Shedding light on the role of LAG-3 in hepatocellular carcinoma: unraveling immunomodulatory pathways
Abstract
Hepatocellular carcinoma (HCC) stands as a primary malignant liver tumor characterized by chronic inflammation and complex alterations within the tumor microenvironment (TME). Lymphocyte activation gene 3 (LAG-3), also known as CD223, has gained prominence as a potential next-generation immune checkpoint, maintaining continuous expression in response to persistent antigen exposure within the TME, warranting our attention. In patients with HCC, LAG-3 expression on T cells, regulatory T cells (Tregs), and natural killer (NK) cells contributes to immune evasion, while high expression of LAG-3 leads to increased angiogenesis and poor prognosis. By interacting with major histocompatibility complex class II molecules, LAG-3 promotes T cell exhaustion and suppresses antitumor responses, often in collaboration with other immune checkpoints like programmed cell death protein 1 (PD-1), while on Tregs and NK cells, LAG-3 modulates their suppressive functions, indirectly facilitating tumor immune escape. LAG-3 expression may offer prognostic insights, correlating with disease progression and outcomes in HCC patients, while various preclinical studies highlight the potential of LAG-3-targeted therapies in reinvigorating immune responses against HCC, with a few combination approaches targeting LAG-3 alongside other checkpoints demonstrating synergistic effects in restoring T cell function. Therefore, harnessing LAG-3 as a therapeutic target holds promise for enhancing antitumor immunity and potentially improving HCC treatment outcomes. Our narrative review aims to delve into the full spectrum of LAG-3 signaling in HCC, with the goal of a better understanding of the pathophysiological and immunological basis of its use to arrest HCC growth and development.
Keywords
INTRODUCTION
Hepatocellular carcinoma (HCC) stands out as the predominant form of primary liver cancer[1]. On a global scale, liver cancer is the fourth leading cause of cancer-related mortality, accounting for 782,000 deaths, holding the sixth position in terms of newly diagnosed cases, and affecting 841,000 patients annually[2]. Additionally, estimations regarding liver cancer-related death predict a significant surge in its impact on cancer-related mortality by 2030, demonstrating a tendency to become the third primary cause of such fatalities[2,3]. In most countries, men experience incidence rates two to four times higher than women[4], whereas a direct correlation between the incidence rate of HCC and age has been established in most populations persisting until approximately 75 years old, although the median age of diagnosis is considered to be at a younger age. The process of hepatocellular carcinogenesis involves angiogenesis, chronic inflammation, and complex modifications within the macro- and micro-environment of the tumor[5-7]. This initiation is driven by intrinsic factors, comprising inherited or acquired genetic mutations, as well as extrinsic factors such as chronic alcohol consumption, smoking, nonalcoholic fatty liver disease (NAFLD) and hepatitis B virus (HBV), hepatitis C virus (HCV), and hepatitis D virus (HDV)[8-10]. These factors assume crucial roles in the development of HCC by triggering alterations in mature hepatocytes or stem cells, leading to processes such as apoptosis, cell proliferation, dysplasia, and eventually neoplasia[11,12].
HCC primarily emerges in a cirrhotic liver where both repeated inflammation and fibrogenesis are prevalent, while the interplay between them predisposes to liver dysplasia and, consequently, malignant transformation. Signaling pathways including Wnt/β-catenin, P53/cell cycle regulation, oxidative stress, and epigenetic modifiers undergo frequent mutations in HCC[13,14]. Immune checkpoint molecules are necessary for immune regulation and the preservation of immune balance as they become upregulated during immune cell activation[15], while their role in inhibiting immunopathological responses contributes significantly to the maintenance of self-tolerance[16]. Monoclonal antibodies (mAbs) directed at inhibitory receptors (IRs) such as cytotoxic T-lymphocyte antigen 4 (CTLA4) and programmed cell death protein-1 (PD-1) have shown substantial clinical efficacy in certain types of tumors, highlighting the potential role of targeted drug therapies in HCC[17]. Lymphocyte activation gene-3 (LAG-3) is a prominent member of the inhibitory receptor family, with extensive expression observed across various immune cell types[18]. A recently discovered primary ligand for LAG-3, fibrinogen-like protein 1 (FGL1), has been confirmed to be abundantly produced by human tumor cells, while increased levels of FGL1 have been strongly associated with an unfavorable prognosis and resistance to therapy[19]. Most importantly, however, concomitant blockade of LAG-3 and PD-1 receptors has demonstrated remarkable synergistic effects in boosting the immune response in clinical trials[20], indicating that LAG-3-related treatment options could contribute to achieving better outcomes for patients struggling with HCC. Even though LAG-3 is acknowledged as a significant inhibitory receptor, its biology and mechanism of action are yet to be clearly defined.
To date, most research regarding LAG-3 has put the emphasis on its implication in T cell dysfunction and its negative regulation of the immune response in various types of cancer and viral infections, but the function and ligands of LAG-3 in the setting of HCC have not been thoroughly defined yet. Our narrative review aims to delve into the potential role of LAG-3 signaling in HCC, aiming for a better understanding of the pathophysiological and immunological basis of its use to arrest HCC growth and development. In spite of the presence of many clinical trials regarding LAG-3, the expression of the FGL1/LAG-3 pathway and its association with PD-1 ligand are yet to be clearly defined in HCC. Enhanced comprehension of the role of LAG-3 in HCC could confer valuable insights into its future management, potentiating the development of novel therapeutic modalities.
UNDERSTANDING LAG-3 SIGNALING
A deeper understanding of the molecular structure, interactions, and signaling of LAG-3 will provide valuable insight into targeting LAG-3. In more detail, LAG-3, also known as CD223, is a cell surface molecule with significant involvement in the functions of various immune cells. It was first discovered by Triebel et al. in 1990 as a new 498-amino acid type I transmembrane protein present on activated human natural killer (NK) cells and T cell lines[18]. The LAG-3 locus is situated on chromosome 12 (12p13.32) in humans and chromosome 6 in mice, demonstrating high structural homology with CD4. The protein structure of LAG-3 can be further subdivided into extracellular, transmembrane, and intracellular components. Initially, the structural insight of LAG-3 provided important information regarding the molecular structure of the LAG-3 ectodomain (D1-D4) and demonstrated that LAG-3 forms a homodimer, with a central axis via a hydrophobic D2 domain with the rest of the domains forming a V-shaped architecture[21]. Moreover, LAG-3 ectodomains were recognized as dimers via the D2 domain, while the binding sites for FGL1 and major histocompatibility complex class II (MHCII) occurred via the flexible loop 2 region in the LAG-3 D1 domain. Afterwards, the main pathway of LAG-3 inhibitory signaling was found, demonstrating the presence of a relationship between LAG-3 and T cell receptor (TCR)-CD3 at the immunological synapse, inhibiting TCR signaling via the co-receptor-Lck signaling pathway[22]. In more detail, LAG-3 was demonstrated to function independently of MHCII and that it alternatively utilized the TCR-CD3 complex as a ligand inside the synapse, leading to disconnection of Lck from CD4 and CD8
Despite existing gaps in our knowledge and comprehension of LAG-3 biology, immunotherapeutics against LAG-3 have demonstrated their utility in restoring T cell function. The extracellular segment of LAG-3 is comprised of four immunoglobulin (Ig)-G domains (D1-D4) with folding patterns closely resembling those of CD4, while LAG-3 includes an individual short amino acid sequence termed "extra loop", in the D1 domain exhibiting a higher affinity to MHCII molecules compared to CD4[23]. Furthermore, the intracellular segment of LAG-3 is relatively short, featuring a distinctive motif (KIEELE) required for modulating T cell function[24]. Notably, transmembrane and cytoplasmic regions do not exhibit many similarities to CD4, suggesting that even though both genes have probably evolved from a pre-existing common evolutionary ancestor, they diverged early in evolution, explaining the differences between those molecules, indicating the unique signaling and inhibitory function of LAG-3. LAG-3 is present in most activated CD4 differentiation subsets including T helper cell type 1 (Th1), but is absent in T helper cell type 2 (Th2)[25]. It is also widely expressed in CD8+ T cells, regulatory T cells (Tregs), B cells, and NK cells[26]. Secretion of specific interleukins is associated with higher expression of LAG-3 molecules in T cell populations. Cytokines like interleukin (IL)-2 and IL-12 enhance LAG-3 expression on activated T cells[27], with its presence being also linked to upregulated IL-10 production[28], Moreover, the surface expression of LAG-3 protein and its shedding by activated CD4 T cells greatly aligns with interferon (IFN)-γ production, whereas LAG-3 expression shows an inverse correlation with IL-4 secretion[25]. Besides various blood cell types, LAG-3 messenger RNA (mRNA) was also identified in the medulla of the thymus gland and at the cerebellum, indicating a potential association of LAG-3 cellular signaling in conditions like Parkinson's disease[29].
While LAG-3 evidently assumes a negative regulatory function in managing T cell activation and function, the precise mechanisms of LAG-3 signal transduction are not yet fully understood. Initially, it was demonstrated that the high affinity between LAG-3 and MHCII elucidated the role of LAG-3 in T cell activation. MHCII molecules are recognized as the primary ligands for LAG-3, forming a stable interaction predominantly through the D1 domain of the extracellular segment. This binding is considered to exert a negative regulatory effect on T cell activation and cytokine production[30]. According to this hypothesis, LAG-3-Ig fusion proteins function as rivals in CD4/MHCII-dependent cellular adhesion assays[31], modulating the immune system response by intervening in the TCR signaling pathway. On the other hand, the possible role of the tailless LAG-3 molecules as competitors to CD4/MHCII ligands binding is highly disputed, and counterarguments propose that LAG-3’s cytoplasmic domain plays a key role in CD4 T cell activation through a single lysine residue (K468) within the KIEELE motif of the intracellular segment of the LAG-3 molecule[19]. The LAG-3 signaling pathway also plays a crucial role in dendritic cells (DCs). When LAG-3 engages with MHCII, it triggers an ITAM-mediated inhibitory signaling pathway. This pathway involves the Fc region of IgG (FcγR) and extracellular signal-regulated kinase (ERK)-mediated recruitment of the tyrosine phosphatase SHP-1, leading to the suppression of DC maturation and immune-stimulatory capacity. Notably, the cytoplasmic tailless form of LAG-3 alone was sufficient for inhibition, indicating that MHCII signaling in DCs, rather than LAG-3 signaling within T cells, was essential for LAG-3-mediated suppression of DC maturation[32]. Despite discrepancies in the proposed signaling mechanisms, there is substantial evidence supporting the idea that the binding of LAG-3 to MHCII leads to cancer cell evasion from apoptosis by facilitating the aggregation of tumor-specific CD4 T cells[33], indicating its unique role in the physiological processes underlying carcinogenesis [Figure 1].
Figure 1. (A) MHCII is one of the main ligands of LAG-3 and the inhibition of LAG-3 in tumor cells is a potential therapeutic option for patients resistant to PD-L1 immunotherapy. The LAG-3 protein structure consists of an extracellular, a transmembrane, and a cytoplasmic part. The extracellular part is composed of four IgG domains: D1, which contains a loop domain rich in proline and an in-chain species-specific disulfide bond, and D2-D4. In the transmembrane part, the metalloproteinases ADAM10/17 regulate LAG-3’s breakaway from the cell membrane. The cytoplasmic part consists of the serine phosphorylation site, the KIEELE motif, and the EP repeat motif. (B) The principal ligand of LAG-3 is MHCII, to which it binds with greater affinity than CD4. LAG-3 is involved in DC maturation and activation; it is involved in decreased T cell proliferation and cytokine production, upregulating the production of interleukins and NF-κB, via PI3K/Akt signaling. LAG-LSEC interaction is positively correlated with tumor growth, while LAG-3-Ig fusion proteins inhibit CD4/MHCII-dependent cellular adhesion assays, modulating MHCII-TCR signaling. (C) Cells with increased LAG-3 expression, as well as basic regulators of LAG-3 expression and interleukins regulated by LAG-3. LAG-3 is highly expressed in various types of immune cells (CD8+, Tregs, B cells, and NK cells), and is positively (IL-1, IL-2) or negatively (IL-4) regulated by interleukins. Additionally, it acts as a regulator of IL-10. ADAM: a disintegrin and metalloproteinase; APC: antigen-presenting cell; CD3: cluster of differentiation 3; CD4: cluster of differentiation 4; CD8: cluster of differentiation 8; IL-1: interleukin 1; IL-2: interleukin 2; IL-4: interleukin 4; IL-10: interleukin 10; LSECs: liver sinusoidal endothelial cells; MHCII: major complex histocompatibility complex class II; mTOR: mammalian target of rapamycin; NFκB: nuclear factor kappa-light-chain-enhancer of activated B cells; NK cells: natural killer cells; PD1: programmed cell death protein 1; PD-L1: programmed death-ligand 1; PI3K/Akt: phosphatidylinositol 3-kinase/protein kinase B pathway; S-S: disulfate bond; TCR: T-cell receptor; Tregs: T regulatory cells.
The distinct role of LAG-3 in reducing CD8+ T cell response has been evidenced in various models[34]. Research reveals that LAG-3 is briefly expressed on CD8+ T cells following acute stimulation, while it persists at elevated levels under tolerizing conditions[35]. As CD8+ T cells do not engage with MHCII, immunologists have posited that LAG-3 potentially modulates the function of these cells via alternative ligands. The initial molecule suggested as a potential ligand is Galectin-3, an extensively distributed lectin across various tissues and cell types, playing a crucial role in regulating immune responses and inflammation[36]. The association between the two molecules has been supported through in vitro experiments, wherein the expression of LAG-3 was found to be necessary for Galectin-3-mediated inhibition of CD8+ T cells[37]. Another possible ligand that could interact with LAG-3 in a comparable fashion is considered to be liver sinusoidal endothelial cell lectin (LSECtin), a cell surface lectin expressed in the liver that belongs to the DC-SIGN family[38]. Recent data have demonstrated that interactions between LAG-3 and LSEC contribute to increased tumor growth in melanomas by inhibiting the IFN-γ secretion of effector T cells, supporting its possible role in mechanisms concerning neoplasia[39]. Engagements with these two potential alternative ligands might also extend the influence of LAG-3 on further T cell function, especially concerning its inherent role on CD8+ T cells within the tumor microenvironment (TME). Finally, it is worth mentioning that there might be additional ligands for LAG-3 that are yet to be identified.
THE IMMUNOLOGIC BASIS OF ANTI-LAG-3 THERAPIES
Cancer growth and development involves evading immune checkpoint mechanisms and molecules such as PD-1, CTLA4, and LAG-3, which regulate immune responses against infectious agents and cancer cells[40]. The expression of LAG-3 extends beyond T cells, as it has also been demonstrated on various other immune cells. Notably, LAG-3 is expressed on activated B cells in a T cell-dependent manner, as well as on 20% of
PD-1 curbs T-cell receptor signaling, inhibiting T-cell proliferation and cytotoxic mediator secretion, potentially leading to T-cell exhaustion[47]. Immune checkpoint inhibitors targeting PD-1 and its ligand, programmed death-ligand 1 (PD-L1), including nivolumab, pembrolizumab, camrelizumab, tislelizumab, durvalumab, and atezolizumab, induce objective tumor responses in about 15% of advanced HCC patients in phase II and III trials, correlating with prolonged survival[48]. The randomized phase 2/3 trial RELATIVITY-047 revealed that relatlimab, a monoclonal antibody against LAG-3, when combined with nivolumab (anti-PD-1), demonstrated a 12-month progression-free survival (PFS) of 47.7% in melanoma patients, surpassing the 36% PFS observed with nivolumab monotherapy[49]. These compelling results marked a significant milestone in 2022, as the US Food and Drug Administration (FDA) granted approval for the relatlimab/nivolumab combination drug Opdualag for the therapy of unresectable or metastatic melanoma[50]. This decision positioned LAG-3 as the third checkpoint inhibitor that demonstrates efficacy in clinical settings when specifically targeted[51]. As mentioned above, monotherapy with PD-1/PD-L1 inhibitors showed limited improvement in overall survival compared to standard treatment[52]. To address this, combination strategies were explored, aiming either to stimulate T-cell activation via checkpoint inhibition or modify the tumor immune microenvironment to favor immune responses[53]. CTLA4 blockade enhances immune responses by targeting T-cell co-stimulation and Tregs[54]. Combining CTLA4 and PD-1/PD-L1 blockade leads to varied immune-stimulating effects, including modulation of effector
Figure 2. Multiple interactions of LAG-3 within the TME of HCC have made LAG-3 a potential therapeutic target with the aid of bispecific antibodies targeting both LAG-3 and PD-L1. The confirmed ligands of LAG-3 are MHCII, Galectin-3, LSECtin, and FGL1. MHCII is the principal ligand of LAG-3, showing higher binding affinity than CD4, while LAG-3 is highly glycosylated and can interact with Galectin-3, regulating T cell responses. LAG-3/LSECtin interaction is considered to promote tumor growth through the suppression of the antitumor T cell response, while the interaction of FGL1/LAG-3 is considered to promote tumor immune escape through the inhibition of the antigen-specific T cell. Galectin-3: galactose lectin-3; FGL1: fibrinogen-like protein 1; LSECtin: liver sinusoidal endothelial cell lectin; MHCII: major histocompatibility complex II; APC: antigen-presenting cell; ITAM: immuno-receptor tyrosine-based activation motif; FCγR: receptors for the Fc region of IgG; ERK: extracellular signal-regulated kinase.
THE CLINICAL LANDSCAPE OF LAG-3-TARGETED HEPATOCELLULAR CARCINOMA THERAPY
Unfortunately, the therapeutic options currently available for HCC, comprising of surgical resection, liver transplantation, and radiofrequency ablation, exhibit an effect only at the early stages of the disease. The majority of patients with HCC, however, are diagnosed at later stages and therefore have poor prοgnosis, disqualifying them from such treatment options[62]. HCC exhibits resistance to chemotherapy, but due to the presence of an inflammatory TME that has been associated with improved patient survival[63], immunotherapy is nowadays considered an important therapeutic modality for HCC[64]. It has been established that intratumoral Treg accumulation is a leading mechanism contributing to the immunoevasion of HCC, while inhibitory receptor-ligand pathways can also lead to the inhibition of antitumor immunity in the TME[65]. It has been demonstrated that tumor-infiltrating T cells, under the effect of chronic tumor antigens, are deprived of their effector and tumoricidal capacity, followed by a gradually greater variety and quantity of inhibitory receptors which are expressed on them, such as PD-1 and LAG-3[47]. When contacting their ligands on tumor cells and tumor-infiltrating APCs, they inhibit T-cell responses of tumor antigens, thus providing potentially unprecedented clinical benefits for patients[66]. In addition, Yang et al. demonstrated that there is an elevated LAG-3 expression in CD8+ tissue-resident memory T cells (Trm) in advanced HCC, while FGL1 levels are negatively associated with CD103 expression and were correlated with worse patient survival in HCC[67]. Patients with higher levels of CD8+ Trm had better outcomes, and FGL1/LAG-3 binding decreased the levels of CD8+ Trm cells in HCC, demonstrating its capabilities as an immunotherapeutic target for HCC.
Although LAG-3 mechanism of action has not been investigated as extensively as PD1, the pleiotropic roles of LAG-3 can potentiate the utilization of other pathways for LAG-3 blockade. LAG-3 is upregulated on tumor-infiltrating lymphocytes (TILs) and inhibition of LAG-3 can potentiate antitumor T cell responses, while combined blockade of the PD1 pathway and LAG-3 has been demonstrated to have a greater effect on antitumor immunity, as compared with targeting each molecule separately, representing a promising combinatorial treatment strategy for HCC[68]. Interestingly, Zhou et al. demonstrated on human HCC biopsies that inhibitory receptors PD-1, T cell immunoglobulin and mucin domain-containing protein 3 (TIM3), LAG-3, and CTLA4 were upregulated on tumor-infiltrating T cells, while PD-1, TIM3, and LAG-3 were elevated on tumor-associated antigen-specific CD8+ TIL[69]. Moreover, the blockade of PD-L1, TIM3, and LAG-3 increased the ex vivo CD4+, CD8+ TIL, and effector cytokine production, while antibodies against PD-L1, TIM3, or LAG-3 restored HCC-derived T cell response to tumor antigens alongside antibodies demonstrating synergistic effects, suggesting that inhibiting antibodies against TIM3, LAG-3, or CTLA4, alongside their respective combinations with PD-L1 blockade, may hold promise for the immunotherapy of individuals with HCC. The FGL1/LAG-3 pathway is also a potential target for immunotherapy and has demonstrated an additive effect with PD-1/PD-L1, while elevated levels of
Anti-LAG-3-based therapies can be subdivided into three categories: anti-LAG-3 monoclonal antibodies, bispecific LAG-3 antibodies, and LAG-3 immunoglobulin fusion proteins. However, there is growing evidence that relying solely on LAG-3 blockade might not constitute the ideal therapeutic approach, mainly due to the capacity of tumor cells to evade antitumor immune responses through various molecular mechanisms. Both PD-1 and LAG-3 constitute emerging mechanisms of HCC immunoescape and hold promise as therapeutic targets, with current evidence indicating that combined blockade of both pathways may be a better alternative than targeting either of the pathways alone in order to overcome the resistance to anti-PD-1/anti-PD-L1 or anti-CTLA4, while combining single or dual immune checkpoint blockade (ICB) therapy with agents that promote immunogenic cell death, is postulated to enhance tumor immunogenicity and potentially expand the pool of patients responsive to immunotherapy. In more detail, a recent study of 191 HCC-patient samples comprising resection specimens from ICB-naïve and before-treatment samples, from individuals with advanced HCC having received immune checkpoint blockade therapy, demonstrated a correlation between before-treatment LAG-3, CD8, STAT1, and LAG-3 CD8+ tissue expression and response rate to mAb immunotherapy in individuals with advanced HCC, while LAG-3 CD8+ cells were the most favorable biomarker. They demonstrated that immunohistochemical analysis of LAG-3 and CD8, as individual markers and the LAG-3+ CD8+ phenotype, was beneficial for predicting the response to ICB in before-treatment individuals with advanced HCC[74]. Interestingly enough, only the LAG-3+ CD8+ cell proportion was statistically correlated with response to ICB, irrespective of viral-related and non-viral HCC sample status, concluding that immunohistochemical staging of before-treatment LAG-3 and CD8 levels in the TME might aid in predicting ICB response in individuals with HCC. Similarly, another study by
Summary of studies evaluating the role of LAG-3 in hepatocellular carcinoma
Study (Year) | Study characteristics and treatment | Study findings |
Yang et al. (2023)[67] | Prospective, early- and advanced-stage human HCC FGL1 knockdown of Hepa1-6 murine HCC (n = 35) | Higher LAG-3 expression in CD8+ TRM in end-stage HCC FGL1/LAG-3 binding leads to greater CD8+ TRM exhaustion in HCC |
Zhou et al. (2017)[69] | Prospective, early- and advanced-stage human HCC Anti-PD-L1/anti-TIM3/anti-LAG-3 as monotherapy, or combined anti-PD-L1 with anti-TIM3/anti-LAG-3/anti-CTLA-4 (n = 59) | LAG-3 blockade increased CD8+ and CD4 proliferation in HCC LAG-3 expression was higher on CD8+ and CD4 T cells in HCC |
Guo et al. (2020)[70] | Retrospective, early- and advanced-stage human HCC Anti-FGL1, anti-LAG-3, anti-PD-L1 or anti-CD8 in human HCC (n = 143) | Elevated density of LAG-3 positive cells correlated with poor disease outcome in patients with HCC Elevated PD-L1 combined with high LAG-3 positive cells had a poorer prognosis |
Wang et al. (2020)[72] | Prospective, early- and advanced-stage murine HCC Oxysophocarpine, anti-LAG-3 therapy in Hep-G2 and Hepa1-6 murine HCC | Oxysophocarpine hindered HCC growth Oxysophocarpine increased LAG-3 blockade effect and decreased FGL1 expression |
Tian et al. (2022)[73] | Prospective, early- and advanced-stage human HCC (n = 127) | Elevated LAG-3 levels demonstrated shorter overall survival in HCC High LAG-3 correlated with increased MVD, preoperative AFP level, tumor diameter, and N stage |
Cheung et al. (2023)[74] | Retrospective, early- and advanced-stage human HCC (n = 191) | High LAG-3 was associated with shorter median progression-free survival and overall survival in HCC LAG-3 positive CD8+ proportion had a better predictive value of overall survival in long-likelihood models |
Guo et al. (2021)[75] | Prospective, advanced-stage human HCC TACE in individuals with HCC (n = 100) | High LAG-3 and PD-L1 levels presented with shorter overall survival High pre-TACE LAG-3 was correlated with poor disease outcome, cirrhosis, elevated AST/ALT and overall survival |
Yang et al. (2023)[76] | Prospective, advanced-stage murine HCC Neoantigen vaccination and anti-PD-1 in Hep-55.1C, Dt-81 and Hepa1-6 murine HCC | Combined neo-antigen vaccination and PD-1 blockade led to increased tumor regression in HCC Combined treatment reduced exhaustion-related markers PD-1 and LAG-3 on CD8+ T cells |
Jia et al. (2022)[77] | Retrospective, early- and advanced-stage human HCC (n = 1,884) | Anti-SPINK1 antibodies impeded HCC growth There was a positive correlation between SPINK1 and LAG-3 |
Li et al. (2022)[78] | Prospective, early- and advanced-stage human and murine HCC STAT3 inhibitor in Huh7 and Hepa1-6 cells (n = 371) | STAT3 inhibition prevented HCC growth STAT3 inhibition led to lower LAG-3 and PD-1 in HCC |
Macek Jilkova et al. (2019)[79] | Prospective, advanced-stage human HCC Sorafenib or Anti-PD-1/PD-L1 in human HCC (n = 21) | Patients irresponsive to Sorafenib had elevated LAG-3 levels Low baseline CD4/CD8+ T cells ratio was associated with better overall survival |
A recent study by Yang et al. implemented neoantigen peptide vaccination alongside anti-PD-1 and investigated the tumoricidal effects, concluding that the combination immunotherapy had better antitumor outcomes than monotherapy and resulted in more significant HCC regression, providing concrete evidence for further clinical research implementing neoantigen vaccination alongside anti-PD-1 therapy for the treatment of individuals with HCC[76]. Neoantigen vaccination enhanced the antitumor potency of anti-PD-1 on HCC, as results demonstrated decreased Tregs and monocytic myeloid-derived suppressor cells, elevated CD8+ T cells, increased granzyme B expression, and decreased PD-1 and LAG-3 on CD8+ T cells. Moreover, Jia et al. provided evidence that serine protease inhibitor Kazal type 1 (SPINK1) could be a potential biomarker for earlier detection, targeted therapy, and prediction of ICB response to therapy in the management of HCC, as SPINK1 enhanced the proliferation, anti-apoptosis, tumorigenic and metastatic potential of HCC cells, while the anti-SPINK1 antibody hindered the proliferation of HCC, indicating that SPINK1 can serve both as a tumor marker and as a target for immunotherapy for HCC management[77]. In more detail, in a transcriptomic analysis of 368 HCC specimens, a positive association between SPINK1 and immune checkpoints was demonstrated, including PD-1, LAG-3, TIM-3, TIGIT, HAVCR2, and CTLA4.
Along the same line, the inhibition of STAT3-mediated direct regulation of CD47 and glycolysis via GLUT1 in HCC cells led to anti-HCC immune memory and the accumulation of important antitumor effector CD8+ T cells, expressing lower levels of LAG-3 and PD-1, facilitating deeper knowledge of targeted inhibition of immune-checkpoints in HCC, while also shedding some light on new therapeutic options for HCC[78]. It is also worth noting that in a study of immunologic features of advanced HCC patients before and during sorafenib or anti-PD-1/PD-L1 therapy, a trend of enhanced activity of LAG-3 and TIM3 inhibitory immune checkpoints on circulating T cells was observed in individuals who were unresponsive to PD-1/PD-L1 pathway blockade, highlighting the clinical necessity of detailed immunomonitoring during therapies, in order to predict factors of response to therapy and to identify the pathway of resistance to immunotherapy, so as to broaden the pool of responders to HCC treatment[79]. Finally, similar to HCC, high expression of LAG-3 was associated with poor survival in head and neck squamous cell carcinoma, melanoma, breast cancer, and soft tissue sarcoma[80-82], whereas other studies evaluating the role of LAG-3 in gastric cancer, esophageal squamous cell carcinoma, and non-small cell lung carcinoma (NSCLC) demonstrated the opposite results[83-85]. In more detail, LAG-3 expression in gastric cancer was positively associated with a better prognosis, as sLAG-3 hindered tumor growth, enhancing the secretion of
DISCUSSION AND FUTURE PERSPECTIVES
LAG-3 holds promise as a target for immunotherapy, marked by the approval of the anti-LAG-3 antibody relatlimab, a therapeutic agent designed to target LAG-3. This approval has opened up avenues for advancing clinical development in this field, positioning LAG-3 as the third checkpoint inhibitor to demonstrate efficacy in clinical targeting. Furthermore, RELATIVITY-073, an ongoing, randomized, phase 2 study in immune-oncology-naive patients with advanced HCC who progressed on prior TKI therapy in the advanced/metastatic setting, will evaluate the efficacy and safety of the combination of relatlimab and nivolumab, aiming to leverage potentially synergistic pathways to enable T-cell activation and improve immune response in patients with HCC[87]. However, numerous unresolved questions persist, and gaining further insights is crucial for optimizing the utilization of current LAG-3-targeting therapeutics for HCC. Apart from therapeutics specifically targeting LAG-3, numerous bispecific antagonists concurrently addressing LAG-3 and PD-1 or PD-L1 are currently undergoing clinical evaluation, while it remains intriguing to ascertain whether these therapeutic agents exhibit efficacy comparable to the existing combination of relatlimab and nivolumab. Moreover, the exploration of the predictive value of RNA and protein biomarkers within the TME has emerged as a central focus of scientific inquiry, utilizing methodologies such as RNA-sequencing (RNA-seq) and immunohistochemistry (IHC), while the principal benefit of IHC methodologies over RNA sequencing lies in their greater translatability and adoptability in clinical practice. Notably, a particularly promising predictive marker for HCC is the four-gene inflammatory signature, encompassing CD8, PD-L1, LAG-3, and STAT1 genes, as demonstrated by the HCC CheckMate 040 clinical trial, which provided evidence that the expression of the four-gene inflammatory signature identified via RNA sequencing was correlated with enhanced responsiveness to nivolumab and improved overall survival[88]. An additional aspect to contemplate is whether the optimal blockade of all ligand interactions, including TCR-CD3 interactions, necessitates the use of antagonists. This consideration underscores the need for a comprehensive understanding of the therapeutic landscape, probing potential benefits and challenges associated with the simultaneous targeting of multiple pathways in immunomodulation.
However, a plethora of additional significant questions pertain to the way that LAG-3 influences or is influenced by various immune and non-immune pathways, while understanding the mechanisms driving the additive combination of treatment options targeting LAG-3 and PD-1 is essential for making informed decisions regarding the selection of new combinatorial treatments. In more detail, LAG-3 exhibits notable interactions with other immune checkpoints and, particularly, PD-1, collectively inhibiting T cell activation[33]. The interaction between LAG-3 and MHCII prevents its binding to both a TCR and CD4, thereby inhibiting TCR signaling, while LAG-3 emits an inhibitory signal through the KIEELE motif in its cytoplasmic tail. Additionally, LAG-3 interacts with two newly proposed ligands, Galectin-3 and LSECtin, which are found on melanoma cells regulating the function of CD8+ T cells within the TME, and when crosslinked with the CD3/TCR complex, it can hinder T cell proliferation and cytokine secretion[39,89]. Moreover, both LAG-3 and CTLA4 have been demonstrated to suppress the TCR signaling pathway, leading to the arrest of cell cycle progression and thereby negatively impacting T-cell homeostasis, promoting the immunosuppressive function of Tregs and exerting significant effects on DCs[90]. Antagonistic antibodies targeting these inhibitory immune checkpoints have demonstrated the potential to restore T cell function and have shown promise in ongoing clinical trials. The interaction of LAG-3 with its major ligand FGL1 is associated with HCC cell infiltration, proliferation, and secretion, while LAG-3 abundance is associated with increased microvessel density in primary HCC, a hallmark tumor angiogenesis[73]. The expression of the FGL1/LAG-3 pathway accelerates tumor proliferation due to the activation of Akt and mTOR, leading to accelerated cell growth, heightened cell migration, tumor angiogenesis, and epithelial-mesenchymal transition[91,92].
Due to their effect on restricting TCR signaling, LAG-3 therapeutics may synergize effectively with other treatment modalities targeting inhibitory molecules, such as TIM3 and TIGIT, co-stimulatory agonists, cytokines, or chemotherapy, while identifying optimal combinations will significantly contribute to advancing therapeutic strategies in the complex landscape of immune-related treatments. A puzzling inquiry arises as to why LAG-3 exhibits lower potency compared to PD-1, despite its focus on targeting TCR signaling, while PD-1 predominantly aims at the subordinate co-stimulatory signaling through CD28. The aforementioned discrepancy may be attributed in part to the broader expression of PD-1 as compared to LAG-3, but further evidence should be provided. Finally, a study of the PI3K/AKT/mTOR pathway-associated genes (PAGs) revealed a prognostic signature associated with immune infiltration in HCC, providing neoteric insights into the immunotherapeutic targets and the personalized treatment in HCC[93]. In more detail, the risk score was correlated with the levels of CD8 T cells, CD4 memory T cells, and Th cells, and the expression of immune checkpoints (PD-1, TIGIT, TIM3, LAG-3, and CTLA4) was positively associated with the risk score, concluding that the signature might be indicative of the status of the TME, and even pave the way for individualized immunotherapy in HCC. In summary, the clinical approval of a therapeutic agent targeting LAG-3 serves as a foundational milestone for advancing HCC immunotherapy, but further investigations are imperative to optimize the efficacy of this therapeutic approach.
CONCLUSION
In conclusion, HCC is, to this day, a significant global health challenge, being the fourth leading cause of cancer-related mortality worldwide, and with its incidence predicted to rise significantly by 2030, there is an urgent need for innovative therapeutic approaches. This narrative review has delved into the emerging importance of the LAG-3 signaling pathway in HCC, shedding light on its diverse roles in modulating T cell function and its potential significance in the context of HCC. Through a detailed examination of its structure, expression, and signaling mechanisms, it becomes evident that LAG-3 operates as a negative regulator of T cell activation, presenting a promising target for therapeutic intervention. We have also emphasized the potential role of LAG-3 signaling in HCC growth and development, aiming for a better understanding of the pathophysiological and immunological basis of the disease, highlighting the prognostic value of LAG-3 expression in HCC, with increased levels being associated with unfavorable outcomes. Recent studies have provided compelling evidence supporting the efficacy of LAG-3-targeted immunotherapies, both as monotherapy and in combination with existing treatments, while the association between pre-treatment LAG-3 levels and responsiveness to immune checkpoint blockade therapy offers a promising avenue for predicting treatment outcomes in advanced HCC patients and the exploration of LAG-3 in conjunction with other immune checkpoint molecules such as PD-1, TIM3, and CTLA4 presents a multifaceted approach to improving therapeutic responses. As we navigate the complexities of HCC treatment, it is crucial to acknowledge the limitations and ongoing challenges in this field, with the heterogeneity of HCC, intricate immune responses, and the dynamic nature of the TME posing significant ongoing challenges. Nevertheless, the promising results from preclinical and clinical studies underscore the potential of LAG-3-targeted therapies in reshaping the landscape of HCC treatment, while the insights gained from such studies lay the foundation for further research, offering hope for the development of novel and effective treatment modalities that can significantly impact the prognosis and outcomes for patients grappling with HCC.
DECLARATIONS
Author Contributions
Conceptualization and investigation: Arvanitakis K, Papadakos SP
Resources: Kalopitas G, Vakadaris G, Chatzikalil E
Data curation: Arvanitakis K, Vakadaris G, Stergiou IE
Data curation and writing-original draft preparation: Arvanitakis K, Vakadaris G, Stergiou IE
Writing-review and editing: Arvanitakis K, Papadakos SP, Kalopitas G, Chatzikalil E, Stergiou IE
Visualization: Theocharis S, Germanidis G
Supervision: Kalopitas G, Theocharis S, Germanidis G
Project administration: Chatzikalil E, Theocharis S, Germanidis G
Read and agreed to the published version of the manuscript: Arvanitakis K, Papadakos SP, Vakadaris G, Chatzikalil E, Stergiou IE, Kalopitas G, Theocharis S, Germanidis G
Availability of data and materials
Not applicable.
Financial support and sponsorship
None.
Conflicts of interest
All authors declared that there are no conflicts of interest.
Ethical approval and consent to participate
Not applicable.
Consent for publication
Not applicable.
Copyright
© The Author(s) 2024.
REFERENCES
2. Arnold M, Abnet CC, Neale RE, et al. Global burden of 5 major types of gastrointestinal cancer. Gastroenterology 2020;159:335-49.e15.
3. Rahib L, Smith BD, Aizenberg R, Rosenzweig AB, Fleshman JM, Matrisian LM. Projecting cancer incidence and deaths to 2030: the unexpected burden of thyroid, liver, and pancreas cancers in the United States. Cancer Res 2014;74:2913-21.
4. Petrick JL, Florio AA, Znaor A, et al. International trends in hepatocellular carcinoma incidence, 1978-2012. Int J Cancer 2020;147:317-30.
5. Arvanitakis K, Koletsa T, Mitroulis I, Germanidis G. Tumor-associated macrophages in hepatocellular carcinoma pathogenesis, prognosis and therapy. Cancers 2022;14:226.
6. Arvanitakis K, Mitroulis I, Chatzigeorgiou A, Elefsiniotis I, Germanidis G. The liver cancer immune microenvironment: emerging concepts for myeloid cell profiling with diagnostic and therapeutic implications. Cancers 2023;15:1522.
7. Arvanitakis K, Mitroulis I, Germanidis G. Tumor-associated neutrophils in hepatocellular carcinoma pathogenesis, prognosis, and therapy. Cancers 2021;13:2899.
8. McGlynn KA, Petrick JL, El-Serag HB. Epidemiology of hepatocellular carcinoma. Hepatology 2021;73 Suppl 1:4-13.
9. Jinjuvadia R, Patel S, Liangpunsakul S. The association between metabolic syndrome and hepatocellular carcinoma: systemic review and meta-analysis. J Clin Gastroenterol 2014;48:172-7.
10. Bouvard V, Baan R, Straif K, et al. WHO International Agency for Research on Cancer Monograph Working Group. A review of human carcinogens--part B: biological agents. Lancet Oncol 2009;10:321-2.
11. Ghouri YA, Mian I, Rowe JH. Review of hepatocellular carcinoma: epidemiology, etiology, and carcinogenesis. J Carcinog 2017;16:1.
12. Caruso S, O'Brien DR, Cleary SP, Roberts LR, Zucman-Rossi J. Genetics of hepatocellular carcinoma: approaches to explore molecular diversity. Hepatology 2021;73 Suppl 1:14-26.
13. Schulze K, Imbeaud S, Letouzé E, et al. Exome sequencing of hepatocellular carcinomas identifies new mutational signatures and potential therapeutic targets. Nat Genet 2015;47:505-11.
14. Meunier L, Hirsch TZ, Caruso S, et al. DNA methylation signatures reveal the diversity of processes remodeling hepatocellular carcinoma methylomes. Hepatology 2021;74:816-34.
15. De Sousa Linhares A, Leitner J, Grabmeier-Pfistershammer K, Steinberger P. Not all immune checkpoints are created equal. Front Immunol 2018;9:1909.
16. Sharpe AH, Pauken KE. The diverse functions of the PD1 inhibitory pathway. Nat Rev Immunol 2018;18:153-67.
18. Triebel F, Jitsukawa S, Baixeras E, et al. LAG-3, a novel lymphocyte activation gene closely related to CD4. J Exp Med 1990;171:1393-405.
19. Wang J, Sanmamed MF, Datar I, et al. Fibrinogen-like protein 1 is a major immune inhibitory ligand of LAG-3. Cell 2019;176:334-47.e12.
20. Lichtenegger FS, Rothe M, Schnorfeil FM, et al. Targeting LAG-3 and PD-1 to enhance T cell activation by antigen-presenting cells. Front Immunol 2018;9:385.
21. Ming Q, Celias DP, Wu C, et al. LAG3 ectodomain structure reveals functional interfaces for ligand and antibody recognition. Nat Immunol 2022;23:1031-41.
22. Guy C, Mitrea DM, Chou PC, et al. LAG3 associates with TCR-CD3 complexes and suppresses signaling by driving co-receptor-Lck dissociation. Nat Immunol 2022;23:757-67.
23. Huard B, Mastrangeli R, Prigent P, et al. Characterization of the major histocompatibility complex class II binding site on LAG-3 protein. Proc Natl Acad Sci U S A 1997;94:5744-9.
24. Workman CJ, Vignali DA. The CD4-related molecule, LAG-3 (CD223), regulates the expansion of activated T cells. Eur J Immunol 2003;33:970-9.
25. Annunziato F, Manetti R, Tomasévic I, et al. Expression and release of LAG-3-encoded protein by human CD4+ T cells are associated with IFN-gamma production . FASEB J 1996;10:769-76.
26. Workman CJ, Cauley LS, Kim IJ, Blackman MA, Woodland DL, Vignali DA. Lymphocyte activation gene-3 (CD223) regulates the size of the expanding T cell population following antigen activation in vivo. J Immunol 2004;172:5450-5.
27. Bruniquel D, Borie N, Hannier S, Triebel F. Regulation of expression of the human lymphocyte activation gene-3 (LAG-3) molecule, a ligand for MHC class II. Immunogenetics 1998;48:116-24.
28. Burton BR, Britton GJ, Fang H, et al. Sequential transcriptional changes dictate safe and effective antigen-specific immunotherapy. Nat Commun 2014;5:4741.
29. Workman C, Rice D, Dugger K, Kurschner C, Vignali D. Phenotypic analysis of the murine CD4-related glycoprotein, CD223 (LAG-3). Eur J Immunol ;32:2255.
30. Long L, Zhang X, Chen F, et al. The promising immune checkpoint LAG-3: from tumor microenvironment to cancer immunotherapy. Genes Cancer 2018;9:176-89.
31. Huard B, Prigent P, Tournier M, Bruniquel D, Triebel F. CD4/major histocompatibility complex class II interaction analyzed with CD4- and lymphocyte activation gene-3 (LAG-3)-Ig fusion proteins. Eur J Immunol 1995;25:2718-21.
32. Liang B, Workman C, Lee J, et al. Regulatory T cells inhibit dendritic cells by lymphocyte activation gene-3 engagement of MHC class II. J Immunol 2008;180:5916-26.
33. Hemon P, Jean-Louis F, Ramgolam K, et al. MHC class II engagement by its ligand LAG-3 (CD223) contributes to melanoma resistance to apoptosis. J Immunol 2011;186:5173-83.
34. Donia M, Andersen R, Kjeldsen JW, et al. Aberrant expression of MHC class II in melanoma attracts inflammatory tumor-specific CD4+ T- Cells, which dampen CD8+ T-cell antitumor reactivity. Cancer Res 2015;75:3747-59.
35. Wherry EJ, Ha SJ, Kaech SM, et al. Molecular signature of CD8+ T cell exhaustion during chronic viral infection. Immunity 2007;27:670-84.
36. Dumic J, Dabelic S, Flögel M. Galectin-3: an open-ended story. Biochim Biophys Acta 2006;1760:616-35.
37. Kouo T, Huang L, Pucsek AB, et al. Galectin-3 shapes antitumor immune responses by suppressing CD8+ T cells via LAG-3 and inhibiting expansion of plasmacytoid dendritic cells. Cancer Immunol Res 2015;3:412-23.
38. Liu W, Tang L, Zhang G, et al. Characterization of a novel C-type lectin-like gene, LSECtin: demonstration of carbohydrate binding and expression in sinusoidal endothelial cells of liver and lymph node. J Biol Chem 2004;279:18748-58.
39. Xu F, Liu J, Liu D, et al. LSECtin expressed on melanoma cells promotes tumor progression by inhibiting antitumor T-cell responses. Cancer Res 2014;74:3418-28.
40. Rimassa L, Finn RS, Sangro B. Combination immunotherapy for hepatocellular carcinoma. J Hepatol 2023;79:506-15.
41. Kisielow M, Kisielow J, Capoferri-Sollami G, Karjalainen K. Expression of lymphocyte activation gene 3 (LAG-3) on B cells is induced by T cells. Eur J Immunol 2005;35:2081-8.
42. Workman CJ, Vignali DA. Negative regulation of T cell homeostasis by lymphocyte activation gene-3 (CD223). J Immunol 2005;174:688-95.
43. Brignone C, Grygar C, Marcu M, Schäkel K, Triebel F. A soluble form of lymphocyte activation gene-3 (IMP321) induces activation of a large range of human effector cytotoxic cells. J Immunol 2007;179:4202-11.
44. Prigent P, El mir S, Dréano M, Triebel F. Lymphocyte activation gene-3 induces tumor regression and antitumor immune responses. Eur J Immunol 1999;29:3867-76.
45. Casati C, Camisaschi C, Rini F, et al. Soluble human LAG-3 molecule amplifies the in vitro generation of type 1 tumor-specific immunity. Cancer Res 2006;66:4450-60.
46. Triebel F, Hacene K, Pichon MF. A soluble lymphocyte activation gene-3 (sLAG-3) protein as a prognostic factor in human breast cancer expressing estrogen or progesterone receptors. Cancer Lett 2006;235:147-53.
48. Machairas N, Tsilimigras DI, Pawlik TM. Current landscape of immune checkpoint inhibitor therapy for hepatocellular carcinoma. Cancers 2022;14:2018.
49. Tawbi HA, Schadendorf D, Lipson EJ, et al. RELATIVITY-047 Investigators. Relatlimab and nivolumab versus nivolumab in untreated advanced melanoma. N Engl J Med 2022;386:24-34.
51. Aggarwal V, Workman CJ, Vignali DAA. LAG-3 as the third checkpoint inhibitor. Nat Immunol 2023;24:1415-22.
52. El-Khoueiry AB, Sangro B, Yau T, et al. Nivolumab in patients with advanced hepatocellular carcinoma (CheckMate 040): an open-label, non-comparative, phase 1/2 dose escalation and expansion trial. Lancet 2017;389:2492-502.
53. Minaei N, Ramezankhani R, Tamimi A, et al. Immunotherapeutic approaches in Hepatocellular carcinoma: building blocks of hope in near future. Eur J Cell Biol 2023;102:151284.
54. Ruff SM, Manne A, Cloyd JM, Dillhoff M, Ejaz A, Pawlik TM. Current landscape of immune checkpoint inhibitor therapy for hepatocellular carcinoma. Curr Oncol 2023;30:5863-75.
55. Hage C, Hoves S, Ashoff M, et al. Characterizing responsive and refractory orthotopic mouse models of hepatocellular carcinoma in cancer immunotherapy. PLoS One 2019;14:e0219517.
56. Voron T, Colussi O, Marcheteau E, et al. VEGF-A modulates expression of inhibitory checkpoints on CD8+ T cells in tumors. J Exp Med 2015;212:139-48.
57. Ziogas AC, Gavalas NG, Tsiatas M, et al. VEGF directly suppresses activation of T cells from ovarian cancer patients and healthy individuals via VEGF receptor type 2. Int J Cancer 2012;130:857-64.
58. Fu Z, Mowday AM, Smaill JB, Hermans IF, Patterson AV. Tumour hypoxia-mediated immunosuppression: mechanisms and therapeutic approaches to improve cancer immunotherapy. Cells 2021;10:1006.
59. Sarcognato S, García-Lezana T, Villanueva A. Mechanisms of action of drugs effective in hepatocellular carcinoma. Clin Liver Dis 2019;14:62-5.
60. Cabrera R, Ararat M, Xu Y, et al. Immune modulation of effector CD4+ and regulatory T cell function by sorafenib in patients with hepatocellular carcinoma. Cancer Immunol Immunother 2013;62:737-46.
61. Esteban-Fabró R, Willoughby CE, Piqué-Gili M, et al. Cabozantinib enhances anti-PD1 activity and elicits a neutrophil-based immune response in hepatocellular carcinoma. Clin Cancer Res 2022;28:2449-60.
62. Reig M, Forner A, Rimola J, et al. BCLC strategy for prognosis prediction and treatment recommendation: the 2022 update. J Hepatol 2022;76:681-93.
63. Chew V, Tow C, Teo M, et al. Inflammatory tumour microenvironment is associated with superior survival in hepatocellular carcinoma patients. J Hepatol 2010;52:370-9.
64. Foerster F, Gairing SJ, Ilyas SI, Galle PR. Emerging immunotherapy for HCC: a guide for hepatologists. Hepatology 2022;75:1604-26.
65. Pedroza-Gonzalez A, Verhoef C, Ijzermans JN, et al. Activated tumor-infiltrating CD4+ regulatory T cells restrain antitumor immunity in patients with primary or metastatic liver cancer. Hepatology 2013;57:183-94.
66. Nguyen LT, Ohashi PS. Clinical blockade of PD1 and LAG3--potential mechanisms of action. Nat Rev Immunol 2015;15:45-56.
67. Yang C, Qian Q, Zhao Y, et al. Fibrinogen-like protein 1 promotes liver-resident memory T-cell exhaustion in hepatocellular carcinoma. Front Immunol 2023;14:1112672.
68. Woo SR, Turnis ME, Goldberg MV, et al. Immune inhibitory molecules LAG-3 and PD-1 synergistically regulate T-cell function to promote tumoral immune escape. Cancer Res 2012;72:917-27.
69. Zhou G, Sprengers D, Boor PPC, et al. Antibodies against immune checkpoint molecules restore functions of tumor-infiltrating T cells in hepatocellular carcinomas. Gastroenterology 2017;153:1107-19.e10.
70. Guo M, Yuan F, Qi F, et al. Expression and clinical significance of LAG-3, FGL1, PD-L1 and CD8+T cells in hepatocellular carcinoma using multiplex quantitative analysis. J Transl Med 2020;18:306.
71. Ascierto P, Bono P, Bhatia S, et al. Efficacy of BMS-986016, a monoclonal antibody that targets lymphocyte activation gene-3 (LAG-3), in combination with nivolumab in pts with melanoma who progressed during prior anti-PD-1/PD-L1 therapy (mel prior IO) in all-comer and biomarker-enriched populations. Ann Oncol 2017;28:v611-2.
72. Wang J, Wei W, Tang Q, et al. Oxysophocarpine suppresses hepatocellular carcinoma growth and sensitizes the therapeutic blockade of anti-Lag-3 via reducing FGL1 expression. Cancer Med 2020;9:7125-36.
73. Tian J, Liu Y, Zhang TL, et al. Clinical significance of LAG-3 on microvessel density in primary hepatocellular carcinoma. Indian J Pathol Microbiol 2022;65:581-8.
74. Cheung CCL, Seah YHJ, Fang J, et al. Immunohistochemical scoring of LAG-3 in conjunction with CD8 in the tumor microenvironment predicts response to immunotherapy in hepatocellular carcinoma. Front Immunol 2023;14:1150985.
75. Guo M, Qi F, Rao Q, et al. Serum LAG-3 predicts outcome and treatment response in hepatocellular carcinoma patients with transarterial chemoembolization. Front Immunol 2021;12:754961.
76. Yang SF, Weng MT, Liang JD, et al. Neoantigen vaccination augments antitumor effects of anti-PD-1 on mouse hepatocellular carcinoma. Cancer Lett 2023;563:216192.
77. Jia J, Ga L, Liu Y, et al. Serine protease inhibitor Kazal type 1, a potential biomarker for the early detection, targeting, and prediction of response to immune checkpoint blockade therapies in hepatocellular carcinoma. Front Immunol 2022;13:923031.
78. Li Y, Song Z, Han Q, et al. Targeted inhibition of STAT3 induces immunogenic cell death of hepatocellular carcinoma cells via glycolysis. Mol Oncol 2022;16:2861-80.
79. Macek Jilkova Z, Aspord C, Kurma K, et al. Immunologic features of patients with advanced hepatocellular carcinoma before and during sorafenib or anti-programmed death-1/programmed death-l1 treatment. Clin Transl Gastroenterol 2019;10:e00058.
80. Deng WW, Mao L, Yu GT, et al. LAG-3 confers poor prognosis and its blockade reshapes antitumor response in head and neck squamous cell carcinoma. Oncoimmunology 2016;5:e1239005.
81. Burugu S, Gao D, Leung S, Chia SK, Nielsen TO. LAG-3+ tumor infiltrating lymphocytes in breast cancer: clinical correlates and association with PD-1/PD-L1+ tumors. Ann Oncol 2017;28:2977-84.
82. Que Y, Fang Z, Guan Y, et al. LAG-3 expression on tumor-infiltrating T cells in soft tissue sarcoma correlates with poor survival. Cancer Biol Med 2019;16:331-40.
83. He Y, Yu H, Rozeboom L, et al. LAG-3 protein expression in non-small cell lung cancer and its relationship with PD-1/PD-L1 and tumor-infiltrating lymphocytes. J Thorac Oncol 2017;12:814-23.
84. Zhang Y, Liu YD, Luo YL, et al. Prognostic value of lymphocyte activation gene-3 (LAG-3) expression in esophageal squamous cell carcinoma. J Cancer 2018;9:4287-93.
85. Hald SM, Rakaee M, Martinez I, et al. LAG-3 in non-small-cell lung cancer: expression in primary tumors and metastatic lymph nodes is associated with improved survival. Clin Lung Cancer 2018;19:249-59.e2.
86. Shepherd DJ, Tabb ES, Kunitoki K, et al. Lymphocyte-activation gene 3 in non-small-cell lung carcinomas: correlations with clinicopathologic features and prognostic significance. Mod Pathol 2022;35:615-24.
87. Sangro B, Numata K, Huang Y, et al. P-61 Relatlimab + nivolumab in patients with advanced hepatocellular carcinoma who are naive to immuno-oncology therapy but progressed on tyrosine kinase inhibitors, a phase 2, randomized, open-label study: RELATIVITY-073. Ann Oncol 2021;32:S117.
88. Sangro B, Melero I, Wadhawan S, et al. Association of inflammatory biomarkers with clinical outcomes in nivolumab-treated patients with advanced hepatocellular carcinoma. J Hepatol 2020;73:1460-9.
89. Matsuzaki J, Gnjatic S, Mhawech-Fauceglia P, et al. Tumor-infiltrating NY-ESO-1-specific CD8+ T cells are negatively regulated by LAG-3 and PD-1 in human ovarian cancer. Proc Natl Acad Sci U S A 2010;107:7875-80.
90. Baumeister SH, Freeman GJ, Dranoff G, Sharpe AH. Coinhibitory pathways in immunotherapy for cancer. Annu Rev Immunol 2016;34:539-73.
91. Bie F, Wang G, Qu X, et al. Loss of FGL1 induces epithelial-mesenchymal transition and angiogenesis in LKB1 mutant lung adenocarcinoma. Int J Oncol 2019;55:697-707.
92. Nayeb-Hashemi H, Desai A, Demchev V, et al. Targeted disruption of fibrinogen like protein-1 accelerates hepatocellular carcinoma development. Biochem Biophys Res Commun 2015;465:167-73.
Cite This Article
Export citation file: BibTeX | EndNote | RIS
OAE Style
Arvanitakis K, Papadakos SP, Vakadaris G, Chatzikalil E, Stergiou IE, Kalopitas G, Theocharis S, Germanidis G. Shedding light on the role of LAG-3 in hepatocellular carcinoma: unraveling immunomodulatory pathways. Hepatoma Res 2024;10:20. http://dx.doi.org/10.20517/2394-5079.2024.36
AMA Style
Arvanitakis K, Papadakos SP, Vakadaris G, Chatzikalil E, Stergiou IE, Kalopitas G, Theocharis S, Germanidis G. Shedding light on the role of LAG-3 in hepatocellular carcinoma: unraveling immunomodulatory pathways. Hepatoma Research. 2024; 10: 20. http://dx.doi.org/10.20517/2394-5079.2024.36
Chicago/Turabian Style
Konstantinos Arvanitakis, Stavros P. Papadakos, Georgios Vakadaris, Elena Chatzikalil, Ioanna E. Stergiou, Georgios Kalopitas, Stamatios Theocharis, Georgios Germanidis. 2024. "Shedding light on the role of LAG-3 in hepatocellular carcinoma: unraveling immunomodulatory pathways" Hepatoma Research. 10: 20. http://dx.doi.org/10.20517/2394-5079.2024.36
ACS Style
Arvanitakis, K.; Papadakos SP.; Vakadaris G.; Chatzikalil E.; Stergiou IE.; Kalopitas G.; Theocharis S.; Germanidis G. Shedding light on the role of LAG-3 in hepatocellular carcinoma: unraveling immunomodulatory pathways. Hepatoma. Res. 2024, 10, 20. http://dx.doi.org/10.20517/2394-5079.2024.36
About This Article
Special Issue
Copyright
Data & Comments
Data
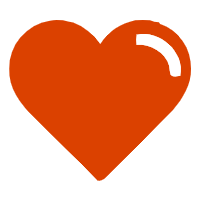
Comments
Comments must be written in English. Spam, offensive content, impersonation, and private information will not be permitted. If any comment is reported and identified as inappropriate content by OAE staff, the comment will be removed without notice. If you have any queries or need any help, please contact us at support@oaepublish.com.