Global left frontal cortex connectivity attenuates the adverse effect of brain atrophy on global cognition in non-dementia participants
Abstract
Aim: To investigate whether higher global left frontal cortex (gLFC) connectivity, a potential neural substrate of cognitive reserve (CR), mitigates the impact of brain atrophy on cognition in non-dementia participants.
Methods: A total of 43 clinically diagnostic normal controls (NC), 63 subjective cognitive decline (SCD) and 30 mild cognitive impairment (MCI) were recruited, who underwent resting-state fMRI and structural MRI scans. The gLFC connectivity was defined as the average positive functional connectivity between the left frontal cortex and each voxel in the gray matter. Hippocampus, parahippocampal gyrus, and amygdala volume volumes/intracranial volume ratio (HpVR, PhgVR, and AmyVR) values were computed as measures of brain atrophy. In general linear analysis, interaction term gLFC connectivity × brain atrophy on global cognition performance were tested, which were repeated in women and men separately. The Trial registration number is NCT03370744.
Results: Significant interaction effects of HpVR * gLFC connectivity (P = 0.031) and PhgVR * gLFC connectivity
Conclusion: The gLFC connectivity is associated with greater resilience against the adverse effect of specified brain atrophy on global cognition, which is more effective in men.
Keywords
INTRODUCTION
Alzheimer’s disease (AD) is characterized by amyloid deposition, neural loss, and progressive cognitive decline, which is the main cause of dementia and has become one of the most costly, lethal, and burdening diseases[1,2]. Given the irreversible nature of the disease, nowadays, research focuses on non-dementia participants in the early stages of the disease, including mild cognitive impairment (MCI)[3,4] and subjective cognitive decline (SCD)[5-7]. MCI is thought to be an intermediate stage between being cognitively unimpaired and AD, while SCD is the initial cognitive manifestation of AD, mainly characterized by a continuous decline in self-feeling cognitive function while age-, sex-, and level of education-adjusted objective cognition assessments remaining unimpaired. With the development of neuroimaging techniques, neuroimaging markers have been the focus of AD research, including amyloid positron emission tomography (PET), Tau PET, fluorodeoxyglucose PET, and functional magnetic resonance imaging (fMRI), structural MRI, and so on. Brain atrophy, in particular, is thought to be a typical and irreversible manifestation of AD[8]. Voxelwise meta-analysis of gray matter(GM) abnormalities has shown that the amnestic MCI (aMCI, easier to progress to AD) exhibited GM atrophy in the hippocampus, parahippocampal gyrus(PHG), and amygdala[9], which are closely related to cognitive performance including memory and global cognition[10]. Previous studies have found that the hat structure mentioned above is significantly atrophied in amnestic MCI and AD patients[9,11-13].
Cognitive reserve (CR) is an adaptability of cognitive processes, which may better reflect the recruitment ability of neurons. This concept explains why cognitive abilities or daily functioning vary in their susceptibility to brain aging, pathology, or injury[14]. The frontal lobe plays a crucial role in cognitive control and cognitive reserve[15,16]. Recently proposed brain network-based global left functional cortex connectivity (gLFC connectivity) may be a potential and reliable substrate for CR, which is the positive connection from the frontal lobe to the whole brain in resting-state fMRI[17]. A wealth of research has indicated that gLFC connectivity can reduce the damage of entorhinal tau tangles and precuneus hypometabolism to the memory domain and global cognition, and delay the progression of cognitive decline[17-19]. The gLFC can promote network efficiency and enhance the reserve of memory ability in aging[20]. Previous studies have explored the effect of gLFC connectivity on the alleviation of pathological proteins or metabolism-related cognitive impairment. However, there are few studies that explore whether gLFC connectivity could alleviate the damage of brain atrophy in the memory domain and global cognition.
The cognitive reserve capacity varies between sexes[21,22]. Previous studies have found that women showed lower reserve than men at the advanced stage of AD[23,24]. Theoretical studies based on sex exploration of reserve differences provide an important basis for delaying the onset of AD by intervening in cognitive reserve-related influencing factors. However, there are few studies that explore whether CR has comparable beneficial effects in women and men separately. In summary, this study aims to explore: (1) Can gLFC connectivity alleviate cognitive impairment caused by neurodegenerative volume atrophy? (2) If so, are there differences in the effects of gLFC connectivity between sexes?
METHODS
Participants
All participants were recruited within the Sino Longitudinal Study on Cognitive Decline (SILCODE)[25], which is a project focusing on the early phase of Alzheimer’s disease. The protocol has been published previously, which described the design, procedure, and imaging parameters in detail. In the study, we included 136 participants [normal controls (NC) = 43, subjective cognitive decline (SCD) = 63, mild cognitive impairment (MCI) = 30 (amnestic MCI = 27)] aged 50-79 years and with education ≥ 9 years. Each participant underwent cognitive assessment, APOE genotype tests, sMRI, and rs-fMRI scans. Only cognitively unimpaired (CU) participants (including NC and SCD) have undergone 18F-AV45 positron emission tomography (AV45 PET) [Figure 1].
The study has been approved by the ethics committee of Xuanwu Hospital of Capital Medical University. Written informed consent was acquired from each participant. The Trial registration number is NCT03370744.
Cognitive assessment
All participants completed a systematic inquiry, including basic information, medical history, and a series of neuropsychological batteries. The details of the self-complaints were assessed by subjective cognitive decline Interview (SCD-I) and subjective cognitive decline questionnaire with nine questions (SCD9). The emotion and mood were evaluated by Hamilton Anxiety Scale (HAMA), Hamilton Depression Scale (HAMD), and Geriatric Depression Scale (GDS). The memory cognitive domain was evaluated by auditory verbal learning test (AVLT) long-term delayed recall and recognition. The speed/executive cognitive domain was assessed by Shape Trail Test-A/B (STT-A/B). The language cognitive domain was evaluated by Boston Naming Test (BNT) and Animal Fluency Test (AFT). The global cognitive function was evaluated by Montreal Cognitive Assessment-Basic (MoCA-B). Functional Activities Questionnaire (FAQ) was applied to assess the ability of daily living. All the inquiries were completed by a professional neurologist, and the diagnosis was confirmed by two experienced neurologists.
Diagnostic criteria for MCI, SCD, and NC participants
The cognitive status was based on the Jak and Bondi criteria. MCI was diagnosed if they met any one of the following three criteria proposed by Bondi[26], and failed to meet the criteria for dementia: (1) impaired scores (defined as > 1.0 SD below the age-corrected normative means) on both measures in at least one cognitive domain (memory, speed/executive function, or language); (2) impaired scores in each of the three cognitive domain (memory, speed/executive function, or language); (3) the FAQ ≥ 9. The normative means in our study are described in detail in the previous study[25].
SCD was defined by the research criteria proposed by Jessen et al.[7]: (1) compared with the previous normal cognitive status, presence of persistent self-perceived cognitive decline, and unrelated to acute event; (2) normal cognitive performance after age-, sex- and education adjustment; (3) participants expressed worry/concern related to their cognitive decline. Participants were diagnosed with NC except for SCD in participants without cognitive impairment.
Individuals were excluded as follows: individuals with dementia; major psychiatric diseases such as severe depression and anxiety; other neurological conditions that may affect brain structure and function; other systemic diseases that may lead to cognitive decline, such as thyroid dysfunction, severe anemia, syphilis or HIV; cognitive impairment caused by traumatic brain injury; unable to complete the protocol or contraindication for MRI.
Preprocessing of resting-state fMRI data and PET
Resting-state fMRI images were preprocessed using Resting-State fMRI Data Analysis Toolkit plus V1.25 (Rest-plus V1.25, http://restfmri.net/forum/index.php?q=rest)[27]. The first 10 EPI volumes were removed to avoid non-equilibrium magnetization effects. The remaining EPI volumes were processed by head motion and slice-timing corrections and then reregistered with the structural images. A multiple linear regression was performed on the six head motion parameters and the average signals of WM and CSF obtained by segmentation to eliminate other false physiological noise sources. The registered EPI volumes for normalization to MNI space by the DARTEL flow fields and affine transformation matrix, and then smoothed by 8 mm Gaussian kernel. All spatially normalized EPI images were detrended and band-pass filtered (0.01-0.08 Hz). In addition, 6 participants with head movements greater than 2 mm translations or 2 degrees rotation per axis were excluded [14].
PET images were registered into structural images, and then the registered images were spatially normalized to Montreal Neurological Institute (MNI) space by using the transformation parameters from structural MRI images, followed by a smoothing step with an 8 mm FWHM Gaussian kernel.
Analysis gLFC of connectivity
GLFC connectivity was defined by seed-based functional connectivity following a previously described protocol[19]. Left frontal cortex (MNI: x = -42, y = 6, z = 28; Brodmann area 6/44) was selected as the region of interest (ROI) referring to previous studies. A spherical region with a radius of 8 mm was used as seed to extract the average value of fMRI time series in the seed region. Pearson correlation analysis was carried out between the two seed points and each voxel in the whole brain, and the correlation coefficient (R) was the functional connection between the various seed regions and the voxel. In order to improve the normality, Fisher’s r-to-z transformation is used to transform a single correlation matrix into a z-score matrix. Finally, we calculated the mean of all positive correlations under the gray matter voxel in each participant to prevent the positive and negative connections from offsetting each other with the aim of obtaining the gLFC connectivity scores. Global functional connectivity was further computed for two unimodal control regions, which we did not expect to contribute to reserve, including one in the occipital pole (MNI: x = -19, y = -102, z = -3) and another in M1 in the motor cortex (MNI: x = -38, y = -22, z = 56)[17].
Assessment of amyloid status
18F-AV45 PET images were preprocessed using Statistical Parameter Map (SPM12, https://www.fil.ion.ucl.ac.uk/spm/software/spm12) software performed on the Matlab2016b platform. The standardized uptake value ratio (SUVR) was determined by the region tracer uptake normalized to the whole cerebellum based on Anatomical Automatic Labeling (AAL) template (area: 91-116)[28]. According to a previous study, a cutoff value of 1.18 was applied and individuals with a global average SUVR greater than 1.18 were diagnosed as Aβ+[29].
Assessment of hippocampus, parahippocampal gyrus, and amygdala volumes
The severity of brain atrophy is enormously correlated with AD pathology and memory impairment. Voxel-based morphology of the whole brain was carried out on the Computational Anatomy Toolbox 12 (Cat12, http://dbm.neuro.uni-jena.de/cat/) implemented in the SPM12 software, and the volumes of bilateral hippocampus, gray matter, and total intracranial volume were obtained.
All 3D-weight T1 images were initially segmented into gray matter (GM), white (WM), and cerebrospinal fluid (CSF), and then were normalized using Diffeomorphic Anatomical Registration Through Exponentiated Lie Algebra (DARTEL). For registration purposes, six iterations and an existing DARTEL template in MNI space, derived from 555 healthy controls of the IXI database (http://brain-development.org/ixi-dataset/), were applied. During the normalization, local GM and WM volumes are conserved by modulating the image intensity of each voxel by the Jacobian determinants of the computed deformation fields. The anatomical structures of the hippocampus, parahippocampal gyrus, and amygdala were extracted from normalized and modulated gray matter maps according to the AAL3 atlas (https://www.oxcns.org/aal3.html). In addition, the total intracranial volume of each person was estimated by adding the segmented GM, WM and CSF volumes.
Statistical analysis
Demographic, clinical, and cognitive assessments were compared between women and men. Analysis of Variance (ANOVA) was used for continuous variables and Chi-square test was performed for categorical variables. GLFC connectivity, brain volume ratios, and cognitive performance were log- and z-transformed, after which the data satisfy the normal distribution. The transformed values were used in subsequent analysis.
General linear regression analysis was conducted to assess whether volumes are a significant predictor of participants’ delayed recall performance (AVLT long term delayed recall) and global cognition (total score for MoCA-B) with age, sex, diagnosis, and education as covariates. We tested whether there is a significant interaction effect of gLFC connectivity by specific volumes on memory performance or global cognition. A significant interaction would mean that the relationship between volumes and memory performance or global cognition differs depending on the strength of gLFC connectivity.
To test whether the gLFC connectivity × specified brain volumes interaction term improves the model fit, we compared the Akaike information criterion of the full model (Model 1: with interaction term) to that of the reduced model (Model2: without interaction term). The Akaike information criterion is an estimate of the quality of a statistical model given a particular set of data.
Furthermore, to test the robustness of our study, we repeated the above analysis accounting for (1) age, sex, diagnosis based on amyloidosis (participants were divided into Aβ-, Aβ+, and MCI), and education as covariates; and (2) age, sex, diagnosis, education, and APOE4 carrying state as covariates.
We further conducted the above general linear analysis accounting for age, diagnosis, and education as covariates in women and men separately. All analyses were performed using the SPSS (IBM for Windows) v20.00; differences were considered significant when meeting a P value < 0.05.
RESULTS
Demographic and neuropsychological characteristics among three groups
The information for each group is available in Table 1. There were no significant differences in age, education, amyloid status, and APOE4 carrying status among groups. SCD groups showed a higher percentage of women than MCI groups (P = 0.009). MCI participants performed worse than NC or SCD participants in all objective cognitive performances [Table 1]. For the memory domain, MCI participants showed lower scores of AVLT long-term delayed recall and recognition than NC or SCD participants
Demographic and neuropsychological characteristics of participants
NC | SCD | MCI | F/χ2 | P | |
Sample size, n | 43 | 63 | 30 | ||
Age, y | 66.93 (5.04) | 65.6 (5.24) | 68.53 (8.87) | 2.356 | 0.099 |
Education | 12.72 (3.07) | 12.65 (2.92) | 11.33 (3.34) | 2.261 | 0.108 |
SCD9†,‡ | 2.72 (2.07) | 5.5 (1.72) | 5.02 (2.1) | 28.052 | < 0.001*** |
Hamilton Depression Scale† | 2.21 (2.49) | 4.11 (3.96) | 3.43 (3.52) | 3.863 | 0.023* |
Hamilton Anxiety Scale† | 2.93 (2.99) | 5.11 (4.36) | 3 (2.79) | 5.858 | 0.004** |
AVLT-long-term delayed recall‡,§ | 7.56 (2.15) | 7.59 (2.1) | 2.4 (1.96) | 71.927 | < 0.001*** |
AVLT-recognition‡,§ | 22.51 (1.55) | 22.16 (1.56) | 17.93 (3.02) | 58.029 | < 0.001*** |
Shape trail test-A | 60.07 (15.43) | 55.08 (17.33) | 92.08 (35.55) | 29.788 | < 0.001*** |
Shape trail test-B | 136.37 (31.97) | 128.19 (34.14) | 213.93 (64.49) | 43.043 | < 0.001*** |
Animal Fluency Test‡,§ | 18.67 (4.12) | 19.67 (5.16) | 14.57 (4.66) | 12.024 | < 0.001*** |
Boston Naming Test‡,§ | 26.05 (2.94) | 25.4 (2.85) | 21.97 (3.85) | 16.923 | < 0.001*** |
Montreal Cognitive Assessment-Basic‡,§ | 26.07 (2.47) | 26.16 (2.19) | 20.13 (3.27) | 64.523 | < 0.001*** |
Sex§, F/M | 25/18 | 46/17 | 12/18 | 9.535 | 0.009** |
Amyloid status, -/+ | 26/17 | 42/21 | - | 0.427 | 0.513 |
APOE4 carriers, -/+ | 32/11 | 42/21 | 17/13 | 2.518 | 0.284 |
Seed-based global left frontal cortex connectivity
Figure 2A-D depicts seed-based gLFC-connectivity results, illustrating higher connectivity in frontal and parietal regions. Women showed lower gLFC connectivity compared to men (β = -0.55, P = 0.002). No significant differences in gLFC connectivity were observed among NC, SCD, and MCI participants, nor between Aβ- and Aβ+ groups.
Figure 2. (A) Blue sphere shows the left frontal cortex as the seed, and whole brain functional connectivity pattern is mainly located in frontal and parietal lobes; Seed-based global left frontal cortex connectivity and differences in gLFC-connectivity between (B) women and men, (C) NC, SCD, and MCI, (D) Aβ- and Aβ+ participants. **P < 0.01. ns: Not significant; gLFC: global left frontal cortex; NC: normal control; SCD: subjective cognitive decline; MCI: mild cognitive impairment.
Hippocampus, parahippocampal gyrus and amygdala volumes
Figure 3 shows the differences in HpVR values, PhgVR values, and AmyVR values between different subgroups. Women showed higher HpVR values than men (β = -0.42, SE = 0.14, P = 0.004). There were no significant differences in PhgVR and AmyVR between women and men. In different diagnostic groups, MCI participants showed lower HpVR, PhgVR, and AmyVR values than NC and SCD participants, with no significant differences observed between NC and SCD groups. Aβ+ participants showed lower PhgVR values than Aβ-(β = 0.34, SE = 0.16, P = 0.038). There were no significant differences in HpVR and AmyVR between Aβ+ and Aβ- groups.
Figure 3. Differences between HpVR, PhgVR and AmyVR in men and women groups (A, D, and G); Differences between HpVR, PhgVR, and AmyVR in AD disease spectrum (B, E, and H); Differences between HpVR, PhgVR, and AmyVR in Aβ- and Aβ+ (C,F and I). *P < 0.05, **P < 0.01. ns: Not significant; HpVR: hippocampus volume/intracranial volume ratio; PhgVR: parahippocampal gyrus volume/intracranial volume ratio; AmyVR: amygdala volume/intracranial volume ratio; NC: normal control; SCD: subjective cognitive decline; MCI: mild cognitive impairment.
Association between cognitive performance and brain volumes ratios
AD-related brain volume values were correlated with delayed recall performance and global cognition. General linear model accounting for age, sex, diagnosis, and education showed that HpVR values were significantly associated with delayed recall performance (β = 0.32, SE = 0.08, P < 0.001) and global cognition (β = 0.31, SE = 0.08, P < 0.001), while PhgVR values (delayed recall performance: β = 0.19, SE = 0.07,
Figure 4. The correlation between HpVR, MoCA-B and delayed recall performance (A and B); Correlation between PhgVR, MoCA-B and delayed recall performance (C and D); Correlation between AmyVR, MoCA-B and delayed recall performance (E and F). AD: Alzheimer’s disease; HpVR: hippocampus volume/intracranial volume ratio; PhgVR: parahippocampal gyrus volume/intracranial volume ratio; AmyVR: amygdala volume/intracranial volume ratio; MoCA-B: Montreal Cognitive Assessment-Basic; AVLT: auditory verbal learning test.
Interaction effects of HpVR * gLFC connectivity, PhgVR * gLFC connectivity, AmyVR * gLFC connectivity on cognition
We examined whether increased gLFC connectivity attenuates the impact of lower HpVR, PhgVR, and AmyVR on memory and global cognition. The interaction effects of HpVR * gLFC connectivity,
Figure 5. Interaction between HpVR/PhgVR/AmyVR and gLFC on global cognition. The association between (A) HpVR or (B) PhgVR or (C) AmyVR and MoCA-B are plotted. HpVR: Hippocampus volume/intracranial volume ratio; PhgVR: parahippocampal gyrus volume/intracranial volume ratio; AmyVR: amygdala volume/intracranial volume ratio; gLFC: global left frontal cortex; MoCA-B: Montreal Cognitive Assessment-Basic.
The interaction effects of AmyVR * gLFC connectivity (β = -0.13, SE = 0.08, P = 0.088) were marginally significant. Based on AIC scores, Model 1 was better than Model 2. For HpVR*gLFC connectivity on global cognition, the AIC of full model was 286.45 and reduced model was 293.73. For PhgVR, the AIC of Model 1 was 286.59 and Model 2 was 291.97. For AmyVR, the AIC of Model 1 was 282.68 and Model 2 was 284.91.
The control ROIs included M1 and occipital pole as the independent variable, and separate linear analyses showed that there were no interactions with HpVR, PhgVR, or AmyVR on global cognition (for the M1 control ROI: HpVR: P = 0.353; PhgVR: P = 0.134; AmyVR: P = 0.328; for the occipital pole control ROI:
Summary of linear models
β | SE | t | P | 95%CI lower | 95%CI upper | |
Reserve effect of gLFC connectivity | ||||||
HpVR * gLFC connectivity | -0.17 | 0.08 | -2.18 | 0.031* | -0.33 | -0.02 |
HpVR | 0.25 | 0.09 | 2.77 | 0.006** | 0.07 | 0.42 |
gLFC connectivity | -0.01 | 0.07 | -0.15 | 0.884 | -0.14 | 0.12 |
Reserve effect of gLFC connectivity | ||||||
PhgVR * gLFC connectivity | -0.21 | 0.07 | -2.80 | 0.006** | -0.35 | -0.06 |
PhgVR | 0.19 | 0.07 | 2.64 | 0.009** | 0.05 | 0.34 |
gLFC connectivity | 0.02 | 0.07 | 0.27 | 0.785 | -0.12 | 0.15 |
Reserve effect of gLFC connectivity | ||||||
AmyVR * gLFC connectivity | -0.13 | 0.08 | -1.72 | 0.088 | -0.29 | 0.02 |
AmyVR | 0.31 | 0.08 | 3.80 | < 0.001*** | 0.15 | 0.47 |
gLFC connectivity | -0.01 | 0.07 | -0.20 | 0.840 | -0.14 | 0.12 |
No reserve effect for control ROI, M1, and occipital pole | ||||||
HpVR * M1 connectivity | -0.09 | 0.10 | -0.93 | 0.353 | -0.28 | 0.10 |
PhgVR * M1 connectivity | -0.12 | 0.08 | -1.51 | 0.134 | -0.27 | 0.04 |
AmyVR * M1 connectivity | -0.08 | 0.08 | -0.98 | 0.328 | -0.24 | 0.08 |
HpVR * occipital connectivity | -0.06 | 0.07 | -0.88 | 0.382 | -0.19 | 0.07 |
PhgVR * occipital connectivity | -0.07 | 0.06 | -1.11 | 0.268 | -0.19 | 0.05 |
AmyVR * occipital connectivity | -0.03 | 0.06 | -0.55 | 0.584 | -0.16 | 0.09 |
gLFC connectivity is not affected by amyloid status but by sex | ||||||
Amyloid status† | -0.15 | 0.20 | -0.74 | 0.459 | -0.55 | 0.25 |
Sex | -0.55 | 0.18 | -3.09 | 0.002** | -0.90 | -0.20 |
When considering (1) age, sex, diagnosis based on amyloidosis (participants were divided into Aβ-, Aβ+, and MCI), and education as covariates; or (2) age, sex, diagnosis, education, and APOE4 carrying status as covariates, the significant results still existed [Supplementary Tables 1 and 2].
Higher gLFC connectivity attenuates the adverse effect of parahippocampal gyrus and amygdala atrophy on global cognition in men
We divided the cohort into men and women subgroups to explored the potential differences in interaction effects between men and women (demographic information of men and women subgroups is provided in Supplementary Table 3). Firstly, we repeated the linear analysis to evaluate the association between specific volumes ratios and cognition in men and women separately. General linear model showed that HpVR values were related to cognitive decline in women (β = 0.38, SE = 0.11, P = 0.001) not in men (β = 0.17, SE = 0.12, P = 0.157), while PhgVR values were associated with global cognition both in men (β = 0.24, SE = 0.10, P = 0.015) and women (β = 0.25, SE = 0.11, P = 0.020). AmyVR values showed the same tendency in women (β = 0.35, SE = 0.10, P = 0.001) and men ( β = 0.32, SE = 0.11, P = 0.005).
We examined whether increased gLFC connectivity attenuates the impact of lower HpVR, PhgVR, and AmyVR on memory and global cognition in men and women separately. In general, the interaction effects occurred in men but not in women [Table 3]. In men, the interaction effects of HpVR * gLFC connectivity
Figure 6. Interaction between PhgVR/AmyVR and gLFC on global cognition in women and men. The association between PhgVR and MoCA-B is plotted separately for (A) men and (B) women; The association between AmyVR and MoCA-B is plotted for (C) men and (D) women. PhgVR: Parahippocampal gyrus volume/intracranial volume ratio; AmyVR: amygdala volume/intracranial volume ratio; gLFC: global left frontal cortex; MoCA-B: Montreal Cognitive Assessment-Basic.
Summary of linear models in women and men separately
Women | Men | |||||||||||
β | SE | t | P | 95%CI lower | 95%CI upper | β | SE | t | P | 95%CI lower | 95%CI upper | |
Reserve effect of gLFC connectivity | ||||||||||||
HpVR * gLFC connectivity | -0.02 | 0.14 | -0.11 | 0.914 | -0.30 | 0.27 | -0.34 | 0.11 | -3.00 | 0.004** | -0.57 | -0.11 |
HpVR | 0.40 | 0.17 | 2.30 | 0.024* | 0.05 | 0.75 | 0.17 | 0.11 | 1.56 | 0.126 | -0.05 | 0.40 |
gLFC connectivity | -0.10 | 0.11 | -0.94 | 0.350 | -0.32 | 0.11 | 0.08 | 0.09 | 0.84 | 0.404 | -0.10 | 0.25 |
Reserve effect of gLFC connectivity | ||||||||||||
PhgVR * gLFC connectivity | -0.18 | 0.11 | -1.75 | 0.085 | -0.39 | 0.03 | -0.25 | 0.10 | -2.48 | 0.017* | -0.46 | -0.05 |
PhgVR | 0.17 | 0.11 | 1.58 | 0.119 | -0.04 | 0.38 | 0.28 | 0.10 | 2.74 | 0.009** | 0.07 | 0.48 |
gLFC connectivity | -0.04 | 0.10 | -0.36 | 0.722 | -0.23 | 0.16 | 0.15 | 0.09 | 1.63 | 0.111 | -0.04 | 0.33 |
Reserve effect of gLFC connectivity | ||||||||||||
AmyVR * gLFC connectivity | -0.06 | 0.11 | -0.55 | 0.582 | -0.28 | 0.16 | -0.25 | 0.11 | -2.28 | 0.027* | -0.48 | -0.03 |
AmyVR | 0.34 | 0.12 | 2.70 | 0.009** | 0.09 | 0.59 | 0.30 | 0.10 | 2.82 | 0.007** | 0.09 | 0.51 |
gLFC connectivity | -0.08 | 0.10 | -0.77 | 0.445 | -0.27 | 0.12 | 0.08 | 0.09 | 0.96 | 0.343 | -0.09 | 0.26 |
DISCUSSION
This study has enrolled participants in the early phase of Alzheimer’s disease, including NC, SCD, and MCI participants. It was found that higher gLFC connectivity levels could attenuate the adverse effect of the hippocampus and parahippocampal gyrus atrophy on global cognition, while the amygdala showed marginal significance. The significant results still existed after accounting for APOE4 carrying status or diagnosis based on amyloidosis. Additional analysis conducted separately for different sexes has shown that the gLFC connectivity, serving as the marker of cognitive reserve, attenuated the effects of parahippocampal gyrus and amygdala atrophy on global cognition exclusively in men.
Our results indicated that MCI participants exhibited smaller volumes of hippocampus, parahippocampal gyrus, and amygdala compared to cognitively unimpaired participants. At the same time, volumes of hippocampus, parahippocampal gyrus, and amygdala were strongly associated with memory and global cognition, which is consistent with previous studies. A meta-analysis showed that regional atrophy of hippocampus, parahippocampal gyrus, and amygdala was found in aMCI patients[9]. The hippocampus and parahippocampal gyrus volumes are closely related to memory and overall cognitive function, which are valuable predictors of progression from aMCI to AD[12,13]. The amygdala is an important brain region in the early phase of AD research, and the magnitude of amygdala atrophy is comparable to that of the hippocampus in the earliest clinical stages of AD, and is related to global illness severity[11]. Brain atrophy, as a structural degenerative disease, is irreversible and is the cause of memory and cognitive decline. Our study supports the hypothesis that these brain regions are key regions to memory and global cognitive impairment due to atrophy, and gLFC connectivity buffered this negative effect.
Our study further supported the idea that gLFC connectivity was a reliable hallmark for CR. The results have expanded the explanation of cognitive capacity for hippocampus, parahippocampal gyrus, and amygdala atrophy-related cognitive impairment. Boots et al. found that occupational complexity, a proxy for cognitive reserve, was associated with decreased hippocampal volume when matched for cognitive function[30]. A functional MRI research showed that recruitments of temporal lobe, contributing to cognitive reserve, protected against the detrimental effect of hippocampal atrophy on associate memory[31]. A psychometric approach that models reserve as residual cognition not explained by demographic and brain variables could buffer against the negative effects of brain atrophy[32]. Previous studies have shown that education, a commonly used proxy for reserve capacity, was positively associated with hippocampal volumes[33,34], while Kalzendorf et al. found no association between CR and either microstructural or macrostructural alterations of the hippocampus in older adults[35]. Our study then found that
Our study provides a potential mechanism that gLFC connectivity can alleviate the detrimental effects of hippocampus, parahippocampal gyrus and amygdala atrophy on global cognition.
In the subgroup analysis based on different sexes, the function of gLFC connectivity that could alleviate the detrimental effects of parahippocampal gyrus and amygdala atrophy on global cognition was only observed in men, which may be related to several reasons: (1) The gLFC connectivity of women is at a lower level, which means in women groups, distinguishing between higher and lower levels of gLFC connectivity is not feasible through statistical analysis with interaction term. A previous study showed that women showed lower gLFC connectivity than men in cognitively unimpaired participants[39]; (2) The longitudinal analysis reveals that women and men showed different patterns and rates of decline over time compared to controls[40,41]. Women showed higher HpVR values than men in our results; however, Shen et al. have found that longitudinal reduction of hippocampal volume ratio (HpVR) was linked to women in the NC and MCI groups, but not in the AD group[42]. (3) GLFC connectivity has been considered as just one of the proxies of CR. Malpetti et al. found that elevated anterior cingulate metabolism may function as a reserve substrate, providing similar benefits to women and men in mitigating the rate of cognitive decline during advanced stages of AD[22,23].
Although this study confirmed the reserve effect of gLFC connectivity, there are still some shortcomings: (1) For neurodegenerative markers, hippocampus, parahippocampal gyrus, and amygdala were chosen based on a meta-analysis[9]; however, there are amount of brain volumes involved in the progression of AD including the middle temporal gyrus, etc. Even so, we considered the variance of TIV, and region of interest brain volume/whole brain volume ratios were applied, which are more reliable[42]; (2) Our study was a cross-sectional study involving only NC, SCD, and MCI participants. It is not known whether the same effect exists in participants with mild dementia, which is an advanced stage of the disease. In the second place, amyloid status was informed only in NC and SCD; women may show more susceptibility to cognitive decline related to amyloidosis[43]. Large sample studies to explore the interaction of CR, sex, and amyloidosis on cognition are still needed in the future; (3) Intracranial changes are a dynamic process, and the longitudinal effects of cognitive reserves on cognition in different sexes are still unknown, which is also a future research direction; (4) The structural aging of the brain is closely related to age. Even though we considered age a factor in our linear regression, this does not completely eliminate its impact. This requires careful consideration. In future research, we would match the ages of the subject groups. Additionally, the impact of age on cognition is also a point of interest for us. In summary, a longitudinal and large sample study is necessary and we should take such findings with extreme caution when extrapolating the results of this study.
In conclusion, gLFC connectivity is linked to enhanced resilience against the detrimental impact of specific brain atrophy on global cognition, with this effect being more pronounced in men, offering implications for future intervention strategies.
DECLARATIONS
Authors’ contributions
Data acquisition, experimental design, statistical analysis, manuscript editing and review: Du W
Data processing, statistical analysis, and manuscript revision: Ding C
Manuscript editing and review: Zhong J
Data acquisition, manuscript editing: Li Y, Fan C
Experimental design, data analysis, manuscript editing and review: Jiang J, Han Y
Availability of data and materials
The datasets used or analyzed during the current study are available from the corresponding author upon reasonable request.
Financial support and sponsorship
This work was supported by the National High Level Hospital Clinical Research Funding-2023-NHLHCRF-BQ-06 and the National Natural Science Foundation of China (Grant 82001773 and 82020108013).
Conflicts of interest
All authors report no disclosures.
Ethical approval and consent to participate
This research program has been reviewed and approved by the Ethics Committee of Xuanwu Hospital of Capital Medical University [No. (2017)046] to ensure that all the implementation processes of this research are in line with the Helsinki Declaration. Written informed consent was acquired from each participant. The Trial registration number is NCT03370744.
Consent for publication
Not applicable.
Copyright
© The Author(s) 2024.
REFERENCES
3. Jia L, Du Y, Chu L, et al; COAST Group. Prevalence, risk factors, and management of dementia and mild cognitive impairment in adults aged 60 years or older in China: a cross-sectional study. Lancet Public Health 2020;5:e661-71.
4. Langa KM, Levine DA. The diagnosis and management of mild cognitive impairment: a clinical review. JAMA 2014;312:2551-61.
5. Jessen F, Amariglio RE, Buckley RF, et al. The characterisation of subjective cognitive decline. Lancet Neurol 2020;19:271-8.
6. Jessen F, Wolfsgruber S, Kleineindam L, et al. Subjective cognitive decline and stage 2 of Alzheimer disease in patients from memory centers. Alzheimers Dement 2023;19:487-97.
7. Jessen F, Amariglio RE, van Boxtel M, et al; Subjective Cognitive Decline Initiative (SCD-I) Working Group. A conceptual framework for research on subjective cognitive decline in preclinical Alzheimer’s disease. Alzheimers Dement 2014;10:844-52.
8. Gao F, Lv X, Dai L, et al. A combination model of AD biomarkers revealed by machine learning precisely predicts Alzheimer’s dementia: China Aging and Neurodegenerative Initiative (CANDI) study. Alzheimers Dement 2022;19:749-60.
9. Chen S, Xu W, Xue C, et al. Voxelwise meta-analysis of gray matter abnormalities in mild cognitive impairment and subjective cognitive decline using activation likelihood estimation. J Alzheimers Dis 2020;77:1495-512.
10. Fan LY, Lai YM, Chen TF, et al. Diminution of context association memory structure in subjects with subjective cognitive decline. Hum Brain Mapp 2018;39:2549-62.
11. Poulin SP, Dautoff R, Morris JC, Barrett LF, Dickerson BC; Alzheimer’s Disease Neuroimaging Initiative. Amygdala atrophy is prominent in early Alzheimer’s disease and relates to symptom severity. Psychiatry Res 2011;194:7-13.
12. Chen J, Zhang Z, Li S. Can multi-modal neuroimaging evidence from hippocampus provide biomarkers for the progression of amnestic mild cognitive impairment? Neurosci Bull 2015;31:128-40.
13. Ferreira LK, Diniz BS, Forlenza OV, Busatto GF, Zanetti MV. Neurostructural predictors of Alzheimer’s disease: a meta-analysis of VBM studies. Neurobiol Aging 2011;32:1733-41.
14. Stern Y, Arenaza-Urquijo EM, Bartrés-Faz D, et al; the Reserve, Resilience and Protective Factors PIA Empirical Definitions and Conceptual Frameworks Workgroup. Whitepaper: defining and investigating cognitive reserve, brain reserve, and brain maintenance. Alzheimers Dement 2020;16:1305-11.
15. Jiang J, Liu T, Crawford JD, et al. Stronger bilateral functional connectivity of the frontoparietal control network in near-centenarians and centenarians without dementia. Neuroimage 2020;215:116855.
16. Barulli D, Stern Y. Efficiency, capacity, compensation, maintenance, plasticity: emerging concepts in cognitive reserve. Trends Cogn Sci 2013;17:502-9.
17. Neitzel J, Franzmeier N, Rubinski A, Ewers M; Alzheimer’s Disease Neuroimaging Initiative (ADNI). Left frontal connectivity attenuates the adverse effect of entorhinal tau pathology on memory. Neurology 2019;93:e347-57.
18. Franzmeier N, Düzel E, Jessen F, et al. Left frontal hub connectivity delays cognitive impairment in autosomal-dominant and sporadic Alzheimer’s disease. Brain 2018;141:1186-200.
19. Franzmeier N, Duering M, Weiner M, Dichgans M, Ewers M; Alzheimer’s Disease Neuroimaging Initiative (ADNI). Left frontal cortex connectivity underlies cognitive reserve in prodromal Alzheimer disease. Neurology 2017;88:1054-61.
20. Franzmeier N, Hartmann J, Taylor ANW, et al. The left frontal cortex supports reserve in aging by enhancing functional network efficiency. Alzheimers Res Ther 2018;10:28.
21. Subramaniapillai S, Almey A, Natasha Rajah M, Einstein G. Sex and gender differences in cognitive and brain reserve: implications for Alzheimer’s disease in women. Front Neuroendocrinol 2021;60:100879.
22. Ewers M. Reserve in Alzheimer’s disease: update on the concept, functional mechanisms and sex differences. Curr Opin Psychiatry 2020;33:178-84.
23. Malpetti M, Ballarini T, Presotto L, Garibotto V, Tettamanti M, Perani D; Alzheimer’s Disease Neuroimaging Initiative (ADNI) database; Network for Efficiency and Standardization of Dementia Diagnosis (NEST-DD) database. Gender differences in healthy aging and Alzheimer’s Dementia: A18 F-FDG-PET study of brain and cognitive reserve. Hum Brain Mapp 2017;38:4212-27.
24. Perneczky R, Drzezga A, Diehl-Schmid J, Li Y, Kurz A. Gender differences in brain reserve: an (18)F-FDG PET study in Alzheimer’s disease. J Neurol 2007;254:1395-400.
25. Li X, Wang X, Su L, Hu X, Han Y. Sino Longitudinal Study on Cognitive Decline (SILCODE): protocol for a Chinese longitudinal observational study to develop risk prediction models of conversion to mild cognitive impairment in individuals with subjective cognitive decline. BMJ Open 2019;9:e028188.
26. Bondi MW, Edmonds EC, Jak AJ, et al. Neuropsychological criteria for mild cognitive impairment improves diagnostic precision, biomarker associations, and progression rates. J Alzheimers Dis 2014;42:275-89.
27. Jia XZ, Wang J, Sun HY, et al. RESTplus: an improved toolkit for resting-state functional magnetic resonance imaging data processing. Sci Bull 2019;64:953-4.
28. Villemagne VL, Burnham S, Bourgeat P, et al; Australian Imaging Biomarkers and Lifestyle (AIBL) Research Group. Amyloid β deposition, neurodegeneration, and cognitive decline in sporadic Alzheimer’s disease: a prospective cohort study. Lancet Neurol 2013;12:357-67.
29. Li TR, Wu Y, Jiang JJ, et al. Radiomics analysis of magnetic resonance imaging facilitates the identification of preclinical Alzheimer’s disease: an exploratory study. Front Cell Dev Biol 2020;8:605734.
30. Boots EA, Schultz SA, Almeida RP, et al. Occupational complexity and cognitive reserve in a middle-aged cohort at risk for Alzheimer’s disease. Arch Clin Neuropsychol 2015;30:634-42.
31. Belleville S, Mellah S, Cloutier S, et al; CIMA-Q group. Neural correlates of resilience to the effects of hippocampal atrophy on memory. Neuroimage Clin 2021;29:102526.
32. Bettcher BM, Gross AL, Gavett BE, et al. Dynamic change of cognitive reserve: associations with changes in brain, cognition, and diagnosis. Neurobiol Aging 2019;83:95-104.
33. Baumgaertel J, Haussmann R, Gruschwitz A, et al. Education and genetic risk modulate hippocampal structure in Alzheimer’s disease. Aging Dis 2016;7:553-60.
34. Shpanskaya KS, Choudhury KR, Hostage C Jr, Murphy KR, Petrella JR, Doraiswamy PM; Alzheimer’s Disease Neuroimaging Initiative. Educational attainment and hippocampal atrophy in the Alzheimer’s disease neuroimaging initiative cohort. J Neuroradiol 2014;41:350-7.
35. Kalzendorf J, Brueggen K, Teipel S. Cognitive reserve is not associated with hippocampal microstructure in older adults without dementia. Front Aging Neurosci 2019;11:380.
36. Perry BL, Roth AR, Peng S, Risacher SL, Saykin AJ, Apostolova LG. Social networks and cognitive reserve: network structure moderates the association between amygdalar volume and cognitive outcomes. J Gerontol B Psychol Sci Soc Sci 2022;77:1490-500.
37. Scott SA, DeKosky ST, Sparks DL, Knox CA, Scheff SW. Amygdala cell loss and atrophy in Alzheimer’s disease. Ann Neurol 1992;32:555-63.
38. Tang X, Holland D, Dale AM, Younes L, Miller MI; Alzheimer’s Disease Neuroimaging Initiative. The diffeomorphometry of regional shape change rates and its relevance to cognitive deterioration in mild cognitive impairment and Alzheimer’s disease. Hum Brain Mapp 2015;36:2093-117.
39. Du W, Ding C, Jiang J, Han Y. Women exhibit lower global left frontal cortex connectivity among cognitively unimpaired elderly individuals: a pilot study from SILCODE. J Alzheimers Dis 2021;83:653-63.
40. Skup M, Zhu H, Wang Y, et al; Alzheimer’s Disease Neuroimaging Initiative. Sex differences in grey matter atrophy patterns among AD and aMCI patients: results from ADNI. Neuroimage 2011;56:890-906.
41. Hua X, Hibar DP, Lee S, et al; Alzheimer’s Disease Neuroimaging Initiative. Sex and age differences in atrophic rates: an ADNI study with n=1368 MRI scans. Neurobiol Aging 2010;31:1463-80.
42. Shen S, Zhou W, Chen X, Zhang J; for Alzheimer’s Disease Neuroimaging Initiative. Sex differences in the association of APOE ε4 genotype with longitudinal hippocampal atrophy in cognitively normal older people. Eur J Neurol 2019;26:1362-9.
Cite This Article

How to Cite
Download Citation
Export Citation File:
Type of Import
Tips on Downloading Citation
Citation Manager File Format
Type of Import
Direct Import: When the Direct Import option is selected (the default state), a dialogue box will give you the option to Save or Open the downloaded citation data. Choosing Open will either launch your citation manager or give you a choice of applications with which to use the metadata. The Save option saves the file locally for later use.
Indirect Import: When the Indirect Import option is selected, the metadata is displayed and may be copied and pasted as needed.
About This Article
Copyright
Data & Comments
Data
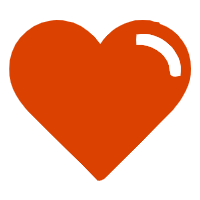
Comments
Comments must be written in English. Spam, offensive content, impersonation, and private information will not be permitted. If any comment is reported and identified as inappropriate content by OAE staff, the comment will be removed without notice. If you have any queries or need any help, please contact us at [email protected].