Advances in external beam radiation for hepatocellular carcinoma, stereotactic body radiation therapy (SBRT) and particle therapy
Abstract
Hepatocellular carcinoma (HCC) represents a leading cause of cancer-related mortality globally. Due to frequently complex disease presentation and comorbid underlying cirrhosis, many patients are not ideal candidates for surgical resection, transplantation, or other local ablative treatments. External beam radiation therapy (EBRT) has emerged as a promising alternative. EBRT has gained prominence due to advancements in targeting and treatment delivery technology. Advanced modalities such as intensity-modulated radiotherapy (IMRT), image-guided radiotherapy (IGRT), stereotactic body radiation therapy (SBRT), and proton beam therapy (PBT) have dramatically improved the precision of radiation delivery, resulting in a far more favorable therapeutic ratio. Here we cover the evolution of current practice and future prospects of EBRT in treating HCC, emphasizing the enhanced efficacy and reduced toxicity provided by these advanced technologies, and improved integration with other loco-regional and systemic therapies in reviewing the current indications for treatment.
Keywords
INTRODUCTION
The normal liver is sensitive to ionizing radiation, which produces fibrosis, leading to veno-occlusive disease within the treated volume. Classic radiation-induced liver disease (RILD) frequently results from historical treatment of exposing large volumes of liver to moderate radiation dose (30-35 Gy in conventionally fractionated regimens), and typically develops between 2 weeks and 4 months after treatment completion[1]. Classic RILD is characterized by anicteric hepatomegaly, ascites, and elevated liver enzymes, especially alkaline phosphatase. Non-classic RILD manifesting as markedly elevated transaminases (> 5X the upper limit of normal) with jaundice and declining Child-Pugh score is also recognized, with several predictive models developed to assess risk related to patient and treatment factors[1].
Historically, external beam radiation therapy (EBRT) used plain X-rays (two-dimensional conventional radiation, 2DCRT), which made it challenging to visualize hepatocellular carcinoma (HCC) accurately and sometimes required substantial margins to account for uncertainty in tumor positioning. The advent of CT-based treatment planning (three-dimensional conformal radiation, 3DCRT) has allowed for greater flexibility in selecting radiation beam angles, improving customizability of the high-dose target volume, but it still necessitated geometrically shaped targets at the intersection of beams. For HCC, which often has a very complex shape, particularly in patients with larger tumors or vascular invasion, modern radiation treatment technologies offer several key advancements, including the ability to conform the high-dose treatment region precisely to the irregular shape of the tumor, optimize radiation beam angles, and improve tumor localization and tracking during treatment, allowing for smaller treatment margins. New delivery technologies, such as protons and carbon ions therapy, allow for a reduction in entry and exit doses, thereby reducing radiation exposure to surrounding tissue. Previously, treatment plans remained fixed across multiple sessions, but adaptive replanning now allows for adjustments in radiation dose distribution to account for observed changes in the positions of sensitive organs-at-risk (OAR) and is increasingly feasible as a daily online adaptation strategy.
While radiation has historically not been effective in managing HCC arising in cirrhotic liver, the emergence of modern radiation treatment technologies has led to a wealth of new data demonstrating the safety and efficacy of external beam radiation in the management of HCC. Despite this, radiation has not yet been integrated into the Barcelona guidelines for HCC management. Herein we seek to discuss these technologies, present emerging data on their efficacy and toxicity, and explore specific scenarios where radiation might offer advantages and be appropriate for incorporation into the multidisciplinary management of HCC.
EVOLUTION OF RADIATION IN HCC
Intensity modulated radiation therapy
While conventional EBRT was historically forward-planned (meaning that radiation beams were applied evenly to a projection of the tumor from multiple angles), intensity-modulated radiotherapy (IMRT) is now typically implemented with inverse-planned arc-based beam arrangement. In inverse planning, all potential beam angles are utilized, with the planning system varying the intensity of the treatment beam over all portions of treatment delivery based on a mathematical optimization algorithm designed to prioritize the majority of treatment through the most efficient angles[2]. IMRT can create very complex shapes with very sharp dose gradients within the treatment target. This allows for appropriate dose to tumor with improved sparing of adjacent and surrounding normal tissue.
Image-guided radiation therapy
Conventional EBRT patients were historically aligned to marks made on the skin at the time of radiation treatment planning scans, with X-rays at the time of actual treatment serving to confirm alignment. As HCC is not visible on X-ray, this was just referenced to surrounding bony anatomy, which in the liver is quite sparse, so large margins for uncertainty were historically applied, particularly in patients where ascites or changes in bowel filling might displace skin marks or tumor relative to skin or bony alignment. image-guided radiotherapy (IGRT) integrates imaging [such as cone-beam CT (CBCT), real-time magnetic reasonance imaging (MRI), or ultrasound] into the linear accelerator to verify target localization more accurately during treatment. In addition to localizing the target prior to the initiation of treatment ultrasound, electronic portal imaging and MRI guidance using technologies like MR-Linac systems[3] enable real-time continuous tracking of tumor position during treatment, as well as daily online adaptive planning, reducing margins for target motion and deformation. For centers without access to MRI, the use of triggered imaging at the start of each breath-hold to verify the reproducibility of diaphragm or other high-resolution anatomy adjacent to the treatment target can be with a similar purpose[4]. The validation of multi-institutional implementation of image guidance and alignment was assessed as part of the Radiation Therapy Oncology Group (RTOG) 1112[5] protocol. While both soft-tissue and fiducial alignment were generally found to be accurate, a few notable large mismatches were observed in the soft tissue alignment group, highlighting the importance of institutional experience and available expertise at the time of treatment when using soft-tissue alignment[6].
Stereotactic body radiation therapy
stereotactic body radiation therapy (SBRT) combines the above tools of IMRT with IGRT and delivers very high dose focal radiation treatment with exceptional precision in a limited number of fractions. While conventional EBRT often relies on differences in potential for normal tissue and tumor to repair DNA damage in long fractionated treatment courses, SBRT typically delivers an ablative intent radiation dose and relies on precise delivery and sharp dose gradient to prevent normal tissue toxicity. While there are no randomized trials published at this time comparing EBRT to SBRT in the treatment of HCC, retrospective trials have quantified advantages[7] and randomized trials in non-small cell lung cancer have shown reduced toxicity with improved local control and overall survival[8] compared to conventional EBRT. Several studies have reported local control rates as high as 95% and overall survival rates that surpass those of traditional treatments in select patient groups[2,9-12]. The case below illustrates the differences between various treatment planning techniques. A 62-year-old patient with a history of treated hepatitis and Child-Pugh A5 cirrhosis presented with an 11.6 cm bi-lobar mass that met the OPTN criteria for HCC, with tumor thrombus in both the right hepatic and right portal vein [Figure 1]. If treated with 3-field or 3D conformal treatment plans, his maximum tolerated radiation dose would have been limited to 20 Gy in 5 fractions (a palliative dose) based on margins and beam arrangements [Figure 2]. IMRT enabled dose escalation to 30 Gy in 5 fractions, and SBRT to 35 Gy in 5 fractions [Figure 3]. The patient demonstrated an excellent radiographic response, and ultimately underwent surgical resection with confirmation of no viable residual disease.
Figure 1. 11.6 cm bi-lobar HCC with tumor thrombus involving the right hepatic and right portal veins. HCC: Hepatocellular carcinoma.
Figure 2. A comparison of the relative dose-distributions for each beam arrangement: 3-field, 3D conformal, IMRT, and SBRT. Compared to IMRT, SBRT utilizes a slightly different beam profile (flattening filter free) and allows for the dose calculation algorithm freedom to not restrict the central hot spot (given motion management) compared to more uniform dose planning in IMRT, ultimately achieving higher tumor dose relative to normal liver dose. IMRT: Intensity-modulated radiotherapy; SBRT: stereotactic body radiation therapy.
Figure 3. Dose-volume histogram showing dose to target (GTV and PTV) relative to normal liver (Liver-GTV). Doses to other critical tissues are simultaneously assessed but not displayed in the current figure. The plans are normalized to similar Liver-GTV mean doses. due to dose-limits to normal liver, the 3-field and 3D Conformal plans are limited to 20 Gy in 5 fractions, IMRT allows 30 Gy in 5 fractions, and SBRT 35 Gy in 5 fractions with even higher dose in the center of the target. IMRT: Intensity-modulated radiotherapy; SBRT: stereotactic body radiation therapy; GTV: gross tumor volume; ITV: internal target volume; PTV: planning tumor volume.
SBRT TREATMENT PLANNING AT CLEVELAND CLINIC
A linear accelerator platform is primarily used for SBRT, employing gated CBCT during multiple breath-holds controlled by a commercial respiratory gating system. In most cases, fiducial-free alignment of the liver contour and vascular anatomy enables consistent tumor positioning.
Treatment planning involves crucial steps such as breath-hold training (in 80% + of patients) and a tri-phasic CT scan with separate gated breath-holds. Localization techniques differ for breath-hold vs. non-breath-hold patients. For breath-hold, strategies include triggered imaging, liver dome windowing, visual verification, and surface recognition. Multimodality fusion with surgical clips, fiducials, or prior embolization deposits can enhance localization.
Intravenous contrast timing is optimized based on tumor type and patient factors. In cases without contrast, additional imaging is fused using manual or deformable registration, focusing on liver anatomy and vascular structures near the tumor. Localization is performed in multiple planes, potentially repeated for each lesion. Diagnostic quality planning images minimize artifacts and fusion errors.
Radiation planning utilizes the approach detailed by RTOG 1112[5], and normal tissue constraints reported in the most recent ASTRO Clinical Practice guidelines[13]. The most standard prescription dose is 50 Gy in 5 fractions, with dose reductions driven by normal liver sparing as per RTOG 1112. In the case of Child-Pugh B cirrhosis, the dose is automatically reduced by two levels (starting at 40 Gy in 5 fractions, with further reductions based on liver dosimetry). In addition to considering liver mean dose as per protocol, we also place a high value on maintaining at least 700 cc of normal liver below an ablative radiation dose (estimated at 17-20 Gy in 5 fractions, or 15-17 Gy in 3 fractions), with adjustment in required residual volume for unusually large or small patient size, as well as evaluation of the ratio of preserved liver to the total liver, with a goal of ablation of no more than 40%-50% in Child-Pugh A cirrhosis and 30%-35% in Child-Pugh B cirrhosis. As the liver is an organ with a parallel arrangement of functional sub-units, we believe that the mean dose may not be the ideal measure of the risk of decompensation and do place greater emphasis on the spared liver and the ratio of preserved to total liver, allowing for small deviations from the mean dose guidelines when these other two parameters are met. It is also important to consider liver dosing in the context of the patient’s clinical situation. For instance, dosing may be approached differently for a patient listed for transplant, where more aggressive dosing may be acceptable due to the availability of a transplant as a backup in case of liver decompensation. In contrast, for a patient requiring downstaging for transplant, as successful downstaging is essential for their eligibility, the rationale for accepting some risk of toxicity might be justified. We frequently allow for undercoverage of a portion of the planning target volume (PTV) to spare sensitive serial structures, such as the duodenum or other bowel structures. In these cases, a lower-dose portion of the PTV is created by expanding the structure to be spared by 7mm and allowing for a dose reduction in the portion of the intended PTV included in this overlap. While this concept has not been proven in all scenarios, dosimetric analysis of the SABR COMET trial, for instance, showed that such underdosing of the PTV - required for nearly 30% of enrolled patients - did not appear to compromise tumor control[14]. Appropriate biliary constraints are subject of controversy, with wide variations in institutional practices. We do not impose a hard constraint but limit the dose to the central biliary tree to 107% of the prescription. For patients with small target volumes not in proximity to any sensitive serial structures, three-fraction and single-fraction regimens are used.
PROTON BEAM THERAPY AND PARTICLE THERAPY
While conventional photon beams pass through the patient, depositing dose in a somewhat linear fashion (and concentrating dose only because of the convergence of multiple beams), proton beam therapy (PBT) and other particle therapies offer distinct dosimetric advantages due to the Bragg peak effect, whereby entrance dose is substantially lower compared to photons, and the majority of the beam energy is deposited over a relatively narrow range of depth, influenced by the beam energy, with minimal to no exit dose. While proton beam radiation will not improve the sparing of organs directly in contact with the radiation target, the reduction in entry and exit dose is particularly suited to reducing toxicities in organs sensitive to relatively lower doses of radiation, making the use of PBT in the setting of cirrhotic liver particularly intriguing. Several randomized trials comparing proton to photon radiation in several body sites are ongoing; early retrospective results for use in the setting of liver tumors and HCC, in particular, are encouraging. Technological advancements in PBT have further improved its efficacy. Pencil beam scanning (PBS) enables intensity-modulated proton therapy (IMPT), which provides better target conformality and sparing of organs at risk (OARs) compared to passive scattering techniques. This is particularly advantageous in treating large, irregularly shaped tumors. IMPT enables dose escalation while reducing radiation exposure to surrounding healthy tissues. Moreover, clinical outcomes with PBS-IMPT have shown promising results, with a significant reduction in RILD in cirrhotic patients. A prospective multi-institutional series enrolling 83 patients (44 with HCC and 37 with intrahepatic cholangiocarcinoma) with a median tumor dimension of 5.0 cm (range: 1.9 to 12.0 cm) and just over 25% with vascular invasion reached a median dose of 58 Gy in 15 fractions, with excellent 2-year local control of 94.8% in HCC and 94.1% in cholangiocarcinoma[15]. A range of retrospective reviews and propensity-matched analysis proton radiation appears to allow for higher treatment dose often associated with improved overall survival and decreased dose to non-target liver accompanied by suggestion of lower incidence of RILD[16,17]. However, a potential confounding factor is that proton radiation is often available only in large centers of excellence and selected for tumors in appropriate locations, introducing a potential bias in retrospective series. Open randomized trials in many disease sites, including HCC (NRG GI-003[18]), will be critical to fully understanding the benefits of this technology, given the substantially increased cost of proton vs. photon radiation at present.
Carbon ion and other heavy-particle treatments offer similar advantages as protons with reduced entry and exit dose due to the Bragg peak effect, while offering additional potential advantages in treatment biology. Due in part to their high atomic mass, carbon ions have a higher linear energy transfer compared to photons and protons and produce very dense ionization, resulting in a high relative biological effectiveness (RBE). RBE is standardized at 1 for photons[19], and considered approximately 1.1 for protons (meaning they create a 10% greater biologic effect in target tissue than photons. The RBE of carbon ions is thought to increase as they penetrate deeper into tissue, resulting in lower relative damage at entry and higher relative damage at depth (typically where the target tissue is located). While quantification of the carbon ion RBE is still under investigation and not fully understood in all treatment scenarios, it is felt to reach values as high as 2.0-3.5 at depth[19]. Carbon ion treatment is not yet broadly available, with limitations in part due to cost; however, published data are increasing and demonstrate generally excellent tolerability and control rates frequently at 90% or higher in the treatment of small to medium HCC[19].
Given that the primary source of toxicity in liver treatment results from the effects of beam entry and exit doses on normal liver tissue, resulting in the progression of cirrhosis, both proton and carbon ion therapies offer promising alternatives to photon-based treatments. As technological improvements reduce associated costs, modeling of dose deposition improves, and understanding of the RBE of heavy-ion therapy is expanded, there is a high chance that these treatments will replace photon-based radiation for liver tumors.
CLINICAL RESULTS
Clinical results are shown in Table 1.
HCC clinical trials investigating various treatment modalities
Study/Context | Treatment | Key results |
Small HCC (South Korean phase II trial. 50 patients, South Korean phase II trial, median follow-up of 47.8 months)[20] | SBRT | - 2-year local control: 100% - 5-year local control: 97.1% - 5-year overall survival: 77.6% - Radiologic responses: 83% (RECIST), 92.4% (mRECIST) - No grade ≥ 3 adverse events |
Meta-analysis (21,468 patients, 1-3 HCC < 5 cm in total identified 773 SBRT patients for matched analysis with 2,483 surgical patients and 3,745 RFA patients)[21] | SBRT vs. Surgery vs. RFA | - Similar disease progression-free survival for all treatments - SBRT had comparable overall survival to RFA - Surgery associated with the highest 3-year survival - SBRT associated with the lowest risk of severe complications |
Bush et al. [Randomized trial, 74 pts within Milan criteria with intent of bridging for liver transplant to either TACE (1-4 sessions, with 58% receiving a single treatment) or proton radiation (70.2 CGyE in 15 fractions)][22] | PBT vs. TACE | - Similar 2-year OS - PBT superior in progression-free survival (HR 3.62, P = 0.002) - PBT superior in local control (HR 5.64, P = 0.003) - PBT required fewer hospitalizations and showed 28% cost savings |
TRENDY trial (Randomized, 30 patients)[23] | SBRT vs. TACE | - Similar overall survival - Better local control with SBRT (median > 40 months vs. 12 months, P = 0.075) - No grade ≥ 3 toxicity in the SBRT arm vs. 3 episodes in the TACE arm |
Italian randomized trial (40 patients, 2nd line therapy)[24] | SBRT vs. TACE rechallenge | - Superior local control with SBRT (median not reached vs. 8 months, P = 0.0002) - Similar overall survival |
Retrospective review (Portal vein invasion)[7] | SBRT vs. conventional EBRT | - SBRT: Higher objective response rates (62% vs. 34%) - SBRT: Better portal vein re-canalization (33% vs. 15%) - SBRT: Superior in-field tumor control (70% vs. 32%) |
RTOG 1112 (Randomized, 193 patients, locally advanced HCC)[5] | SBRT + Sorafenib vs. Sorafenib alone | - SBRT + Sorafenib: Improved progression-free survival (9.2 months vs. 5.5 months, HR 0.55, P = 0.0001) - SBRT + Sorafenib: Improved overall survival (15.8 months vs. 12.3 months, HR 0.72, P = 0.042) - SBRT + Sorafenib: Better quality of life outcomes |
Retrospective study (Taiwan, China)[25] | Atezolizumab + Bevacizumab + Proton RT vs. Atezolizumab + Bevacizumab | - With RT: Median survival not reached (> 60% at 18 months) - Without RT: Median survival 5.5 months - With RT: 0% intrahepatic tumor growth at 12 months - Without RT: > 50% intrahepatic tumor growth at 12 months |
Retrospective review (Yonsei University, Seoul)[26] | Chemoradiation followed by resection | - Best outcomes with chemoradiation followed by resection (about 50% disease-free survival at 5 years despite vascular invasion). |
Yoon et al. (90 patients with hepatitis B-driven HCC in South Korea)[27] | TACE + EBRT vs. Sorafenib | - TACE + EBRT resulted in a 3-fold higher progression-free survival compared to Sorafenib. - Improved overall survival. |
Prospective multi-institutional series [83 patients (44 with HCC, 37 with cholangiocarcinoma), median tumor size 5.0 cm, 25% with vascular invasion][15] | PBT (58 Gy in 15 fractions) | - 2-year local control: 94.8% for HCC, 94.1% for cholangiocarcinoma. - Promising outcomes with reduced RILD. |
Small HCC
While small, isolated HCC is typically treated with surgical resection or radiofrequency ablation (RFA), SBRT similarly has demonstrated excellent outcomes when applied in this setting. In a single-arm South Korean phase II clinical trial, SBRT was evaluated as an alternative treatment for small HCCs (≤ 5 cm) that were not amenable to standard approaches. The study included 50 patients with a median follow-up period of 47.8 months. Notably, the 2-year local control rate was 100%, and the 5-year local control rate remained high at 97.1%. Overall survival at 5 years was 77.6%. Radiologic responses were evident in 83% of lesions (according to RECIST criteria) and 92.4% (according to modified RECIST criteria). Importantly, no grade ≥ 3 adverse events were observed, highlighting SBRT’s minimal toxicity profile[20]. Similarly, a meta-analysis of 21,468 patients with 1-3 HCC < 5 cm in total identified 773 SBRT patients for matched analysis with 2,483 surgical patients and 3,745 RFA patients[21]. The pooled analysis showed that disease progression-free survival was similar across all three treatments. SBRT had comparable overall survival to RFA, while surgery was associated with the highest 3-year survival. Additionally, SBRT was linked to the lowest risk of severe complications. Furthermore, in the unadjusted population, the authors found that all SBRT groups had inferior baseline characteristics compared to both surgery and RFA, such as older age, larger tumors, worse liver function, poorer performance status, and more extensive pre-treatment history, illustrating the difficulty in making retrospective comparisons between treatments at different positions in triage considerations.
Comparison to trans-arterial chemoembolization in early HCC
While the availability of trans-arterial chemoembolization (TACE) predates SBRT, the potential treatment indications are highly overlapping in the management of early-stage HCC, and few comparative data exist. Comparing large databases, such as the NCDB and SEER, is challenging because evaluating outcomes across different institutions - comparing first-line vs. second-line treatment options - introduces a significant risk of selection bias. A few early randomized trials have recently compared radiation to TACE, though they involve only a small population of enrolled patients. Bush et al. randomized 74 pts within Milan criteria with the intent of bridging for liver transplant to either TACE (1-4 sessions, with 58% receiving a single treatment) or proton radiation (70.2 CGyE in 15 fractions)[22]. The 2-year OS for PBT vs. TACE was similar; however, progression-free survival (HR 3.62, P = 0.002) and local control (HR 5.64, P = 0.003) both substantially favored PBT. Subjects receiving TACE required significantly more frequent hospitalization for treatment-related complications and totaled more inpatient days (166) compared to PBT (24), even after subtracting routine post-TACE hospital admission days (53). Additionally, the total mean cost per patient for treatment and post-treatment care revealed a 28% cost savings for PBT. The TRENDY trial also randomized patients to TACE vs. SBRT (48-54 Gy/6 fractions). However, it was terminated early after enrolling only 30 patients due to slow accrual[23]. The results mirrored those of Bush et al.’s PBT trial with similar overall survival, but numerically better local control in the SBRT arm, which did not meet statistical significance on the pre-specified endpoint of median local control (> 40 months for SBRT, 12 months for TACE, P = 0.075) but favored SBRT in the post-hoc analysis of 1- and 2-year local control (P = 0.019)[22]. Three episodes of possibly related toxicity grade ≥ 3 were observed after TACE. No episodes were observed after SBRT. QoL remained stable after both treatment arms.
Recurrent tumor after incomplete response to other local therapies
While SBRT and PBT therapy appear to offer favorable outcomes compared to TACE in early HCC, the above trials remain numerically small, and a greater volume of data may be needed to establish SBRT as a primary standard of care for unresectable early HCC. A third randomized trial investigated the role of SBRT vs. TACE as second-line salvage therapy after an upfront incomplete TACE. Much like TRENDY, this single-center Italian randomized trial was underpowered and closed after enrolling only 40 patients due to slow accrual[24]. Despite this, the use of SBRT resulted in superior LC compared to TACE rechallenge (median not reached vs. 8 months, P = 0.0002), though overall survival remained quite similar. Overall, although the limited patient numbers in these trials may not provide sufficient evidence to support replacing TACE with SBRT or PBT as the primary treatment for inoperable HCC, the findings certainly establish SBRT as an excellent option either upfront or following incomplete embolization.
Vascular tumor invasion
Patients with vascular tumor invasion of either the portal or hepatic venous systems represent some of the most challenging cases in HCC. Not only does this result in complex target volumes but it also significantly increases the risk of systemic dissemination and metastatic disease. Additionally, the presence of vascular invasion often leads to decompensated cirrhosis with a concomitant decline in patient function and performance status, which also substantially impacts their ability to tolerate the complex therapies needed to address their disease. Vascular invasion is associated with a dramatic decrease in survival. For example, in a series of 1,317 patients treated at a German tertiary referral center between 2005-2016, 37% were found to have vascular invasion. Median survival dropped in this group from 35.7 months without invasion to 7.2 months with invasion[28]. Outcomes can also be further stratified by the degree of vascular invasion, with a median survival of 14.6, 9.4, 5.8, and 4.8 months, respectively, for patients with segmental, second-order, first-order (right or left), or main portal vein invasion.
While vascular invasion has a significant impact on the outcomes of HCC, this has been a notable area of progress in HCC over the last decade, and we do expect future data to reflect these improvements. The development of immune checkpoint inhibitors containing regimens such as nivolumab, atezolizumab with bevacizumab, and durvalumab with tremelimumab has demonstrated improvement in overall survival in multiple randomized trials, compared to the prior standard of care Sorafenib, in patients with advanced or unresectable HCC, including those with vascular invasion[29-31]. Despite these significant improvements in overall survival, including some long-term survivors, objective response rates to chemo-immune therapy remain limited. The IMbrave trial, for example, reported an objective response rate of 30% (8% complete, 22% partial) for atezolizumab and bevacizumab (compared to 11% for sorafenib), with stable disease in another 44%; however, only 69% of responses lasted longer than 12 months, 51% lasted beyond 18 months, and the 2-year progression-free survival was < 20%[30]. Though this represents a significant improvement compared to sorafenib, additional therapies are needed in this advanced disease population. Radiation, especially when administered using modern techniques to deliver an adequate dose, represents an excellent candidate, given generally excellent in-field tumor control to augment the modest response rates of systemic therapy, particularly for patients with vascular invasion, which frequently leads to decompensated cirrhosis following local progression.
In a retrospective review of patients with portal vein invasion, stereotactic radiation allows for greater delivered dose compared to conventionally fractionated external beam radiation, and is associated with improved objective response rates (62% vs. 34%), portal vein re-canalization (33% vs. 15%), and in-field tumor control (70% vs. 32%). Higher delivered dose (BED10 > 65 Gy, and higher performance status correlated with improved survival[7]. A SEER analysis of 3,181 HCC patients with major vascular invasion found that the receipt of external beam radiation was associated with improved overall survival, independent of tumor size, after propensity score analysis[32]. Additionally, SBRT has been tested in the setting of HCC with invasion extending to the right atrium. A prospective multi-center Korean trial KROG 17-10 demonstrated favorable local control, although with high rates of distant disease progression in the Sorafenib-only era[33].
Two randomized trials have also investigated the addition of external beam radiation to systemic therapy, though both in the era of Sorafenib. Yoon et al. randomized 90 patients with predominately hepatitis B-driven HCC in South Korea to receive either Sorafenib or TACE for the primary tumor and EBRT for the tumor thrombus[27]. External beam radiation was administered using a 3D conformal approach, delivering 45 Gy in 15-18 fractions, with each fraction 2.5-3 Gy. Despite the relatively low total radiation dose and 3D conformal technique, TACE + EBRT demonstrated 3-fold higher progression-free survival at the primary endpoint of 24 weeks and maintained statistically significant improvements in progression-free and overall survival throughout the study follow-up, with almost 20% of the patients in the local therapy arm alive at the study conclusion compared to none in the Sorafenib alone arm. More recently, the landmark RTOG 1112 trial was completed, randomizing 193 patients from 23 predominately North American centers to Sorafenib vs. SBRT followed by Sorafenib. However, the results are currently reported only in abstract form[5]. This study accrued patients with locally advanced HCC with < 5 lesions with a sum of < 20 cm and up to 3 cm of extrahepatic metastasis. Almost 60% of accrued patients had multiple sites of disease with the median sum of diameter of lesions at 7.8 cm. Over 70% had vascular invasion (over 60% at least to first order branches), 80% had Barcelona C disease, and half had Zubrod performance status of 0-1 vs. half status of 2. Radiation consisted of SBRT delivering 50 Gy in 5 fractions, with planned dose reductions as needed to meet normal liver constraints, resulting in a median delivered dose of 35 Gy in 5 fractions. Despite the very advanced nature of disease in the enrolled population, the addition of SBRT improved progression-free (5.5 months vs. 9.2 months, HR 0.55, P = 0.0001) and overall survival (12.3 months vs. 15.8 months, HR 0.72, P-0.042 on multi-variate analysis), without any significant increase in adverse treatment-related events. Moreover, on quality-of-life assessment using the Functional Assessment of Cancer Therapy-Hepatobiliary (FACT-Hep) scoring system comparing baseline to 6 months post treatment, 80% of patients suffered a decline in scores (with only 10% improving by at least 5 points) in the Sorafenib group, compared to only 47% in the SBRT group (with 35% improving by at least 5 points) - likely reflective of the role of radiation in managing symptoms of local portal vein invasion and preventing or reversing liver decompensation.
These two trials convincingly establish the role of radiation in improving long-term tumor control as well as progression-free and overall survival compared to Sorafenib alone, with all of the cured/non-progressing patients in arms containing radiation in the above randomized trials. The critical current question is whether the benefit of radiation will hold in the setting of more modern systemic therapy regimens, including those that involve immune therapy. Combining radiation with immunotherapy can induce an anti-tumor immune response through mechanisms such as immunogenic cell death. Early clinical trials combining SBRT with immunotherapy have shown promising results, and ongoing studies aim to establish these combinations as standard treatments for advanced HCC[34,35]. There is a suggestion of the potential synergy of immune therapy with liver-directed SBRT, and interestingly, pre-treatment immune phenotyping of patients has some promise in selecting those with and without disease progression after treatment[36]. While prospective trials have yet to evaluate the role of SBRT in addition to modern immune therapy combinations, a recent small retrospective trial in Taiwan highlights the potential promise of this treatment combination. In the study, patients receiving atezolizumab plus bevacizumab and proton radiation (to 72.6 GyE in 22 fractions) demonstrated significantly increased survival compared to those receiving atezolizumab and bevacizumab alone [median survival not reached (> 60% at 18 months) vs. 5.5 months][25]. This was attributed primarily to the prevention of intra-hepatic tumor growth (0% vs. > 50% at 12 months) and associated better preservation of liver function in the radiated patients, despite a higher incidence of main portal vein invasion in the radiated group at presentation.
INTEGRATIVE APPROACHES: COMBINATION THERAPIES
SBRT as a selection for surgery in HCC with vascular invasion or other advanced features
While resection represents the standard of care for HCC, this is a challenging endeavor for patients with advanced disease including vascular invasion. Perioperative mortality ranges from 1%-6%, and rates of distant recurrence are high compared to early HCC[37], such that selection for resection is complicated. A retrospective review from the Yonsei University College of Medicine in Seoul, South Korea, explored the results for patients with portal vein tumor thrombus who underwent either upfront resection, chemoradiation alone, or chemoradiation followed by resection, with radiation consisting of 3D conformal radiation to 45 Gy in 25 fractions, along with hepatic infusion of 5-fluoro-uracil followed by further adjuvant chemotherapy. Patients with favorable liver function after chemoradiation then underwent surgical resection[26]. On retrospective assessment of outcomes, patients receiving either upfront surgery or chemoradiation only had similar outcomes, while patients receiving chemoradiation followed by resection had the best outcomes, with about 50% disease-free survival at 5 years despite presenting with portal vein invasion. Chemoradiation allows for time for enlargement of functional liver reserve to facilitate resection and can offer an opportunity for biological selection prior to decision making on complex surgical resection. It is difficult to assess the extent to which surgery will improve local control after SBRT, as imaging alone cannot reliably identify patients who might harbor microscopic residual disease despite a complete radiographic response. However, further data on resection after definitive SBRT, along with advances in imaging, will help clarify patient selection in this setting. The pre- and post-treatment MRI images from the patient in the treatment example above are presented in Figure 4. He ultimately underwent resection with no evidence of residual disease in the radiated field, though he did have microscopic tumor in other portions of the resection specimen, indicating undetected sub-clinical disease.
Figure 4. Radiographic response after SBRT. The patient underwent SBRT and concurrent durvalumab and tremelimumab. At the 3-month mark, early imaging typically shows mild residual enhancement. However, the response often continues to evolve, with subsequent imaging revealing no residual enhancement, even without additional treatment. This indicates that radiation destroys tumor DNA, leading to genetically non-viable tumors when a sufficient dose is applied. The timeframe for radiographic response, however, can vary significantly. SBRT: Stereotactic body radiation therapy.
SBRT combined with TACE
Some centers have combined EBRT with TACE as a planned dual-modality approach. For example, the prospective trial by Yoon et al. compared TACE + EBRT with Sorafenib in patients with vascular invasion. While retrospective series have suggested potential benefits to a combined modality approach[27], this has not been assessed prospectively, and we do not routinely offer radiation after complete TACE at our institution. For patients with vascular invasion where complete TACE might not be feasible, we often favor either transarterial radioembolization (TARE) or SBRT. For those considering combined TACE and SBRT, the issue of timing is relevant. A single Chinese randomized trial randomized 120 patients with portal vein invading HCC planned for combined modality therapy to SBRT followed by TACE vs. TACE followed by SBRT[38]. Delivery of radiation prior to TACE was associated with marginally improved disease control [61.7% vs. 46.7% (P = 0.099) at 6 months] and overall survival (15.4 months vs. 11.5 months, HR = 0.68, P = 0.054), though this was only noted to be statistically significant in the subgroup with type III or IV tumor thrombus. This represents a single small randomized trial; however, it is reasonable that starting with the modality that directly addresses the tumor thrombus might present an advantage as it represents the most likely cause for the decline in function.
SBRT combined with TARE
Adding SBRT to TARE has also demonstrated acceptable tolerability and effectiveness. Although there is a concern about cumulative radiation toxicity, current studies indicate that the combination is generally well-tolerated for smaller tumor volumes, with similar tumor control and survival rates as seen with SBRT combined with TACE[3,39]. Clinicians should consider this combined strategy, particularly in multifocal disease contexts or when managing tumors with complex spatial anatomy, to enhance patient outcomes and maintain quality of life[40]. At Cleveland Clinic, we do not routinely plan combined SBRT and TARE, but do utilize SBRT to cover portions of the tumor unable to be completely addressed by TARE, either shortly thereafter in cases of gross underdosing, or at the time of first follow-up imaging demonstrating residual disease when it is less clear. Patients receiving TARE undergo post-injection positron emission tomography (PET) scan, which enables visualization of TARE dose and facilitates the integration of dosimetry in combined therapy approaches.
Recurrence after SBRT
Recurrence after SBRT should be classified into the category of local recurrence (at or within 2cm of the treated target), liver recurrence (new sites of disease more than 2 cm outside the prior treatment target), or distant (outside the liver). For local recurrence, other liver-directed therapies (RFA, TACE, or TARE) should be considered first. While one might consider re-irradiation of a local recurrence, particularly if a significant time interval has elapsed, data in this setting are quite limited and other liver-directed therapies would be favored. For liver recurrence outside of the initial radiation target, further radiation would be considered, provided a favorable response and tolerance to the initial radiation regimen, as well as sufficient liver reserve. Other liver-directed therapies are also options, and the treatment decision should be based on a multidisciplinary consensus on the best management option for tumor location. Liver transplant could also be an option for patients with local or liver recurrence, provided their tumor remains within Milan criteria and they are otherwise appropriate for transplant. Systemic therapy is typically reserved for either local or liver recurrence untreatable with any liver-directed therapy, or for patients with extrahepatic metastasis.
SBRT for extrahepatic metastasis
Patients with advanced HCC often experience extrahepatic metastasis, commonly involving the lungs, lymph nodes, or bones. SBRT has shown promise as a non-surgical, radical treatment option for reducing symptoms and complications related to metastatic implants. Achieving local control in the primary tumor using SBRT may also promote longer survival compared to systemic therapies alone[40-44]. The landmark SABR-COMET trial[44] ignited interest in focal ablative treatment of metachronous oligometastasis (defined in this trial as 1-5 metastatic lesions in a patient with a controlled primary tumor, though > 90% of enrolled patients had 3 or fewer metastasis). This study was not specific to HCC, with 99 patients with a range of primary tumor histologies randomized 2:1 to systemic therapy + SBRT vs. systemic therapy alone. Not only did the addition of SBRT to oligometastatic disease more than double progression-free survival (P = 0.001; HR, 0.48, with 5y PFS of 17% with vs. 0% without SBRT), but it also suggested a significant improvement to overall survival with a 25% absolute benefit from SBRT (5y survival 42.3% with SBRT compared to 17.7% without, P = 0.001). It is difficult to know how this specifically applies to HCC patients given the preponderance of more common histologies accrued to this trial; however, given the magnitude of observed benefits, it is reasonable to offer treatment to patients meeting trial criteria while awaiting confirmatory evidence in specific diseases. It is also notable from a radiation planning perspective that nearly 30% (32/109 treated lesions with available dosimetry) required under-dosing of the target tissue in order to spare adjacent normal tissue. However, on sub-set analysis of these patients, there was no observed detriment in local control, progression-free or overall survival, and no increase in toxicity[14], suggesting that dose reduction to spare adjacent organs-at-risk is likely appropriate in treating oligometastatic disease.
QUALITY OF LIFE AND TOXICITIES AFTER EXTERNAL RADIATION
While in general, we would hope that the addition of radiation would improve the preservation of quality of life by preventing disease-related liver decompensation (particularly in patients with vascular invasion or advanced disease), it should be noted that some normal liver immediately adjacent to the treated tumor will be damaged in all cases. This must be balanced against the potential benefits of radiation-related tumor control. For any patient receiving radiation, their functional liver reserve will be temporarily impacted. Generally, studies have reported the risk of a two-point or greater decline in Child-Pugh score of 20%-30%[45,46]. This would be expected to vary significantly with tumor size and location (with central tumors resulting in exposure of more adjacent liver) as well as treatment technology (with protons and other heavy particles offering the potential of better sparing, particularly for central tumors)[47]. Patients with poorly controlled cirrhosis, including those with Child-Pugh C disease, generally should not be treated with radiation (outside of very specific circumstances related to transplant candidacy or other unusual exceptions, or low-dose palliative radiation directed at symptomatic tumor thrombus), as their risk of liver decompensation with treatment is substantial[48].
Another significant consideration is the risk of damage to neighboring GI viscous structures, with the stomach and small bowel being the most sensitive, although the large bowel is also potentially at risk[49].
Generally, SBRT fares well overall in terms of quality of life assessment. Compared to Sorafenib, quality of life preservation was significantly better in the SBRT arm of RTOG 1112, with 33% less chance of quality-of-life decline in the SBRT arm, likely reflective of SBRT being well tolerated and preventing disease-related quality of life decline in this population of patients with vascular invasion[5]. Similarly, less treatment-related toxicity and far fewer hospitalized days were observed with SBRT than TACE in Bush et al.’s randomized trial[22]. Klein et al. at Princess Margaret completed a longitudinal prospective quality of life study for 222 patients with liver metastasis, HCC and cholangiocarcinoma undergoing SBRT, using QLQ-C30 (available for 205 patients) and FACTHep (available for 196 patients) questionnaires[50]. No differences in baseline quality of life were noted for metastasis vs. HCC vs. cholangiocarcinoma. These questionnaires assess quality of life on a wide range of relevant domains and found appetite loss and fatigue were clinically and statistically worsened 1 month after treatment, but recovered by 3 months, while other facets remained essentially unchanged. At 3 and 12 months after treatment, respectively, the FACT-Hep score had improved relative to baseline in 13%/19%, worsened in 36%/27%, and remained stable in 51%/54%. Using the QLQ-C30 Global Health score, QoL improved in 16%/23%, worsened in 34%/39%, and remained stable in 50%/38% at 3 and 12 months, respectively.
PERSONALIZED MEDICINE AND RADIO-SENSITIZATION STRATEGIES
Personalized medicine involves a deep understanding of the genetic makeup of tumors, which influences their sensitivity to radiation. For instance, genetic markers such as TP53, CTNNB1, and TERT mutations play a crucial role in tumor behavior and response to therapy, enabling more tailored treatment approaches[51]. By characterizing specific genetic and molecular profiles, clinicians can adjust RT doses to improve patient outcomes. This customization aids in delivering optimum doses that maximize tumor control and minimize side effects, thus preserving healthy tissue functions. Integrating SBRT with radiosensitizers has emerged as a promising strategy for enhancing the radiosensitivity of HCC tumors. Radiosensitizers include chemotherapy, targeted therapies, and novel agents like nanoparticles. Nanoparticles, especially those containing high Z atoms such as gold, can absorb radiation and generate reactive oxygen species (ROS) to induce DNA double-strand breaks, significantly increasing apoptosis in tumor cells. These agents enhance the effect of radiation on tumor cells, making them more vulnerable to radiation damage[9,11,35,42].
CHALLENGES AND FUTURE DIRECTIONS
While significant strides have been made in integrating RT into HCC treatment paradigms, several challenges remain. These include identifying the optimal patient subsets for various RT modalities, managing radiation toxicity, and understanding the long-term benefits of combination therapies. Future research directions include:
1. Technological innovations: Enhancing RT technology further, such as increased utilization of proton beam radiation, as well as implementing MR-Linac and other systems for real-time tumor tracking and adaptive radiotherapy[3].
2. Personalized medicine: Utilizing genomic profiling and molecular imaging to tailor RT doses and schedules based on individual tumor characteristics.
3. Large-scale clinical trials: Conducting randomized controlled trials to definitively establish the roles of various RT modalities and combinations in treating HCC, thereby refining treatment algorithms and improving outcomes.
4. Multidisciplinary integration: As radiation is not yet formally integrated into the Barcelona guidelines, there is likely significant variation in its clinical application across institutions. While awaiting a larger volume of comparative data between modalities, interdisciplinary communication between radiation oncologists with hepatologists, hepatobiliary surgeons, medical oncologists, diagnostic radiologists, and interventional radiologists is critical. The care of HCC patients is remarkably interdisciplinary, with the best care plan driven by many features including stage, liver health, tumor location and anatomy, transplant candidacy and more, leading to a range of best plans for otherwise similar patients, and the connection of all of the above factors driving decision making far more than any single factor. In this environment, collaborative teamwork with a shared understanding of the strengths and limitations of the available treatments and how they apply to each individual situation is critical to achieving the best outcomes.
CONCLUSION
Radiation therapy has become a pivotal component in the multidisciplinary management of HCC. Advances in RT technology, coupled with emerging combination therapies, have significantly improved the efficacy and safety of this treatment modality. As ongoing research continues to explore and refine these technologies, the future of RT in HCC management holds promising potential for enhanced patient outcomes.
DECLARATIONS
Acknowledgments
The authors would like to thank and acknowledge Madison Dylong, CMD, for assistance with developing figures for this review.
Authors’ contributions
Both made substantial contributions to the conception and design of the review paper, including interpretation of literature, writing, and editing of the paper: Seth L, Stephans KL
Availability of data and materials
Not applicable.
Financial support and sponsorship
None.
Conflicts of interest
All authors declared that there are no conflicts of interest.
Ethical approval and consent to participate
Not applicable.
Consent for publication
De-identified individual patient images without any protected health information were included in this manuscript. As the patient was deceased at the time of publication, written consent for inclusion in the publication was obtained from the patient’s spouse.
Copyright
© The Author(s) 2024.
REFERENCES
1. Koay EJ, Owen D, Das P. Radiation-induced liver disease and modern radiotherapy. Semin Radiat Oncol 2018;28:321-31.
2. Zaki P, Chuong MD, Schaub SK, Lo SS, Ibrahim M, Apisarnthanarax S. Proton beam therapy and photon-based magnetic resonance image-guided radiation therapy: the next frontiers of radiation therapy for hepatocellular carcinoma. Technol Cancer Res Treat 2023;22:15330338231206335.
3. Liu Y, Chou B, Yalamanchili A, Lim SN, Dawson LA, Thomas TO. Local therapies for hepatocellular carcinoma and role of MRI-guided adaptive radiation therapy. J Clin Med 2023;12:3517.
4. Guo B, Stephans K, Woody N, Antolak A, Moazzezi M, Xia P. Online verification of breath-hold reproducibility using kV-triggered imaging for liver stereotactic body radiation therapy. J Appl Clin Med Phys 2023;24:e14045.
5. Dawson LA, Winter KA, Knox JJ, et al. NRG/RTOG 1112: randomized phase III study of sorafenib vs. stereotactic body radiation therapy (SBRT) followed by sorafenib in hepatocellular carcinoma (HCC). JCO 2023;41:489-489.
6. Craig T, Xiao Y, McNulty S, Dawson LA. Insights from image guided radiation therapy credentialing for the NRG oncology RTOG 1112 liver stereotactic body radiation therapy trial. Pract Radiat Oncol 2023;13:239-45.
7. Yang JF, Lo CH, Lee MS, et al. Stereotactic ablative radiotherapy versus conventionally fractionated radiotherapy in the treatment of hepatocellular carcinoma with portal vein invasion: a retrospective analysis. Radiat Oncol 2019;14:180.
8. Lin Q, Zhou N, Zhu X, et al. Outcomes of SBRT for lung oligo-recurrence of non-small cell lung cancer: a retrospective analysis. J Radiat Res 2022;63:272-80.
9. Pérez-Romasanta LA, González-Del Portillo E, Rodríguez-Gutiérrez A, Matías-Pérez Á. Stereotactic radiotherapy for hepatocellular carcinoma, radiosensitization strategies and radiation-immunotherapy combination. Cancers 2021;13:192.
10. Schaub SK, Hartvigson PE, Lock MI, et al. Stereotactic body radiation therapy for hepatocellular carcinoma: current trends and controversies. Technol Cancer Res Treat 2018;17:1533033818790217.
11. Sayan M, Yegya-Raman N, Greco SH, et al. Rethinking the role of radiation therapy in the treatment of unresectable hepatocellular carcinoma: a data driven treatment algorithm for optimizing outcomes. Front Oncol 2019;9:345.
12. Tong VJW, Shelat VG, Chao YK. Clinical application of advances and innovation in radiation treatment of hepatocellular carcinoma. J Clin Transl Res 2021;7:811-33.
13. Apisarnthanarax S, Barry A, Cao M, et al. External beam radiation therapy for primary liver cancers: an ASTRO clinical practice guideline. Pract Radiat Oncol 2022;12:28-51.
14. Van Oirschot M, Bergman A, Verbakel WFAR, et al. Determining planning priorities for SABR for oligometastatic disease: a secondary analysis of the SABR-COMET phase II randomized trial. Int J Radiat Oncol Biol Phys 2022;114:1016-21.
15. Hong TS, Wo JY, Yeap BY, et al. Multi-institutional phase II study of high-dose hypofractionated proton beam therapy in patients with localized, unresectable hepatocellular carcinoma and intrahepatic cholangiocarcinoma. J Clin Oncol 2016;34:460-8.
16. Roberts HJ, Hong TS. Proton versus photon radiotherapy for hepatocellular carcinoma: current data and technical considerations. J Radiosurgery SBRT 2023;9:9-16.
17. Cheng JY, Liu CM, Wang YM, et al. Proton versus photon radiotherapy for primary hepatocellular carcinoma: a propensity-matched analysis. Radiat Oncol 2020;15:159.
18. NRG oncology. A phase III randomized trial of protons versus photons for hepatocellular carcinoma. Available from: https://www.nrgoncology.org/Clinical-Trials/Protocol/nrg-gi003?filter=nrg-gi003. [Last accessed on 22 Nov 2024].
19. Byun HK, Kim C, Seong J. Carbon ion radiotherapy in the treatment of hepatocellular carcinoma. Clin Mol Hepatol 2023;29:945-57.
20. Yoon SM, Kim SY, Lim YS, et al. Stereotactic body radiation therapy for small (≤ 5 cm) hepatocellular carcinoma not amenable to curative treatment: results of a single-arm, phase II clinical trial. Clin Mol Hepatol 2020;26:506-15.
21. Li LQ, Su TS, Wu QY, Lin ZT, Liang SX. Therapeutic outcome of stereotactic body radiotherapy for small hepatocellular carcinoma lesions - a systematic review and network meta-analysis. Clin Oncol 2023;35:652-64.
22. Bush DA, Volk M, Smith JC, et al. Proton beam radiotherapy versus transarterial chemoembolization for hepatocellular carcinoma: results of a randomized clinical trial. Cancer 2023;129:3554-63.
23. Méndez Romero A, van der Holt B, Willemssen FEJA, et al. Transarterial chemoembolization with drug-eluting beads versus stereotactic body radiation therapy for hepatocellular carcinoma: outcomes from a multicenter, randomized, phase 2 trial (the TRENDY trial). Int J Radiat Oncol Biol Phys 2023;117:45-52.
24. Comito T, Loi M, Franzese C, et al. Stereotactic radiotherapy after incomplete transarterial (chemo-) embolization (TAE\TACE) versus exclusive TAE or TACE for treatment of inoperable HCC: a phase III trial (NCT02323360). Curr Oncol 2022;29:8802-13.
25. Su CW, Teng W, Shen EY, et al. Concurrent atezolizumab plus bevacizumab and high-dose external beam radiotherapy for highly advanced hepatocellular carcinoma. Oncologist 2024;29:e922-31.
26. Chong JU, Choi GH, Han DH, et al. Downstaging with localized concurrent chemoradiotherapy can identify optimal surgical candidates in hepatocellular carcinoma with portal vein tumor thrombus. Ann Surg Oncol 2018;25:3308-15.
27. Yoon SM, Ryoo BY, Lee SJ, et al. Efficacy and safety of transarterial chemoembolization plus external beam radiotherapy vs sorafenib in hepatocellular carcinoma with macroscopic vascular invasion: a randomized clinical trial. JAMA Oncol 2018;4:661-9.
28. Mähringer-Kunz A, Steinle V, Düber C, et al. Extent of portal vein tumour thrombosis in patients with hepatocellular carcinoma: the more, the worse? Liver Int 2019;39:324-31.
29. Yau T, Kang YK, Kim TY, et al. Efficacy and safety of nivolumab plus ipilimumab in patients with advanced hepatocellular carcinoma previously treated with sorafenib: the CheckMate 040 randomized clinical trial. JAMA Oncol 2020;6:e204564.
30. Cheng AL, Qin S, Ikeda M, et al. Updated efficacy and safety data from IMbrave150: Atezolizumab plus bevacizumab vs. sorafenib for unresectable hepatocellular carcinoma. J Hepatol 2022;76:862-73.
31. Sangro B, Chan SL, Kelley RK, et al; HIMALAYA investigators. Four-year overall survival update from the phase III HIMALAYA study of tremelimumab plus durvalumab in unresectable hepatocellular carcinoma. Ann Oncol 2024;35:448-57.
32. Lin Q, Huang X, Zhong C, Luo T, Zeng X, Chen S. Improved survival with radiotherapy in hepatocellular carcinoma with major vascular invasion: a propensity-matched analysis of surveillance, epidemiology, and end results database. Cancer Med 2019;8:515-26.
33. Rim CH, Jeong BK, Kim TH, Hee Kim J, Kang HC, Seong J. Effectiveness and feasibility of external beam radiotherapy for hepatocellular carcinoma with inferior vena cava and/or right atrium involvement: a multicenter trial in Korea (KROG 17-10). Int J Radiat Biol 2020;96:759-66.
34. Lewis S, Dawson L, Barry A, Stanescu T, Mohamad I, Hosni A. Stereotactic body radiation therapy for hepatocellular carcinoma: from infancy to ongoing maturity. JHEP Rep 2022;4:100498.
35. Tojjari A, Yu J, Saeed A. Immunotherapy and radiation therapy combinatorial approaches in hepatocellular carcinoma. Cancers 2024;16:1058.
36. Reiss KA, Wattenberg MM, Damjanov N, et al. A pilot study of galunisertib plus stereotactic body radiotherapy in patients with advanced hepatocellular carcinoma. Mol Cancer Ther 2021;20:389-97.
37. Quirk M, Kim YH, Saab S, Lee EW. Management of hepatocellular carcinoma with portal vein thrombosis. World J Gastroenterol 2015;21:3462-71.
38. Guo L, Wei X, Feng S, et al. Radiotherapy prior to or after transcatheter arterial chemoembolization for the treatment of hepatocellular carcinoma with portal vein tumor thrombus: a randomized controlled trial. Hepatol Int 2022;16:1368-78.
39. Kimura T, Fujiwara T, Kameoka T, Adachi Y, Kariya S. The current role of stereotactic body radiation therapy (SBRT) in hepatocellular carcinoma (HCC). Cancers 2022;14:4383.
41. Chen L, Zhang R, Lin Z, Tan Q, Huang Z, Liang B. Radiation therapy in the era of immune treatment for hepatocellular carcinoma. Front Immunol 2023;14:1100079.
42. Yang Y, Xiong L, Li M, Jiang P, Wang J, Li C. Advances in radiotherapy and immunity in hepatocellular carcinoma. J Transl Med 2023;21:526.
43. Su K, Wang F, Li X, et al. Effect of external beam radiation therapy versus transcatheter arterial chemoembolization for non-diffuse hepatocellular carcinoma (≥ 5 cm): a multicenter experience over a ten-year period. Front Immunol 2023;14:1265959.
44. Palma DA, Olson R, Harrow S, et al. Stereotactic ablative radiotherapy for the comprehensive treatment of oligometastatic cancers: long-term results of the SABR-COMET phase II randomized trial. J Clin Oncol 2020;38:2830-8.
45. Toesca DAS, Osmundson EC, von Eyben R, Shaffer JL, Koong AC, Chang DT. Assessment of hepatic function decline after stereotactic body radiation therapy for primary liver cancer. Pract Radiat Oncol 2017;7:173-82.
46. Upadhyay R, Doe N, Gokun Y, et al. Outcomes and predictors of toxicity after stereotactic body radiotherapy for child-pugh B or C hepatocellular carcinoma in a fiducial-less setting. Int J Radiat Oncol Biol Phys 2024;120:e489.
47. Sanford NN, Pursley J, Noe B, et al. Protons versus photons for unresectable hepatocellular carcinoma: liver decompensation and overall survival. Int J Radiat Oncol Biol Phys 2019;105:64-72.
48. Culleton S, Jiang H, Haddad CR, et al. Outcomes following definitive stereotactic body radiotherapy for patients with child-pugh B or C hepatocellular carcinoma. Radiother Oncol 2014;111:412-7.
49. Thomas TO, Hasan S, Small W Jr, et al. The tolerance of gastrointestinal organs to stereotactic body radiation therapy: what do we know so far? J Gastrointest Oncol 2014;5:236-46.
50. Klein J, Dawson LA, Jiang H, et al. Prospective longitudinal assessment of quality of life for liver cancer patients treated with stereotactic body radiation therapy. Int J Radiat Oncol Biol Phys 2015;93:16-25.
Cite This Article

How to Cite
Seth, L.; Stephans, K. L. Advances in external beam radiation for hepatocellular carcinoma, stereotactic body radiation therapy (SBRT) and particle therapy. Hepatoma. Res. 2024, 10, 52. http://dx.doi.org/10.20517/2394-5079.2024.91
Download Citation
Export Citation File:
Type of Import
Tips on Downloading Citation
Citation Manager File Format
Type of Import
Direct Import: When the Direct Import option is selected (the default state), a dialogue box will give you the option to Save or Open the downloaded citation data. Choosing Open will either launch your citation manager or give you a choice of applications with which to use the metadata. The Save option saves the file locally for later use.
Indirect Import: When the Indirect Import option is selected, the metadata is displayed and may be copied and pasted as needed.
About This Article
Special Issue
Copyright
Data & Comments
Data
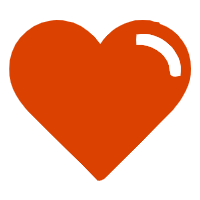
Comments
Comments must be written in English. Spam, offensive content, impersonation, and private information will not be permitted. If any comment is reported and identified as inappropriate content by OAE staff, the comment will be removed without notice. If you have any queries or need any help, please contact us at [email protected].