Impact of gut microbiome on immunotherapy resistance in hepatocellular carcinoma: a review article
Abstract
This article reviews the interactions between gut microbiota and cancer immunotherapies and discusses microbiota-based patient stratification strategies such as the prediction of response and the early recognition of toxic events. Additionally, it explores the emerging potential of microbiotherapy to enhance anti-neoplastic efficacy and alleviate toxicity, with the ultimate goal of developing personalized treatment regimens for patients with hepatocellular carcinoma (HCC).
Keywords
INTRODUCTION
The incidence of hepatocellular carcinoma (HCC) has alarmingly increased over the last few decades, with studies reporting a nearly 2- to 3-fold rise, particularly in developed nations[1]. This concerning trend is primarily attributed to the growing prevalence of chronic liver diseases, such as hepatitis B and C, metabolic-associated steatotic liver disease (most commonly related to obesity and insulin resistance), and alcohol-related liver disease[1-3]. Understanding the complex interplay among these factors and their contributions to the rising incidence of HCC is critical for implementing effective preventive strategies and improving patient outcomes.
In recent years, there has been a significant breakthrough in the treatment of HCC due to the emergence of immunotherapy, such as immune checkpoint inhibitors (ICI)[4]. Among these, Cytotoxic T-Lymphocyte Antigen 4 (CTLA-4) inhibitors (ipilimumab) and PD-1 inhibitors (nivolumab, pembrolizumab) have shown promising results in the management of HCC[5]. Although the mechanisms of action of these therapies have been reviewed elsewhere, a brief overview is as follows: the programmed death (PD)-1 protein is found on the surface of immune cells and belongs to the CD-28 protein family[4]. The binding of PD-1 to programmed death ligand (PDL)-1 or PDL-2 on target cells suppresses the immune system’s response, culminating in peripheral tolerance and the growth of malignant cells. Blocking the binding of PD-1 to its receptor can prevent this inhibitory signal and help the immune system mount an adaptive, targeted response[6].
Despite advancements in the treatment of HCC with the use of ICIs, outcomes remain poor for patients with advanced HCC, especially in the presence of metastases. In a landmark trial of Nevolumab, response rates were only 15%-20%[7], and in a phase 1b trial of atezolizumab and bevacizumab, the median progression-free survival was only 6.8 months[7,8]. Additionally, a significant proportion of patients experienced severe immune-related side effects[9]. Over one-half (56.5%) of patients who received atezolizumab and bevacizumab suffered Grade 3 or 4 adverse effects[8]. Immunotherapy resistance also poses a significant obstacle to successful treatment outcomes. This resistance can be attributed to several factors including tumor heterogeneity, genomic instability, and the suppressive tumor microenvironment (TME)[10]. Discovering biomarkers that can help predict clinical response to immunotherapy offers significant potential for optimizing patient selection and maximizing treatment outcomes[9]. In light of these findings, microbiotherapy is also undergoing evaluation as a potential antitumor strategy through the modulation of intestinal flora[11].
The gut microbiome plays a crucial role in modulating immune responses[12]. It has been observed that the gut microbiota can influence both innate and adaptive immune responses. The gut microbiota is involved in the development and maturation of the immune system, helping to educate and train immune cells to recognize and respond appropriately to pathogens and harmless antigens. Additionally, the gut microbiota can also impact immune responses by influencing the production of immune cells and molecules, such as cytokines, that regulate inflammation and immune activity. Increasing evidence suggests that the gut microbiome plays a significant role in age-related changes in immune system function and, therefore, has the potential to modulate immune responses in older adults, influencing their susceptibility to infections and chronic low-grade inflammation[13,14].
This article reviews the interactions between gut microbiota and cancer immunotherapies, as well as microbiota-based patient stratification strategies that may help predict not only responses to therapy but early recognition of toxic events. Additionally, it discusses the potential of microbiotherapy to enhance the anti-neoplastic effects of immunotherapy and alleviate toxicity, with the ultimate goal of developing personalized treatment regimens for patients with HCC.
GUT MICROBIOME AND THE IMMUNE SYSTEM
The human gut harbors a vast and diverse ecosystem of microorganisms collectively known as the gut microbiome. This intricate community of archaea, bacteria, fungi, and viruses plays a crucial role in numerous physiological processes, including digestion, metabolism, and immunology. Among these commensals, Bacteroidetes and Firmicutes are the dominant bacterial phyla, with Bacteroides, Prevotella, and Bifidobacterium being prominent genera. The interplay between the gut microbiome and the immune system is dynamic, with the goal of maintaining intestinal homeostasis to influence immune responses throughout the body [Figure 1].
Figure 1. Gut microbiome and the immune system. SCFAs: Short-chain fatty acids; PD-L1: programmed death ligand 1.
At the frontline of this interaction lies the intestinal barrier, a complex system comprising physical, chemical, and immunological components. This barrier enables the absorption of nutrients while preventing the translocation of harmful bacteria and their products into the bloodstream. Resident gut microbiota maintains barrier integrity by stimulating mucus production and preserving epithelial cell tight junctions. A healthy gut microbiome fosters a tolerogenic environment, suppressing excessive inflammation while maintaining the ability to mount effective responses against pathogens. Specific bacterial species can directly interact with immune cells, leading to their activation and cytokine production. For example, Bifidobacterium infantis induces the production of anti-inflammatory cytokines such as interleukin (IL)-10[15]. Bacteria can also influence the activation and differentiation of various immune cell populations, such as Th1, Th2, and Th17 cells, to modify the overall immune response toward specific targets.
Furthermore, the gut microbiome actively shapes the immune system through various mechanisms:
• Pattern recognition receptors (PRRs): Immune cells express PRRs that recognize specific molecular patterns on microbes. Commensal bacteria activate PRRs, triggering signaling pathways that promote immune tolerance and suppress inflammation. Conversely, PRRs can also identify pathogen-associated molecular patterns, initiating an inflammatory response to eliminate invaders.
• Metabolite production: Gut bacteria produce a variety of metabolites, including short-chain fatty acids (SCFAs) such as acetate, propionate, and butyrate. SCFAs can activate the immune system via receptor-mediated and epigenetic mechanisms such as inflammasome activation, host defense peptide synthesis, and cytokine synthesis. SCFAs also promote the development of regulatory T cells (Tregs), which are essential for maintaining immune tolerance and preventing excessive inflammation [Figure 2].
• Direct cell-cell interactions: Certain bacterial species also directly interact with immune cells to modulate their activation and cytokine production. For example, certain commensals induce the production of the anti-inflammatory cytokine IL-10, which contributes to immune homeostasis[16].
When this delicate balance is disrupted by factors such as diet, antibiotics, or stress, it leads to dysbiosis, a state characterized by an altered composition and diversity of the gut microbiome. Dysbiosis, in turn, can be linked to:
• Increased intestinal permeability: A weakened gut barrier allows for the translocation of bacteria and their products into the bloodstream, triggering systemic inflammation.
• Dysregulation of immune responses: Altered microbial composition can skew the immune response toward excessive inflammation, contributing to the development of autoimmune conditions such as inflammatory bowel disease (IBD).
• Impaired immune function: Dysbiosis can weaken the immune system’s ability to combat infections and increase the susceptibility to various pathogens[15].
Recent advancements in sequencing technologies have revolutionized our understanding of the gut microbiome. Metagenomic and 16S rRNA sequencing enable detailed characterization of the microbial composition and identification of specific bacterial species with functional significance. This knowledge has paved the way for the development of novel therapeutic strategies aimed at modulating the gut microbiome for disease prevention and treatment[17].
The gut and the liver are intimately connected organs, forming a bidirectional communication network known as the gut-liver axis, a key component of which is the gut microbiome. Gut bacteria help to metabolize dietary components, producing metabolites such as SCFAs, which are transported to the liver. SCFAs influence various liver functions, including lipid metabolism, bile acid synthesis, and gluconeogenesis[18]. As gut bacteria can also modulate the immune system, they can potentially contribute to the development of chronic inflammatory conditions such as metabolic dysfunction-associated steatotic liver disease (MASLD)[19].
The gut-liver axis also involves the exchange of various bacterial products, including lipopolysaccharides (LPS) and peptidoglycans. These components can translocate from the gut lumen into the portal circulation and trigger inflammatory responses in the liver. In a healthy gut, the intestinal barrier restricts the translocation of these potentially harmful products, but during dysbiosis, there is translocation of bacterial products, which leads to hepatic inflammation and injury[13]. The liver itself also plays a crucial role in regulating systemic immune responses and thus impacts the overall gut immune environment[20].
Understanding the gut-liver axis is particularly relevant in the context of MASLD, a growing public health concern characterized by excessive fat accumulation in the liver. Studies have shown a strong association between gut dysbiosis and MASLD progression. Specific bacterial taxa have been linked to increased inflammation, insulin resistance, and steatosis, key features of MASLD pathogenesis[21].
Targeting the gut-liver axis offers promising avenues for therapeutic interventions in MASLD and other liver diseases. Prebiotics, probiotics, and fecal microbiota transplantation (FMT) are being explored as means to modulate the gut microbiome and potentially improve liver health, with current research aimed at identifying specific bacterial strains or metabolites that could serve as therapeutic targets for preventing or treating liver diseases[22].
In conclusion, the gut-liver axis is a dynamic and multifaceted communication system with profound implications for health and disease. Understanding the intricate interplay between the gut microbiome, the liver and the immune system is crucial for deciphering the mechanisms underlying liver pathology and developing novel therapeutic strategies that target this interconnected network to promote overall well-being.
GUT MICROBIOME AND IMMUNOTHERAPY
Standard cytotoxic chemotherapeutic agents are not effective in managing HCC. Multi-kinase inhibitors such as sorafenib and regorafenib are more effective against HCC and used as first- and second-line therapy, respectively, with lenvatinib as a third-line salvage agent. Unfortunately, these drugs are only recommended for advanced disease cases where no other intervention is possible, and they extend survival by just a few weeks or months[23].
Immunotherapy has become a promising alternative in this field of limited therapeutic options. However, a significant portion of patients exhibit resistance to these therapies, highlighting the need for personalized approaches. Emerging research sheds light on the crucial role of the gut microbiome in influencing immunotherapy efficacy across various cancers. Several lines of evidence point toward a clear link between the gut microbiome and immunotherapy response. Studies consistently demonstrate that patients with diverse and healthy gut microbial communities tend to experience better outcomes with ICIs, the most widely used form of immunotherapy. Conversely, dysbiosis is associated with poorer response rates and increased treatment resistance[24,25].
The underlying mechanisms by which the gut microbiome influences immunotherapy efficacy are multifaceted and involve intricate communication with the immune system. Gut bacteria can directly interact with and activate immune cells like dendritic cells (DCs) and T lymphocytes, shaping their antitumor response. Recent studies suggest butyrate, an important SCFA and gut microbial metabolite, can potentially enhance the effectiveness of anti-PD-1 therapy by increasing the infiltration of CD4+ and CD8+ T cells in tumors in chimeric humanized mice colonized with microbiota from colon carcinoma patients[26,27]. These studies suggest that in patients who do not respond to anti-PD-1 therapy, supplementing butyrate before starting treatment may reclaim treatment response. Additionally, another study indicates that SCFA butyrate could boost CD8+ T cell activity via the IL-12 pathway[28].
The intestinal microbiota profile of patients with HCC is observed to be abundant in proinflammatory bacteria and deficient in SCFA-producing organisms. Production of IL17, a proinflammatory cytokine produced by T helper 17 (Th17), is a postulated mechanism for the development of HCC. Th17 subtype cells and IL17 are found in greater abundance in serum and tumor samples of patients with HCC, with Th17 cells derived from the gut prior to their differentiation following interactions with gut microbiota[29]. Reducing gut microbiomes with antibiotics can lower the burden of bacteria that transform primary bile acids into secondary bile acids. Primary bile acids are hypothesized to improve immune response to tumors by increasing the expression of CXCL16, a membrane-bound chemokine and mediator of innate immunity. This pathway is crucial in the activation of natural killer T (NKT) cells that hinder the development of both B16 and EL4 tumor cells[30].
Fecal calprotectin, a well-known biomarker of gut inflammation, has also been noted to vary with the course of immune checkpoint inhibitor therapy[31]. A study of HCC patients who responded well to tremelimumab and durvalumab showed lower levels of fecal calprotectin and more Akkermansia bacteria before treatment, compared to poor responders, with the ratio of Akkermansia to Enterobacteriaceae (AE ratio) now being utilized as a marker of intestinal dysbiosis[30]. This suggests a connection between Akkermansia and a better response to ICI treatment, since Akkermansia has potential anti-inflammatory properties. Another study found patients with high levels of Faecalibacterium had significantly longer progression-free survival as opposed to patients with low levels, while those with high levels of Bacteroidales had a shorter progression-free survival[31].
Clinical trials are actively investigating the potential for manipulating the gut microbiome to improve immunotherapy outcomes. FMT, the process of transferring healthy gut bacteria from a donor to a recipient, has shown promising results in enhancing ICI efficacy in some cancer patients[32]. Prebiotics and probiotics are also being explored as potential therapeutic agents to modulate the gut microbiome and improve immune responses to cancer.
In conclusion, the gut microbiome emerges as a critical and dynamic player in shaping immunotherapy efficacy in various cancers. Understanding the intricate interplay between gut bacteria, the immune system, and the TME holds immense potential for optimizing cancer immunotherapy strategies. Further research into specific bacterial strains and their metabolites as therapeutic targets, coupled with clinical trials of microbiome-modulating interventions, paves the way for personalized approaches to cancer treatment that harness the power of the gut microbiome to improve patient outcomes.
GUT microbiome and immunotherapy resistance in HCC
While a balanced microbiome can support antitumor immunity, dysbiosis can dampen the immune response, leading to HCC progression and treatment resistance[33]. Following are the key mechanisms by which the gut microbiome contributes to this effect[33,34,35,36,37]:
Modulation of the TME
Immune cell suppression: Gut bacteria can directly interact with immune cells within the TME, promoting the expansion of Tregs. Tregs suppress the activation and cytotoxic activity of T lymphocytes, hindering their ability to eliminate tumor cells. Certain gut bacteria can induce the accumulation and activation of Myeloid-derived suppressor cells (MDSCs), another type of immunosuppressive cell. MDSCs further suppress T cell function and promote tumor immune escape.
Inflammatory milieu: Dysbiosis can lead to increased production of proinflammatory cytokines and chemokines within the TME. This inflammatory environment can impair the infiltration and function of cytotoxic T lymphocytes (CTLs), further weakening the antitumor immune response.
Modulation of antigen presentation
Reduced antigen presentation: Dysbiosis can impair the function of antigen-presenting cells (APCs) such as DCs, leading to decreased presentation of tumor antigens to T lymphocytes. This dampens the T cell response against tumor cells.
Activation of PRRs
PRR overactivation: Certain bacterial components can overstimulate PRRs, such as Toll-like receptors (TLRs), leading to chronic inflammation and the production of immunosuppressive cytokines. This chronic inflammation can hinder the efficacy of ICIs.
Direct interaction with immune checkpoint molecules
PD-L1 expression: Certain bacterial components and metabolites can directly induce the expression of PD-L1 on tumor cells, a ligand for the PD-1 immune checkpoint receptor. This interaction suppresses T cell activation and promotes tumor immune escape.
Metabolic alterations
SCFA depletion: SCFAs, particularly butyrate, are bacterial metabolites with potent immunomodulatory properties. Decreased SCFA production due to dysbiosis can impair the differentiation and activation of T cells, leading to a weakened immune response against tumor cells.
Bile acid metabolism: Gut bacteria play a crucial role in bile acid metabolism. Specific bacteria can convert primary bile acids into secondary bile acids with immunosuppressive properties. This altered bile acid composition within the TME can contribute to immune suppression and dampen the antitumor response.
Bacterial translocation and endotoxemia
Gut barrier disruption: Dysbiosis can lead to increased intestinal permeability, allowing bacterial translocation from the gut lumen into the systemic circulation. This influx of bacteria and their components, such as LPS, can trigger systemic inflammation and activate suppressive immune pathways, hindering the efficacy of immunotherapy.
Bacterial-derived metabolites
Tryptophan metabolism: Certain gut bacteria metabolize tryptophan into metabolites such as kynurenine, which can suppress T cell activation and promote Treg expansion, creating an immunosuppressive environment within the TME.
Dysbiosis-induced immunosuppressive pathways
Activation of aryl hydrocarbon receptor (AhR): Specific gut bacteria can activate the AhR pathway, leading to the production of immunosuppressive molecules that inhibit T cell function and promote tumor growth.
Understanding these mechanisms is crucial for developing strategies to overcome dampened antitumor immunity in patients with HCC. FMT with a microbiome enriched in beneficial bacteria, modulation of the gut microbiota through prebiotics and probiotics, and targeting specific bacterial pathways offer promising avenues for restoring a gut microbiome conducive to a robust antitumor immune response and improving the efficacy of immunotherapy.
FUTURE DIRECTIONS AND THERAPEUTIC IMPLICATIONS
The management of HCC remains a formidable challenge, with ICIs offering promising yet variable responses. Emerging research unveils the gut microbiome as a crucial player influencing ICI efficacy, opening doors for novel strategies to improve HCC treatment outcomes. Table 1 summarizes the current and recent clinical trials investigating the role of microbiota in the treatment and outcomes of HCC.
Current and recent clinical trials on the role of microbiota in HCC treatment and outcomes
Study | Date of completion | Country | Details |
Pinter | Ongoing (expected to complete in 2025) | Austria | This trial aims to study FMT combined with atezolizumab plus bevacizumab in patients who have previously failed immunotherapy for HCC |
Yu et al.[38] | Published in 2023 | China | 123 patients with HCC underwent 16S sequencing of stool samples. Compared to those with delayed recovery, patients with normal liver function recovery had enrichment of Bifidobacterium species. Patients with delayed recovery of liver functions had short long-term survival |
Han | Ongoing (expected to be completed in 2025) | China | Intestinal microbiome multi-omics analysis to evaluate the predictive value of microbial-derived proteins and metabolites on treatment efficacy and patient outcomes |
Cremona | Completed in 2023 - results not published | Argentina | Role of probiotics in the prevention of hepatocellular carcinoma in cirrhosis |
Sun | Ongoing (expected to be completed in 2024) | China | This study evaluated intestinal flora and found that Proteobacteria and Actinobacteria were the most abundant phyla in the HCC TME |
Greten | Withdrawn | US | This study aimed to evaluate the gut microbiota in patients undergoing HCC resection at the Mount Sinai Medical Center |
Xie | Ongoing (expected to be completed in 2024) | China | Patients with advanced HCC treated with carrilizumab and apatinib mesylate will be divided into two groups. Intervention arm given oral bifidobacterium. 16S sequencing will be used to assess variations in the composition of gut microbiota. Clinical data will be used to establish any relationship with treatment response. |
Roussel et al.[39] | Published in 2022 | France | No significant change in endotoxin levels, postoperative liver function, or overall complication rates observed in patients with compensated cirrhosis treated with probiotics prior to liver resection for HCC |
Studies have identified distinct gut microbial signatures associated with resistance to ICIs in HCC patients. These communities may suppress T cell activity, promote the expansion of Tregs, and generate metabolites that dampen the antitumor immune response[40]. Manipulating the gut microbiome to foster a more immunotherapy-supportive environment holds immense potential for the treatment of HCC.
Several promising avenues exist for achieving this:
1. Fecal microbiota transplantation: Introducing a microbiome enriched in beneficial bacteria through FMT has shown promise in reversing ICI resistance in other cancers[32]. Adapting this approach for HCC patients could potentially improve their response to therapy.
2. Prebiotics and probiotics: Targeted use of prebiotics and probiotics can selectively enrich beneficial bacterial populations within the gut. This could help restore a microbiome conducive to a robust antitumor immune response[41].
3. Dietary modulation: Dietary interventions, such as increasing fiber intake and incorporating fermented foods, can promote the growth of beneficial bacteria and potentially enhance ICI efficacy.
By manipulating the gut microbiome, we can potentially:
• Enhance T cell activation and function: Promoting the growth of bacteria that stimulate T cell responses can lead to a more robust antitumor immune attack.
• Reduce the activity of immunosuppressive pathways: Suppressing the production of metabolites and bacterial components that dampen the immune response can create a more favorable environment for ICI therapy.
• Personalize treatment strategies: Identifying individual gut microbiome signatures associated with ICI response could pave the way for personalized therapeutic approaches tailored to each patient.
However, several challenges remain, including:
• Standardization of protocols: Optimal donor selection, fecal processing methods, and FMT delivery routes need further optimization for HCC patients.
• Clinical trial design: Rigorous clinical trials are necessary to definitively establish the efficacy of prebiotics in conjunction with ICIs for HCC treatment.
• Optimize prebiotic selection and dosage: Identifying the most effective prebiotic combinations and dosages tailored to individual gut microbiota profiles is crucial for maximizing their benefits.
• Long-term efficacy: The long-term durability of the transplanted microbiome and its impact on sustained antitumor immunity require further investigation.
• Safety considerations: Potential risks associated with FMT, such as the transmission of antibiotic-resistant bacteria, necessitate careful donor screening and vigilant monitoring.
Although many studies have been conducted on the alteration of gut microbiota in HCC, our understanding of the underlying mechanisms is still incomplete. The majority of clinical studies are single-center studies and are constrained by small population samples, affecting the generalizability of their findings. In addition, analyzing different factors such as diverse etiologies of liver disease, stage of cirrhosis, varied diet, antibiotic exposure, and pattern of alcohol consumption can lead to confounding factors that can result in significant dissimilarities in the gut microbiome. Therefore, to overcome these limitations, multi-center studies with appropriately powered sample sizes are necessary[42].
CONCLUSION
The gut microbiome, the complex ecosystem of microorganisms residing within the intestines, plays a crucial role in shaping the immune response to cancer immunotherapy. In HCC patients, specific gut microbial signatures associated with resistance to ICIs have been identified. These microbiomes often harbor bacteria that suppress T cell activity, promote regulatory T cell expansion, and generate metabolites that dampen the antitumor immune response. This dysbiosis contributes to ICI resistance and poorer treatment outcomes. Therefore, manipulating the gut microbiome through strategies such as FMT, prebiotics, and probiotics holds immense potential to improve ICI efficacy and potentially transform the treatment landscape for HCC patients.
While the potential of gut microbiome modulation for HCC immunotherapy is significant, further research is crucial to optimize its clinical application. Identifying reliable predictive biomarkers, determining the most effective microbiome-modulating strategies for individual patients, and ensuring long-term safety and efficacy are essential steps toward fully realizing the power of the gut microbiome in this exciting new frontier of HCC management.
DECLARATIONS
Authors’ contributions
Writing, editing and revision: Shah AR, Mukherjee S
Availability of data and materials
Not applicable.
Financial support and sponsorship
None.
Conflicts of interest
All authors declared that there are no conflicts of interest.
Ethical approval and consent to participate
Not applicable.
Consent for publication
Not applicable.
Copyright
© The Author(s) 2024.
REFERENCES
1. El-Serag HB, Rudolph KL. Hepatocellular carcinoma: epidemiology and molecular carcinogenesis. Gastroenterology 2007;132:2557-76.
2. Bruix J, Cheng AL, Meinhardt G, Nakajima K, De Sanctis Y, Llovet J. Prognostic factors and predictors of sorafenib benefit in patients with hepatocellular carcinoma: analysis of two phase III studies. J Hepatol 2017;67:999-1008.
3. ZM, Koenig AB, Abdelatif D, Fazel Y, Henry L, Wymer M. Global epidemiology of nonalcoholic fatty liver disease-Meta-analytic assessment of prevalence, incidence, and outcomes. Hepatology 2016;64:138-49.
4. Huang Y, Liang D, Liu J, Zeng J, Zeng Y. The breakthroughs in cancer immune checkpoint based therapy: a review of development in immune checkpoint study and its application. Comb Chem High Throughput Screen 2017;20:430-39.
5. Lee JB, Kim HR, Ha SJ. Immune checkpoint inhibitors in 10 years: contribution of basic research and clinical application in cancer immunotherapy. Immune Netw 2022;22:e2.
6. Nguyen LT, Ohashi PS. Clinical blockade of PD1 and LAG3--potential mechanisms of action. Nat Rev Immunol 2015;15:45-56.
7. El-Khoueiry AB, Sangro B, Yau T, et al. Nivolumab in patients with advanced hepatocellular carcinoma (CheckMate 040): an open-label, non-comparative, phase 1/2 dose escalation and expansion trial. Lancet 2017;389:2492-502.
8. Finn RS, Qin S, Ikeda M, et al; IMbrave150 Investigators. Atezolizumab plus bevacizumab in unresectable hepatocellular carcinoma. N Engl J Med 2020;382:1894-905.
9. Asrani SK, Devarbhavi H, Eaton J, Kamath PS. Burden of liver diseases in the world. 2019;70:151-71. Available from: https://www.journal-of-hepatology.eu/article/S0168-8278(18)32388-2/abstract. [Last accessed on 7 Nov 2024].
10. Fu J, Wang H. Precision diagnosis and treatment of liver cancer in China. Cancer Lett 2018;412:283-8.
11. Shi Z, Li H, Song W, Zhou Z, Li Z, Zhang M. Emerging roles of the gut microbiota in cancer immunotherapy. Front Immunol 2023;14:1139821.
12. Ragonnaud E, Biragyn A. Gut microbiota as the key controllers of “healthy” aging of elderly people. Immun Ageing 2021;1:2.
13. Badal VD, Vaccariello ED, Murray ER, et al. The gut microbiome, aging, and longevity: a systematic review. Nutrients 2020;12:3759.
14. Claesson MJ, Cusack S, O'Sullivan O, et al. Composition, variability, and temporal stability of the intestinal microbiota of the elderly. Proc Natl Acad Sci USA 2011;108 Suppl 1:4586-91.
15. Ni Y, Lu M, Xu Y, et al. The role of gut microbiota-bile acids axis in the progression of non-alcoholic fatty liver disease. Front Microbiol 2022;13:908011.
16. Renz H, von Mutius E, Brandtzaeg P, Cookson WO, Autenrieth IB, Haller D. Gene-environment interactions in chronic inflammatory disease. Nat Immunol 2011;12:273-7.
17. Ursell LK, Clemente JC, Rideout JR, Gevers D, Caporaso JG, Knight R. The interpersonal and intrapersonal diversity of human-associated microbiota in key body sites. J Allergy Clin Immunol 2012;129:1204-8.
18. Cani PD, Bibiloni R, Knauf C, et al. Changes in gut microbiota control metabolic endotoxemia-induced inflammation in high-fat diet-induced obesity and diabetes in mice. Diabetes 2008;57:1470-81.
19. Henao-Mejia J, Elinav E, Jin C, et al. Inflammasome-mediated dysbiosis regulates progression of NAFLD and obesity. Nature 2012;482:179-85.
20. Paolella G, Mandato C, Pierri L, Poeta M, Di Stasi M, Vajro P. Gut-liver axis and probiotics: their role in non-alcoholic fatty liver disease. World J Gastroenterol 2014;20:15518-31.
21. Li R, Mao Z, Ye X, Zuo T. Human gut microbiome and liver diseases: from correlation to causation. Microorganisms 2021;9:1017.
22. Ji Y, Yin Y, Sun L, Zhang W. The molecular and mechanistic insights based on gut-liver axis: nutritional target for non-alcoholic fatty liver disease (NAFLD) improvement. Int J Mol Sci 2020;21:3066.
23. Vaziri F, Colquhoun S, Wan YY. Hepatocellular carcinoma immunotherapy: the impact of epigenetic drugs and the gut microbiome. Liver Res 2020;4:191-8.
24. Matson V, Chervin CS, Gajewski TF. Cancer and the Microbiome-Influence of the Commensal Microbiota on Cancer, Immune Responses, and Immunotherapy. Gastroenterology 2021;160:600-13.
25. Gopalakrishnan V, Spencer CN, Nezi L, et al. Gut microbiome modulates response to anti-PD-1 immunotherapy in melanoma patients. Science 2018;359:97-103.
26. Lu Y, Yuan X, Wang M, et al. Gut microbiota influence immunotherapy responses: mechanisms and therapeutic strategies. J Hematol Oncol 2022;15:47.
27. Zhang SL, Mao YQ, Zhang ZY, et al. Pectin supplement significantly enhanced the anti-PD-1 efficacy in tumor-bearing mice humanized with gut microbiota from patients with colorectal cancer. Theranostics 2021;11:4155-70.
28. He Y, Fu L, Li Y, et al. Gut microbial metabolites facilitate anticancer therapy efficacy by modulating cytotoxic CD8+ T cell immunity. Cell Metab 2021;33:988-1000.e7.
29. Wan MLY, El-Nezami H. Targeting gut microbiota in hepatocellular carcinoma: probiotics as a novel therapy. Hepatobiliary Surg Nutr 2018;7:11-20.
30. Ponziani FR, De Luca A, Picca A, et al. Gut dysbiosis and fecal calprotectin predict response to immune checkpoint inhibitors in patients with hepatocellular carcinoma. Hepatol Commun 2022;6:1492-501.
31. Li L, Ye J. Characterization of gut microbiota in patients with primary hepatocellular carcinoma received immune checkpoint inhibitors: a Chinese population-based study. Medicine 2020;99:e21788.
32. Davar D, Dzutsev AK, McCulloch JA, et al. Fecal microbiota transplant overcomes resistance to anti-PD-1 therapy in melanoma patients. Science 2021;371:595-602.
33. McQuade JL, Daniel CR, Helmink BA, Wargo JA. Modulating the microbiome to improve therapeutic response in cancer. Lancet Oncol 2019;20:e77-91.
34. Pei B, Peng S, Huang C, Zhou F. Bifidobacterium modulation of tumor immunotherapy and its mechanism. Cancer Immunol Immunother 2024;73:94.
35. Park JS, Gazzaniga FS, Kasper DL, Sharpe AH. Microbiota-dependent regulation of costimulatory and coinhibitory pathways via innate immune sensors and implications for immunotherapy. Exp Mol Med 2023;55:1913-21.
36. Gunjur A, Shao Y, Rozday T, et al. A gut microbial signature for combination immune checkpoint blockade across cancer types. Nat Med 2024;30:797-809.
37. Xue P, Fu J, Zhou Y. The aryl hydrocarbon receptor and tumor immunity. Front Immunol 2018;9:286.
38. Yu J, Zhu P, Shi L, et al. Bifidobacterium longum promotes postoperative liver function recovery in patients with hepatocellular carcinoma. Cell Host Microbe 2024;32:131-144.e6.
39. Roussel E, Brasse-Lagnel C, Tuech JJ, et al. Influence of probiotics administration before liver resection in patients with liver disease: a randomized controlled trial. World J Surg 2022;46:656-65.
40. Mao J, Wang D, Long J, et al. Gut microbiome is associated with the clinical response to anti-PD-1 based immunotherapy in hepatobiliary cancers. J Immunother Cancer 2021;9:e003334.
41. Russo E, Fiorindi C, Giudici F, Amedei A. Immunomodulation by probiotics and prebiotics in hepatocellular carcinoma. World J Hepatol 2022;14:372-85.
Cite This Article
How to Cite
Shah, A. R.; Mukherjee, S. Impact of gut microbiome on immunotherapy resistance in hepatocellular carcinoma: a review article. Hepatoma. Res. 2024, 10, 48. http://dx.doi.org/10.20517/2394-5079.2024.76
Download Citation
Export Citation File:
Type of Import
Tips on Downloading Citation
Citation Manager File Format
Type of Import
Direct Import: When the Direct Import option is selected (the default state), a dialogue box will give you the option to Save or Open the downloaded citation data. Choosing Open will either launch your citation manager or give you a choice of applications with which to use the metadata. The Save option saves the file locally for later use.
Indirect Import: When the Indirect Import option is selected, the metadata is displayed and may be copied and pasted as needed.
About This Article
Copyright
Data & Comments
Data
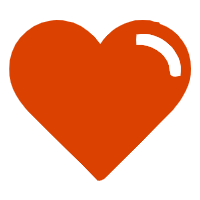
Comments
Comments must be written in English. Spam, offensive content, impersonation, and private information will not be permitted. If any comment is reported and identified as inappropriate content by OAE staff, the comment will be removed without notice. If you have any queries or need any help, please contact us at support@oaepublish.com.