乙型肝炎相关原发性肝癌的流行病学特征和精确定义的预防控制。(translate by chatgpt 4.0)
摘要 (translate by chatgpt 4.0)
关键词 (translate by chatgpt 4.0)
INTRODUCTION
Primary liver cancer (PLC) represents a spectrum of malignant hepatic neoplasms, including hepatocellular carcinoma (HCC) originating from hepatocytes, intrahepatic cholangiocarcinoma (ICC) arising from bile duct epithelial cells, and combined hepatocellular cholangiocarcinoma (CHC). The etiology of HCC is frequently associated with hepatitis B virus (HBV) or hepatitis C virus (HCV) infections[1]. In mainland China, the majority of PLC cases are HCC (93.0%), followed by ICC (4.3%) and CHC (1.6%), with HBV and HCV infections accounting for 84.4% and 3.2% of HCC cases, respectively[1,2]. Globally, the distribution and etiology of PLC vary significantly across different countries and ethnic populations. A comprehensive understanding of the global epidemiological characteristics of HBV infection and the mechanism by which HBV induces hepatocarcinogenesis is crucial for establishing a specific prevention and control technology system and realizing precise prevention and control.
THE GLOBAL EPIDEMIOLOGICAL CHARACTERISTICS OF PLC
According to the statistics from Globocan, PLC ranks sixth in incidence and the third leading cause of cancer-related death worldwide in 2022[3,4]. PLC is endemic in East Asia and sub-Saharan Africa. Asia accounts for 70% of the global burden of PLC in terms of both incidence and mortality. China has the highest number of cases, with 367,657 PLC cases reported in 2022[4-6]. In all regions worldwide, the incidence and mortality rates of PLC are higher in males than in females[2], with an age-standardized male-to-female ratio of 1.2 to 3.6[7]. It is speculated that by 2040, the number of PLC cases will reach 1.4 million, with
HCC is the predominant form of PLC. The global incidence of HCC varies due to differing prevalence of risk factors. Sub-Saharan Africa and East Asia are the endemic areas where the majority (80%) of HCC occur, with chronic HBV infection and exposure to aflatoxin B1 (AFB1) being the major risk factors[14]. China accounts for more than 51% of the global number of HCC cases[15]. According to the global burden of disease from 1990 to 2015, HBV and HCV led to 432,000 HCC deaths (54%), alcohol caused 245,000 HCC deaths (30%), and other causes accounted for 133,000 HCC deaths (16%)[16]. ICC is a heterogeneous group of aggressive tumors of the biliary system, accounting for about 15% of PLC worldwide, with an incidence that is low in most high-income countries (0.3-2 cases per 100,000) but much higher (even 40-fold increase) in regions such as China and Thailand[16,17]. The incidence of ICC is highest in Asia, especially in South Korea (ASR = 2.80), Thailand (ASR = 2.19), and Japan (ASR = 0.95). The incidence of ICC increased in most of the countries between 1993 and 2012[12].
MAIN RISK FACTORS OF PLC
Any factor that can lead to persistent chronic liver injury is the etiology of PLC. The main cause of HCC in China is chronic HBV infection, followed by aflatoxin exposure, infection with Clonorchis sinensis (liver fluke), heavy alcohol consumption, metabolic syndrome, HCV infection, and smoking. Adding any of these risk factors to HBV infection or together with hepatitis D virus infection will substantially increase the risk of HCC; liver fluke, metabolic syndrome [obesity, diabetes mellitus, non-alcoholic fatty liver disease (NAFLD)], heavy alcohol consumption, HBV and HCV infections are the major risk factors for ICC[2]. Recent studies have demonstrated a clear association between metabolic dysfunction-associated fatty liver disease (MASLD) and ICC[18]; however, the direct causal chain between them has not been clearly verified[19,20].
HBV infection
Chronic HBV infection is mainly transmitted perinatally (mother-to-child) or horizontally (contacted with infected blood). Approximately 90% of chronic HBV infections are acquired during infancy or early childhood. Booster vaccinations during childhood are effective in preventing HBV infection. In addition to this, HBV is spread through needle-stick injuries, tattoos, and piercings. WHO estimates that 254 million people were living with chronic hepatitis B and that hepatitis B caused 1.1 million deaths in 2022[21]. Regions with a high burden of chronic hepatitis B infection mainly include the Western Pacific (97 million), Africa (65 million), and Southeast Asia (61 million)[21].
HCV infection
HCV is a blood-borne virus that is transmitted primarily through sharing unsterilized needles, transfusion of unscreened blood, and sexual contact with bleeding[22]. An estimated 50 million people worldwide will be chronically infected with HCV by 2024, with approximately 1 million new infections per year. Chronic HCV infection occurs in all regions, with the most severely affected regions in the Eastern Mediterranean region (12 million people chronically infected), the Southeast Asia region and the European region
Metabolic dysfunction-associated steatotic liver disease
The International Diabetes Federation (IDF) recently defined MASLD as a condition characterized by central obesity (measured by a waist circumference of ≥ 94 cm for European men and ≥ 80 cm for European women, with other ethnic groups having their own specific values) plus any two of the following four factors [triglyceride (TG) level > 1.7 mmol/L; high-density lipoprotein cholesterol (HDL-C) < 1.0 mmol/L in men and < 1.3 mmol/L in women; systolic blood pressure ≥ 130 mm Hg or diastolic blood pressure ≥
Alcohol
Excessive alcohol consumption has been widely recognized as a risk factor for PLC, although the relationship between small amounts of alcohol and PLC remains unclarified[44]. The annual incidence of HCC in patients with alcohol-associated cirrhosis ranges from 0.9% to 5.6%. Approximately 1/5 of HCC deaths globally in 2019 will be attributable to alcohol consumption. The highest per capita alcohol consumption is in Europe (12.3 liters in 2005 and 9.8 liters in 2016) and the Western Pacific (4.6 liters in 2005 and 7.3 liters in 2016)[45]. This fact is corroborated by the number of alcohol-related liver cancer deaths in these two regions [22,215 (18,146-26,413) deaths in Europe in 2019, and 27,623 (21,296-34,686) deaths in the Western Pacific][46]. In Europe and the United States, most HCC is associated with alcoholic liver disease (ALD)[47]. The factors that promote the progression of ALD to HCC include malnutrition caused by long-term drinking, hepatic stellate cells (HSCs) activation, damage caused by alcohol and its metabolites, oxidative stress in liver tissue, immune reaction, and genetic factors[48]. Heavy alcohol consumption and aldehyde dehydrogenase 2 (ALDH2) rs671 polymorphism significantly increased the risk of HBV-HCC development and mortality[49]. There exists a synergistic effect between HBV, HCV, and alcoholic hepatotoxicity, especially HCV infection with alcohol intake[48,49].
Other factors
Aflatoxin exposure, schistosomiasis, water contamination, nicotine use, and genetic predisposition are thought to be other possible risk factors for PLC[14,47,50,51]. Globally, 5%-28% of HCC is associated with AFB1[52]. The incidence of HCC in HIV-infected patients has shown an increasing trend, especially in those patient groups co-infected with HBV or HCV[53]. The incidence of HCC in HIV-infected patients has shown an increasing trend, especially in those patient groups co-infected with HBV or HCV[14,53]. In addition to the above causative factors, many causes are still being discovered, and the synergistic mechanisms of carcinogenesis among these factors need to be further explored.
EVOLUTIONARY PATTERNS OF HBV IN THE “TRILOGY” OF HEPATOCELLULAR CARCINOGENESIS
HBV mutations at the core promoter and enhancer regions
Viral sequences from over 1,000 asymptomatic HBV-infected patients, particularly those seropositive for HBeAg, were used to identify “wild sequences”, which served as controls for recognizing disease-associated HBV mutations. We characterized the HBV mutations at the core promoter and enhancer region at each stage of the HBV oncogenic “trilogy” and revealed 66 previously unreported HCC-associated HBV variants. Key mutations in HBV genotype C, including C1673T, A1726C, A1727T, C1730G, C1766T, A1762T/G1764A, T1768A, C1773T, and C1799G, were found to increase the risk of cirrhosis significantly. Multifactorial logistic regression analysis disclosed that age, abnormal aminotransferase (ALT), HBV DNA ≥ 104 copies/mL, and genotypes C, C1653T, T1674C/G, T1753V, and A1762T/G1764A were independent risk factors for HCC development. The haplotype 1653C-1674G/C-1753V-1762T/1764A, a predominant HBV variant, was detected in HCC 6.28 times more frequently than in non-HCC-infected individuals, demonstrating high specificity and detection frequency, rendering it suitable for HCC prediction[54] [Table 1]. These studies contribute to the definition of the high-risk group for HCC.
Key mutation sites of HBV/HCV-HCC
Viral type | Mutation sites | Country/Region | Reference |
HBV | C1673T, A1726C, A1727T, C1730G, C1766T, A1762T/G1764A, T1768A, C1773T, C1799G,1653C-1674G/C-1753V-1762T/1764A | China | Yin et al. 2011[54] |
HBV | pre-S1 deletion, pre-S2 deletion, pre-S2 start site mutations,C2964A, C3116T, C7A, T53C, preS PreS2 start C3116T, C7A, T53C | China | Yin et al. 2010[55] |
HBV | C105T, T1753V, A1762T/G1764A | China | Liu et al. 2011[56] Xie et al. 2010[57] |
HBV | G40C | China | Chen et al. 2021[62] |
HBV | G2950A/G2951A/A2962G/C2964A, C3116T/T31C | China | Pu et al. 2022[63] |
HBV | IL-17A rs2275913 (G197A), IL-17F rs763780 (A7488G) | Iran | Gheshlaghi et al. 2021[213] |
HBV | rs3077(HLA-DP), rs9277535(HLA-DP) | China, Korea, Japan, Saudi Arabia, Thailand | Yu et al. 2015[214] |
HBV | IL10RB-rs2834167, PAPL-rs423058A, DEPDC5-rs1012068 | China | Ma et al. 2014[215] |
HCV | A028C, G209A, C219U/A, U264C, A271C/U, C378U/A, G435A/C, G481A, U303C/A | Europe, America | Hu et al. 2009[205] |
HCV | CHI3L1 (rs880633), intergenic (rs597533) polymorphisms | Egypt | Mangoud et al. 2021[216] |
HCV | HCP5-rs2244546 | Switzerland | Lange et al. 2013[217] |
Characteristics of HBV mutations in the pre-S region
Significant differences in the judgment of the HBV mutations in the preS region were observed, which was particularly distinct in different HBV genotypes. Asymptomatic HBV-infected carriers, chronic hepatitis B patients, cirrhotic patients, and HBV-HCC patients were used as mutual controls to screen the HBV mutations in the preS region and part of the S region. The pre-S1 deletion, pre-S2 deletion, and pre-S2 start site mutations were substantially more frequent in HCC than in asymptomatic HBV carriers, chronic hepatitis B patients, and cirrhotic patients. HBV preS wild types were predominantly associated with an increased risk of cirrhosis, while their mutation types were mostly associated with an increased risk of developing HCC. Multifactorial logistic regression analysis confirmed that age, HBeAg conversion, ALT ≥ 45 U/L, and representative wild-type T3116C and A2964C were independent risk factors for increased risk of cirrhosis; age, HBV DNA ≥ 104 copies/mL, HBV genotype C, and HBV mutations including C2964A, C3116T, C7A, T53C, pre-S2 start site variants, and pre-S1 deletion were independent risk factors for HCC[55] [Table 1], confirming the advantages and feasibility of the HBV mutations in predicting various liver diseases in the “trilogy” of HBV-induced carcinogenesis.
Effect of combined HBV variants on the occurrence of HCC
HCC-associated HBV variants are numerous, and age- and sex-matched case-control studies revealed the independent effects of HBV core promoter and preS mutations on the risk of HCC. It was found that HBV genotype C, preS 2 promoter mutations, C105T, T1753V, A1762T/G1764A, and other HBV variants were independent risk factors for HCC[56,57] [Table 1]. A systematic analysis of HBV variants with high specificity and detection rates in HCC revealed that the frequency of preS deletion, C1653T, T1753V, and A1762T/G1764A mutations gradually increased at each node of the HBV oncogenic “trilogy” disease. The HCC-associated variants in the HBV pre-S region and core promoter/enhancer II accumulate during the progression of HBV chronic infection to HCC, and these variants and their combination can predict the development of HCC[58]. The HBV signature disease mutations A1762T/G1764A can be detected 10 years prior to the onset of HCC, and the combination of mutations increases the specificity of monitoring HCC development more dramatically than single-point mutations. It was found that A1762T/G1764A appeared earlier, and preS deletion, C1653T, and T1753V appeared later after the acquisition of HBV infection[59]. A cohort study found that combined variants predicted HCC development up to 6 years earlier[60]. Therefore, a combined detection technique for high-risk HBV variants was established, which has been used for the surveillance of HCC occurrence in HBV-infected patients, for defining high-risk groups, and for the development of HCC secondary prevention.
Predictive role of HBV variants in HCC recurrence
Analysis of HBV variants in different liver tissues revealed asynchronous degrees of HBV evolution in cancerous tissues, adjacent liver tissues, and peripheral blood in HCC patients. In cancerous tissues, evolution was slowest due to the weakest immune pressure; in peripheral blood, immune selection was strongest, and HBV evolution was the fastest; and in adjacent liver tissues, the degree of HBV evolution was intermediate. Adjacent liver tissue is the most important site for HBV synthesis, and the evolutionary trajectory of HBV undergoing different immune pressures in the microenvironment after “leaving the factory” is completely different, resulting in conflicting roles of serum and tumor tissues in predicting HCC metastasis. Inflammatory molecules trans-activate APOBEC3s, which promote genomic mutation of HBV during replication throughout the RNA reverse transcription stage. APOBEC3B-related HBV mutations are important risk factors for HCC development after evolutionary selections. Among these, cancer-driving HBV mutations, such as A1762T/G1764A, in serum predict postoperative recurrence and metastasis of HCC. This effect was absent both in cancerous and adjacent liver tissues[61]. During HBV-HCC recurrence and metastasis, the direction of immune selection of HBV mutations tends to promote cancer metastasis, possibly because of immune selection in the tumor microenvironment (TME). For the first time, it was found that the G40C mutation in the pre-S region of HBV was able to independently promote the poor prognosis of HCC[62] [Table 1]. In this way, HBV evolution promotes not only HCC occurrence but also poor prognosis of HCC, which can play a guiding role in both tertiary prevention of HBV-induced carcinogenesis as markers for the prediction of HCC occurrence and prognosis as well as therapeutic efficacy.
MOLECULAR MECHANISMS OF HBV MAJOR VARIANT GENES IN PROMOTING CARCINOGENESIS
The Sleeping Beauty (SB) transposon animal model was first used to imitate the early stage of HBV infection and to integrate it into the host genome. It was found that hepatitis B x (HBx) genes with combined mutations upregulated more “yin” inflammatory cytokines and caused a higher rate of HCC and more metastasis than did the wild-type counterpart. Combined HBx mutations C1653T-T1674G-A1762T/G1764A cause the maximal level of proinflammatory cytokine, thus promoting hepatocarcinogenesis. Mutant HBx promotes HCC malignant transformation and stemness characteristics mainly by activating the expression of plasminogen activator inhibitor 1 (PAI-1) and cell division cyclin 20 (CDC20). These findings in the SB animal model were confirmed by subsequent cellular models and their xenograft animal models. PAI-1 has been shown to be a prognostic biomarker of HCC and a therapeutic target for HCC[63]. Meanwhile, our cohort study has also demonstrated that the frequency of combined C3116T/T31C mutations is significantly higher than that of preS 2 deletion (43.61% vs. 7.16%). Using the xenograft immunodeficiency animal model, we found that transfection of the large S gene carrying the major combined mutations G2950A/G2951A/A2962G/C2964A and C3116T/T31C in the preS region resulted in rapid tumor growth[63] [Table 1]. The mutant HBV large S gene was found to have a stronger ability to cause HCC and promote HCC metastasis and death than did the wild-type large S gene in the SB animal model. Both G2950A/G2951A/A2962G/C2964A and C3116T/T31C combination mutations activate inflammatory regulatory networks and metabolism-related signaling, inducing endoplasmic reticulum stress, affecting the body’s response to hypoxia, and upregulating the interleukin-6 (IL-6) signal transducer and activator of transcription 3 (STAT3) signaling pathway in the SB animal model. Thus, the HBV mutations at the large S gene promote HCC occurrence and metastasis by amplifying the IL-6/STAT3 signaling pathway[64]. Oncogenic HBV mutations arise due to immune selection and amplify oncogenic and cancer progression-related inflammatory signaling pathways in vivo. Thus, HBV evolution through the “wild-type HBV - immune selection - mutant HBV - local inflammation - carcinogenesis” eventually promotes HCC evolution by amplifying the proinflammatory and oncogenic pathways.
Drivers of HBV evolution: genetically determined inflammatory immune mechanisms
Genome-wide association analysis was employed to uncover new susceptibility loci for HBV-HCC; the STAT4 gene rs7574865 and the class II human leukocyte antigen (HLA)-DQ rs9275319 were identified to be the major susceptible genetic loci. STAT4, a crucial transcription factor and key regulator of the immune response, plays a significant role in transmitting signals from interleukin IL-12 and type I interferon (IFN-α or IFN-β) to induce IFN-γ, thereby influencing the immune response to HCC. The rs7574865 G allele, which affects STAT4 expression, has been shown to significantly increase the risk of HCC. Notably, STAT4 expression levels were significantly lower in cancerous tissues compared to paracancerous liver tissues, with the lowest levels observed in individuals with the rs7574865 GG genotype[65]. Additionally, a PRMT7-encoded gene intron region on chromosome 16q22.1 was identified to harbor an HCC-risk region, with the high-risk allele at rs73613962 exhibiting allele-specific enhancer activity. This “allele-specific enhancer” promotes PRMT7 expression through the binding of the transcription factor HNF4A, impacting HCC susceptibility via the P53 pathway[66]. We also discovered that rare genotypes of genetic polymorphisms of HLA-II antigen genes (HLA-DR, HLA-DP, and HLA-DQ) were negatively associated with the chronicity of HBV infection. Dominant alleles of these genetic polymorphisms promote chronic infection and immune selection of viral variants, making HBV more likely to cause HCC in Chinese populations. A significant finding was that major and rare genotypes in Chinese and white European populations are interchanged: genetic loci promoting the chronicity of HBV infection (HLA-DQ, HLA-DP, HLA-DR, and NFKBIA genetic loci) are major genotypes in the Chinese genome and rare in Europeans, and vice versa[67-69]. Furthermore, SNPs in the promoters of the NF-κB1 and IκB genes, which are part of the NF-κB pathway - a key inflammatory signaling network in the oncogenic process of HBV - were found to be responsible for the development of HCC in patients infected with HBV type C2. The polymorphism NFKBIA -881 (rs3138053, A>G) at the promoter region of the IκB, a key molecule of the NF-κB pathway, promotes the process of chronic inflammation and inflammation-carcinoma transformation[70]. Differences in the frequency of this polymorphic allele among different human races provide insights into the reason why the Chinese population is more susceptible to chronic HBV infection, which accounts for one-third of the world’s cases, and more likely to develop HCC, which accounts for half of the world’s cases. Genetic polymorphisms in NF-κB, STAT3, and other major inflammatory pathways in the HBV oncogenic pathway were also found to significantly interact with the pro-cancer HBV mutations in the development of HCC and cirrhosis[71,72]. Immunogenetic factors modulate the expression of immune/inflammatory molecules, influencing inflammatory-immune functions and predisposing individuals to develop chronic active hepatitis following HBV infection, thereby promoting the evolutionary development of HCC.
Inflammatory factors affect the balance between APOBEC3B - UNG
Immunogenetic traits, when interacting with environmental factors, foster the formation and maintenance of a chronic inflammatory microenvironment. This microenvironment serves as a driving force for the generation of HBV mutations and the selection of hepatocytes following somatic mutations. Dysregulation of the balance between APOBEC3s and uracil DNA glycosylase (UNG) is a central driver of somatic mutations in both the HBV genome and host cell genomes. The functional polymorphic locus of the APOBEC3B promoter rs2267401 has been shown to promote the generation of high-risk HBV mutations. Alleles that enhance APOBEC3B expression interact with alleles that reduce UNG enhancer function, significantly contributing to the development and poor prognosis of HCC. “Yin” inflammatory molecules, such as IL-6 and other trans-activators, activate APOBEC3B expression and reduce UNG expression. This leads to a dysregulation of the APOBEC3B-UNG balance, promoting somatic mutations and HBV mutations. The tumor inflammatory-immune microenvironment effectively selects the mutated cells and mutated viruses, promoting the evolutionary process of HCC through a process termed “mutation-selection-adaptation”[73]. We then depict a figure to show the current understanding of HBV-induced hepatocarcinogenesis [Figure 1]. The discovery of this mechanism further supports the theoretical basis of cancer evolution and development “Cancer Evo-Dev”. The imbalance of APOBEC3s-UNG plays a significant role not only in HBV carcinogenesis but also in other tumors, such as cholangiocarcinoma, gallbladder carcinoma, and renal cell carcinoma. The APOBEC3B promoter with rs2267401-G was found to be associated with a decreased risk of cholangiocarcinoma but associated with an increased risk of gallbladder cancer, possibly due to the high expression of the transcriptional repressor TFAP2A in cholangiocarcinoma. Tumor necrosis factor α (TNFα) increases the expression level of APOBEC3B by repressing TFAP2A. The genotype rs12157810-C at the APOBEC3A promoter was associated with a decreased risk of cholangiocarcinoma and gallbladder cancer, mainly due to the fact that TNFα increases the binding of the transcription factor Ets-1p68 to the APOBEC3A promoter carrying the rs12157810-C allele, promoting APOBEC3A overexpression. The mutagenicity of APOBEC3A is nearly 1,000-fold higher than that of APOBEC3B, and high expression of APOBEC3A leads to widespread breakage of host genomic DNA and induces apoptosis, thereby reducing cancer risk[74]. Similar findings have been reported in renal cell carcinoma[75]. Therefore, the APOBEC3A/APOBEC3B promoter allelic genotype might take part in regulating the direction of cancer evolution in inflammatory environments through interactions with specific transcription factors inherent to a given tissue type. To identify cancer-promoting pathways after somatic mutation, organoid technology was applied to analyze the mechanism by which the proinflammatory microenvironment selects the stem-like progenitors of HCC, ICC, and CHC. We found that the stemness characteristics of cancer cells in tumor tissues were positively associated with the success rate of organoid culture. These stemness characteristics not only determine the primary and sorafenib-induced resistance of HCC but also play a central role in cancer recurrence and metastasis[76]. Histological techniques applied to analyze the expression profiles associated with malignant tumors, such as HCC and colorectal cancer, revealed that inflammation promotes the cancer stemness-related signaling pathway and tumor-specific protein expression regulation mechanism by modulating the driver mutations of APOBECs[77]. The abnormal regulation of these tumor growth genes is a target for cancer treatment.
Figure 1. The mechanisms of HBV-induced hepatocarcinogenesis: the current understanding. The interplay between HBV infection, age, and genetic predisposition leads to chronic inflammation. Inflammatory mediators such as IL-6, STAT3, TNF-α, and NF-κB are activated in this process. These factors not only upregulate the expression of APOBEC but also downregulate the expression of the UNG, resulting in an APOBEC-UNG imbalance. This imbalance fosters both HBV mutation and somatic cell mutation. While most of the mutated HBV are eliminated by the immune system, a minority survives and integrates into the genome of these residual somatic cells. This integration triggers the activation of the EMT and oncogenic pathways, potentially leading to the development of HCC. Created with Biorender (https://www.biorender.com/) and Bioart (https://bioart.niaid.nih.gov/). HBV: Hepatitis B virus; HCC: hepatocellular carcinoma; IL-6: interleukin-6; STAT3: signal transducer and activator of transcription 3; TNF-α: tumor necrosis factor α; NF-κB: nuclear factor‐kappa B; APOBEC: apolipoprotein B mRNA editing enzyme, catalytic polypeptide-like; UNG: uracil-N-glycosylase; EMT: epithelial-mesenchymal transition.
In The Cancer Genome Atlas classification, HBV infection is significantly associated with Cluster 1, whereas HCV infection is significantly associated with Cluster 4[78]. In addition, other authors analyzed transcriptomic datasets composed of 99% HBV-related HCCs and showed that the molecular diversity of HBV-related HCCs spans from non-proliferative, well-differentiated tumors preserving a periportal or perivenous phenotype to poorly differentiated tumors enriched in metastasis signatures and stem cell markers[79].
ICC PATHOGENESIS
Many parts of the pathogenesis of ICC remain to be elucidated. Several meta-analyses have shown that HBV and HCV infections significantly increase the risk of developing ICC[80-84]. For ICC, HBV may produce multiple inflammatory mediators through inflammation, including IL-6, TNF-α, cyclooxygenase-2, and Wnt. Furthermore, transforming growth factor β (TGF-β), vascular endothelial growth factor, hepatocyte growth factor, and several microRNAs were also found to be upregulated, creating an environment conducive to the generation of successive oncogene mutations and cell proliferation[85]. In addition to this, HBV may act as an oncogene to promote cancer development. Experiments in mice have shown that high expression of HBx protein may lead to the amplification of EpCAM(+) or OV6(+) tumor cells[86]. It has also been shown that amplification of the RNA editing enzyme ADAR1 induced increased expression and caused aberrant editing of the p.M8V locus of the KPC1 gene in ICC. This aberrant editing activates the NF-κB signaling pathway, which in turn promotes the progression of ICC[87].
SCREENING AND DIAGNOSIS
To find PLC patients at the early stage, screening of PLC is an important public health action in the endemic areas. The cost-effective ratio should be considered for this action, even in the high-risk population. Specific techniques used for PLC screening can be categorized as invasive and non-invasive. Non-invasive means include simple technical scoring, imaging means [computed tomography (CT), magnetic resonance imaging (MRI), ultrasound (US), positron emission tomography-computed tomography (PET-CT), positron emission tomography - magnetic resonance imaging (PET-MRI)], and serum marker testing. US plus serum alpha-fetoprotein (AFP) is the most popular non-invasive protocol for HCC screening. The invasive screening means mainly refers to liver biopsy.
Screening
HCC screening is a long-term, cyclical and universal endeavor. Experts generally agree that early diagnosis is the key to successful treatment[88]. The selection of screening methods should take into account factors such as accuracy, operational difficulty, degree of impairment, and economic cost[89]. The monitoring of HCC can be divided into invasive and non-invasive methods. Invasive monitoring includes liver biopsy, which may lead to some complications and is also subject to potential bias due to variations in test samples and observer interpretation. Non-invasive methods encompass some simple counting methods [Fibrosis 4 Index (FIB-4)and NAFLD Fibrosis Score (NFS)], US, MRI, serum biomarkers, and shear wave elastography[90,91]. Currently, abdominal US and serum AFP testing remain the primary routine screening methods because they are well-established, inexpensive, and easy to perform and administer in areas with varying medical conditions. US combined with AFP screening is significantly more sensitive than either test alone[92]. According to current guidelines[92-96], HCC surveillance using US with or without serum AFP levels should be considered every 6 months in patients with NASH-associated cirrhosis in high-risk groups such as those with hepatitis viral infections, chronic alcohol consumption, obesity, and diabetes. However, US visualization may be less sensitive for HCC[97-99], especially in patients with early tumors[98,100,101], ascites, cirrhosis, and obesity[97,102,103]. In 2022, the Korean Liver Cancer Association (KLCA) and NCC guidelines also recommend dynamic contrast-enhanced CT or dynamic contrast-enhanced MRI as an alternative screening tool if liver ultrasound is limited or not indicated[104]. The use of simple and easy-to-generate parameters, such as fibrosis scores, can help screen patients with advanced liver fibrosis or cirrhosis who are at increased risk of developing HCC[105]. Due to the low prevalence of ICC in the population as a whole, screening is not recommended by medical societies, given the cost-effective ratio[106,107].
Diagnosis
In 2023, experts from the Italian Association for the Study of the Liver (AISF), the Italian Association of Medical Oncology (AIOM), and the Italian Association of Hepatobiliary and Pancreatic Surgery (AICEP), among others, jointly recommended that the diagnosis and therapeutic evaluation of patients with HCC should be carried out by a team of professionals working in a multidisciplinary manner and not independently by a single specialist[108]. Non-invasive imaging findings are the standard for the diagnosis of HCC in patients with cirrhosis, and the typical imaging features of arterial versus venous flushing have good specificity and acceptable sensitivity and are recommended by national guidelines (AASLD or APASL guidelines)[109]. Enhanced CT or MRI should be performed in patients with nodules (especially < 2 cm in long diameter) or unexplained elevated serum AFP[110]. For individuals with US nodules or/and elevated serum AFP who do not yet meet diagnostic criteria, enhanced screening is recommended every 2-3 months, primarily using abdominal CT or MRI and specific contrast agents[111]. For tumors of > 2 cm in diameter, there is no significant difference in the sensitivity between MRI and CT[112]. However, early HCC (single nodule < 2.0 cm in diameter) is detected more frequently with MRI combined with gadoxetic acid disodium, a liver-specific MRI contrast agent, than with enhanced CT[113]. For nodules with a diameter of 1-2 cm, gadolinium-ethoxybenzyl-diethylenetriamine pentaacetic acid-enhanced MRI (EOB-MRI) and contrast-enhanced ultrasound (CEUS) have been included in China as screening tests for the diagnosis of nodes of undetermined size to improve the sensitivity for the diagnosis[114].
MRI provides more accurate and comprehensive information, which is better suited for early diagnosis. Biopsy should be performed to confirm the diagnosis of HCC as soon as atypical features of the liver lesion in terms of contrast uptake are observed[115]. Biopsy is the basis for the diagnosis of HCC[116], but biopsy may also produce a false-negative rate of about 30%[117].
Serum markers such as vitamin deficiency or antagonist II (PIVKA-II/DCP)-induced proteins can be used as complementary screening techniques. AFP-L3 is predominantly present in patients with HCC. The ratio of AFP-L3 to AFP eliminates the effects of other factors that contribute to the upregulation of AFP[118]. Gender, age, AFP-L3, AFP, and des-gamma-carboxy prothrombin (DCP) (GALAD) improve the sensitivity of early HCC detection, but increase false-positive results[119]. In addition, novel molecular markers such as the HBV mutations, circulating tumor DNA (ctDNA), tumor-associated methylation, circulating free DNA (cfDNA), and non-coding RNAs have been shown to have high accuracy in HCC screening[54,120,121]. ctDNA, in combination with TP53 mutation, RASSF1a methylation, and GSTP1, could be used as a potential non-invasive screening method for HCC in patients with low AFP[122].
ICC is diagnosed primarily through imaging techniques. Multiple modality approaches, including CT, MRI, magnetic resonance cholangiopancreatography (MRCP), and PET, are often required to arrive at a final diagnosis and to screen patients for potential surgery[123]. US is usually the first test to detect ICC, which presents as a solid mass with variable echoes[124]. CT is considered the standard imaging method for preoperative evaluation. ICCs are usually of low density, have irregular borders and may be accompanied by peritoneal retraction. Contrast-enhanced scan is usually used to show ICC[125]. MRI is the most commonly used imaging method for periportal cholangiocarcinoma[123]. In some cases, the imaging features of ICC are similar to those of HCC and are, therefore, easily misdiagnosed[126]. Concurrent problems include the low diagnostic accuracy of current tumor markers, the difficulty in identifying benign and malignant biliary structures with imaging techniques, and confirmatory diagnostic modalities for invasive procedures[127]. Notably, the use of portal-phase flushing rather than conventional flushing in gadoxetic acid-enhanced MRI imaging may prevent the misdiagnosis of ICC as HCC in cirrhotic liver[128]. Deep migration learning models are considered the future direction for the diagnosis of lymph node metastasis, showing good predictive performance[129-131]. Lymph node metastasis may result in a significant increase in CD8+ T cell and NK-cell infiltration, reflecting an enhanced antitumor immune response, which might provide a direction for the prediction of lymph node metastasis of ICC[132].
TREATMENT
According to the Barcelona Liver Cancer Clinic (BCLC) algorithm, patients with HCC are classified into five clinical stages: very early stage (BCLC 0), early stage (BCLC A), intermediate stage (BCLC B), advanced stage (BCLC C), and terminal stage (BCLC D)[133]. Currently, locoregional therapy (LRT) is used for early to intermediate HCC, while sorafenib is used for advanced HCC. Recognition of the limitations of these treatments and the potential to enhance their efficacy has driven the exploration of combinative therapeutic options, particularly combining LRT with systemic therapy[134].
Surgical treatment
Surgical resection is the treatment of choice for cirrhosis-free patients with early-stage HCC according to Barcelona Clinical Liver Cancer Staging (BCLC 0 or A)[12]. According to the BCLC staging guidelines, hepatectomy is indicated in patients with a single tumor diameter of ≤ 2 cm and a Child-Pugh classification of A[133]. Differences in patient conditions may lead to different postoperative outcomes, with a 5-year overall survival (OS) of ~ 70% and perioperative mortality of < 2% in patients selected by strict criteria[95,135]. When HCC is found to be unsuitable for resection during surgical exploration, hepatic artery and portal vein catheterization chemotherapy or other intraoperative locoregional treatments may be considered[136]. Surgical resection is usually considered curative for HCC, but HCC also recurs from time to time in patients with multinodular disease, microvascular infiltrates and/or poorly differentiated tumors (5-year incidence of 50%-70%)[137]. Furthermore, patients associated with MASLD may avoid surgical resection due to the increased risk of perioperative complications[41,138]. Nevertheless, a meta-analysis based on 7,226 patients has shown that OS appeared to be better in patients with MASLD-associated than in those with virus-associated HCC (HR: 0.80; 95%CI: 0.67-0.96; P = 0.017)[139]. However, at the time of diagnosis, less than 10% of patients are eligible for surgery for liver resection or transplantation. LRT, the precise manipulation of liver tumors through minimally invasive image guidance, has become an integral part of HCC treatment[140]. Minimally invasive liver resection (MILS) is emerging as an alternative to open hepatectomy, which not only reduces intraoperative bleeding and hospital stay, but also has similar prognostic outcomes[141]. Robotic Liver Resection (RLR) frees up manpower and promises to be a new surgical modality. However, the high cost may be an important reason why RLR has not been performed on a large scale[141]. From November 2018 to June 2021, surgeon Broering D and the bedside surgical team completed 356 robotic donor hepatectomies with no donor deaths, only two open conversions, and an overall complication rate of just under 6% for all donor types (no grade 3 or higher complications). Due to the special capabilities of articulated instruments and magnified 3D vision, as well as considerable ergonomic advantages, RLR overcomes the drawbacks of inflexible fixation of surgical instruments and large oscillations of the visual field leverage effect of conventional laparoscopic surgery (LLR). However, the high cost may be an important reason why RLR has not been performed on a large scale[141]. The recognized cure for ICC is still surgical excision, which is only possible in 20% of cases at the time of diagnosis[142]. The aim of surgical treatment is complete margin-negative (R0) resection, and the functional residual capacity of the liver and the radical nature of the tumor, as well as the patient’s physical status, are determinants of surgical success[143]. ICC patients who undergo staged laparoscopy (SL) have better perioperative outcomes and longer OS than those who do not[144,145]. The therapeutic efficacy of lymph node dissection (LND) for ICC is controversial and remains to be proven. The impact of LND on ICC and its extent seems to be closely related to the specific location of the tumor. A multicenter analysis showed that in hilar lesions, more extensive lymph node removal of level 2 (retro-pancreatic or common hepatic artery lymph nodes) could be recommended, whereas in peripheral lesions, LND might not be removed beyond the entrapment of level 1 (hepatoduodenal ligament lymph nodes)[146]. A multicenter cohort study involving 159 patients showed that LND did not significantly improve the prognosis of patients with clinically lymph node-negative ICC [median recurrence-free survival (12.0 months vs. 10.0 months; P = 0.37) and median OS (22.0 months vs. 26.0 months; P = 0.47) in the LND vs. non-LND groups][147]. Interestingly, in a meta-analysis involving 4,407 patients, LND did not improve prognosis in patients with ICC (HR: 1.05; 95%CI: 0.83-1.32). Meanwhile, LND was significantly associated with superior OS in low-risk group patients (HR: 0.76; 95%CI: 0.59-0.98)[148].
According to the Milan criteria, LT is usually indicated for a single tumor of ≤ 5 cm or 2-3 tumors of ≤ 3 cm without vascular infiltration[149]. According to the BCLC staging guidelines, early LT is usually considered the best treatment for patients with HCC when a single tumor has a diameter of ≤ 2 cm and a Child-Pugh grade of C. In addition, early LT is often considered the best treatment for patients with HCC. LT has a median OS of > 10 years and a recurrence rate of ~ 10% in patients selected according to strict criteria[150]. LT is considered superior to resection in terms of long-term outcomes (70% recurrence rate and 7%-15% survival at 10 years)[151]. Living donor liver transplantation (LDLT) is considered the optimal therapeutic option for patients with HBV-HCC due to its shorter waiting period, better quality of donor liver, and almost negligible cold ischemia time[152]. However, patients with HCC may have to wait longer for reasons such as organ shortages, which can lead to patients being pushed off waiting lists due to tumor progression, and the likelihood of cure by resection is similar to LT when the dropout rate reaches 20% and above[153,154]. There is not sufficient evidence to use LT as the absolute standard of care for ICC. However, LT has demonstrated positive therapeutic effects in carefully screened patient populations, and prospective studies combining systemic neoadjuvant chemotherapy (NAC) with localized therapies in conjunction with LT have revealed some encouraging long-term efficacy[155]. For unresectable perihilar cholangiocarcinoma (pCCA), adjuvant radiotherapy combined with LT offers a new idea of resolution, with the largest consecutive series published by the Mayo Clinic showing that 221 patients underwent LT between 1993 and 2018, and that the 5-year OS of pCCA was 58%[156].
Adjuvant therapy
While waiting for LT, patients receive treatments known as bridging therapies to avoid HCC progression. Common bridging modalities include transarterial chemoembolization (TACE), ablation, and radiotherapy[14]. Bridging therapies significantly decrease the risk of HCC recurrence after liver transplantation[157,158]. Ablation is an adjuvant therapy that directly damages the tumor by chemical, thermal, or electrical means[159]. For patients with early-detected HCC (BCLC 0 or A) who are not candidates for surgery or transplantation, local ablation with radiofrequency ablation (RFA) or microwave (MWA) radiation is the standard of care, with selective transarterial radioembolization (TARE) or stereotactic radiotherapy (SBRT) as an alternative[103,121-123]. The AASLD and EASL guidelines have identified RFA as the primary first-line treatment for single tumors < 2 cm and as an alternative to surgery for early single tumors of 3-4 cm or for 2-3 tumors of < 3 cm[92,160]. For unresectable ICC measuring ≤ 3 cm without evidence of extrahepatic disease, the guidelines consider ablation as a palliative treatment option[106]. However, in patients with HCC tumors > 3 cm in diameter or serum AFP levels > 200 ng/ml, hepatectomy may perform better than RFA in terms of local disease control and long-term survival[161]. RFA results in a median OS of ~ 60 months and a 5-year recurrence rate of 50%-70% in HCC[162,163]. Currently, RFA and MWA are well-established thermal techniques, while other ablation techniques such as cryoablation (CRA), laser interstitial thermotherapy, and irreversible electroporation (IRE) are less commonly used[159]. TACE is the standard of care for intermediate-term HCC at Child-Pugh class B. In a prospective study of 175 patients with HCC, the mean time of chemoembolization was 58.1 min, and this duration was significantly correlated with the number of nodules[164]. TARE is a treatment technique that kills tumor cells by releasing radiation from microspheres with a radioactive substance, usually for intermediate-stage disease[165]. A meta-analysis demonstrated a median OS of 26-30 months for TACE, with no difference in OS between the two modalities[166]. An increasing number of patients with unresectable ICC are now being treated with TACE and TARE[167,168]. Drug-eluting bead transarterial chemoembolization (DEB-TACE) is an endovascular therapeutic technique specifically targeting unresectable HCC. This technique achieves effective control of drug release kinetics by encapsulating chemotherapeutic drugs within microspheres and releasing them precisely in the lesion area[169]. The 2023 Korean Hepatocellular Carcinoma Association Expert Consensus states that DEB-TACE has less severe postembolization syndrome, shorter hospital stay, and similar survival compared to conventional TACE. However, DEB-TACE may not be indicated for HCC ≤ 3 cm[170]. In patients with locally advanced HCC or those who do not respond to TACE, hepatic arterial infusion chemotherapy (HAIC) with the FOLFOX regimen consisting of oxaliplatin, fluorouracil, and folinic acid or in combination with systemic therapy is recommended[171,172].
Adoption of adjunctive therapeutic techniques for postoperative ICC patients is thought to be beneficial to patient prognosis[147,173,174]. There is a cohort study showing that postoperative adjuvant therapy is associated with prolonged survival in patients with ICC, especially in the non-LND group (P = 0.02 for recurrence-free survival, P = 0.03 for OS)[147]. A meta-analysis involving 10,181 patients showed that chemotherapy significantly improves postoperative prognosis in ICC (HR: 0.57; 95%CI: 0.44-0.70)[173]. A study from the National Cancer Institute’s Surveillance, Epidemiology, and End Results (SEER) involving 3,226 patients with lymph node-positive biliary tract cancers showed that the median survival time in the surgery-combination-chemotherapy group was significantly longer than in the surgery-alone group (median survival time of 19 months vs.10 months, P < 0.01)[174].
Systemic therapy
Systemic therapy is divided into targeted therapy and immunotherapy. The approach is suitable for treating advanced and intermediate-stage HCC patients who are not eligible for local treatment[14]. Six systemic therapies have been approved (atilizumab plus bevacizumab, sorafenib, levatinib, regorafenib, cabozantinib and ramorubicin)[14]. In the absence of contraindications (e.g., bleeding risk), multiple authoritative guidelines recommend atezolizumab plus bevacizumab as a first-line therapeutic option for HCC[92,175-177]. In recent years, the combination of interventional and systemic therapy has become an important tool in the treatment of PLC[178,179].
There are two types of multi-targeted kinase inhibitors: one with anti-proliferative and anti-angiogenic properties through inhibition of receptors, such as platelet-derived growth factor receptor (PDGFR) and vascular endothelial growth factor receptor (VEGFR) tyrosine kinases, and the other, monoclonal antibodies that inhibit VEGF/VEGFR pathway[180]. The former drugs include sorafenib, lenvatinib, cabozantinib, and regorafenib[181]. Sorafenib was the first adopted first-line treatment for patients with advanced HCC. Superior efficacy of sorafenib over placebo has been demonstrated in patients with advanced HCC (median OR: 10.7 months vs. 7.9 months; HR: 0.69; 95%CI: 0.55-0.87)[182]. Lenvatinib is an inhibitor of VEGF receptors 1-3, FGF receptors 1-4, PDGFRα, RET, and KIT. A randomized, non-inferiority trial enrolling 1,492 patients has demonstrated that in untreated advanced HCC, lenvatinib is proven to be non-inferior to sorafenib in terms of OS (Median OS: 13.6 months vs. 12.3 months; risk ratio: 0.92; 95%CI: 0.79-1.06). However, Lenvatinib adverse reactions are more frequent, mainly focusing on skin reactions, diarrhea, and hypertension[183]. In addition to these two drugs, first-line treatment options include the combination of Atezolizumab and Bevacizumab, which has been shown in phase 1b trials to have better OS outcomes and progression-free survival compared to sorafenib [OS: 67.2%; (95%CI: 61.3 to 73.1) for the former and 54.6%, (95%CI: 45.2 to 64.0) for the latter]. Second-line therapies mainly included regorafenib, Cabozantinib, and Ramucirumab[184-186]. Phase 3 clinical trial studies have shown that Regorafenib has the best median survival time (10.6 months), followed by Cabozantinib (10.2 months), and Ramucirumab was the worst (8.5 months); common adverse events mainly include hypertension and skin reactions. The most common immunotherapy is immune checkpoint inhibitors to enhance existing immune responses. The use of ICI is becoming increasingly common[187]. There are successful trials showing the ability of ICI to improve survival[188]. Drugs include PD-L1 antibodies (durvalumab, atezolizumab, and avelumab), anti-PD-1 antibodies (nivolumab, pembrolizumab, and camrelizumab), and anti-CTLA-4 antibodies (ipilimumab and tremelimumab)[180]. Nivolumab, a PD-1 inhibitor, is currently used as a second-line systemic therapy for patients with advanced HCC. Median OS was 16.4 months (95%CI: 13.9-18.4) in the nivolumab group and 14.7 months (95%CI: 11.9-17.2) in the sorafenib group [risk ratio: 0.85; (95%CI: 0.72-1.02); P = 0.075]. Nivolumab is considered to have a better safety profile compared to sorafenib, although OS was not significantly improved[189].
The gemcitabine combined with cisplatin (GEMCIS) chemotherapy regimen has been selected for the first-line treatment of ICC[190]. Mutation-based therapies offer promising prospects for personalized treatment. Representative drugs include those targeting fibroblast growth factor receptors (FGFRs), isocitrate dehydrogenase-1 (IDH1), human epidermal growth factor receptor-2 (HER2), and the B-Raf proto-oncogene (BRAF)[191]. These drugs have positive therapeutic effects in ICC by specifically targeting genetic alterations[192]. ICC is associated with a rich and diverse TME[193,194]. TME may be associated with the generation of immune escape responses in CCA[195] and an important entry point for molecularly targeted therapies, immunotherapies, and combination therapies[196]. A growing body of research data support that various components of the tumor-responsive stroma (TRS) play a crucial role in the development of CCA[197,198]. Other studies have shown that regulation of PD-1 and PD-L1 is the key mechanism of immune escape in ICC[199].
PREVENTION
Antiviral therapy
Patients with active chronic hepatitis B, defined as HBV DNA greater than 2,000 IU/mL, alanine aminotransferase (ALT) above the upper limit of normal, or at least moderate hepatic necroinflammation or fibrosis, are candidates for treatment. In addition, candidates for treatment include patients with detectable HBV DNA, compensated or uncompensated cirrhosis, and a family history of HCC. Patients with extrahepatic manifestations are also candidates for treatment[200]. Those with serum HBeAg antibodies
HBV plays an important role in promoting hepatocarcinogenesis and unfavorable prognosis. We have demonstrated that the key mutations including C1673T, A1726C, A1727T, C1730G, C1766T, A1762T/G1764A, T1768A, C1773T, and C1799G in HBV genotype C significantly increased the risk of cirrhosis, and that the major HBV mutant haplotype 1653C-1674G/C1753V-1762T/1764A was detected 6.28 times more frequently in HCC than in non-HCC HBV-infected patients, with high specificity and high frequency of detection, making it applicable to HCC prediction[54]. HBV genotype C, PreS2 promoter variants, C105T, T1753V, A1762T/G1764A, and other HBV variants were found to be independent risk factors for HCC[55-57]. Through a systematic analysis of HBV mutations with high specificity and detection rate in HCC, we have found that the frequencies of PreS deletion and the C1653T, T1753V, and A1762T/G1764A mutations gradually increase at each stage of “triology” of HBV-induced hepatocarcinogenesis. Upon HBV infection, A1762T/G1764A mutation appears earlier, while PreS deletion, C1653T and T1753V mutations appear later[59]. HCC-associated mutations in the HBV pre-S region and the core promoter/enhancer II region have been found to accumulate progressively during the progression of chronic HBV infection to HCC, and these mutations and their combination predict the development of HCC[58]. Our cohort study has demonstrated that these combined mutations predict HCC development 6 years earlier[60]. The application of the combined detection technology of high-risk HBV mutations to monitor the occurrence of HCC in HBV-infected patients and to identify high-risk populations lays the foundation for the development of secondary prevention of HCC. HBV mutations are also predictive of HCC recurrence and poor prognosis. The presence of some HBV mutations such as A1762T/G1764A in serum not only predicts hepatocarcinogenesis, but also indicates the likelihood of HCC recurrence and metastasis, whereas HBV mutations in either cancerous or paracancerous tissues do not have such effects[61]. The G40C mutation in the pre-HBV S region also independently predicts a poor prognosis in HCC[62]. In this way, HBV evolution not only promotes HCC occurrence but also predicts a poor HCC prognosis, and thus serves as a marker for the prediction of HCC occurrence (diagnostic), prognosis (prognostic), and therapeutic efficacy (predictive), all of which play a guiding role in tertiary prevention of HBV-induced carcinogenesis.
Antiviral therapy for HCV includes Direct-Acting Antivirals (DAAs), PEG-IFN, NA, and ribavirin. The emergence of DAAs has led to an improvement in HCV antiviral therapy, with sustained viral response (SVR) rates of more than 90%[203]. Combination therapy is thought to have better efficacy. In a large cohort study of more than 3,000 first-treatment patients, IFN-ribavirin combined therapy was effective in reducing fibrosis and halting the progression of fibrosis in 92% of patients[204]. HCV mutations in the core genes including A028C, G209A, C219U/A, U264C, A271C/U, C378U/A, G435A/C, and G481A were significantly associated with an increased risk of HCC, whereas HCV U303C/A mutation was associated with a decreased risk of HCC[205]. There is a lack of studies on the association between characteristic HCV mutations and HCC. It remains to be explored whether the mechanism of HCV-HCC is similar to that described for HBV-HCC by Cancer Evo-Dev theory. This could be a potential breakthrough for future HCV surveillance and prevention.
Lifestyle interventions
Physical activity has a clear protective effect against HCC. Exercise not only significantly prevents the development of cancer, but also has a clear protective effect on all types of cancer-related deaths[206]. Physical activity is effective in improving insulin tolerance, promoting hepatic fatty acid metabolism, and modulating inflammatory molecules, which in turn has significant benefits for liver health. A large body of evidence has suggested that physical activity has a clear inhibitory effect on the development of PLC, particularly HCC, reducing the overall incidence of PLC by more than 20%. Non-professional sports of 7.5-15 h per week reduce the risk of PLC by 18%-27%, and moderate-intensity exercise reduces the risk of HCC by 23% and the risk of death from HCC by 19%[207]. Aggressive and effective clinical treatment combined with public health measures including aerobic exercise such as running and swimming is important for the tertiary prevention of HCC.
SPECIFIC PREVENTION AND TREATMENT FOR THE OCCURRENCE AND RECURRENCE OF HBV-HCC METASTASIS
Intrinsic factors resisting HCC recurrence and metastasis
From a study cohort of HBV-HCC patients with recurrence, tumor tissue samples were selected and subjected to high-throughput RNA sequencing, miRNA sequencing, and circRNA sequencing, resulting in the identification of 59,711 circRNA sequences, 55,752 of which were annotated in the CircAtlas database. The 3,959 novel circRNA sequences were analyzed using bioinformatics lineage enrichment analysis, identifying circRNAs significantly associated with recurrence and prognosis. Two of the most significant circRNAs, circKCNN2 and circGLS2, were experimentally validated. High expression of circKCNN2 in tumor tissues indicated a reduced risk of recurrence in patients. Ectopic expression of circKCNN2 in HCC cell lines significantly inhibited proliferation, migration, invasion, clonal formation, and in vivo tumorigenic capacity of HCC cells. The upstream transcriptional regulatory repressor nuclear factor Y α subunit (NFYA) and downstream effector molecules miR-520c-3p, MBD2, and FGFR4 of circKCNN2 were identified, demonstrating that circKCNN2 upregulated MBD2 expression through the adsorption of miR-520c-3p to inhibit the malignant phenotype of HCC. Studies utilizing HCC cell lines and organoids revealed that short-term overexpression of circKCNN2 produced a synergistic antitumor effect with Lenvatinib by inhibiting FGFR4[208,209]. This research offers a novel intervention for tertiary prevention, such as the recurrence of HBV-HCC.
Establishment of innovative technologies for tumor-specific gene therapy for HCC and other tumors
By leveraging the gene transcriptional regulatory sequences - promoters and enhancers - of HCC and liver tissue-specific molecules such as AFP and albumin, we have developed a cancer-specific gene therapy for the treatment of HCC. This approach has enabled recombinant retroviral vectors to express exogenous genes within HCC tissues, not in extrahepatic tissues. Notably, the expression of immune-regulatory factors in tumor tissues has significantly improved the tumor immune microenvironment, while avoiding damage to normal tissues, such as bone marrow. This strategy exerts potent antitumor effects[210,211]. This study introduces an effective and specific new measure for HCC-based cancer-specific prevention and treatment, representing a valuable attempt at targeted therapy for the microenvironment of HCC.
Establishment of a predictive index system and specific preventive measures for postoperative recurrence of HBV-HCC
In our established postoperative follow-up cohort study of post-HBV HCC, we identified independent risk factors for HCC postoperative recurrence and death. These include tumor diameter greater than 3 cm, incomplete tumor envelope, numerous satellite foci, microvascular infiltration, low tumor differentiation, advanced Barcelona staging, elevated gamma-glutamyl transpeptidase levels, and viral concentrations exceeding 104 copies/mL. Anti-HBV treatment emerged as the sole independent protective factor against HCC recurrence and death. A subsequent standard randomized controlled clinical trial, coupled with a multifactorial Cox proportional hazards model analysis, confirmed that standard antiviral therapy with NAs significantly reduced HBV-HCC postoperative recurrence [HR, 95%CI; 0.41, 0.32-0.70] and prolonged HCC-related death (0.26, 0.14-0.50). Importantly, antiviral therapy significantly reduced deaths within two years after HCC (0.41, 0.27-0.62) and significantly improved liver function in HBV-HCC patients at six months postoperatively (P < 0.0001), facilitating rapid recovery of postoperative residual liver size and function. Overall, specific antiviral therapy improved four-year postoperative survival by 82.3%. However, antiviral therapy did not exhibit these effects in patients with the integration of the X gene of 3’ truncated HBV (Ct-HBx) in the genome of the residual liver[212]. This finding has propelled postoperative antiviral therapy for HBV-HCC into clinical guidelines and raised a new question: the integration of Ct-HBx in the genome of the residual liver promotes recurrent metastasis, which can only be addressed by specifically splicing Ct-HBx in the genome of the residual liver in patients with Ct-HBx integration or by conducting specific gene therapy.
SUMMARY AND CONCLUSION
PLC as a global disease will likely continue to increase in number in the future, and although infectious factors are still the major risk factors, metabolic factors seem to be on the rise as the quality of life improves. China has the largest number of PLC cases, and HBV infection remains the major risk factor for HCC in the Chinese population. Cancer Evo-Dev has discovered the regulation of tumor suppressor genes, which provides new ideas for antitumor therapy. There is still a lack of studies on the relevance of HCV-characteristic mutated genes for HCC, and whether Cancer Evo-Dev can be applied to HCV-HCC remains to be clarified. Since the prognosis of PLC is extremely poor, its early monitoring and primary prevention are especially important. Abdominal ultrasound and serum marker tests are usually used as early screening tools for PLC because of their maturity and cheapness. However, its sensitivity remains suboptimal, and monitoring of relevant virus-specific genotypes in patients on combination antiviral therapy for infectious hepatitis may be an important preventive measure to achieve precise prevention and control of HBV/HCV-HCC. Surgical resection and liver transplantation are often applied for early-stage PLC, while systemic drugs are often used for intermediate and late-stage PLC, and the combination of multiple methods is considered to be the main strategy to improve the survival rate and time of patients. Vaccination, adopting a good lifestyle (abstaining from smoking and alcohol, avoiding a sedentary lifestyle, and eating more fruits and vegetables), and increasing physical activity are effective in preventing PLC.
DECLARATIONS
Authors’ contributions
Contributed to the conception and the design of the study, data search, and manuscript writing: Feng Y
Made contributions to conception and data search: Fang L
Supervised the article concept and revised the manuscript: Cao G
Availability of data and materials
Not applicable.
Financial support and sponsorship
This research was funded by the Shanghai Municipal Health Commission (GWVI-11.1-10).
Conflicts of interest
Cao G is the Editor-in-Chief of the journal Hepatoma Research. Cao G was not involved in any steps of editorial processing, notably including reviewers' selection, manuscript handling and decision making. The other authors declare that there are no conflicts of interest.
Ethical approval and consent to participate
Not applicable.
Consent for publication
Not applicable.
Copyright
© The author(s) 2025.
REFERENCES
1. Devarbhavi H, Asrani SK, Arab JP, Nartey YA, Pose E, Kamath PS. Global burden of liver disease: 2023 update. J Hepatol. 2023;79:516-37.
2. Bray F, Laversanne M, Sung H, et al. Global cancer statistics 2022: GLOBOCAN estimates of incidence and mortality worldwide for 36 cancers in 185 countries. CA Cancer J Clin. 2024;74:229-63.
3. Lin J, Zhang H, Yu H, et al. Epidemiological characteristics of primary liver cancer in mainland china from 2003 to 2020: a representative multicenter study. Front Oncol. 2022;12:906778.
4. GBD Results. Institute for Health Metrics and Evaluation. Available from: https://vizhub.healthdata.org/gbd-results. [Last accessed on 15 Jan 2025].
5. Yang JD, Hainaut P, Gores GJ, Amadou A, Plymoth A, Roberts LR. A global view of hepatocellular carcinoma: trends, risk, prevention and management. Nat Rev Gastroenterol Hepatol. 2019;16:589-604.
7. Rumgay H, Arnold M, Ferlay J, et al. Global burden of primary liver cancer in 2020 and predictions to 2040. J Hepatol. 2022;77:1598-606.
8. Petrick JL, Florio AA, Znaor A, et al. International trends in hepatocellular carcinoma incidence, 1978-2012. Int J Cancer. 2020;147:317-30.
9. Arnold M, Abnet CC, Neale RE, et al. Global burden of 5 major types of gastrointestinal cancer. Gastroenterology. 2020;159:335-49.e15.
10. Chang MH, Chen CJ, Lai MS, et al. Universal hepatitis B vaccination in Taiwan and the incidence of hepatocellular carcinoma in children. Taiwan childhood hepatoma study group. N Engl J Med. 1997;336:1855-9.
11. Marengo A, Rosso C, Bugianesi E. Liver cancer: connections with obesity, fatty liver, and cirrhosis. Annu Rev Med. 2016;67:103-17.
12. Florio AA, Ferlay J, Znaor A, et al. Global trends in intrahepatic and extrahepatic cholangiocarcinoma incidence from 1993 to 2012. Cancer. 2020;126:2666-78.
13. Chen W, Zheng R, Baade PD, et al. Cancer statistics in China, 2015. CA Cancer J Clin. 2016;66:115-32.
14. Vogel A, Meyer T, Sapisochin G, Salem R, Saborowski A. Hepatocellular carcinoma. Lancet. 2022;400:1345-62.
15. Ahn JC, Lee YT, Agopian VG, et al. Hepatocellular carcinoma surveillance: current practice and future directions. Hepatoma Res. 2022;8:10.
16. McGlynn KA, Petrick JL, El-Serag HB. Epidemiology of hepatocellular carcinoma. Hepatology. 2021;73 Suppl 1:4-13.
18. Li Z, Chen J, Tang Y, Qin D, Tang Z. MASLD: an emerging factor in the pathophysiology and clinical management of ICC. Hepatoma Res. 2024;10:41.
19. Turati F, Bertuccio P, Negri E, La Vecchia C. Epidemiology of cholangiocarcinoma. Hepatoma Res. 2022;8:19.
20. Yu Q, Lei Z, Ma W, et al. Postoperative prognosis of non-alcoholic fatty liver disease-associated intrahepatic cholangiocarcinoma: a multi-center propensity score matching analysis. J Gastrointest Surg. 2023;27:2403-13.
21. World Health Organization. Hepatitis B. Available from: https://www.who.int/news-room/fact-sheets/detail/hepatitis-b. [Last accessed on 15 Jan 2025].
22. World Health Organization. Hepatitis C. Available from: https://www.who.int/news-room/fact-sheets/detail/hepatitis-c. [Last accessed on 15 Jan 2025].
23. Asrani SK, Devarbhavi H, Eaton J, Kamath PS. Burden of liver diseases in the world. J Hepatol. 2019;70:151-71.
24. Tian Z, Xu C, Yang P, et al. Molecular pathogenesis: connections between viral hepatitis-induced and non-alcoholic steatohepatitis-induced hepatocellular carcinoma. Front Immunol. 2022;13:984728.
25. De Mitri MS, Poussin K, Baccarini P, et al. HCV-associated liver cancer without cirrhosis. Lancet. 1995;345:413-5.
26. World Health Organization (WHO). Available from: https://www.who.int/zh. [Last accessed on 15 Jan 2025].
27. International Diabetes Federation. 1 in 10 people are living with diabetes. Available from: https://idf.org/. [Last accessed on 15 Jan 2025].
28. Lazarus JV, Newsome PN, Francque SM, Kanwal F, Terrault NA, Rinella ME. Reply: a multi-society Delphi consensus statement on new fatty liver disease nomenclature. Hepatology. 2024;79:E93-4.
29. Huang DQ, El-Serag HB, Loomba R. Global epidemiology of NAFLD-related HCC: trends, predictions, risk factors and prevention. Nat Rev Gastroenterol Hepatol. 2021;18:223-38.
30. Wegermann K, Diehl AM, Moylan CA. Challenges and barriers in hepatocellular carcinoma (HCC) surveillance for patients with non-alcoholic fatty liver disease (NAFLD). Hepatoma Res. 2023;9:11.
31. Younossi ZM, Otgonsuren M, Henry L, et al. Association of nonalcoholic fatty liver disease (NAFLD) with hepatocellular carcinoma (HCC) in the United States from 2004 to 2009. Hepatology. 2015;62:1723-30.
32. Piciotti R, Longo M, Agresta A, et al. Old-fashioned and newly discovered biomarkers: the future of NAFLD-related HCC screening and monitoring. Hepatoma Res. 2022;8:37.
33. Desai A, Sandhu S, Lai JP, Sandhu DS. Hepatocellular carcinoma in non-cirrhotic liver: a comprehensive review. World J Hepatol. 2019;11:1-18.
34. Park EJ, Lee JH, Yu GY, et al. Dietary and genetic obesity promote liver inflammation and tumorigenesis by enhancing IL-6 and TNF expression. Cell. 2010;140:197-208.
35. Yang JD, Mohamed HA, Cvinar JL, Gores GJ, Roberts LR, Kim WR. Diabetes mellitus heightens the risk of hepatocellular carcinoma except in patients with hepatitis C cirrhosis. Am J Gastroenterol. 2016;111:1573-80.
36. Jinjuvadia R, Patel S, Liangpunsakul S. The association between metabolic syndrome and hepatocellular carcinoma: systemic review and meta-analysis. J Clin Gastroenterol. 2014;48:172-7.
37. Hydes TJ, Cuthbertson DJ, Graef S, et al. The impact of diabetes and glucose-lowering therapies on hepatocellular carcinoma incidence and overall survival. Clin Ther. 2022;44:257-68.
38. Lee JY, Jang SY, Nam CM, Kang ES. Incident hepatocellular carcinoma risk in patients treated with a sulfonylurea: a nationwide, nested, case-control study. Sci Rep. 2019;9:8532.
39. Zhang X, Goh GB, Chan WK, et al. Unhealthy lifestyle habits and physical inactivity among Asian patients with non-alcoholic fatty liver disease. Liver Int. 2020;40:2719-31.
40. Singh S, Singh PP, Singh AG, Murad MH, Sanchez W. Statins are associated with a reduced risk of hepatocellular cancer: a systematic review and meta-analysis. Gastroenterology. 2013;144:323-32.
41. Pradelli D, Soranna D, Scotti L, et al. Statins and primary liver cancer: a meta-analysis of observational studies. Eur J Cancer Prev. 2013;22:229-34.
42. Gregory SN, Perati SR, Brown ZJ. Alteration in immune function in patients with fatty liver disease. Hepatoma Res. 2022;8:31.
43. Llovet JM, Willoughby CE, Singal AG, et al. Nonalcoholic steatohepatitis-related hepatocellular carcinoma: pathogenesis and treatment. Nat Rev Gastroenterol Hepatol. 2023;20:487-503.
44. Bagnardi V, Rota M, Botteri E, et al. Alcohol consumption and site-specific cancer risk: a comprehensive dose-response meta-analysis. Br J Cancer. 2015;112:580-93.
45. World Health Organization. Global status report on alcohol and health 2018. Available from: https://www.who.int/publications/i/item/9789241565639. [Last accessed on 15 Jan 2025].
46. Huang DQ, Mathurin P, Cortez-Pinto H, Loomba R. Global epidemiology of alcohol-associated cirrhosis and HCC: trends, projections and risk factors. Nat Rev Gastroenterol Hepatol. 2023;20:37-49.
47. Chidambaranathan-reghupaty S, Fisher PB, Sarkar D. Hepatocellular carcinoma (HCC): epidemiology, etiology and molecular classification. Adv Cancer Res. 2021;149:1-61.
48. Liu SY, Tsai IT, Hsu YC. Alcohol-related liver disease: basic mechanisms and clinical perspectives. Int J Mol Sci. 2021;22:5170.
49. Tsai MC, Yang SS, Lin CC, et al. Association of heavy alcohol intake and ALDH2 rs671 polymorphism with hepatocellular carcinoma and mortality in patients with hepatitis B virus-related cirrhosis. JAMA Netw Open. 2022;5:e2223511.
50. Su D. Drinking water and liver cell cancer: an epidemiologic approach to the etiology of this disease in China. Chin Med J. 1979;92:748-56. Available from: https://pubmed.ncbi.nlm.nih.gov/116818/. [Last accessed on 15 Jan 2025].
51. Jin J, Kouznetsova VL, Kesari S, Tsigelny IF. Synergism in actions of HBV with aflatoxin in cancer development. Toxicology. 2023;499:153652.
52. Liu Y, Wu F. Global burden of aflatoxin-induced hepatocellular carcinoma: a risk assessment. Environ Health Perspect. 2010;118:818-24.
53. Sun J, Althoff KN, Jing Y, et al; North American AIDS Cohort Collaboration on Research and Design of IeDEA. Trends in hepatocellular carcinoma incidence and risk among persons with HIV in the US and Canada, 1996-2015. JAMA Netw Open. 2021;4:e2037512.
54. Yin J, Xie J, Liu S, et al. Association between the various mutations in viral core promoter region to different stages of hepatitis B, ranging of asymptomatic carrier state to hepatocellular carcinoma. Am J Gastroenterol. 2011;106:81-92.
55. Yin J, Xie J, Zhang H, et al. Significant association of different preS mutations with hepatitis B-related cirrhosis or hepatocellular carcinoma. J Gastroenterol. 2010;45:1063-71.
56. Liu S, Xie J, Yin J, et al. A matched case-control study of hepatitis B virus mutations in the preS and core promoter regions associated independently with hepatocellular carcinoma. J Med Virol. 2011;83:45-53.
57. Xie JX, Zhao J, Yin JH, et al. Association of novel mutations and haplotypes in the preS region of hepatitis B virus with hepatocellular carcinoma. Front Med China. 2010;4:419-29.
58. Liu S, Zhang H, Gu C, et al. Associations between hepatitis B virus mutations and the risk of hepatocellular carcinoma: a meta-analysis. J Natl Cancer Inst. 2009;101:1066-82.
59. Li Z, Xie Z, Ni H, et al. Mother-to-child transmission of hepatitis B virus: evolution of hepatocellular carcinoma-related viral mutations in the post-immunization era. J Clin Virol. 2014;61:47-54.
60. Yin J, Wang J, Pu R, et al. Hepatitis B virus combo mutations improve the prediction and active prophylaxis of hepatocellular carcinoma: a clinic-based cohort study. Cancer Prev Res. 2015;8:978-88.
61. Yin J, Chen X, Li N, et al. Compartmentalized evolution of hepatitis B virus contributes differently to the prognosis of hepatocellular carcinoma. Carcinogenesis. 2021;42:461-70.
62. Chen X, Zhang M, Li N, et al. Nucleotide variants in hepatitis B virus preS region predict the recurrence of hepatocellular carcinoma. Aging. 2021;13:22256-75.
63. Pu R, Liu W, Zhou X, et al. The effects and underlying mechanisms of hepatitis B virus X gene mutants on the development of hepatocellular carcinoma. Front Oncol. 2022;12:836517.
64. Liu W, Cai S, Pu R, et al. HBV preS mutations promote hepatocarcinogenesis by inducing endoplasmic reticulum stress and upregulating inflammatory signaling. Cancers. 2022;14:3274.
65. Jiang DK, Sun J, Cao G, et al. Genetic variants in STAT4 and HLA-DQ genes confer risk of hepatitis B virus-related hepatocellular carcinoma. Nat Genet. 2013;45:72-5.
66. Shen T, Ni T, Chen J, et al. An enhancer variant at 16q22.1 predisposes to hepatocellular carcinoma via regulating PRMT7 expression. Nat Commun. 2022;13:1232.
67. Zhang Q, Yin J, Zhang Y, et al. HLA-DP polymorphisms affect the outcomes of chronic hepatitis B virus infections, possibly through interacting with viral mutations. J Virol. 2013;87:12176-86.
68. Song J, Yang F, Wang S, et al. Efficacy and safety of antiviral treatment on blocking the mother-to-child transmission of hepatitis B virus: a meta-analysis. J Viral Hepat. 2019;26:397-406.
69. Ji X, Zhang Q, Li B, et al. Impacts of human leukocyte antigen DQ genetic polymorphisms and their interactions with hepatitis B virus mutations on the risks of viral persistence, liver cirrhosis, and hepatocellular carcinoma. Infect Genet Evol. 2014;28:201-9.
70. He Y, Zhang H, Yin J, et al. IkappaBalpha gene promoter polymorphisms are associated with hepatocarcinogenesis in patients infected with hepatitis B virus genotype C. Carcinogenesis. 2009;30:1916-22.
71. Zhang Q, Ji XW, Hou XM, et al. Effect of functional nuclear factor-kappaB genetic polymorphisms on hepatitis B virus persistence and their interactions with viral mutations on the risk of hepatocellular carcinoma. Ann Oncol. 2014;25:2413-9.
72. Xie J, Zhang Y, Zhang Q, et al. Interaction of signal transducer and activator of transcription 3 polymorphisms with hepatitis B virus mutations in hepatocellular carcinoma. Hepatology. 2013;57:2369-77.
73. Liu W, Wu J, Yang F, et al. Genetic polymorphisms predisposing the interleukin 6-induced APOBEC3B-UNG imbalance increase HCC risk via promoting the generation of APOBEC-signature HBV mutations. Clin Cancer Res. 2019;25:5525-36.
74. Liu W, Ji H, Zhao J, et al. Transcriptional repression and apoptosis influence the effect of APOBEC3A/3B functional polymorphisms on biliary tract cancer risk. Int J Cancer. 2022;150:1825-37.
75. Tan X, Zheng S, Liu W, et al. Effect of APOBEC3A functional polymorphism on renal cell carcinoma is influenced by tumor necrosis factor-α and transcriptional repressor ETS1. Am J Cancer Res. 2021;11:4347-63. Available from: https://www.worldobesity.org/resources/resource-library/world-obesity-atlas-2023. [Last accessed on 15 Jan 2025].
76. Xian L, Zhao P, Chen X, et al. Heterogeneity, inherent and acquired drug resistance in patient-derived organoid models of primary liver cancer. Cell Oncol. 2022;45:1019-36.
77. Chang W, Gao X, Han Y, et al. Gene expression profiling-derived immunohistochemistry signature with high prognostic value in colorectal carcinoma. Gut. 2014;63:1457-67.
78. Cancer Genome Atlas Research Network. Electronic address: [email protected]; Cancer Genome Atlas Research Network. Comprehensive and integrative genomic characterization of hepatocellular carcinoma. Cell. 2017;169:1327-41.e23.
79. Désert R, Rohart F, Canal F, et al. Human hepatocellular carcinomas with a periportal phenotype have the lowest potential for early recurrence after curative resection. Hepatology. 2017;66:1502-18.
80. Tan JH, Zhou WY, Zhou L, Cao RC, Zhang GW. Viral hepatitis B and C infections increase the risks of intrahepatic and extrahepatic cholangiocarcinoma: evidence from a systematic review and meta-analysis. Turk J Gastroenterol. 2020;31:246-56.
81. Zhang H, Zhu B, Zhang H, Liang J, Zeng W. HBV infection status and the risk of cholangiocarcinoma in Asia: a meta-analysis. Biomed Res Int. 2016;2016:3417976.
82. Li M, Li J, Li P, et al. Hepatitis B virus infection increases the risk of cholangiocarcinoma: a meta-analysis and systematic review. J Gastroenterol Hepatol. 2012;27:1561-8.
83. Palmer WC, Patel T. Are common factors involved in the pathogenesis of primary liver cancers? J Hepatol. 2012;57:69-76.
84. Zhou Y, Zhao Y, Li B, et al. Hepatitis viruses infection and risk of intrahepatic cholangiocarcinoma: evidence from a meta-analysis. BMC Cancer. 2012;12:289.
85. Labib PL, Goodchild G, Pereira SP. Molecular pathogenesis of cholangiocarcinoma. BMC Cancer. 2019;19:185.
86. Wang C, Yang W, Yan HX, et al. Hepatitis B virus X (HBx) induces tumorigenicity of hepatic progenitor cells in 3,5-diethoxycarbonyl-1,4-dihydrocollidine-treated HBx transgenic mice. Hepatology. 2012;55:108-20.
87. Gao C, Zhou G, Shi J, et al. The A-to-I editing of KPC1 promotes intrahepatic cholangiocarcinoma by attenuating proteasomal processing of NF-κB1 p105 to p50. J Exp Clin Cancer Res. 2022;41:338.
88. Beal EW, Gorji L, Volney J, Sova L, Mcalearney AS, Tsung A. Barriers to surveillance for hepatocellular cancer among patients with chronic liver disease -providers’ perspectives. Hepatoma Res. 2023;9:45.
89. Lee YT, Fujiwara N, Yang JD, Hoshida Y. Risk stratification and early detection biomarkers for precision HCC screening. Hepatology. 2023;78:319-62.
90. Mózes FE, Lee JA, Selvaraj EA, et al; LITMUS Investigators. Diagnostic accuracy of non-invasive tests for advanced fibrosis in patients with NAFLD: an individual patient data meta-analysis. Gut. 2022;71:1006-19.
91. Hydes TJ, Cuthbertson DJ, Palmer DH, et al. Ultrasonography in surveillance for hepatocellular carcinoma in patients with non-alcoholic fatty liver disease. Hepatoma Res. 2023;9:12.
92. Singal AG, Llovet JM, Yarchoan M, et al. AASLD Practice Guidance on prevention, diagnosis, and treatment of hepatocellular carcinoma. Hepatology. 2023;78:1922-65.
93. Omata M, Cheng AL, Kokudo N, et al. Asia-Pacific clinical practice guidelines on the management of hepatocellular carcinoma: a 2017 update. Hepatol Int. 2017;11:317-70.
94. Vogel A, Cervantes A, Chau I, et al; ESMO Guidelines Committee. Hepatocellular carcinoma: ESMO Clinical Practice Guidelines for diagnosis, treatment and follow-up. Ann Oncol. 2018;29:iv238-55.
95. European Association for the Study of the Liver. EASL Clinical Practice Guidelines: management of hepatocellular carcinoma. J Hepatol. 2018;69:182-236.
96. Hasegawa K, Takemura N, Yamashita T, et al; committee for Revision of the Clinical Practice Guidelines for Hepatocellular Carcinoma, Tokyo, Japan. Clinical Practice Guidelines for hepatocellular carcinoma: the Japan society of hepatology 2021 version (5th JSH-HCC Guidelines). Hepatol Res. 2023;53:383-90.
97. Colli A, Fraquelli M, Casazza G, et al. Accuracy of ultrasonography, spiral CT, magnetic resonance, and alpha-fetoprotein in diagnosing hepatocellular carcinoma: a systematic review. Am J Gastroenterol. 2006;101:513-23.
98. Kim SY, An J, Lim YS, et al. MRI with liver-specific contrast for surveillance of patients with cirrhosis at high risk of hepatocellular carcinoma. JAMA Oncol. 2017;3:456-63.
99. Park J, Lee JM, Kim TH, Yoon JH. Imaging diagnosis of hepatocellular carcinoma: future directions with special emphasis on hepatobiliary magnetic resonance imaging and contrast-enhanced ultrasound. Clin Mol Hepatol. 2022;28:362-79.
100. Tzartzeva K, Obi J, Rich NE, et al. Surveillance imaging and alpha fetoprotein for early detection of hepatocellular carcinoma in patients with cirrhosis: a meta-analysis. Gastroenterology. 2018;154:1706-18.e1.
101. Singal A, Volk ML, Waljee A, et al. Meta-analysis: surveillance with ultrasound for early-stage hepatocellular carcinoma in patients with cirrhosis. Aliment Pharmacol Ther. 2009;30:37-47.
102. Yu NC, Chaudhari V, Raman SS, et al. CT and MRI improve detection of hepatocellular carcinoma, compared with ultrasound alone, in patients with cirrhosis. Clin Gastroenterol Hepatol. 2011;9:161-7.
103. Samoylova ML, Mehta N, Roberts JP, Yao FY. Predictors of ultrasound failure to detect hepatocellular carcinoma. Liver Transpl. 2018;24:1171-7.
104. Goh MJ, Sinn DH, Kim JM, et al. Clinical practice guideline and real-life practice in hepatocellular carcinoma: a Korean perspective. Clin Mol Hepatol. 2023;29:197-205.
105. Yip TC, Lee HW, Chan WK, Wong GL, Wong VW. Asian perspective on NAFLD-associated HCC. J Hepatol. 2022;76:726-34.
106. Bridgewater J, Galle PR, Khan SA, et al. Guidelines for the diagnosis and management of intrahepatic cholangiocarcinoma. J Hepatol. 2014;60:1268-89.
107. Cai Y, Cheng N, Ye H, Li F, Song P, Tang W. The current management of cholangiocarcinoma: a comparison of current guidelines. Biosci Trends. 2016;10:92-102.
108. Cabibbo G, Daniele B, Borzio M, et al. Multidisciplinary treatment of hepatocellular carcinoma in 2023: Italian practice treatment guidelines of the Italian association for the study of the liver (AISF), Italian association of medical oncology (AIOM), Italian ASSOCIATION OF HEPATO-BILIO-PANCREATIC SURGery (AICEP), Italian association of hospital gastroenterologists (AIGO), Italian association of radiology and clinical oncology (AIRO), Italian society of pathological anatomy and diagnostic cytology (SIAPeC-IAP), Italian society of surgery (SIC), Italian society of gastroenterology (SIGE), Italian society of medical and interventional radiology (SIRM), Italian organ transplant society (SITO), and association of patients with hepatitis and liver disease (EpaC) - part I - surgical treatments. Dig Liver Dis. 2024;56:223-34.
109. Ricke J, Seidensticker M, Mohnike K. Noninvasive diagnosis of hepatocellular carcinoma in cirrhotic liver: current guidelines and future prospects for radiological imaging. Liver Cancer. 2012;1:51-8.
110. Harris PS, Hansen RM, Gray ME, Massoud OI, McGuire BM, Shoreibah MG. Hepatocellular carcinoma surveillance: an evidence-based approach. World J Gastroenterol. 2019;25:1550-9.
111. Singal AG, El-Serag HB. Rational HCC screening approaches for patients with NAFLD. J Hepatol. 2022;76:195-201.
112. Rao SX, Wang J, Wang J, et al. Chinese consensus on the clinical application of hepatobiliary magnetic resonance imaging contrast agent: Gadoxetic acid disodium. J Dig Dis. 2019;20:54-61.
113. Mao Y, Wang J, Zhu Y, et al. Gd-EOB-DTPA-enhanced MRI radiomic features for predicting histological grade of hepatocellular carcinoma. Hepatobiliary Surg Nutr. 2022;11:13-24.
114. Xie D, Shi J, Zhou J, Fan J, Gao Q. Clinical practice guidelines and real-life practice in hepatocellular carcinoma: a Chinese perspective. Clin Mol Hepatol. 2023;29:206-16.
116. Gisder DM, Tannapfel A, Tischoff I. Histopathology of hepatocellular carcinoma - when and what. Hepatoma Res. 2022;8:4.
117. Forner A, Vilana R, Ayuso C, et al. Diagnosis of hepatic nodules 20 mm or smaller in cirrhosis: prospective validation of the noninvasive diagnostic criteria for hepatocellular carcinoma. Hepatology. 2008;47:97-104.
118. Xing H, Zheng YJ, Han J, et al. Protein induced by vitamin K absence or antagonist-II versus alpha-fetoprotein in the diagnosis of hepatocellular carcinoma: a systematic review with meta-analysis. Hepatobiliary Pancreat Dis Int. 2018;17:487-95.
119. Tayob N, Kanwal F, Alsarraj A, Hernaez R, El-Serag HB. The performance of AFP, AFP-3, DCP as Biomarkers for detection of hepatocellular carcinoma (HCC): a phase 3 biomarker study in the United States. Clin Gastroenterol Hepatol. 2023;21:415-23.e4.
120. Zhang X, Xu Y, Qian Z, et al. circRNA_104075 stimulates YAP-dependent tumorigenesis through the regulation of HNF4a and may serve as a diagnostic marker in hepatocellular carcinoma. Cell Death Dis. 2018;9:1091.
121. Cai J, Chen L, Zhang Z, et al. Genome-wide mapping of 5-hydroxymethylcytosines in circulating cell-free DNA as a non-invasive approach for early detection of hepatocellular carcinoma. Gut. 2019;68:2195-205.
122. Kim AK, Hamilton JP, Lin SY, et al. Urine DNA biomarkers for hepatocellular carcinoma screening. Br J Cancer. 2022;126:1432-8.
123. Corte A, Di Gaeta E, Steidler S, De Cobelli F. Radiological imaging and non-surgical local treatments for cholangiocarcinoma. Hepatoma Res. 2022;8:5.
124. Hamaoka M, Kozaka K, Matsui O, et al. Early detection of intrahepatic cholangiocarcinoma. Jpn J Radiol. 2019;37:669-84.
125. Bragazzi MC, Venere R, Ribichini E, Covotta F, Cardinale V, Alvaro D. Intrahepatic cholangiocarcinoma: evolving strategies in management and treatment. Dig Liver Dis. 2024;56:383-93.
126. Krenzien F, Nevermann N, Krombholz A, et al. Treatment of intrahepatic cholangiocarcinoma-a multidisciplinary approach. Cancers. 2022;14:362.
127. Muñoz-martínez S, Rimola J, Londoño MC, Cárdenas A, Forner A. Cholangiocarcinoma: early detection and screening in high-risk population. Hepatoma Res. 2022;8:30.
128. Choi SH, Lee SS, Kim SY, et al. Intrahepatic cholangiocarcinoma in patients with cirrhosis: differentiation from hepatocellular carcinoma by using gadoxetic acid-enhanced MR imaging and dynamic CT. Radiology. 2017;282:771-81.
129. Lu T, Ma J, Zou J, Jiang C, Li Y, Han J. CT-based intratumoral and peritumoral deep transfer learning features prediction of lymph node metastasis in non-small cell lung cancer. J Xray Sci Technol. 2024;32:597-609.
130. Wang H, Zhang J, Li Y, et al. Deep-learning features based on F18 fluorodeoxyglucose positron emission tomography/computed tomography (18F-FDG PET/CT) to predict preoperative colorectal cancer lymph node metastasis. Clin Radiol. 2024;79:e1152-8.
131. Zhu Y, Fu C, Du J, Jin Y, Du S, Zhao F. Prediction of cervical cancer lymph node metastasis via a multimodal transfer learning approach. Br J Hosp Med. 2024;85:1-14.
132. Zhang B, Jin B, Wu X, et al. Investigation of transcriptional and immunological disparities among patient groups with varied prognostic risk factors in cholangiocarcinoma. Cancer Med. 2024;13:e70135.
133. Llovet JM, Brú C, Bruix J. Prognosis of hepatocellular carcinoma: the BCLC staging classification. Semin Liver Dis. 1999;19:329-38.
134. Wu Y, Wakil A, Salomon F, Pyrsopoulos N. Issue on combined locoregional and systemic treatment for hepatocellular carcinoma. Hepatoma Res. 2023;9:6.
135. Roayaie S, Obeidat K, Sposito C, et al. Resection of hepatocellular cancer ≤ 2 cm: results from two Western centers. Hepatology. 2013;57:1426-35.
136. Zhou J, Sun H, Wang Z, et al. Guidelines for the diagnosis and treatment of hepatocellular carcinoma (2019 Edition). Liver Cancer. 2020;9:682-720.
137. Tabrizian P, Jibara G, Shrager B, Schwartz M, Roayaie S. Recurrence of hepatocellular cancer after resection: patterns, treatments, and prognosis. Ann Surg. 2015;261:947-55.
138. Koh YX, Tan HJ, Liew YX, et al. Liver resection for nonalcoholic fatty liver disease-associated hepatocellular carcinoma. J Am Coll Surg. 2019;229:467-78.e1.
139. Molinari M, Kaltenmeier C, Samra PB, et al. Hepatic resection for hepatocellular carcinoma in nonalcoholic fatty liver disease: a systematic review and meta-analysis of 7226 patients. Ann Surg Open. 2021;2:e065.
140. Kung JWC, Ng KKC. Role of locoregional therapies in the management of patients with hepatocellular carcinoma. Hepatoma Res. 2022;8:17.
141. Cassese G, Han H, Lee B, Lee HW, Cho JY, Troisi R. The role of minimally invasive surgery in the treatment of HCC. Hepatoma Res. 2022;8:26.
142. Squires MH, Cloyd JM, Dillhoff M, Schmidt C, Pawlik TM. Challenges of surgical management of intrahepatic cholangiocarcinoma. Expert Rev Gastroenterol Hepatol. 2018;12:671-81.
143. Dopazo C, Charco R. Liver transplantation for perihilar cholangiocarcinoma. Do we need to move forward? Hepatoma Res. 2023;9:10.
144. Qin D, Chen J, Tang Y, et al. Evaluation of the outcomes of staging laparoscopy in intrahepatic cholangiocarcinoma patients undergoing surgical resection: a multicenter retrospective study in China. Hepatoma Res. 2024;10:40.
145. Aliseda D, Sapisochin G, Martí-Cruchaga P, et al. Association of laparoscopic surgery with improved perioperative and survival outcomes in patients with resectable intrahepatic cholangiocarcinoma: a systematic review and meta-analysis from propensity-score matched studies. Ann Surg Oncol. 2023;30:4888-901.
146. Umeda Y, Takagi K, Matsuda T, et al. Clinical implications and optimal extent of lymphadenectomy for intrahepatic cholangiocarcinoma: a multicenter analysis of the therapeutic index. Ann Gastroenterol Surg. 2023;7:512-22.
147. Sha M, Cao J, Qin CL, et al. Impact of lymph node dissection for patients with clinically node-negative intrahepatic cholangiocarcinoma: a multicenter cohort study. World J Oncol. 2024;15:579-91.
148. Ishii T, Iwaki K, Nakakura A, Yoh T, Uchida Y, Hatano E. Is routine lymph node dissection recommended for liver resection of intrahepatic cholangiocarcinoma? HPB. 2024;26:731-40.
149. Mazzaferro V, Regalia E, Doci R, et al. Liver transplantation for the treatment of small hepatocellular carcinomas in patients with cirrhosis. N Engl J Med. 1996;334:693-9.
150. Tabrizian P, Holzner ML, Mehta N, et al. Ten-year outcomes of liver transplant and downstaging for hepatocellular carcinoma. JAMA Surg. 2022;157:779-88.
151. Franssen B, Jibara G, Tabrizian P, Schwartz ME, Roayaie S. Actual 10-year survival following hepatectomy for hepatocellular carcinoma. HPB. 2014;16:830-5.
152. Mok JHM, Ma KW, Chok KSH. Experience of living donor liver transplantation for hepatocellular carcinoma in the University of Hong Kong Hospital. Hepatoma Res. 2022;8:6.
153. Cucchetti A, Zhong J, Berhane S, et al. The chances of hepatic resection curing hepatocellular carcinoma. J Hepatol. 2020;72:711-7.
154. Llovet JM, Fuster J, Bruix J. Intention-to-treat analysis of surgical treatment for early hepatocellular carcinoma: resection versus transplantation. Hepatology. 1999;30:1434-40.
155. Novoa F, Ardiles V, Pekolj J, Mattera J, de Santibañes M. Liver transplantation for intrahepatic cholangiocarcinoma: a narrative review of the latest advances. Hepatoma Res. 2023;9:33.
156. Sturdevant M, Zidan A, Broering D. Robotic donor hepatectomy: a niche advancement or the way forward? Hepatoma Res. 2022;8:1.
157. Kulik L, Heimbach JK, Zaiem F, et al. Therapies for patients with hepatocellular carcinoma awaiting liver transplantation: a systematic review and meta-analysis. Hepatology. 2018;67:381-400.
158. Yao FY, Mehta N, Flemming J, et al. Downstaging of hepatocellular cancer before liver transplant: long-term outcome compared to tumors within Milan criteria. Hepatology. 2015;61:1968-77.
159. Llovet JM, De Baere T, Kulik L, et al. Locoregional therapies in the era of molecular and immune treatments for hepatocellular carcinoma. Nat Rev Gastroenterol Hepatol. 2021;18:293-313.
160. Ng KKC, Chok KSH, Chan ACY, et al. Randomized clinical trial of hepatic resection versus radiofrequency ablation for early-stage hepatocellular carcinoma. Br J Surg. 2017;104:1775-84.
161. Xia Y, Li J, Liu G, et al. Long-term effects of repeat hepatectomy vs percutaneous radiofrequency ablation among patients with recurrent hepatocellular carcinoma: a randomized clinical trial. JAMA Oncol. 2020;6:255-63.
162. Chen L, Zhang Q, Chang W, Du Y, Zhang H, Cao G. Viral and host inflammation-related factors that can predict the prognosis of hepatocellular carcinoma. Eur J Cancer. 2012;48:1977-87.
163. Heimbach JK, Kulik LM, Finn RS, et al. AASLD guidelines for the treatment of hepatocellular carcinoma. Hepatology. 2018;67:358-80.
164. Renzulli M, Gentilini M, Marasco G, et al. The duration of the conventional chemoembolization for hepatocellular carcinoma: factors affecting the procedural time. Hepatoma Res. 2022;8:27.
165. Woerner AJ, Johnson GE. Advances in Y-90 radioembolization for the treatment of hepatocellular carcinoma. Hepatoma Res. 2022;8:2.
166. Zhang Y, Li Y, Ji H, Zhao X, Lu H. Transarterial Y90 radioembolization versus chemoembolization for patients with hepatocellular carcinoma: a meta-analysis. Biosci Trends. 2015;9:289-98.
167. An T, Wehrenberg-Klee E. Trans-arterial chemoembolization and radioembolization for treatment of intrahepatic cholangiocarcinoma. Hepatoma Res. 2023;9:43.
168. Owen M, Makary MS, Beal EW. Locoregional therapy for intrahepatic cholangiocarcinoma. Cancers. 2023;15:2384.
169. Melchiorre F, Patella F, Pescatori L, et al. DEB-TACE: a standard review. Future Oncol. 2018;14:2969-84.
170. Cho Y, Choi JW, Kwon H, et al; Research Committee of the Korean Liver Cancer Association. Transarterial chemoembolization for hepatocellular carcinoma: 2023 expert consensus-based practical recommendations of the Korean liver cancer association. Clin Mol Hepatol. 2023;29:521-41
171. Gu Y, Zhang T, Zuo M, et al. Hepatic artery infusion chemotherapy (HAIC) combined with apatinib and camrelizumab for hepatocellular carcinoma (HCC) in BCLC stage c: a prospective, single-arm, phase II trial (TRIPLET study). JCO. 2022;40:4106.
172. Liu D, Mu H, Liu C, et al. Hepatic artery infusion chemotherapy (HAIC) combined with sintilimab and bevacizumab biosimilar (IBI305) for initial unresectable hepatocellular carcinoma (HCC): a prospective, single-arm phase II trial. JCO. 2022;40:4073.
173. Ke Q, Lin N, Deng M, Wang L, Zeng Y, Liu J. The effect of adjuvant therapy for patients with intrahepatic cholangiocarcinoma after surgical resection: a systematic review and meta-analysis. PLoS One. 2020;15:e0229292.
174. Sheka AC, Altman A, Marmor S, et al. Failure to administer multimodality therapy leads to sub-optimal outcomes for patients with node-positive biliary tract cancers in the United States. Surg Oncol. 2020;34:298-303.
175. Vogel A, Martinelli E; ESMO Guidelines Committee. Electronic address: [email protected]; ESMO Guidelines Committee. Updated treatment recommendations for hepatocellular carcinoma (HCC) from the ESMO clinical practice guidelines. Ann Oncol. 2021;32:801-5
176. Bruix J, Chan SL, Galle PR, Rimassa L, Sangro B. Systemic treatment of hepatocellular carcinoma: an EASL position paper. J Hepatol. 2021;75:960-74.
177. Su GL, Altayar O, O'Shea R, et al. AGA clinical practice guideline on systemic therapy for hepatocellular carcinoma. Gastroenterology. 2022;162:920-34.
178. Fu C, Chen H, Chen Y, Liu W, Cao G. Transarterial intervention therapy combined with systemic therapy for HCC: a review of recent five-year articles. Hepatoma Res. 2024;10:42.
179. Zhou H, Song T. Conversion therapy and maintenance therapy for primary hepatocellular carcinoma. Biosci Trends. 2021;15:155-60.
180. Cheng H, Sun G, Chen H, et al. Trends in the treatment of advanced hepatocellular carcinoma: immune checkpoint blockade immunotherapy and related combination therapies. Am J Cancer Res. 2019;9:1536-45.
181. Bteich F, Di Bisceglie AM. Current and future systemic therapies for hepatocellular carcinoma. Gastroenterol hepatol. 2019;15:266-72.
182. Llovet JM, Ricci S, Mazzaferro V, et al; SHARP Investigators Study Group. Sorafenib in advanced hepatocellular carcinoma. N Engl J Med. 2008;359:378-90
183. Kudo M, Finn RS, Qin S, et al. Lenvatinib versus sorafenib in first-line treatment of patients with unresectable hepatocellular carcinoma: a randomised phase 3 non-inferiority trial. Lancet. 2018;391:1163-73.
184. Abou-Alfa GK, Meyer T, Cheng AL, et al. Cabozantinib in patients with advanced and progressing hepatocellular carcinoma. N Engl J Med. 2018;379:54-63.
185. Bruix J, Qin S, Merle P, et al; RESORCE Investigators. Regorafenib for patients with hepatocellular carcinoma who progressed on sorafenib treatment (RESORCE): a randomised, double-blind, placebo-controlled, phase 3 trial. Lancet. 2017;389:56-66
186. Zhu AX, Kang YK, Yen CJ, et al; REACH-2 study investigators. Ramucirumab after sorafenib in patients with advanced hepatocellular carcinoma and increased α-fetoprotein concentrations (REACH-2): a randomised, double-blind, placebo-controlled, phase 3 trial. Lancet Oncol. 2019;20:282-96
187. Anugwom CM, Leventhal TM, Debes JD. Understanding immune perspectives and options for the use of checkpoint immunotherapy in HCC post liver transplant. Hepatoma Res. 2022;8:7.
188. Greten TF, Villanueva A, Korangy F, et al. Biomarkers for immunotherapy of hepatocellular carcinoma. Nat Rev Clin Oncol. 2023;20:780-98.
189. Yau T, Park JW, Finn RS, et al. Nivolumab versus sorafenib in advanced hepatocellular carcinoma (CheckMate 459): a randomised, multicentre, open-label, phase 3 trial. Lancet Oncol. 2022;23:77-90.
190. Zeng TM, Pan YF, Yuan ZG, Chen DS, Song YJ, Gao Y. Immune-related RNA signature predicts outcome of PD-1 inhibitor-combined GEMCIS therapy in advanced intrahepatic cholangiocarcinoma. Front Immunol. 2022;13:943066.
191. Sy, Ye YH, Zhou ZJ, Fan J, Zhou J, Zhou SL. Mutation-based therapies for intrahepatic cholangiocarcinoma: new options on the horizon. Hepatoma Res. 2024;10:3.
192. Serifis N, Tsilimigras DI, Cloonan DJ, Pawlik TM. Challenges and opportunities for treating intrahepatic cholangiocarcinoma. Hepat Med. 2021;13:93-104.
193. Wei P, Li Z. Advancing immunotherapy for intrahepatic cholangiocarcinoma: exploring the tumor immune microenvironment and innovative treatments. Hepatoma Res. 2024;10:39.
194. Vlerken-Ysla L, Tyurina YY, Kagan VE, Gabrilovich DI. Functional states of myeloid cells in cancer. Cancer Cell. 2023;41:490-504.
195. Cigliano A, Strain AJ, Cadamuro M. Signaling and molecular networks related to development and inflammation involved in CCA initiation and progression. Hepatoma Res. 2023;9:14.
196. Cadamuro M, Fabris L, Zhang X, Strazzabosco M. Tumor microenvironment and immunology of cholangiocarcinoma. Hepatoma Res. 2022;8:11.
197. Brivio S, Cadamuro M, Strazzabosco M, Fabris L. Tumor reactive stroma in cholangiocarcinoma: the fuel behind cancer aggressiveness. World J Hepatol. 2017;9:455-68.
198. Caligiuri A, Parola M, Marra F, Cannito S, Gentilini A. Cholangiocarcinoma tumor microenvironment highlighting fibrosis and matrix components. Hepatoma Res. 2023;9:30.
199. Gim G, Badri N. Challenges and opportunities for treating intrahepatic cholangiocarcinoma: targeted therapy. Hepatoma Res. 2023;9:51.
200. Cacoub P, Asselah T. Hepatitis B virus infection and extra-hepatic manifestations: a systemic disease. Am J Gastroenterol. 2022;117:253-63.
201. Asselah T, Loureiro D, Boyer N, Mansouri A. Targets and future direct-acting antiviral approaches to achieve hepatitis B virus cure. Lancet Gastroenterol Hepatol. 2019;4:883-92.
202. Nasser N, Tonnerre P, Mansouri A, Asselah T. Hepatitis-B virus: replication cycle, targets, and antiviral approaches. Curr Opin Virol. 2023;63:101360.
203. Wirth TC, Manns MP. The impact of the revolution in hepatitis C treatment on hepatocellular carcinoma. Ann Oncol. 2016;27:1467-74.
204. Poynard T, McHutchison J, Manns M, et al. Impact of pegylated interferon alfa-2b and ribavirin on liver fibrosis in patients with chronic hepatitis C. Gastroenterology. 2002;122:1303-13.
205. Hu Z, Muroyama R, Kowatari N, Chang J, Omata M, Kato N. Characteristic mutations in hepatitis C virus core gene related to the occurrence of hepatocellular carcinoma. Cancer Sci. 2009;100:2465-8.
206. Lavery JA, Boutros PC, Knight D, Tammela T, Moskowitz CS, Jones LW. Association of exercise with pan-cancer incidence and overall survival. Cancer Cell. 2024;42:169-71.
207. Zhang Y, Cao C, Li C, et al. Physical exercise in liver diseases. Hepatology. 2024;Online ahead of print.
208. Chen X, Wu T, Xian L, et al. circGLS2 inhibits hepatocellular carcinoma recurrence via regulating hsa-miR-222-3p-PTEN-AKT signaling. Signal Transduct Target Ther. 2023;8:67.
209. Liu D, Liu W, Chen X, et al. circKCNN2 suppresses the recurrence of hepatocellular carcinoma at least partially via regulating miR-520c-3p/methyl-DNA-binding domain protein 2 axis. Clin Transl Med. 2022;12:e662.
210. Cao G, Kuriyama S, Du P, et al. Complete regression of established murine hepatocellular carcinoma by in vivo tumor necrosis factor alpha gene transfer. Gastroenterology. 1997;112:501-10.
211. Cao G, Kuriyama S, Gao J, et al. Gene therapy for hepatocellular carcinoma based on tumour-selective suicide gene expression using the alpha-fetoprotein (AFP) enhancer and a housekeeping gene promoter. Eur J Cancer. 2001;37:140-7.
212. Yin J, Li N, Han Y, et al. Effect of antiviral treatment with nucleotide/nucleoside analogs on postoperative prognosis of hepatitis B virus-related hepatocellular carcinoma: a two-stage longitudinal clinical study. J Clin Oncol. 2013;31:3647-55.
213. Gheshlaghi A, Haghshenas MR, Safarpour AR, et al. IL-17 genetic variations increase the risk of cirrhotic/hepatocellular carcinoma in patients with hepatitis B virus infection. Iran J Immunol. 2021;18:130-40.
214. Yu L, Cheng Y ju, Cheng M liang, et al. Quantitative assessment of common genetic variations in HLA-DP with hepatitis B virus infection, clearance and hepatocellular carcinoma development. Sci Rep. 2015;5:14933.
215. Ma N, Zhang X, Yu F, et al. Role of IFN-ks, IFN-ks related genes and the DEPDC5 gene in hepatitis B virus-related liver disease. J Viral Hepat. 2014;21:e29-38.
216. Mangoud NOM, Ali SA, El Kassas M, Soror SH. Chitinase 3-like-1, Tolloid-like protein 1, and intergenic gene polymorphisms are predictors for hepatocellular carcinoma development after hepatitis C virus eradication by direct-acting antivirals. IUBMB Life. 2021;73:474-82.
How to Cite
Download Citation
Export Citation File:
Type of Import
Tips on Downloading Citation
Citation Manager File Format
Type of Import
Direct Import: When the Direct Import option is selected (the default state), a dialogue box will give you the option to Save or Open the downloaded citation data. Choosing Open will either launch your citation manager or give you a choice of applications with which to use the metadata. The Save option saves the file locally for later use.
Indirect Import: When the Indirect Import option is selected, the metadata is displayed and may be copied and pasted as needed.
About This Article
Copyright
Data & Comments
Data
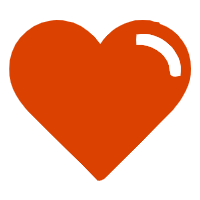
Comments
Comments must be written in English. Spam, offensive content, impersonation, and private information will not be permitted. If any comment is reported and identified as inappropriate content by OAE staff, the comment will be removed without notice. If you have any queries or need any help, please contact us at [email protected].