol 1992;40:259-63.
91. Watanabe K, Terakura S, Martens AC, et al. Target antigen density governs the efficacy of anti-CD20-CD28-CD3ζ chimeric antigen receptor-modified effector CD8+ T cells. J Immunol 2015;194:911-20.
92. Zah E, Lin MY, Silva-Benedict A, Jensen MC, Chen YY. T cells expressing CD19/CD20 bispecific chimeric antigen receptors prevent antigen escape by malignant B cells. Cancer Immunol Res 2016;4:498-508.
93. Schneider D, Xiong Y, Wu D, et al. A tandem CD19/CD20 CAR lentiviral vector drives on-target and off-target antigen modulation in leukemia cell lines. J Immunother Cancer 2017;5:42.
94. Shah NN, Johnson BD, Schneider D, et al. Bispecific anti-CD20, anti-CD19 CAR T cells for relapsed B cell malignancies: a phase 1 dose escalation and expansion trial. Nat Med 2020;26:1569-75.
95. Lee BK, Wan Y, Chin ZL, et al. Developing ROR1 targeting CAR-T cells against solid tumors in preclinical studies. Cancers 2022;14:3618.
96. Hudecek M, Schmitt TM, Baskar S, et al. The B-cell tumor-associated antigen ROR1 can be targeted with T cells modified to express a ROR1-specific chimeric antigen receptor. Blood 2010;116:4532-41.
97. Cui B, Ghia EM, Chen L, et al. High-level ROR1 associates with accelerated disease progression in chronic lymphocytic leukemia. Blood 2016;128:2931-40.
98. Prussak CE, Oh C, Velez Lujan J, et al; University of California, San Diego Moores Cancer Center. Preclinical evaluation of anti-ROR1 CAR T cells employing a ROR1 binding SCFV derived from the clinical stage mab cirmtuzumab. J Clin Oncol 2020;38:41.
99. Ranganathan R, Shou P, Ahn S, et al. CAR T cells targeting human immunoglobulin light chains eradicate mature B-cell malignancies while sparing a subset of normal B cells. Clin Cancer Res 2021;27:5951-60.
100. Ramos CA, Savoldo B, Torrano V, et al. Clinical responses with T lymphocytes targeting malignancy-associated κ light chains. J Clin Invest 2016;126:2588-96.
101. Qin H, Wei G, Sakamaki I, et al. Novel BAFF-receptor antibody to natively folded recombinant protein eliminates drug-resistant human B-cell malignancies in vivo. Clin Cancer Res 2018;24:1114-23.
102. Luo Y, Lui K, To TT, et al. Abstract 2855: antitumor activity of BAFF-R targeting CAR T-cells on chronic lymphocytic leukemia. Cancer Res 2022;82:2855.
103. Dong Z, Cheng WA, Smith DL, et al. Antitumor efficacy of BAFF-R targeting CAR T cells manufactured under clinic-ready conditions. Cancer Immunol Immunother 2020;69:2139-45.
104. Qin H, Dong Z, Wang X, et al. CAR T cells targeting BAFF-R can overcome CD19 antigen loss in B cell malignancies. Sci Transl Med 2019;11:eaaw9414.
105. Wang X, Dong Z, Awuah D, et al. CD19/BAFF-R dual-targeted CAR T cells for the treatment of mixed antigen-negative variants of acute lymphoblastic leukemia. Leukemia 2022;36:1015-24.
106. Udd KA, Bujarski S, Wirtschafter E, et al. Plasma B-cell maturation antigen levels are elevated and correlate with disease activity in patients with chronic lymphocytic leukemia. Target Oncol 2019;14:551-61.
107. Munshi NC, Anderson LD Jr, Shah N, et al. Idecabtagene vicleucel in relapsed and refractory multiple myeloma. N Engl J Med 2021;384:705-16.
108. Berdeja JG, Madduri D, Usmani SZ, et al. Ciltacabtagene autoleucel, a B-cell maturation antigen-directed chimeric antigen receptor T-cell therapy in patients with relapsed or refractory multiple myeloma (CARTITUDE-1): a phase 1b/2 open-label study. Lancet 2021;398:314-24.
109. Faitschuk E, Hombach AA, Frenzel LP, Wendtner CM, Abken H. Chimeric antigen receptor T cells targeting Fc μ receptor selectively eliminate CLL cells while sparing healthy B cells. Blood 2016;128:1711-22.
110. Alwan LM, Grossmann K, Sageser D, Van Atta J, Agarwal N, Gilreath JA. Comparison of acute toxicity and mortality after two different dosing regimens of high-dose interleukin-2 for patients with metastatic melanoma. Target Oncol 2014;9:63-71.
111. Palomba ML, Mei MG, Caimi PF, et al. Abstract CT125: a phase 1 study to evaluate the safety and tolerability of a combination autologous CD19 CAR T cell therapy (SYNCAR-001) and orthogonal IL-2 (STK-009) in subjects with relapsed or refractory CD19 expressing hematologic malignancies (NCT05665062). Cancer Res 2023;83:CT125.
112. Morgan MA, Büning H, Sauer M, Schambach A. Use of cell and genome modification technologies to generate improved “off-the-shelf” CAR T and CAR NK cells. Front Immunol 2020;11:1965.
113. Brudno JN, Somerville RP, Shi V, et al. Allogeneic T cells that express an anti-CD19 chimeric antigen receptor induce remissions of B-cell malignancies that progress after allogeneic hematopoietic stem-cell transplantation without causing graft-versus-host disease. J Clin Oncol 2016;34:1112-21.
114. Mehta A, Farooq U, Chen A, et al. Interim phase I clinical data of FT819-101, a study of the first-ever, off-the-shelf, iPSC-derived TCR-less CD19 CAR T-cell therapy for patients with relapsed/refractory B-cell malignancies. Blood 2022;140:4577-8.
115. Neelapu SS, Budde LE, Mcguirk JP, et al. Phase 1 study of SC291, a hypoimmune, allogeneic CD19-directed CAR T cell therapy for relapsed/refractory B-cell malignancies (ARDENT) - initial clinical data. Blood 2023;142:6852.
116. Hermanson DL, Kaufman DS. Utilizing chimeric antigen receptors to direct natural killer cell activity. Front Immunol 2015;6:195.
117. Liu E, Tong Y, Dotti G, et al. Cord blood NK cells engineered to express IL-15 and a CD19-targeted CAR show long-term persistence and potent antitumor activity. Leukemia 2018;32:520-31.
118. Herrera L, Juan M, Eguizabal C. Purification, culture, and CD19-CAR lentiviral transduction of adult and umbilical cord blood NK cells. Curr Protoc Immunol 2020;131:e108.
119. Wang ZH, Li W, Dong H, Han F. Current state of NK cell-mediated immunotherapy in chronic lymphocytic leukemia. Front Oncol 2022;12:1077436.
Cite This Article
Export citation file: BibTeX | EndNote | RIS
OAE Style
Borogovac A, Siddiqi T. Advancing CAR T-cell therapy for chronic lymphocytic leukemia: exploring resistance mechanisms and the innovative strategies to overcome them. Cancer Drug Resist 2024;7:18. http://dx.doi.org/10.20517/cdr.2023.100
AMA Style
Borogovac A, Siddiqi T. Advancing CAR T-cell therapy for chronic lymphocytic leukemia: exploring resistance mechanisms and the innovative strategies to overcome them. Cancer Drug Resistance. 2024; 7: 18. http://dx.doi.org/10.20517/cdr.2023.100
Chicago/Turabian Style
Azra Borogovac, Tanya Siddiqi. 2024. "Advancing CAR T-cell therapy for chronic lymphocytic leukemia: exploring resistance mechanisms and the innovative strategies to overcome them" Cancer Drug Resistance. 7: 18. http://dx.doi.org/10.20517/cdr.2023.100
ACS Style
Borogovac, A.; Siddiqi T. Advancing CAR T-cell therapy for chronic lymphocytic leukemia: exploring resistance mechanisms and the innovative strategies to overcome them. Cancer Drug Resist. 2024, 7, 18. http://dx.doi.org/10.20517/cdr.2023.100
About This Article
Copyright
Data & Comments
Data
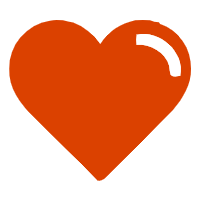
Comments
Comments must be written in English. Spam, offensive content, impersonation, and private information will not be permitted. If any comment is reported and identified as inappropriate content by OAE staff, the comment will be removed without notice. If you have any queries or need any help, please contact us at support@oaepublish.com.