Activator protein-1 in liver pathology: from precancerous diseases to hepatocellular carcinoma
Abstract
Activator protein 1 (AP-1) is a family of transcription factors composed of Jun, Fos, activating transcription factor (ATF), and musculoaponeurotic fibrosarcoma (MAF) homodimers and heterodimers that regulate diverse cellular processes such as proliferation, apoptosis, differentiation, survival, and migration. Accordingly, AP-1 plays an essential role in several diseases including cancer. During liver cancer development, AP-1 abnormal activation has been implicated from cancer initiation to cancer progression. This review aims to provide a current integrative understanding of the role of AP-1 in liver carcinogenesis and suggests potential settings for its clinical use in diagnosis and treatment. Novel AP-1-based therapeutic approaches deserve further investigation and clinical application for the benefit of liver cancer patients.
Keywords
INTRODUCTION
Activator protein 1 (AP-1) is a widely distributed transcription factor found in various cell types, playing an essential role in the regulation of gene transcription. Structurally, AP-1 is composed of homodimers and heterodimers that originate from protein families including Jun (comprising c-Jun, JunB, and JunD), Fos (encompassing c-Fos, FosB, Fra1, and Fra2), activating transcription factor (ATF) (including ATF2-7, ATF3/LRF1, B-ATF1-3, JDP1 and 2), and musculoaponeurotic fibrosarcoma (MAF) (involving c-MAF, MAFA, MAFB, and MAFF, MAFG, MAFK). These subunits contain highly conserved dimeric basic leucine zipper (bZIP) DNA-binding domains, allowing them to form various dimer combinations[1]. In particular, the Fos/Jun heterodimer represents the most prevalent form of AP-1, while the Jun/Jun homodimer displays lower affinity among them and is less abundant[2].
Furthermore, AP-1 has a pivotal role in orchestrating cellular responses to acute challenges; for instance, it is involved in oxidative stress and DNA damage response. Beyond its essential role in immune-related disorders, AP-1 also demonstrates a multifaceted capacity to govern major cellular processes that promote cancer, namely, proliferation, apoptosis, differentiation, survival, and migration, among others[3].
Of particular significance, the oncoproteins c-Jun and c-Fos have been subjected to comprehensive characterization for their major contributions to virus-induced tumorigenesis, especially in the initiation and progression of tumors[2,4,5].
It is noteworthy to mention that some proteins involved in cancer-associated processes may have opposite roles depending on the cellular context/environment, such as proteins involved in apoptosis. In fact, AP-1 has also been reported to possess antitumor activity, as JunB and JunD have been identified as tumor suppressors as they can repress cyclin D1 (CycD1) and are negative regulators of cell growth[6,7]. Whether AP-1 is oncogenic or anti-oncogenic depends on the cell type, tumor development stage, and genetic context[7]. This very important topic related to the opposite roles of proteins involved in cancer-associated processes, as well as other very important issues regarding the role of AP-1 in a variety of normal and pathological conditions other than hepatocellular carcinoma (HCC), undoubtedly deserves special extensive review.
The AP-1 family contributes to the development of many types of cancer, particularly in liver cancer; c-Jun activity enhances tumor development during the early stages, and its inactivation drastically reduces the number and size of liver tumors in mice[8]. However, its involvement in precancerous stages has yet to be fully explored. This review aims to provide a current integrative understanding of the role of AP-1 in the development and progression of liver cancer, specifically HCC. The diverse and potential molecular mechanisms by which AP-1 may enhance progression to cancer from precancer stages, as well as its participation in several hallmarks of cancer, emphasize its potential clinical use in the diagnosis and treatment of HCC.
AP-1 ACTIVATION AND EXPRESSION DURING CHRONIC LIVER DISEASE
The role of AP-1 during earlier stages of tumor establishment has attracted considerable attention. In clinical investigations, AP-1 has been consistently reported to be overexpressed in liver tissue at various stages before the development of HCC. For instance, analysis of liver biopsies obtained from patients previously diagnosed with metabolic dysfunction-associated steatotic liver disease (MASLD) and metabolic dysfunction-associated steatohepatitis (MASH) reported increased expression of hepatic AP-1/c-Jun[9]. Another study informed a marked increase in AP-1 binding to liver DNA in obese patients diagnosed with MASH, compared to control subjects; this heightened AP-1 activity may be a consequence of elevated oxidative stress resulting from the metabolic dysregulation characteristic of obesity[10].
Eferl et al. found that c-Jun inhibits apoptosis through antagonism of the tumor suppressor activity of p53, which interestingly only occurred during the early stages of cancer development in animal models[8]. This finding was further supported by Min et al., who found increased expression of c-Jun and surviving, and decreased levels of c-Fos and SIRT6 in human dysplastic liver nodules but not in well-established malignant liver tumors. Using a diethylnitrosamine (DEN)-induced liver cancer model in mice, the authors suggested that AP-1 may regulate cell survival during cancer initiation through the modulation of survivin activity and may also function in parallel with nuclear factor kappa B (NF-κB) signaling[11]. Survivin is an important anti-apoptotic protein whose expression is regulated by SIRT6, so it makes sense that c-Jun-mediated inhibition of SIRT6 generates cancer-promoting activities[12].
Dorn et al. suggest that c-Jun facilitates the development and progression of MASH. Their observations indicated that c-Jun is not only overexpressed, but also activated in the livers of mice subjected to a MASH-inducing diet. Furthermore, they found elevated c-Jun in liver tissues of MASLD patients, with this increased expression correlating with inflammation and the extent of hepatic steatosis[13]. Additionally, it is known that under hypoxic conditions in liver tissue, the DNA binding activities of hypoxia-inducible factor 1 alpha (HIF-1α), NF-κB, and
The tumor microenvironment plays a relevant role in the pathogenesis of chronic liver disease [Figure 1]. Schulien et al. studied the participation of c-Jun during MASH in c-Jun knockout mice fed with a methionine- and choline-deficient diet (MCD). Their findings revealed that c-Jun exhibits cell type-dependent activities; in hepatocytes, it promotes cell survival and restricts fibrogenesis, while in non-parenchymal liver cells (NPLC), it promotes fibrogenesis through the expression of the fibrogenic gene Opn and its receptor CD44[9]. Since Opn is a well-identified target gene of AP-1[15], this transcription factor, as well as Hedgehog signaling, may be involved in liver damage during progression to MASH[16,17].
Figure 1. AP-1 in chronic liver disease. The AP-1 transcription factor has been described to play a critical role in the pathogenesis and progression of various liver diseases, including precancerous diseases and HCC. This scheme illustrates the different mechanisms modulated by abnormal AP-1 activity, which enhance a context of chronic liver damage, such as MASLD and cirrhosis, preceding the development of HCC. AP-1 is involved in both the damaged hepatocyte and non-parenchymal liver cells through the regulation of gene expression, leading to disease progression. Furthermore, AP-1 promotes the activity mediated by HBV and HCV to eventually favor hepatic pathogenesis. Figure created in Biorender.com. MASLD: Metabolic dysfunction-associated steatotic liver disease; MASH: metabolic dysfunction-associated steatohepatitis; PLC: parenchymal liver cells; NPLC: non-parenchymal liver cells; AP-1: activator protein 1; HCC: hepatocellular carcinoma; HBV: hepatitis B viruses; HCV: hepatitis C viruses; HSCs: hepatic stellate cells; JNK: c-Jun NH2-terminal kinase; TGF-β1: transforming growth factor-β1; IKK: IκB kinase; ER: endoplasmic reticulum; NF-κB: nuclear factor kappa B; MMPs: matrix metallopeptidases; COX-2: cyclooxygenase 2; TIMP1: tissue inhibitor of metalloprotease-1; ROS: reactive oxygen species.
A mouse model with constitutive overexpression of Fra-1 was studied by Kireva et al., and they found that these animals developed liver fibrosis. They also found upregulation of genes involved with collagen production, profibrogenic and fibrolytic genes, including strong induction of procollagen α1 (I), α2 (I), and α1 mRNA expression, as well as induction of the profibrogenic cytokine transforming growth factor-β1 (TGF-β1)[18]. Moreover, Hasenfuss et al. reported similar findings in Fra-1 knockout and in Fra-1 hepatocyte-specific and general overexpressing mice models. Further, they reported that Fra-1 induced the Opn gene and inhibited the anti-fibrotic gene, cxcl9[19]. According to these observations, hepatocyte-specific Fra-1 expression is insufficient to trigger the disease, suggesting that the involvement of NPLCs is also essential for developing and progressing liver fibrosis. The same authors later found that Fra-1/2 can also prevent and reverse MASLD progression through the dimer it establishes with AP-1 components. The Peroxisome proliferator-activated receptor γ (PPAR-γ) is a relevant component in the pathogenesis of MASLD[20]; in fact, its gene, Pparg2, is directly modulated by AP-1, but interestingly, only c-Fos/c-Jun dimers activate it, while Fra/c-Jun dimers repress it[21]. According to Hasenfuss et al., Fra/c-Jun may interact with AP-1 co-repressors such as Sirt1 and histone deacetylase 3 to inhibit Pparg2 promoter activity[21]. However, the precise context in which different Fos-related components either inhibit or promote the progression of chronic liver disease remains to be explored.
AP-1 activity is closely related to the c-Jun NH2-terminal kinases (JNKs), components of the msitogen-activated protein kinase (MAPK) signaling pathway that activate AP-1 through c-Jun phosphorylation. The involvement of JNKs in tumor development has been extensively studied and they are also implicated in the development of obesity, insulin resistance, MASLD, and MASH[22]. Schattenberg et al. found that JNK1, c-Jun, and AP-1 are overactive in parallel to the development of steatohepatitis in MCD-fed mice[23]. There is evidence of a positive feedback process between phosphorylated c-Jun and JNK1 signaling, which promotes AP-1 overactivity and contributes to MASH progression[24]. Indeed, Videla et al. reported a marked increase in DNA binding of AP-1 and hepatic NF-κB in obese patients with MASH[10].
Active JNK and c-Jun are critical effectors in Ras-induced malignant cell transformation and tumorigenesis[25, 26]. Ras is a well-studied oncogene involved in developing HCC, associated with poor prognosis of patients diagnosed with this cancer[27]. Additionally, JNKs play a role in regulating liver damage through mechanisms such as lipid accumulation, insulin resistance, and fibrosis development via activation of hepatic stellate cells (HSCs). They are also implicated in the development of steatohepatitis[28,29].
In addition, under conditions of hepatic ferroptosis, fibrogenesis is promoted through the activation of HSCs by a mechanism dependent on AP-1 activation[30]. In HSCs, tissue inhibitor of metalloprotease-1 (TIMP1) is a critical component that regulates extracellular matrix (ECM) synthesis and degradation, and its overexpression has been linked to liver disease progression[31]. Xu et al. demonstrated that AP-1 mediates TIMP1 expression in HSCs and that IL-32 expression is increased in liver tissues with fibrosis and may activate AP-1[32].
Endoplasmic reticulum stress (ER stress) has been extensively studied, revealing that its persistence in the liver under abnormal conditions can induce inflammatory responses and cell death. It stands as a critical factor in the development and progression of chronic liver disease[33,34]. Chronic ER stress-triggered inflammatory processes are mediated through IRE1α, which activates NF-κB and AP-1 signaling via IκB kinase (IKK) and JNK1, respectively[35]. In fact, Olivares et al. discovered that ER stress activates the hepatic AP-1 complex through MAPK-dependent signaling pathways[36]. Thus, this context would provide another molecular mechanism by which AP-1 contributes to the progression of chronic liver disease. Additionally, elevated levels of hyperuricemia (HUA) are frequently detected both in MASLD and through the progression of the disease.
Hepatitis, a condition characterized by liver inflammation, can manifest due to multiple etiologies, with viral infections being preeminent and part of the risk factors for chronic liver disease. AP-1 activity has been implicated in viral infections and linked to liver disease progression[37], as discussed below.
The interplay between hepatitis viruses and AP-1
Several molecular mechanisms have linked hepatitis B and C viruses (HBV and HCV, respectively) to AP-1 activation [Figure 1]. Kato et al. demonstrated that among several HBV- and HCV-proteins, the HCV core had the strongest influence on activating AP-1 in liver-derived cell lines[38]. This observation was consistent with later findings by Trierweiler et al., who showed that c-Jun expression was strongly induced during liver carcinogenesis in transgenic mice expressing all HBV envelope proteins (HBV+), suggesting that c-Jun may promote cell proliferation and tumor progression. In addition, they also observed that c-Jun regulates hepatic expression of Opn, which correlates with histological tumor grading, tumor stage and early recurrence after surgical resection, particularly in HBV-infected patients[39]. Tsutsumi et al. found that AP-1 is one of the main proteins activated in constitutively expressing HCV core protein transgenic mice, proposing that its activity may be part of a mechanism favoring HCV-induced hepatocarcinogenesis[40]. Actually, in an established mouse model of HBV-related HCC, c-Jun expression was found to be specifically induced at various times during carcinogenesis. The HBV(+) mice lacking c-Jun displayed fewer premalignant tumors and modified some other cancer-promoting events, suggesting that c-Jun has important roles during HBV-associated tumorigenesis[38]. Furthermore, Ren et al. found that Sirt1, a class III histone deacetylase, enhanced HBV activity by targeting the AP-1 transcription factor[41]. Another study described that this event may occur through the interaction of HBV X protein (HBx) and Jab1 (Jun activation domain-binding protein 1)[42], also through the interaction of HBx with SMYD3[43], and by upregulation of MEKK2 signaling[44]. It has even been reported that during HBV-induced ER stress, AP-1 activity is promoted through the activation of CREBH (cAMP-responsive element-binding protein H), a transcription factor inducing specific genes involved in lipid and glucose metabolism[45-46]. Likewise, another study showed that AP-1 increases the transcription of MANF, an ER stress-inducible protein involved in inflammatory diseases. Interestingly, MANF is strongly expressed in liver tissues of HBV patients compared to normal liver tissues, which has been confirmed in animal models[47].
The reactive oxygen species (ROS)/JNK pathway contributes to the development of different liver diseases, as well as the formation of malignant tumors. However, since JNK has different downstream targets, it is possible that it modulates the activity of components such as JunB, as suggested by Deng et al.[48]. Findings from this group showed that ROS/JNK/JunB signaling leads to inhibition of HCV replication[49], providing a relevant mechanism involved in the development of HCC by HCV infection; thus, JunB phosphorylation targeting could be an interesting pharmacological strategy to treat this condition.
Unfortunately, when chronic liver injury is not treated on time, the risk of developing liver cancer is high. In this sense, AP-1 activity plays a significant role and is involved in several hallmarks of cancer, even during the later stages of tumor establishment.
Aberrant AP-1 activity plays a key role in liver damage processes, such as metabolic diseases and hepatitis B and C virus infections, the main risk factors in the development of HCC, prior to tumor establishment.
AP-1 AND CANCER HALLMARKS IN HCC
According to Hanahan and Weinberg, the development of a malignant tumor requires that human cells exhibit certain acquired biological features (Hallmarks of Cancer) that are indispensable in the transformation from their “healthy” to their “malignant” state[50,51]. Initially, they proposed six key capabilities during tumor development; however, the list of hallmarks has been updated and novel features are emerging[50]. AP-1 transcriptional activity has been implicated in several processes during liver carcinogenesis [Table 1 and Figure 2], and is closely associated with some hallmarks of cancer as discussed below.
Figure 2. AP-1 participation in hepatocellular carcinoma progression. Abnormal AP-1 activity is involved in the early stages of liver cancer and plays a relevant role during its progression. AP-1 enhances key processes in liver carcinogenesis in the hepatic cells and the tumor microenvironment, promoting drug resistance. Black arrows represent targets downstream of AP-1; colored arrows represent upstream components that activate AP-1. Figure created in Biorender.com. AP-1: Activator protein 1; JNK: c-Jun NH2-terminal kinase; EGF: epidermal growth factor; TGF-β: transforming growth factor-β; YAP: yes-associated protein; TAZ: WW-domain-containing transcription regulator 1; PD-1: programmed cell death protein 1; PD-L1: programmed cell death protein-ligand 1; ER: endoplasmic reticulum; MMPs: matrix metallopeptidases; VEGF: vascular endothelial growth factor.
Processes and pathways regulated by AP-1, promoting HCC development
Hallmark of cancer | Target involved in HCC | Ref. |
Sustaining proliferative signaling and evading growth suppressors | p53, p21, p38, Nogo-B, cyclin D, Myc | [5,8,54-57,58-61,68,72] |
Resisting cell death and apoptosis | Bcl-2, Bcl-XL | [73,74] |
Tumor-promoting inflammation | MMPs, COX-2, IFN- | [6,76-78] |
Avoiding immune destruction | TITRs, PD-1, PD-L1 | [80,82-86] |
Activating invasion and metastasis | MMPs, KITENIN, KRT19, miR-21, BORIS, ASIC1 | [88-93,94-102] |
Inducing or accessing vasculature | VEGF, Nogo-B, HLJ1 | [68,103,105-109] |
Senescence | BMAL, FOXF1 | [112,113] |
Sustaining proliferative signaling and evading growth suppressors
AP-1 has been described as a cellular life-and-death decision-maker[52]. In normal conditions, c-Jun/AP-1 participates in liver regeneration by regulating hepatocyte proliferation via a p53/p21-dependent mechanism[5,53]. However, deregulation of AP-1 activity contributes to tumor growth by significantly increasing cell proliferation.
Eferl et al. investigated the participation of the AP-1 protein in liver carcinogenesis. Their work provided compelling evidence that c-Jun serves as a pivotal factor driving the growth of liver tumors in murine models, primarily through the inhibition of p53. Notably, their observations led them to an intriguing conclusion: the involvement of c-Jun appears to be confined to the initial phases of liver cancer development[8].
Explaining the function of AP-1 as a promoter of carcinogenesis has been a topic of interest for several years, and diverse molecular mechanisms have been proposed. For example, the yes-associated protein (YAP) and WW-domain-containing transcription regulator 1 (WWTR1; also known as and hereafter referred to as TAZ) are transcriptional coactivators that regulate cell growth and organ size, but their hyperactivation enhances tumorigenesis and hypertrophy; YAP/TAZ signaling has been characterized in different human cancers, including HCC[54,55]. YAP/TAZ cooperates with AP-1 to promote cell proliferation, but its deregulation promotes tumor growth[56]. Koo et al. inhibited AP-1 activity in human cancer cell lines and observed that YAP-induced cell growth was suppressed, and the hepatomegaly induced by YAP overexpression in mice was also blocked[57].
AP-1 contains binding sites to the promoter of the CycD1 gene, thereby regulating the expression of this cell cycle regulatory protein[58]. CycD1 is an important modulator of cell proliferation and is involved in tissue restoration in the liver after partial hepatectomy[59]. Nevertheless, under disease conditions, CycD1 activity is exacerbated and uncontrolled; thus, it is considered an oncogene in HCC[60]. Actually, CycD1 overexpression is associated with poor prognosis in patients diagnosed with this cancer[61].
Interestingly, the epidermal growth factor (EGF) can induce the expression of AKR1B10 (aldo-keto reductase 1B10)[62], an overexpressed enzyme in liver cancer that has an important task in tumor development and progression through AP-1 signaling[63,64]. Geng et al. reported an interesting finding whereby the combination of sorafenib with epalrestat (an inhibitor targeting AKR1B10) inhibited HepG2 cell proliferation. Furthermore, this combined treatment arrested the cell cycle at the G0/G1 phase[65].
In addition, Li et al. reported that Mucin-1 (MUC1) induces the secretion of the TGF-β through JNK/AP-1 signaling[66]. It is well known that, depending on the context, TGF-β may exhibit either oncogenic or tumor suppressive activities: during early stages of liver cancer, it participates as a tumor suppressor, but during late stages, it contributes to tumor progression[67]. In this manner, AP-1 could favor cell progression through the expression of TGF-β during the late stages of HCC.
Han et al. showed that Nogo-B is one of the AP-1 targeted genes[68]. Nogo-B codes for an endoplasmic reticulum-residential protein involved in a variety of diseases[69]. Its receptor (NgBR) is upregulated in patients with HCC and associated with poor disease prognosis[70]. Overexpression of NgBR contributes to cell proliferation of human HCC cells both in vivo and in vitro, and its knockdown exerts the opposite effect[71].
Recently, Bakiri et al. investigated a novel mechanism of liver tumorigenesis involving the activation of the c-Jun/Fra-2/Myc pathway leading to exacerbated proliferation of hepatocytes[72], which is another potentially useful drug target for developing pharmacological strategies against HCC. Thus, AP-1 represents another potential indirect pathway promoting HCC.
Resisting cell death and apoptosis
The role of AP-1 in modulating apoptosis depends on the cellular context. Shaulian and Karin described a model to explain the function of AP-1 in cell death and survival. They suggested that this factor can regulate the expression of pro- and anti-apoptotic genes, and that its persistent activation promotes cell proliferation and survival[3]. When cells deal with stress, damage, or external signals that normally trigger apoptosis, AP-1 can promote cell survival by positively regulating anti-apoptotic genes. This regulation may include genes encoding proteins such as Bcl-2 and inhibitors of apoptosis proteins (IAPs), which prevent the activation of caspases and other factors involved in the apoptotic cascade[73]. In addition, Takeuchi et al. investigated the role of EGF in cell survival of human gastric adenocarcinoma cells. They found that EGF transmits an anti-apoptotic signal through the activation of AP-1, as the expression of c-Fos and c-Jun increased in response to EGF. The expression of the Bcl-XL protein was also increased. This protein is a member of the anti-apoptotic Bcl-2 family whose expression is known to be regulated by AP-1[74]. In accordance, Eferl et al. found that AP-1 antagonizes apoptosis, since inhibition of c-Jun in an in vivo model of chemically-induced HCC resulted in inhibition of tumor development, which in turn correlated with increased levels of p53 and the NOXOI gene, a process that promoted apoptosis[8].
Tumor-promoting inflammation
AP-1 has a significant impact on the regulation of inflammation. It acts as a central coordinator, influencing the expression of genes associated with the inflammatory response[75]. AP-1 activation leads to the production of pro-inflammatory cytokines, including matrix metallopeptidases (MMPs) and cyclooxygenase 2 (COX-2), as well as other molecules that contribute to the recruitment of immune cells and the amplification of the inflammatory response[6,76]. Understanding the intricate interplay between AP-1 and inflammation in the liver holds promise for developing targeted therapies aimed at modulating the inflammatory response and mitigating the progression of liver diseases[77].
Thomsen et al. demonstrated that JunB plays an essential role in modulating the immune response in the liver. They observed that this component of AP-1 is expressed in liver samples from patients and mice with hepatitis. Additionally, using animal models of liver inflammation, they reported that JunB/AP-1 regulates the expression of IFN-γ (an important cytokine during the immune response) in NK and NKT immune cells during acute hepatitis[78]. The same group demonstrated that c-Jun/AP-1 is strongly expressed in the liver of patients with acute liver inflammation, and that it contributes to cell survival during liver injury. They also observed that this effect is caused by regulating nitric oxide synthase 2/nitric oxide (NOS2/NO) activity, which are transcriptional targets of AP-1[79].
Avoiding immune destruction
Recently, Zhuo et al. showed that AP-1 family motifs are enriched in the enhancer regions of tumor-infiltrating Treg cells (TITR), and that AP-1 signaling is required to activate these cells, a mechanism that hinders effective antitumor immunity[80]. They suggested developing new therapeutic strategies targeting TITRs, taking advantage of AP-1 signaling.
The use of immune checkpoint inhibitors (ICIs) has gained importance in recent years as a therapeutic indication in different malignancies including liver cancer, due to the observed benefit in clinical studies[81]. In fact, inhibitors of the programmed cell death protein 1 (PD-1) and its ligand (PD-L1), or cytotoxic
Activating invasion and metastasis
Hsiang et al. reported for the first time that acetaldehyde from alcohol consumption could enhance AP-1 activity, thereby promoting the invasion of hepatocarcinoma cells through overexpression of matrix metalloproteinase-9 (MMP-9)[88]. This proteinase is overexpressed in tumor tissues of HCC patients. Its overactivity has a strong association with invasion and metastasis, and has also been related to vascular invasion[89,90]. Different research groups have studied AP-1-mediated MMP-9 expression and its involvement in cell invasion. Pan et al., and later, Lee et al., observed that AP-1 activity induces MMP-9 gene transcription, promoting the invasive phenotype of HepG2 cells[91,92]. Another significant contribution is the study by Yu and Lee, who observed that hydroquinone, an environmental carcinogen, increased AP-1 activity, achieving a strong induction of MMP-9 expression and further invasion capacity of HCC cells[93]. Furthermore, Cho et al. reported the involvement of KAI1 COOH-terminal interacting tetraspanin (KITENIN) in HCC migration and invasion, concluding that this component is associated with the activation of AP-1 target genes that promote the expression of MMP-1 and MMP-3[94]. In this respect, Sugioka et al., and later, Yoon et al., confirmed that AP-1 activity is necessary to induce MMP-1 expression in human HCC cells[95,96], which is associated with poor prognosis disease, tumor invasion and metastasis[97].
On the other hand, Rhee et al. found in HCC cells that AP-1 induces the expression of keratin 19 (KRT19), a marker of poor prognosis in this cancer, associated with an invasive phenotype[98].
Additionally, a study by Zhu et al. demonstrated that microRNA-21 (miR-21) activity is associated with tumor migration and invasion ability in HCC, and, interestingly, AP-1 could directly activate its transcription[99]. In this regard, Liu et al. reported that miR-101 prevents AP-1 overactivation, inhibiting
Xian et al. suggested that AP-1 binds to the Brother of the Regulator of Imprinted Sites (BORIS), a DNA-binding protein involved in tumorigenesis and a specific indicator of HCC. According to these authors, such interaction increases BORIS expression in liver tumor tissue and promotes metastatic features[101]. Finally, Liu et al. recently found that the acid-sensing ion channel 1 (ASIC1) promotes migration and invasion in HCC models in vitro and in vivo through the activation of the cFos/cJun heterodimer[102].
Inducing or accessing vasculature
AP-1 directly modulates angiogenesis; for example, in the peritoneal mesothelium, TGF-β1, IL-1β, and TNF-α act through c-Fos to upregulate vascular endothelial growth factor (VEGF) production[103]. In hepatoma cell lines, Ji et al. demonstrated that, as expected, decreasing c-Fos expression reduces AP-1 transcriptional activity, leading to a diminishing of vascular endothelial growth factor-D (VEGFD) expression, which contributes to moderate angiogenesis[104].
Nogo-B is not only involved in tumor cell proliferation, but is also considered a novel regulator of tumor angiogenesis in several types of cancer, especially in HCC[105,106]. There is evidence suggesting that in the tumor microenvironment, AP-1 may activate the transcription of VEGF and Nogo-B, promoting tumor angiogenesis. According to Han et al., under hypoxia conditions, Nogo-B expression in HCC tissues and cell lines is significantly enhanced, and such overexpression is induced by c-Fos. Through deletion and mutation analysis of the predicted activator protein‐1 binding sites, Han et al. found that the functional element mediates the induction of Nogo-B promoter activity[68].
Conversely, AP-1 may also be an inhibitor of angiogenesis through its JunB component, which, as previously mentioned, exhibits tumor suppressor activity. The HLJ1 heat shock protein has been identified as a tumor suppressor in several types of cancer[107,108]. Wang et al. focused on elucidating its activation mechanism and found that the AP-1 binding site on the HLJ1 gene is essential for promoting its expression, and confirmed that FosB, JunB, and JunD bind to this site[109]. Nevertheless, the precise determinants that dictate whether AP-1 exhibits pro-angiogenic or anti-angiogenic activity within a cell remain to be fully elucidated. Clearly, additional research in this domain is warranted.
Senescence
Several data suggest that AP-1 is a key regulator of cellular senescence[110,111]. Jachim et al. found that BMAL, a transcription factor and master regulator of the molecular circadian clock, modulates cellular senescence via AP-1 transcriptional activity[112].
Interestingly, Han et al. identified that AP-1 is a major regulator of the transcriptional program activated upon oncogenic stress, and identified that the expression of FOXF1 (one of its targeted genes) plays a relevant role in the regulation of the senescence phenotype[113]. In their study, Martínez-Zamudio et al. highlighted that AP-1 is essential for the expression of senescence-associated secretory phenotype genes (SASP), and they consider it a relevant therapeutic for the modulation of senescence in age-related diseases and cancer[110]. Moreover, during colon carcinogenesis, AP-1-dependent transcription is active in the initiation and maintenance phases of senescence[114].
In vitro treatment of human HCC cells with rapamycin and 5-fluorouracil induced apoptosis and cellular senescence[115]. However, the authors reported that the senescent phenotype was accompanied by negative regulation of AP-1 and NF-κB transcriptional activity, a contradictory finding to the above discussed. Hence, research on the role of the AP-1 family in the modulation of cellular senescence in malignant conditions is controversial. A study published in 2006 showed that both c-Jun and JunB are involved in chemotherapy-induced cellular senescence[116]; however, it remains to be elucidated in which context AP-1 components act as inducers or repressors of cellular senescence, and whether this activity could be harnessed as a therapeutic target to manage HCC.
The normal activity of AP-1 is involved in different cellular processes that lead to optimal cell function; however, deregulation of this transcription factor leads to the activation of essential molecular processes in liver carcinogenesis known as cancer hallmarks, which establish key features of malignant cell transformation. Thus, inhibiting AP-1 constitutes a reasonable therapeutic strategy for the treatment of liver tumors.
POTENTIAL CLINICAL VALUE OF AP-1 IN LIVER CANCER: EARLY DIAGNOSIS, PROGNOSIS, AND DRUG RESISTANCE
Liu et al. were pioneers in detecting the presence of AP-1 in tumor tissues of patients diagnosed with HCC. Their study revealed that this transcription factor is upregulated in more than 50% of the cases studied during the early stage of liver cancer development. Interestingly, they observed only weak activation of AP-1 in metastatic tumors, suggesting that the regulation of non-hepatocyte-derived tumors within the liver likely involves distinct mechanisms[117]. Chen et al. evaluated the expression of the Jun dimerization protein 2 (JDP2), an AP-1 repressor protein known to play a significant role in liver cancer development[118], in tissue samples from HCC patients. Their findings showed a strong correlation between high JDP2 expression and smaller tumor size and early-stage HCC. This association suggests that elevated JDP2 expression improves patient survival and may serve as a valuable prognostic biomarker[119].
On the other hand, AP-1 activity has been implicated in conferring resistance to various chemotherapeutic drugs, and Daschner et al. reported that AP-1 provides chemo-resistance to breast cancer cells[120].
In liver cancer, it has been estimated that only 30% of patients benefit from pharmacological treatment with sorafenib, and most of these responders will develop drug resistance within six months[122]. Consequently, understanding the molecular mechanisms underlying either poor or no sensitivity to chemotherapy has become a major issue in cancer research[123]. For instance, Cheng et al. reported that inhibiting IRAK1 (interleukin-1 receptor-associated kinase 1), a mediator of Toll-like receptor (TLR) and interleukin-1 receptor (IL-1R) signaling, and a regulator of liver tumor-initiating cell (TIC) functions, can suppress liver TIC populations and mitigate sorafenib resistance in HCC cells. They propose that the IRAK4/IRAK1/
Taken together, it is conceivable to consider AP-1 as an early and prognostic marker for liver cancer, as well as a therapeutic target and an adjuvant to overcome drug resistance in clinical settings.
AP-1 inhibitors
Liver cancer ranks sixth in incidence and second in mortality worldwide, with a mortality:incidence ratio of 89.9% (The Global Cancer Observatory). As discussed above, AP-1 is a potential therapeutic target for therapy against HCC, so developing drugs to block its activity is a useful strategy in the search for new targeted therapies. Recently, Song et al. reviewed AP-1-targeted small molecule inhibitors that have been tested and have demonstrated some anticancer effects[126]. For example, the small-molecule c-Fos/AP-1 inhibitor (T-5224) was shown to inhibit the invasion and migration of head and neck squamous cell carcinoma cells[127]. On the other hand, the selective AP-1 inhibitor XR5944 inhibited head and neck squamous cell carcinoma (HNSCC) invasion and migration in vitro and in vivo[127]. Interestingly,
In a Setd2-deficient mice model, Li et al. found that liver depletion of setd2-induced c-Jun/AP-1 activation and high-fat diet enhanced lipid accumulation and promoted the development of HCC[131]. Developing pharmacological strategies targeting the modulation of upstream components in the AP-1 signaling pathway is key to the discovery of new drugs.
Due to its inhibitory role of AP-1 signaling, the anti-inflammatory property of curcumin was evaluated in patients with atopic asthma; however, its oral administration (1,000 mg, twice daily) showed no significant effects (ClinicalTrial ID: NCT01179256). On the other hand, doxycycline, which has an inhibitory effect on JNK1/2 signaling, was tested in patients with symptomatic peripheral arterial disease, finding minimal activation of AP-1 in both the non-progressive and progressive phases of atherosclerosis, respectively[132]. A Phase I study evaluated the pharmacokinetic and toxicity parameters of bis-phenazine XR5944.14, a novel cytotoxic drug with antitumor activity; however, the lack of correlation between toxicity and pharmacokinetic values made it difficult to recommend a dose for further studies in Phase 2 trials[133].
The endogenous bioactive lipid mediators, lipoxins, were recently reported to be involved in processes such as chronic inflammation and cancer through the modulation of transcription factors like AP-1[134]. Research and development of small molecules targeted to modulate the activity of these mediators could represent a novel option for targeting cancer. Since the MyD88 signaling pathway triggers the activation of transcription factors such as NF-κB and AP-1 to promote the release of immune and inflammatory mediators that promote the development of various diseases including cancer, Liu et al. suggest that targeting MyD88 through the use of ST2825 inhibitor may be a possible therapeutic strategy to modulate the regulation of inflammatory diseases[135].
CONCLUSIONS AND PERSPECTIVES
While the impact of AP-1 on gene expression varies depending on the cellular context, there is sufficient evidence to postulate this transcription factor as a key contributor to the carcinogenesis of several types of cancer, including HCC. Initially, several research groups suggested that the involvement of the AP-1 family was confined to the early stages of liver cancer[8,11,107]. However, increasing evidence indicates that abnormal AP-1 activity not only occurs as an early event during HCC development, but also plays an important role in HCC progression during later stages when the tumor is already established[136]. Liver carcinogenesis studies such as the DEN animal model could be useful to evaluate if there is differential expression of AP-1 subunits during the distinct stages of chronic liver disease.
It is important to point out that Columbano et al. reported that the participation of c-Jun is not required to increase hepatocyte proliferation in the presence of carcinogen 1,4-Bis [2-(3,5-Dichloropyridyloxy)] benzene (TCPOBOP)[137]. This interesting observation may be explained by the different proliferative responses independent of AP-1 activation. However, whether this transcription factor has a relevant contribution in the late stages of HCC, in which a large percentage of patients are diagnosed, remains to be elucidated[138]. Understanding the participation of AP-1 in these stages could pave the way for AP-1-targeted treatments that would benefit these patients. It would be interesting to perform polymerase chain reaction (PCR) assays on tumoral tissues from patients diagnosed during advanced stages of HCC to determine their expression levels.
Developing drugs that effectively inhibit AP-1 is challenging but crucial to improving liver disease therapy; however, there are some limitations. Since AP-1 is a critical transcription factor that regulates gene expression in healthy cells, it is necessary to investigate and propose small molecules targeting AP-1 subunits responsible for hepatic carcinogenesis. In addition, considering that AP-1 exhibits either oncogenic or anti-oncogenic activities depending on the conforming dimer, further research is needed to reveal which conditions favor the conformation of one AP-1 dimer or another. Additionally, in silico tools may prove valuable in identifying repurposed drugs capable of achieving this objective. Molecules with proven activity against AP-1 should be thoroughly investigated as potential candidates for cancer therapeutics[1-2,126].
Drug combination is a therapeutic approach that has brought many benefits as a modality against various diseases, especially cancer. It is noteworthy to propose drug combinations modulating the activity of AP-1 as the basis of translational studies, since there are no clinical trials evaluating the activity of drugs targeting this transcription factor. Recently, the use of gene editing strategies such as CRISPR-Cas9 has generated many benefits in research and represents a wide field of application. For instance, Xu et al. demonstrated that this tool could be useful in providing drug combination options[139]. Therefore, it may also be applied to identify drugs that can be combined with AP-1 inhibitors. Moreover, since AP-1 is also able to modulate the immune response, blocking its activity could also be a promising component in immunotherapy. Additionally, the recent reclassification of steatotic liver disease, which reflects clinical practice and prognosis, should help to improve diagnosis and treatment[140].
In conclusion, AP-1 represents a powerful tool as an early marker of liver damage, as well as an HCC prognostic marker and therapeutic target. The potential use of AP-1 in clinical settings may benefit many patients, from preventing precancer liver disease to drug resistance in HCC patients.
DECLARATIONS
Acknowledgments
Villarruel-Melquiades F received the CONAHCyT fellowship (number 787907) to support her PhD studies.
Author contributions
Wrote the first draft of the manuscript: Villarruel-Melquiades F
Wrote the paper and made substantial contributions to the conception of the manuscript: Villarruel-Melquiades F, Aguirre‐Maldonado I, Herrera‐López EE
Made critical revisions related to the important intellectual content of the manuscript: Camacho J, Mendoza-Garrido ME, Pérez-Carreón JI
All authors read and approved the final version of the article to be published.
Availability of data and materials
Not applicable.
Financial support and sponsorship
None.
Conflicts of interest
All authors declared that there are no conflicts of interest.
Ethical approval and consent to participate
Not applicable.
Consent for publication
Not applicable.
Copyright
© The Author(s) 2024.
REFERENCES
1. Ye N, Ding Y, Wild C, Shen Q, Zhou J. Small molecule inhibitors targeting activator protein 1 (AP-1). J Med Chem. 2014;57:6930-48.
2. Manning AM, Davis RJ. Targeting JNK for therapeutic benefit: from junk to gold? Nat Rev Drug Discov. 2003;2:554-65.
4. Bejjani F, Evanno E, Zibara K, Piechaczyk M, Jariel-Encontre I. The AP-1 transcriptional complex: local switch or remote command? Biochim Biophys Acta Rev Cancer. 2019;1872:11-23.
5. Brennan A, Leech JT, Kad NM, Mason JM. Selective antagonism of cJun for cancer therapy. J Exp Clin Cancer Res. 2020;39:184.
6. Bhosale PB, Kim HH, Abusaliya A, et al. Structural and functional properties of activator protein-1 in cancer and inflammation. Evid Based Complement Alternat Med. 2022;2022:9797929.
8. Eferl R, Ricci R, Kenner L, et al. Liver tumor development. c-Jun antagonizes the proapoptotic activity of p53. Cell. 2003;112:181-92.
9. Schulien I, Hockenjos B, Schmitt-Graeff A, et al. The transcription factor c-Jun/AP-1 promotes liver fibrosis during non-alcoholic steatohepatitis by regulating Osteopontin expression. Cell Death Differ. 2019;26:1688-99.
10. Videla LA, Tapia G, Rodrigo R, et al. Liver NF-kappaB and AP-1 DNA binding in obese patients. Obesity. 2009;17:973-9.
11. Min L, Ji Y, Bakiri L, et al. Liver cancer initiation is controlled by AP-1 through SIRT6-dependent inhibition of survivin. Nat Cell Biol. 2012;14:1203-11.
12. Fiorentino F, Carafa V, Favale G, Altucci L, Mai A, Rotili D. The two-faced role of SIRT6 in cancer. Cancers. 2021;13:1156.
13. Dorn C, Engelmann JC, Saugspier M, et al. Increased expression of c-Jun in nonalcoholic fatty liver disease. Lab Invest. 2014;94:394-408.
14. Lau TYH, Xiao J, Liong EC, Liao L, Leung TM, et al. Hepatic response to chronic hypoxia in experimental rat model through HIF-1 alpha, activator protein-1 and NF-kappa B. Histol Histopatho. 2013;28:463-71.
15. El-Tanani MK, Campbell FC, Kurisetty V, Jin D, McCann M, Rudland PS. The regulation and role of osteopontin in malignant transformation and cancer. Cytokine Growth Factor Rev. 2006;17:463-74.
16. Wai PY, Kuo PC. Osteopontin: regulation in tumor metastasis. Cancer Metastasis Rev. 2008;27:103-18.
17. Syn WK, Choi SS, Liaskou E, et al. Osteopontin is induced by hedgehog pathway activation and promotes fibrosis progression in nonalcoholic steatohepatitis. Hepatology. 2011;53:106-15.
18. Kireva T, Erhardt A, Tiegs G, et al. Transcription factor Fra-1 induces cholangitis and liver fibrosis. Hepatology. 2011;53:1259-69.
19. Hasenfuss SC, Bakiri L, Thomsen MK, Hamacher R, Wagner EF. Activator protein 1 transcription factor Fos-related antigen 1 (Fra-1) is dispensable for murine liver fibrosis, but modulates xenobiotic metabolism. Hepatology. 2014;59:261-73.
20. Chen H, Tan H, Wan J, et al. PPAR-γ signaling in nonalcoholic fatty liver disease: pathogenesis and therapeutic targets. Pharmacol Ther. 2023;245:108391.
21. Hasenfuss SC, Bakiri L, Thomsen MK, Williams EG, Auwerx J, Wagner EF. Regulation of steatohepatitis and PPARγ signaling by distinct AP-1 dimers. Cell Metab. 2014;19:84-95.
22. Wu Q, Wu W, Fu B, Shi L, Wang X, Kuca K. JNK signaling in cancer cell survival. Med Res Rev. 2019;39:2082-104.
23. Schattenberg JM, Singh R, Wang Y, et al. JNK1 but not JNK2 promotes the development of steatohepatitis in mice. Hepatology. 2006;43:163-72.
24. Steensels S, Qiao J, Ersoy BA. Transcriptional regulation in non-alcoholic fatty liver disease. Metabolites. 2020;10:283.
25. Johnson R, Spiegelman B, Hanahan D, Wisdom R. Cellular transformation and malignancy induced by ras require c-jun. Mol Cell Biol. 1996;16:4504-11.
26. Talotta F, Mega T, Bossis G, et al. Heterodimerization with Fra-1 cooperates with the ERK pathway to stabilize c-Jun in response to the RAS oncoprotein. Oncogene. 2010;29:4732-40.
27. Delire B, Stärkel P. The Ras/MAPK pathway and hepatocarcinoma: pathogenesis and therapeutic implications. Eur J Clin Invest. 2015;45:609-23.
28. Singh R, Wang Y, Xiang Y, Tanaka KE, Gaarde WA, Czaja MJ. Differential effects of JNK1 and JNK2 inhibition on murine steatohepatitis and insulin resistance. Hepatology. 2009;49:87-96.
29. Czaja MJ. JNK regulation of hepatic manifestations of the metabolic syndrome. Trends Endocrinol Metab. 2010;21:707-13.
30. Cho SS, Yang JH, Lee JH, et al. Ferroptosis contribute to hepatic stellate cell activation and liver fibrogenesis. Free Radic Biol Med. 2022;193:620-37.
31. Roeb E. Matrix metalloproteinases and liver fibrosis (translational aspects). Matrix Biol. 2018;68-69:463-73.
32. Xu H, Zhang S, Pan X, et al. TIMP-1 expression induced by IL-32 is mediated through activation of AP-1 signal pathway. Int Immunopharmacol. 2016;38:233-7.
33. Lebeaupin C, Vallée D, Hazari Y, Hetz C, Chevet E, Bailly-Maitre B. Endoplasmic reticulum stress signalling and the pathogenesis of non-alcoholic fatty liver disease. J Hepatol. 2018;69:927-47.
34. Zhang J, Guo J, Yang N, Huang Y, Hu T, Rao C. Endoplasmic reticulum stress-mediated cell death in liver injury. Cell Death Dis. 2022;13:1051.
35. Sharma RS, Harrison DJ, Kisielewski D, et al. Experimental nonalcoholic steatohepatitis and liver fibrosis are ameliorated by pharmacologic activation of Nrf2 (NF-E2 p45-related factor 2). Cell Mol Gastroenterol Hepatol. 2018;5:367-98.
36. Olivares S, Green RM, Henkel AS. Endoplasmic reticulum stress activates the hepatic activator protein 1 complex via mitogen activated protein kinase-dependent signaling pathways. PLoS One. 2014;9:e103828.
37. Zahra M, Azzazy H, Moustafa A. Transcriptional regulatory networks in hepatitis C virus-induced hepatocellular carcinoma. Sci Rep. 2018;8:14234.
38. Kato N, Yoshida H, Ono-Nita SK, et al. Activation of intracellular signaling by hepatitis B and C viruses: C-viral core is the most potent signal inducer. Hepatology. 2000;32:405-12.
39. Trierweiler C, Hockenjos B, Zatloukal K, et al. The transcription factor c-JUN/AP-1 promotes HBV-related liver tumorigenesis in mice. Cell Death Differ. 2016;23:576-82.
40. Tsutsumi T, Suzuki T, Moriya K, et al. Alteration of intrahepatic cytokine expression and AP-1 activation in transgenic mice expressing hepatitis C virus core protein. Virology. 2002;304:415-24.
41. Ren JH, Tao Y, Zhang ZZ, et al. Sirtuin 1 regulates hepatitis B virus transcription and replication by targeting transcription factor AP-1. J Virol. 2014;88:2442-51.
42. Tanaka Y, Kanai F, Ichimura T, et al. The hepatitis B virus X protein enhances AP-1 activation through interaction with Jab1. Oncogene. 2006;25:633-42.
43. Hayashi M, Deng L, Chen M, et al. Interaction of the hepatitis B virus X protein with the lysine methyltransferase SET and MYND domain-containing 3 induces activator protein 1 activation. Microbiol Immunol. 2016;60:17-25.
44. Kong GY, Zhang JP, Zhang S, Shan CL, Ye LH, Zhang XD. Hepatitis B virus X protein promotes hepatoma cell proliferation via upregulation of MEKK2. Acta Pharmacol Sin. 2011;32:1173-80.
45. Cho HK, Kim SY, Kyaw YY, et al. HBx induces the proliferation of hepatocellular carcinoma cells via AP1 over-expressed as a result of ER stress. Biochem J. 2015;466:115-21.
46. Junli Z, Shuhan W, Yajuan Z, Xiaoling D, Jiahuan L, Keshu X. The role and mechanism of CREBH regulating SIRT3 in metabolic associated fatty liver disease. Life Sci. 2022;306:120838.
47. Wang CH, Jiang TC, Qiang WM, et al. Activator protein-1 is a novel regulator of mesencephalic astrocyte-derived neurotrophic factor transcription. Mol Med Rep. 2018;18:5765-74.
48. Deng L, Liang Y, Ariffianto A, et al. Hepatitis C virus-induced ROS/JNK signaling pathway activates the E3 ubiquitin ligase itch to promote the release of HCV particles via polyubiquitylation of VPS4A. J Virol. 2022;96:e0181121.
49. Ariffianto A, Deng L, Harada S, Liang Y, Matsui C, et al. Transcription factor JunB suppresses hepatitis C virus replication. Kobe J Med Sci. 2023;69:E86-95.
52. Ameyar M, Wisniewska M, Weitzman JB. A role for AP-1 in apoptosis: the case for and against. Biochimie. 2003;85:747-52.
53. Stepniak E, Ricci R, Eferl R, et al. C-Jun/AP-1 controls liver regeneration by repressing p53/p21 and p38 MAPK activity. Gene Dev. 2006;20:2306-14.
54. Moya IM, Halder G. Hippo-YAP/TAZ signalling in organ regeneration and regenerative medicine. Nat Rev Mol Cell Biol. 2019;20:211-26.
55. Zhang S, Zhou D. Role of the transcriptional coactivators YAP/TAZ in liver cancer. Curr Opin Cell Biol. 2019;61:64-71.
56. Zanconato F, Forcato M, Battilana G, et al. Genome-wide association between YAP/TAZ/TEAD and AP-1 at enhancers drives oncogenic growth. Nat Cell Biol. 2015;17:1218-27.
57. Koo JH, Plouffe SW, Meng Z, et al. Induction of AP-1 by YAP/TAZ contributes to cell proliferation and organ growth. Genes Dev. 2020;34:72-86.
58. Pawlonka J, Rak B, Ambroziak U. The regulation of cyclin D promoters - review. Cancer Treat Res Commun. 2021;27:100338.
59. Núñez KG, Gonzalez-Rosario J, Thevenot PT, Cohen AJ. Cyclin D1 in the liver: role of noncanonical signaling in liver steatosis and hormone regulation. Ochsner J. 2017;17:56-65.
60. Deane NG, Parker MA, Aramandla R, et al. Hepatocellular carcinoma results from chronic cyclin D1 overexpression in transgenic mice. Cancer Res. 2001;61:5389-95.
61. Che Y, Ye F, Xu R, et al. Co-expression of XIAP and cyclin D1 complex correlates with a poor prognosis in patients with hepatocellular carcinoma. Am J Pathol. 2012;180:1798-807.
62. Liu Z, Yan R, Al-Salman A, et al. Epidermal growth factor induces tumour marker AKR1B10 expression through activator protein-1 signalling in hepatocellular carcinoma cells. Biochem J. 2012;442:273-82.
63. DiStefano JK, Davis B. Diagnostic and prognostic potential of AKR1B10 in human hepatocellular carcinoma. Cancers. 2019;11:486.
64. Wang YY, Qi LN, Zhong JH, et al. High expression of AKR1B10 predicts low risk of early tumor recurrence in patients with hepatitis B virus-related hepatocellular carcinoma. Sci Rep. 2017;7:42199.
65. Geng N, Jin Y, Li Y, Zhu S, Bai H. AKR1B10 inhibitor epalrestat facilitates sorafenib-induced apoptosis and autophagy via targeting the mTOR pathway in hepatocellular carcinoma. Int J Med Sci. 2020;17:1246-56.
66. Li Q, Liu G, Shao D, et al. Mucin1 mediates autocrine transforming growth factor beta signaling through activating the c-Jun N-terminal kinase/activator protein 1 pathway in human hepatocellular carcinoma cells. Int J Biochem Cell Biol. 2015;59:116-25.
67. Tu S, Huang W, Huang C, Luo Z, Yan X. Contextual regulation of TGF-β signaling in liver cancer. Cells. 2019;8:1235.
68. Han D, Yang P, Qin B, et al. Upregulation of Nogo-B by hypoxia inducible factor-1 and activator protein-1 in hepatocellular carcinoma. Cancer Sci. 2021;112:2728-38.
69. Rao J, Cheng F, Zhou H, et al. Nogo-B is a key mediator of hepatic ischemia and reperfusion injury. Redox Biol. 2020;37:101745.
70. Dong C, Zhao B, Long F, et al. Nogo-B receptor promotes the chemoresistance of human hepatocellular carcinoma via the ubiquitination of p53 protein. Oncotarget. 2016;7:8850-65.
71. Dong C, Liu Y, Jiang K, et al. The Nogo-B receptor promotes human hepatocellular carcinoma cell growth via the Akt signal pathway. J Cell Biochem. 2018;119:7738-46.
72. Bakiri L, Hasenfuss SC, Guío-Carrión A, Thomsen MK, Hasselblatt P, Wagner EF. Liver cancer development driven by the AP-1/c-Jun~Fra-2 dimer through c-Myc. Proc Natl Acad Sci U S A. 2024;121:e2404188121.
73. Maulik N, Goswami S, Galang N, Das DK. Differential regulation of Bcl-2, AP-1 and NF-kappaB on cardiomyocyte apoptosis during myocardial ischemic stress adaptation. FEBS Lett. 1999;443:331-6.
74. Takeuchi K, Motoda Y, Ito F. Role of transcription factor activator protein 1 (AP1) in epidermal growth factor-mediated protection against apoptosis induced by a DNA-damaging agent. FEBS J. 2006;273:3743-55.
75. Qiao Y, He H, Jonsson P, Sinha I, Zhao C, Dahlman-Wright K. AP-1 is a key regulator of proinflammatory cytokine TNFα-mediated triple-negative breast cancer progression. J Biol Chem. 2016;291:18309.
76. Jang J, Hunto ST, Kim JW, Lee HP, Kim HG, Cho JY. Anti-inflammatory activities of an anti-histamine drug, loratadine, by suppressing TAK1 in AP-1 pathway. Int J Mol Sci. 2022;23:3986.
77. Kyriakis JM. Activation of the AP-1 transcription factor by inflammatory cytokines of the TNF family. Gene Exp. 1999;7:217-31.
78. Thomsen MK, Bakiri L, Hasenfuss SC, Hamacher R, Martinez L, Wagner EF. JUNB/AP-1 controls IFN-γ during inflammatory liver disease. J Clin Invest. 2013;123:5258-68.
79. Hasselblatt P, Rath M, Komnenovic V, Zatloukal K, Wagner EF. Hepatocyte survival in acute hepatitis is due to c-Jun/AP-1-dependent expression of inducible nitric oxide synthase. Proc Natl Acad Sci U S A. 2007;104:17105-10.
80. Zhuo B, Zhang Q, Xie T, et al. Integrative epigenetic analysis reveals AP-1 promotes activation of tumor-infiltrating regulatory T cells in HCC. Cell Mol Life Sci. 2023;80:103.
81. Pinter M, Scheiner B, Pinato DJ. Immune checkpoint inhibitors in hepatocellular carcinoma: emerging challenges in clinical practice. Lancet Gastroenterol Hepatol. 2023;8:760-70.
82. Doorn DJ, Takkenberg RB, Klümpen HJ. Immune checkpoint inhibitors in hepatocellular carcinoma: an overview. Pharmaceuticals. 2020;14:3.
83. Venkatraman S, Meller J, Hongeng S, Tohtong R, Chutipongtanate S. Transcriptional regulation of cancer immune checkpoints: emerging strategies for immunotherapy. Vaccines. 2020;8:735.
84. Xiao G, Deng A, Liu H, Ge G, Liu X. Activator protein 1 suppresses antitumor T-cell function via the induction of programmed death 1. Proc Natl Acad Sci U S A. 2012;109:15419-24.
85. You X, Liu F, Binder M, et al. Asxl1 loss cooperates with oncogenic Nras in mice to reprogram the immune microenvironment and drive leukemic transformation. Blood. 2022;139:1066-79.
86. Luo B, Zhang S, Tan D, Yu X, Lin J, Wang M. Anlotinib benefits the αPDL1 immunotherapy by activating ROS/JNK/AP-1 pathway to upregulate PDL1 expression in colorectal cancer. Oxid Med Cell Longev. 2022;2022:8965903.
87. Yu P, Wei H, Li K, et al. The traditional chinese medicine monomer Ailanthone improves the therapeutic efficacy of anti-PD-L1 in melanoma cells by targeting c-Jun. J Exp Clin Cancer Res. 2022;41:346.
88. Hsiang CY, Wu SL, Chen JC, et al. Acetaldehyde induces matrix metalloproteinase-9 gene expression via nuclear factor-kappaB and activator protein 1 signaling pathways in human hepatocellular carcinoma cells: association with the invasive potential. Toxicol Lett. 2007;171:78-86.
89. Arii S, Mise M, Harada T, et al. Overexpression of matrix metalloproteinase 9 gene in hepatocellular carcinoma with invasive potential. Hepatology. 1996;24:316-22.
90. Nart D, Yaman B, Yilmaz F, Zeytunlu M, Karasu Z, Kiliç M. Expression of matrix metalloproteinase-9 in predicting prognosis of hepatocellular carcinoma after liver transplantation. Liver Transpl. 2010;16:621-30.
91. Pan MH, Chiou YS, Chen WJ, Wang JM, Badmaev V, Ho CT. Pterostilbene inhibited tumor invasion via suppressing multiple signal transduction pathways in human hepatocellular carcinoma cells. Carcinogenesis. 2009;30:1234-42.
92. Lee KH, Yeh MH, Kao ST, et al. The inhibitory effect of hesperidin on tumor cell invasiveness occurs via suppression of activator protein 1 and nuclear factor-kappaB in human hepatocellular carcinoma cells. Toxicol Lett. 2010;194:42-9.
93. Yu MH, Lee SO. Hydroquinone stimulates cell invasion through activator protein-1-dependent induction of MMP-9 in HepG2 human hepatoma cells. Food Chem Toxicol. 2016;89:120-5.
94. Cho SB, Park YL, Park SJ, et al. KITENIN is associated with activation of AP-1 target genes via MAPK cascades signaling in human hepatocellular carcinoma progression. Oncol Res. 2011;19:115-23.
95. Sugioka Y, Watanabe T, Inagaki Y, et al. c-Jun NH2-terminal kinase pathway is involved in constitutive matrix metalloproteinase-1 expression in a hepatocellular carcinoma-derived cell line. Int J Cancer. 2004;109:867-74.
96. Yoon JH, Choi YJ, Lee SG. Ginsenoside Rh1 suppresses matrix metalloproteinase-1 expression through inhibition of activator protein-1 and mitogen-activated protein kinase signaling pathway in human hepatocellular carcinoma cells. Eur J Pharmacol. 2012;679:24-33.
97. Geervliet E, Bansal R. Matrix metalloproteinases as potential biomarkers and therapeutic targets in liver diseases. Cells. 2020;9:1212.
98. Rhee H, Kim HY, Choi JH, et al. Keratin 19 expression in hepatocellular carcinoma is regulated by fibroblast-derived HGF via a MET-ERK1/2-AP1 and SP1 axis. Cancer Res. 2018;78:1619-31.
99. Zhu Q, Wang Z, Hu Y, et al. miR-21 promotes migration and invasion by the miR-21-PDCD4-AP-1 feedback loop in human hepatocellular carcinoma. Oncol Rep. 2012;27:1660-8.
100. Liu JJ, Lin XJ, Yang XJ, et al. A novel AP-1/miR-101 regulatory feedback loop and its implication in the migration and invasion of hepatoma cells. Nucleic Acids Res. 2014;42:12041-51.
101. Xian L, Xiong Y, Qin L, et al. Jun/Fos promotes migration and invasion of hepatocellular carcinoma cells by enhancing BORIS promoter activity. Int J Biochem Cell Biol. 2024;169:106540.
102. Liu Y, Wang B, Cheng Y, et al. ASIC1 promotes migration and invasion of hepatocellular carcinoma via the PRKACA/AP-1 signaling pathway. Carcinogenesis. 2024;45:399-408.
103. Catar R, Witowski J, Wagner P, et al. The proto-oncogene c-Fos transcriptionally regulates VEGF production during peritoneal inflammation. Kidney Int. 2013;84:1119-28.
104. Ji L, Zheng Z, Shi L, Huang Y, Lu B, Wang Z. Andrographolide decreased VEGFD expression in hepatoma cancer cells by inducing ubiquitin/proteasome-mediated cFos protein degradation. Biochim Biophys Acta. 2015;1850:750-8.
105. Cai H, Saiyin H, Liu X, et al. Nogo-B promotes tumor angiogenesis and provides a potential therapeutic target in hepatocellular carcinoma. Mol Oncol. 2018;12:2042-54.
106. Li YK, Xie YJ, Wu DC, Long SL, Tang S, Mo ZC. Nogo-B receptor in relevant carcinoma: current achievements, challenges and aims (review). Int J Oncol. 2018;53:1827-35.
107. Acun T, Doberstein N, Habermann JK, et al. HLJ1 (DNAJB4) gene is a novel biomarker candidate in breast cancer. OMICS. 2017;21:257-65.
108. Chen CH, Chang WH, Su KY, et al. HLJ1 is an endogenous Src inhibitor suppressing cancer progression through dual mechanisms. Oncogene. 2016;35:5674-85.
109. Wang CC, Tsai MF, Dai TH, et al. Synergistic activation of the tumor suppressor, HLJ1, by the transcription factors YY1 and activator protein 1. Cancer Res. 2007;67:4816-26.
110. Martínez-Zamudio RI, Roux PF, de Freitas JANLF, et al. AP-1 imprints a reversible transcriptional programme of senescent cells. Nat Cell Biol. 2020;22:842-55.
111. Wang Y, Liu L, Song Y, Yu X, Deng H. Unveiling E2F4, TEAD1 and AP-1 as regulatory transcription factors of the replicative senescence program by multi-omics analysis. Protein Cell. 2022;13:742-59.
112. Jachim SK, Zhong J, Ordog T, et al. BMAL1 modulates senescence programming via AP-1. Aging. 2023;15:9984-10009.
113. Han R, Li L, Ugalde AP, et al. Functional CRISPR screen identifies AP1-associated enhancer regulating FOXF1 to modulate oncogene-induced senescence. Genome Biol. 2018;19:118.
114. Dabrowska M, Uram L, Dabrowski M, Sikora E. Antigen presentation capability and AP-1 activation accompany methotrexate-induced colon cancer cell senescence in the context of aberrant β-catenin signaling. Mech Ageing Dev. 2021;197:111517.
115. Bu X, Le C, Jia F, et al. Synergistic effect of mTOR inhibitor rapamycin and fluorouracil in inducing apoptosis and cell senescence in hepatocarcinoma cells. Cancer Biol Ther. 2008;7:392-6.
116. Yogev O, Anzi S, Inoue K, Shaulian E. Induction of transcriptionally active Jun proteins regulates drug-induced senescence. J Biol Chem. 2006;281:34475-83.
117. Liu P, Kimmoun E, Legrand A, et al. Activation of NF-kappa B, AP-1 and STAT transcription factors is a frequent and early event in human hepatocellular carcinomas. J Hepatol. 2002;37:63-71.
118. Chen YL, Chan SH, Lin PY, Chu PY. The expression of a tumor suppressor gene JDP2 and its prognostic value in hepatocellular carcinoma patients. Hum Pathol. 2017;63:212-6.
119. Bitton-Worms K, Pikarsky E, Aronheim A. The AP-1 repressor protein, JDP2, potentiates hepatocellular carcinoma in mice. Mol Cancer. 2010;9:54.
120. Daschner PJ, Ciolino HP, Plouzek CA, Yeh GC. Increased AP-1 activity in drug resistant human breast cancer MCF-7 cells. Breast Cancer Res Treat. 1999;53:229-40.
121. Wang Y, Wan GH, Wu YM, et al. AP-1 confers resistance to anti-cancer therapy by activating XIAP. Oncotarget. 2018;9:14124-37.
122. Tang W, Chen Z, Zhang W, et al. The mechanisms of sorafenib resistance in hepatocellular carcinoma: theoretical basis and therapeutic aspects. Signal Transduct Target Ther. 2020;5:87.
123. Cabral LKD, Tiribelli C, Sukowati CHC. Sorafenib resistance in hepatocellular carcinoma: the relevance of genetic heterogeneity. Cancers. 2020;12:1576.
124. Cheng BY, Lau EY, Leung HW, et al. IRAK1 augments cancer stemness and drug resistance via the AP-1/AKR1B10 signaling cascade in hepatocellular carcinoma. Cancer Res. 2018;78:2332-42.
125. Yan D, Yan X, Dai X, et al. Activation of AKT/AP1/FoxM1 signaling confers sorafenib resistance to liver cancer cells. Oncol Rep. 2019;42:785-96.
126. Song D, Lian Y, Zhang L. The potential of activator protein 1 (AP-1) in cancer targeted therapy. Front Immunol. 2023;14:1224892.
127. Kamide D, Yamashita T, Araki K, et al. Selective activator protein-1 inhibitor T-5224 prevents lymph node metastasis in an oral cancer model. Cancer Sci. 2016;107:666-73.
128. Fukuda K, Hibiya Y, Mutoh M, Koshiji M, Akao S, Fujiwara H. Inhibition of activator protein 1 activity by berberine in human hepatoma cells. Planta Med. 1999;65:381-3.
129. Tang S, Zhang F, Li J, et al. The selective activator protein-1 inhibitor T-5224 regulates the IRF4/MYC axis and exerts cooperative antimyeloma activity with bortezomib. Chem Biol Interact. 2023;384:110687.
130. Tewari D, Nabavi SF, Nabavi SM, et al. Targeting activator protein 1 signaling pathway by bioactive natural agents: possible therapeutic strategy for cancer prevention and intervention. Pharmacol Res. 2018;128:366-75.
131. Li XJ, Li QL, Ju LG, et al. Deficiency of histone methyltransferase SET domain-containing 2 in liver leads to abnormal lipid metabolism and HCC. Hepatology. 2021;73:1797-815.
132. Meijer CA, Le Haen PA, van Dijk RA, et al. Activator protein-1 (AP-1) signalling in human atherosclerosis: results of a systematic evaluation and intervention study. Clin Sci. 2012;122:421-8.
133. Verborg W, Thomas H, Bissett D, et al. First-into-man phase I and pharmacokinetic study of XR5944.14, a novel agent with a unique mechanism of action. Br J Cancer. 2007;97:844-50.
134. Suchitha GP, Devasahayam Arokia Balaya R, Prasad TSK, Dagamajalu S. A signaling network map of lipoxin (LXA4): an anti-inflammatory molecule. Inflamm Res. 2024;73:1099-106.
135. Liu M, Kang W, Hu Z, Wang C, Zhang Y. Targeting MyD88: therapeutic mechanisms and potential applications of the specific inhibitor ST2825. Inflamm Res. 2023;72:2023-36.
136. Skrzycki M, Scibior-Bentkowska D, Podsiad M, Czeczot H. [Protein level of transcription factors AP-1 and NF-kappaB in selected human gastrointestinal tract tumors]. Pol Merkur Lekarski. 2008;25:510-15.
137. Columbano A, Ledda-Columbano GM, Pibiri M, et al. Increased expression of c-fos, c-jun and LRF-1 is not required for in vivo priming of hepatocytes by the mitogen TCPOBOP. Oncogene. 1997;14:857-63.
138. Innes H, Nahon P. Statistical perspectives on using hepatocellular carcinoma risk models to inform surveillance decisions. J Hepatol. 2023;79:1332-7.
139. Xu F, Tong M, Tong CSW, et al. A combinatorial CRISPR-Cas9 screen identifies ifenprodil as an adjunct to sorafenib for liver cancer treatment. Cancer Res. 2021;81:6219-32.
Cite This Article
How to Cite
Download Citation
Export Citation File:
Type of Import
Tips on Downloading Citation
Citation Manager File Format
Type of Import
Direct Import: When the Direct Import option is selected (the default state), a dialogue box will give you the option to Save or Open the downloaded citation data. Choosing Open will either launch your citation manager or give you a choice of applications with which to use the metadata. The Save option saves the file locally for later use.
Indirect Import: When the Indirect Import option is selected, the metadata is displayed and may be copied and pasted as needed.
About This Article
Copyright
Data & Comments
Data
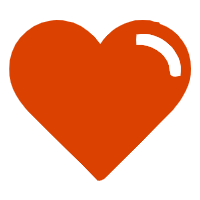
Comments
Comments must be written in English. Spam, offensive content, impersonation, and private information will not be permitted. If any comment is reported and identified as inappropriate content by OAE staff, the comment will be removed without notice. If you have any queries or need any help, please contact us at [email protected].