The role of gut microbiome and fecal microbiota transplantation in liver cancer and related complications: mechanisms and therapeutic potentials
Abstract
Liver cancer is the sixth commonest cancer and the third leading cause of cancer mortality worldwide. Accumulating evidence suggests a pivotal role of the gut microbiome in the progression of chronic liver disease and the subsequent development of liver cancer. Additionally, gut microbiome has been shown to contribute to the hosts’ antitumor responses following immunotherapy and chemotherapy for liver cancers, highlighting the therapeutic potential of gut microbiome modulation in enhancing treatment efficacy and reducing drug resistance. Fecal microbiota transplantation (FMT), a novel therapeutic modality to deliver a healthy donor's stool by endoscopy or capsule, has demonstrated potential in managing liver diseases and cancers by restoring and modulating the recipient’s gut microbiome composition. However, existing data on the clinical application of FMT in liver cancers are still limited. This review summarizes the underlying roles and mechanisms of gut microbiome in liver cancer and discusses the therapeutic potential of FMT in liver cancer treatment and the management of its related complications (e.g., hepatic encephalopathy).
Keywords
INTRODUCTION
Primary liver cancer is the sixth commonest cancer and the third leading cause of cancer mortality worldwide in 2020, with approximately 906,000 new cases and 830,000 deaths respectively[1]. Primary liver cancer includes 75%-85% of hepatocellular carcinoma (HCC), 10%-15% of intrahepatic cholangiocarcinoma(CCA), and some other rarer histological subtypes[1]. Hepatitis B, hepatitis C, heavy alcohol consumption, non-alcoholic fatty liver disease (NAFLD), type 2 diabetes, and smoking are major risk factors for HCC[2]. Although the molecular pathways leading to carcinogenesis in HCC are complex, recent evidence suggests that the gut microbiome plays a significant role in the progression of chronic liver disease and the subsequent development of HCC[3,4]. In addition, emerging evidence indicates that the gut microbiome may play a role in the host responding to antitumor treatment during immunotherapy and chemotherapy. This highlights the therapeutic potential of gut microbiome modulation in enhancing treatment efficacy and reducing drug resistance for liver cancer[3,4].
FMT is a novel treatment modality to modulate and restore the recipient’s gut microbiome composition by transplanting the functional gut microbiota from healthy donors to patients[5]. FMT solution can be delivered into the recipient’s gastrointestinal tract through various routes such as oral capsules, esophagogastroduodenoscopy, nasojejunal tube, rectal enema, and colonoscopy[6,7]. FMT has shown high efficacy in patients with recurrent and refractory Clostridioides difficile infection (CDI), which has now been recommended as a viable treatment option according to the United States, British, and European guidelines[8-11]. FMT has also demonstrated therapeutic potential in liver diseases, including alcoholic hepatitis[12], NAFLD[13], hepatitis B[14], and liver cirrhosis[15,16].
This review aims to summarize the current literature by exploring the mechanism of the gut microbiome in liver cancer and related complications and to discuss the recent advances in the role of FMT in antitumor therapies and responses.
DYSBIOSIS IN LIVER CANCERS
HCC is usually the sequelae of chronic liver disease (CLD). The majority of HCCs (80%-90%) are found in patients with advanced fibrosis or cirrhosis[17,18]. The development of HCC in patients with viral hepatitis is associated with persistent liver inflammation, but the precise mechanism in NAFLD cases remains unclear[19]. There is increasing evidence from both animal and human studies that suggests a connection between gut microbiota and the development of HCC. Changes in gut microbiome composition and microbiome-derived metabolites are thought to be responsible for promoting the progression of CLD and HCC occurrence[19,20].
It is widely acknowledged that dysbiosis occurs in HCC[21]. The α-diversity, which refers to the number of species present in each stool sample, has been found to be notably reduced in patients with HCC[22-24]. In addition, tissue samples from HCC also showed reduced α-diversity compared to patients with benign liver disease (e.g., hemangioma)[25]. At the phylum level, compared with the fecal microbiota of healthy individuals, the abundance of Firmicutes species was decreased. In contrast, that of Proteobacteria species was increased in both CLD and HCC patients[26]. In addition, the intrahepatic analysis indicated that HCC patients had a higher abundance of specific microbes in the Stenotrophomonas genus and the Proteobacteria phylum. This specific bacterium was very rare among the normal control group[25]. The dysbiosis in HCC also displayed a significant decrease in the relative abundance of short-chain fatty acids (SCFAs)-producing bacteria, such as Ruminococcaceae, Butyricicoccus, and Lachnospiraceae[27,28].
The etiologies of HCC can be divided into viral-related and non-viral-related, with different degrees of dysbiosis. Liu et al. compared the microbiota profile of HBV-related HCC (HBV-HCC) and non-HBV non-HCV related HCC (NBNC-HCC) and found that the HBV-HCC patients had a much higher level of species richness in fecal microbiota than NBNC-HCC patients[29]. An increase in the levels of proinflammatory bacteria (Escherichia-Shigella, Enterococcus) and a decrease in the levels of anti-inflammatory bacteria
Microbial dysbiosis has also been reported in different samples of patients with CCA[23,30-33]. An increased abundance of Prevotella species was identified in the oral, gut, and bile samples in patients with CCA[23]. In addition, the abundance of Actinomyces has been found to increase in the gut and bile[30] but decreased in oral samples in patients with CCA[32]. Actinomyces is associated with the disruption of mucosal membranes[34]. Zhang et al. found that the impaired gut barrier function could make gut-derived bacteria and lipopolysaccharide into hepatocytes, increasing polymorphonuclear myeloid-derived suppressor cells (PMN-MDSCs) to create an immunosuppressive environment, which will promote liver carcinogenesis[35]. The increased Actinomyces in the gut might be associated with the impaired gut barrier function, leading to the increase of Actinomyces in bile and the association with CCA. Therefore, it is now believed that dysbiosis has a pivotal role in the pathogenesis of both HCC and CCA.
GUT MICROBIOME-RELATED PATHOGENESIS OF LIVER CANCERS AND COMPLICATIONS
The hepatic portal circulation is a process that transports nutrients from the intestines to the liver. During this process, the liver is exposed to metabolites and products derived from gut microbiota. This crosstalk between the gut and liver is termed as “gut-liver-axis”[3]. As alcoholic liver disease (ALD), NAFLD, liver cirrhosis, and associated complications are closely linked to increased bacterial translocation and dysbiosis[36,37], it is thought to be one of the key drivers that lead to hepatic microenvironment inflammation and progression toward cirrhosis and HCC[3,4].
Experiments in animal models have provided pre-clinical evidence that both microbiota-derived metabolites and their activated pathways may play a role in the development of HCC. Fox et al. investigated the role of a specific bacteria, Helicobacter hepaticus, in promoting tumorigenesis by chemicals or viruses in a transgenic mice model[38]. Their results showed that colonization with H. hepaticus in the gut could promote aflatoxin- and HCV transgene-induced HCC without hepatic bacterial translocation or induction of hepatitis[38]. In addition, the study from Dapito et al. using an HCC mice model induced by diethylnitrosamine (DEN) and hepatotoxin carbon tetrachloride (CCl4) further demonstrated that gut microbiota and Toll-like receptor 4 (TLR4) activation were essential in the hepatocarcinogenesis in chronically injured livers[39]. It was demonstrated by Zhang et al. that long-term DEN treatment led to a notable reduction in the level of Lactobacillus, Bifidobacterium, and Enterococcus species and inflammation in the gut[20]. The presence of penicillin or dextran sulfate sodium (DSS)-induced intestinal dysbiosis or inflammation significantly increases the risk of tumor formation[20]. Supplementation with probiotics significantly alleviated gut dysbiosis, improved intestinal inflammation, and suppressed liver tumor growth and multiplicity[20]. Another experiment conducted by Yoshimoto et al. investigated obesity-related HCC by using a 7,12-dimethylbenz[a]anthracene (DMBA)-high-fat diet (HFD)-induced HCC mice model[40]. They found that obesity could lead to a rise in deoxycholic acid (DCA) levels. Elevated levels of DCA may trigger the senescence-associated secretory phenotype (SASP) in hepatic stellate cells, in turn causing the release of inflammatory and tumor-promoting molecules in the liver, promoting the progression of HCC in mice[40]. Similarly, Zhang et al. used a high-fat/high-cholesterol (HFHC) diet-induced NAFLD-HCC model[41]. They were able to demonstrate that cholesterol-induced NAFLD-HCC formation was associated with gut microbiome dysbiosis. They also used germ-free mice receiving FMT products from donor mice fed with HFHC, which led to hepatic lipid accumulation, inflammation, and cell proliferation[41].
Epidemiological data showed that the incidence rates of HCC in males were two or three times higher than that in females[42], and it is well-acknowledged that sex hormones play a significant role in the gender disparity of HCC[43,44]. It is commonly considered that estrogens are protective, while androgens promote hepatocellular carcinogenesis[45]. Sex-specific enterotypes may influence the metabolism of hormones, subsequently affecting the development of HCC[46,47]. In addition, gut microbiota might be another source of sex hormones, as Clostridium scindens has been reported to convert glucocorticoids into androgens[48]. Mouse experiments showed that the bacteria involved in bile acid metabolism (e.g., Clostridiales, Corynebacterium, Bacillus, Desulfovibrio, Rhodococcus) were different between males and females, and the differences became more significant after induction of HCC model (streptozotocin-high fat diet)[49]. This study revealed that gut microbiota was involved in the sex-dependent effects on bile acid metabolism, which plays a pivotal role in the development of non-alcoholic steatohepatitis (NASH) and NASH-associated HCC[50,51]. On the other hand, Huang et al. studied the change of gut microbiota in male and female HCC mice models (i.e., liver-specific Tsc1 knockout mice)[52]. They found that the female mice had dysbiosis earlier than male mice in the process of developing HCC[52]. Specific bacteria associated with the risk of HCC in males (e.g., Paraprevotella, Paraprevotellaceae) and females (e.g., Allobaculum, Erysipelotrichaceae) were observed[52]. These studies based on animal models revealed the sex-dependent involvement of gut microbiota in the pathogenesis of HCC. More well-designed studies involving human or human-to-mice models to investigate the role of gut microbiota in the sexual dimorphism of HCC are needed.
The majority of HCC patients suffer from concurrent liver cirrhosis, which is not only the risk factor for liver cancers but also related complications (e.g., hepatic encephalopathy, HE)[53,54]. Concurrent HE can interfere with the antitumor therapy and thus negatively affect the prognosis of liver cancer. Multiple studies have demonstrated that the beneficial SCFAs-producing bacteria (i.e., Lachnospiraceae and Ruminococcaceae) were decreased, and the potentially pathogenic (i.e., Enterobacteriaceae) were increased in patients with HE[36,55-57]. This microbial profile has also been associated with cognitive impairment and the presence of systemic inflammation[57,58]. These emerging data support that the brain and the "gut-liver axis" are closely intertwined, and possibly these clinical manifestations are reflective of the "gut-liver-brain axis”[59]. The increasing understanding of the influence of gut microbiota on the development of liver cancers provides valuable insight into the use of microbiome modulation as a potential therapy for managing liver cancer and its associated complications.
THERAPEUTIC POTENTIAL OF MICROBIOME MODULATION IN ONCOLOGICAL TREATMENT FOR LIVER CANCER
Treatment options for liver cancer include surgical resection, chemotherapy, radiotherapy, radiofrequency ablation, hepatic artery chemoembolization, immunotherapy, and liver transplantation. In the case of advanced cancers, systemic therapy and multikinase inhibitors such as sorafenib and lenvatinib are the mainstay of treatment[60,61]. However, fewer than one-third of patients can benefit from these treatments as drug resistance and treatment-related adverse events are major roadblocks[62]. On the other hand, since 2017, the US Federal Drug Administration has approved immune checkpoint inhibitors (ICIs) as a second-line treatment for advanced HCC with sorafenib resistance. The combination of atezolizumab and bevacizumab has proven superior to sorafenib[63]. An infusion regimen termed STRIDE (Single Tremelimumab Regular Interval Durvalumab) significantly improved overall survival versus sorafenib (36 months overall survival rate 30.7% vs. 20.2%)[64]. However, the overall success rate of ICI monotherapy or combination therapy has been reported to be only around 36%[65,66]. In addition, some patients develop drug resistance after treatment. Therefore, it would be beneficial if there is an adjunctive therapy to enhance the treatment efficacy and reduce the risk of drug resistance for chemotherapy and immunotherapy.
Chemotherapy and targeted agents
The gut microbiota modulates the host response to chemotherapeutic drugs in two major domains: drug efficacy and toxicity [Table 1][67]. Jin et al. found that gut microbiota can enhance the chemosensitivity of HCC to 5-fluorouracil in vivo by increasing curcumin bioavailability[68]. On the other hand, gut microbiota alteration may also affect drug toxicity and treatment-related adverse events (AEs). Inukai et al. compared the gut microbiota in patients who developed diarrhea and those who did not after receiving Lenvatinib treatment for HCC[69]. The group with diarrhea showed a higher relative abundance of Parabacteroides and Prevotella[69]. Similarly, Yamamoto et al. also found an increased relative abundance of Butyricimonas, a butyric acid-producing bacteria, in the asymptomatic group than in the group with diarrhea[70]. Butyrate has a vital role in suppressing inflammatory and allergic responses[70]. Based on the above preliminary findings, chemotherapy combined with adjunctive microbiota modulation may be a promising therapeutic approach.
Gut microbiome alteration in antitumor therapies for liver cancer
Reference | Models | Therapies | Diseases | Implicated microbiota | Sequencing method |
Inukai et al. | Human | Chemotherapy | HCC (diarrhea and non-diarrhea) | ↑ Parabacteroides and Prevotella in the diarrhea group | 16S rRNA gene sequencing |
Yamamoto et al.[70] | Human | Chemotherapy | HCC (diarrhea and non-diarrhea) | ↑ Butyricimonas, ↓ Citrobacter, Peptostreptococcus, and Staphylococcaceae in non-diarrhea group | 16S rRNA gene sequencing |
Jin et al.[68] | Mice | Chemotherapy (curcumin combined with 5-fluorouracil) | HCC | ↑ Richness (Chao 1 index); curcumin treatment significantly ↑ Bifidobacterium and Lactobacillus in gut | 16S rDNA sequencing |
Wu et al.[75] | Human | Immunotherapy (anti-PD-1) | HCC (R and NR) | ↑ α-diversity (Shannon and inverse Simpson indexes) in R; ↑ Faecalibacterium, Blautia, Lachnospiracea incertae Sedis, Megamonas, Ruminococcus, Coprococcus, Dorea and Haemophilus in R; ↑ Atopobium, Leptotrichia, Campylobacter, Allisonella, Methanobrevibacter, Parabacteroides, Bifidobacterium, and Lactobacillus in NR | 16S rRNA gene sequencing |
Lee et al.[78] | Human | Immunotherapy (ICIs) | HCC (R and NR) | ↑ Prevotella 9 in NR, ↑ Lachnoclostridium, Lachnospiraceae, and Veillonella in R; ↑Lachnoclostridium and ↓ Prevotella 9 were associated with better overall survival | 16S rRNA gene sequencing |
Chung et al. | Human | Immunotherapy (nivolumab) | HCC (R and NR) | NR: a skewed Firmicutes/Bacteroidetes ratio and a ↓ Prevotella/Bacteroides ratio; presence of Akkermansia species was associated with good response | 16S rRNA gene sequencing |
Shen et al.[108] | Human | Immunotherapy (ICIs) | HCC (R and NR) | ↑Bifidobacterium, Coprococcus, and Acidaminococcus- in patients with disease control | 16S rRNA gene sequencing |
Zheng et al. | Human | Immunotherapy (anti-PD-1 antibodies) | HCC | ↑ Four Lactobacillus species (L. oris, L. mucosae, L. gasseri, and L. vaginalis), Bifidobacterium dentium and Streptococcus thermophilus in R | Metagenomic sequencing |
Mao et al.[109] | Human | Immunotherapy (anti-PD-1) | HCC or advanced biliary tract cancers | ↑ Ruminococcus calidus and Erysipelotichaceae bacterium-GAM147 in response. ↑ Veillonellaceae in NR | Metagenomic sequencing |
Li et al.[84] | Human and mice | Radiotherapy | HCC (R and NR) | ↓ Diversity in NR; the distribution of the R group samples was closer to healthy control group samples; At the genus level, ↑ Faecalibacterium was observed in the healthy control and R group; ↑ order Clostridiales, family Ruminococcaceae, and genus Faecalibacterium in the R group; ↑ order Lactobacillales in the NR group | 16S rRNA sequencing |
Bian et al.[86] | Rabbit | Transarterial chemoembolization | HCC | ↑Ruminococcus and Roseburia; ↓ Bacteroides; Parabacteroides and Escherichia after surgery | qPCR |
Immunotherapy
Immunotherapy is a promising oncological treatment by modulating the interaction between the host's immune system and cancer cells. Emerging evidence demonstrates the close crosstalk between the gut microbiota and patients’ response to ICIs [Table 1]. For example, the role of gut microbiota in modulating treatment response among patients on ICI targeting programmed cell death protein 1 (anti-PD-1) has garnered increasing attention in recent years[71,72]. Zheng et al. collected stool samples in HCC patients who received immunotherapy and revealed that responders had higher taxa richness and more gene counts than non-responders[73]. Responders were enriched with some beneficial species, such as Akkermansia muciniphila and Ruminococcaceae spp[73]. Similarly, Chung et al. proposed that a skewed Firmicutes/Bacteroidetes ratio and a low Prevotella/Bacteroides ratio could be predictive markers of non-responders among HCC patients receiving ICIs[74]. In contrast, the presence of Akkermansia species predicted a good response[74]. Wu et al. also found that responders had a higher level of α-diversity at baseline than non-responders[75]. They also profiled the serum metabolites and found that responders were enriched with Ruminococcus which was positively correlated with serum galactaric acid[75]. Furthermore, Routy et al. performed FMT on germ-free or antibiotic-treated mice using fecal samples from epithelial cancer patients who responded to ICIs and found that it could show the antitumor effects of PD-1 blockade, while FMT from non-responders could not restitute the same effect[76]. In a subgroup of patients with primary PD-1-refractory melanoma, FMT using a donor from the anti-PD1 responder could overcome drug resistance and re-capture anti-PD1 treatment response among 40% (6/15) of them by re-programming the gut microbiome and tumor microenvironment[77]. Metagenomics analysis revealed correlations between the relative abundance of A. muciniphila and clinical responses to ICIs[76]. Oral administration of A. muciniphila after FMT in non-responders has been shown to restore the efficacy of PD-1 blockade[76]. In addition, microbiota-derived metabolites also showed an association with clinical outcomes in patients with HCC receiving ICIs[78]. Responders had a significantly higher level of secondary bile acids[78]. If these findings can be replicated, modulating gut microbiota before immunotherapy by FMT from selected donors enriched in certain favorable species may potentially increase the clinical response rate of immunotherapy for liver cancer. In addition, subgroup analyses of survival outcomes according to clinical trials which evaluated the efficacy of ICIs revealed a discrepancy between viral HCC and non-viral HCC[79-82], implying a potential influence of tumor etiologies on treatment response[83]. Considering the variation in dysbiosis[29], more studies are needed to support the gut microbiome modulation before immunotherapy based on the etiologies of HCC.
Locoregional therapies
The role of gut microbiota in other locoregional treatments for liver cancer has also attracted considerable interest recently [Table 1]. Li et al. analyzed the fecal microbiota from HCC patients who received radiotherapy and found that the distribution of microbiota from responders was closer to that found in healthy controls[84]. Responders were enriched with specific bacteria at the genus level, such as Faecalibacteriu, while non-responders had a high abundance of members of the genus Streptococcus[84]. Further animal experiments demonstrated that gut dysbiosis induced by antibiotics significantly impaired radiotherapy-induced T-cell infiltration and that FMT could restore the antitumor effects of radiotherapy[84].
Another treatment option for intermediate and advanced liver cancer is transarterial chemoembolization (TACE), using localized high concentrations of chemotherapy agents and embolizing feeding vessels by oil iodide emulsion[85]. Bian et al. conducted TACE in rabbit HCC models and found that the procedure partially reversed the tumor-induced dysbiosis, improving the intestinal barrier and liver functions and decreasing the lipopolysaccharide (LPS) level in the blood[86]. Some key operational taxonomic units (OTUs) were closely associated with clinical factors, which provides a theoretical basis for the therapy combination of TACE with microbiota-targeted intervention in the future[86].
FMT IN LIVER CANCERS AND RELATED COMPLICATIONS
Endoscopic procedures of FMT
FMT can be delivered into patients’ gastrointestinal tract via several routes, such as esophagogastroduodenoscopy (EGD), colonoscopy, feeding tubes, or rectal enemas[87]. Fresh or frozen fecal suspensions can be delivered into the duodenum or colon through the working channel of the flexible endoscope. Caution needs to be exercised when FMT is given by EGD in patients with a history of stomach or duodenal surgery to avoid adverse events such as aspiration[88]. FMT through colonoscopy allows for the concomitant diagnosis of colonic diseases and may be a preferable route in some situations[89,90].
Current evidence of FMT in liver cancers and related complications
To date, published data on FMT in liver cancer treatment is unavailable. Yet, it is worth noting that there is a recently registered clinical trial to investigate the effect of FMT in overcoming drug resistance to atezolizumab/bevacizumab among patients with liver cancers, which has been registered in ClinicalTrials.gov (NCT05690048) [Table 2]. Apart from atezolizumab and bevacizumab, the intervention group will receive three days of oral vancomycin followed by two rounds of FMT capsules, compared with placebo capsules in the control group. We eagerly await the results of this trial.
Application of FMT in liver cancer and related complications
Reference | Model /study type | Disease | FMT details | Clinical outcome | Implicated microbiota |
Clinicaltrial.gov: NCT05690048 | Human/phase II, single blind, RCT, | Liver cancer (unresectable HCC, BCLC stage C) | FMT via capsule (50 g of fecal matter) on day 0 and day 21 | The primary outcomes are differential tumoral CD8 T-cell infiltration and adverse event documentation of FMT | NA |
Clinicaltrial.gov: NCT05170971 | Human/open label | Liver failure | Four times of FMT every 5 days, follow-up for 2 months | The primary outcomes are the change in liver function, coagulation function, MELD score, clinical manifestations, safety and adverse events and change of proinflammatory cytokine | The change in gut microbiota will be checked |
Bloom et al.[91] | Human/open label (10 subjects) | HE | FMT capsules 5 times over 3 weeks, follow-up for 6 months | The PHES improved after three doses and five doses of FMT, and four weeks after the fifth dose of FMT. One SAE was extended‐spectrum beta‐lactamase Escherichia coli bacteremia | Responders had higher levels of Bifidobacterium and other beneficial species at baseline and throughout the study |
Bajaj et al.[16] | Human/phase 1, RCT (20 subjects) | HE | 15 FMT capsules (universal donor with high Lachnospiraceae and Ruminococcaceae relative abundance), follow up for 5 months | All subjects tolerated the procedures (EGD, sigmoidoscopy and capsule); ↓ SAEs and number of patients with SAEs in the FMT group; ↑ improvement in brain function in the FMT group compared to baseline | In the duodenum, ↑ Ruminococcaceae and Bifidobacteriaceae and ↓ Veillonellaceae and Streptococcaceae post-FMT compared to pre-FMT |
Bajaj et al.[15] | Human/RCT (20 subjects) | HE | Three frozen FMT units (90 mL total) were administered by enema and retained for 30 min, follow-up for five months | ↓SAE in FMT group compared to SOC group (8 vs. 2); Five SOC group and no FMT participants developed further HE. Cognition improved in FMT, but not SOC group | ↑ Lactobacillaceae, Bifidobacteriaceae post-FMT; patients in the FMT arm showed ↑ Lachnospiraceaeae and Ruminococcaceae after FMT |
Sharma et al.[110] | Human/open label (13 subjects) | Alcohol-associated acute-on-chronic liver failure | FMT through nasojejunal tube from selected family members, follow up 3 months | Survival at 28 and 90 days was significantly better in the FMT arm (100% vs. 60%; 53.84% vs. 25%). More HE and ascites resolved in the FMT group compared to the SOC group (100% vs. 57.14%; 100% vs. 40%). Adverse events were similar in both groups | NA |
Huang et al.[111] | Rats | portal hypertension and portosystemic collaterals | FMT by oral gavage | FMT ↓ portal pressure in cirrhotic rats | ↓Lachnospiraceae in cirrhotic rats. FMT ↑ Bifidobacterium |
On the other hand, more published data are available to demonstrate the efficacy and safety of FMT in liver cancer-related complications [Table 2]. FMT is now a potential therapeutic option for HE. Bajaj et al. conducted a randomized controlled trial (RCT) investigating the safety and efficacy of FMT from donors with high levels of Lachnospiraceae and Ruminococcaceae[15]. The results showed that patients receiving a single FMT enema developed less severe adverse events (SAE) (20% vs. 80%) and less recurrent HE (0% vs. 50%) compared with the control group (who were given the standard of care)[15]. Two SAEs (i.e., hospitalization) in the FMT group were considered unrelated to the FMT by the independent Data Safety Monitoring Board[15]. Furthermore, FMT group showed improved cognition, but the control group did not[15]. Microbiome analysis indicated that, after FMT, a significant increase was found in the relative abundance of Lachnospiraceaeae and Ruminococcaceaen[15]. Based on these findings, Bajaj et al. conducted another RCT treating patients with HE by FMT capsules from a single donor who had enriched Lachnospiraceaeae and Ruminococcaceae, demonstrating that oral FMT capsules were safe and well-tolerated even in patients with cirrhosis and recurrent HE[16]. This trial also confirmed the efficacy of FMT in improving duodenal mucosal diversity, dysbiosis, and resolution of HE. Similarly, a recent study investigated the efficacy and safety of FMT capsules from different donors in HE[91]. They found that the levels of Bifidobacterium and other known beneficial bacteria at baseline and throughout the study were higher in responders[91]. Moreover, the fecal SCFAs levels from donors were the lowest among recipients with worse cognitive outcomes[91]. These data suggested that the efficacy of FMT can be affected by recipient and donor effects. More data exploring the crosstalk between the microbiome of donor and recipient are needed before more definitive conclusions can be drawn.
LIMITATIONS AND FUTURE PERSPECTIVE
Although the safety of FMT has been demonstrated in patients with CDI[90,92,93], inflammatory bowel diseases (IBD)[94-98], other types of cancers[77,99,100], graft versus host disease (GVHD)[101,102], and critically ill patients hospitalized in the intensive care unit (ICU)[103], safety issues relating to FMT in patients with liver cirrhosis and liver cancer should be taken into consideration. One SAE of extended-spectrum beta-lactamase (ESBL) Escherichia coli bacteremia has been reported previously[91]. Portal hypertension, which results in increased intestinal permeability and impaired barrier function, may induce additional risks to bacterial translocation, leading to an excessive risk of bacteremia. Recently, a new methodology based on an automatic purification system of FMT, known as washed microbiota transplantation (WMT), showed lower AE rates than traditional manual methods[97,104], WMT was regarded as a safer and cleaner type of FMT[105,106], which could be an alternative option in high-risk patients with liver cancers and related complications. In addition to the safety issues, cost-effectiveness is another critical area worth further study. A multidisciplinary team, including endoscopists, hepatologists, oncologists, microbiologists, pharmacists, and other related healthcare professionals, can work together to develop an appropriate and pragmatic strategy to help these patients. The primary diagnosis, cancer stage, complications, microbiota profiles, and oncological therapies should be considered on an individualized basis to determine the optimal delivery route, dose, and frequency of FMT. Selective microbiota transplantation (SMT) by matching selected donors could be considered to reduce the potential infective risks further. Under the premise of ensuring safety, FMT and other microbiome modulation modalities can be considered adjunctive therapy for liver cancer and related complications[107].
CONCLUSION
In conclusion, the role of gut microbiota modulation in patients with liver cancer and related complications is biologically plausible and supported by a growing body of literature. More studies on efficacy and safety are needed if FMT is to be incorporated into mainstream clinical applications.
DECLARATIONS
Authors’ contributionsDid literature review, drafted and revised the manuscript: Dai M
Revised the manuscript: Lau LHS, Lui RN
Availability of data and materialsNot applicable.
Financial support and sponsorshipNone.
Conflicts of interestLau LHS has received research grant support from GenieBiome Ltd. Lau LHS has served as a lecture speaker for Olympus, Boston Scientific, Pfizer, and GenieBiome Ltd. Lui NR has served as an advisory board member for Gilead Sciences and as a speaker for GenieBiome, Gilead Sciences, and Pierre Fabre, and owns equity in Pfizer.
Ethical approval and consent to participateNot applicable.
Consent for publicationNot applicable.
Copyright© The Author(s) 2023.
REFERENCES
1. Sung H, Ferlay J, Siegel RL, et al. Global cancer statistics 2020: GLOBOCAN estimates of incidence and mortality worldwide for 36 cancers in 185 countries. CA Cancer J Clin 2021;71:209-49.
2. McGlynn KA, Petrick JL, London WT. Global epidemiology of hepatocellular carcinoma: an emphasis on demographic and regional variability. Clin Liver Dis 2015;19:223-38.
3. Tripathi A, Debelius J, Brenner DA, et al. The gut-liver axis and the intersection with the microbiome. Nat Rev Gastroenterol Hepatol 2018;15:397-411.
4. Yu LX, Schwabe RF. The gut microbiome and liver cancer: mechanisms and clinical translation. Nat Rev Gastroenterol Hepatol 2017;14:527-39.
6. Peng Z, Xiang J, He Z, et al. Colonic transendoscopic enteral tubing: a novel way of transplanting fecal microbiota. Endosc Int Open 2016;4:E610-3.
7. Wang W, Lu G, Wu X, Wen Q, Zhang F. Colonic transendoscopic enteral tubing is a new pathway to microbial therapy, colonic drainage, and host-microbiota interaction research. J Clin Med 2023;12:780.
8. van Nood E, Vrieze A, Nieuwdorp M, et al. Duodenal infusion of donor feces for recurrent Clostridium difficile. N Engl J Med 2013;368:407-15.
9. Johnson S, Lavergne V, Skinner AM, et al. Clinical practice guideline by the infectious diseases society of America (IDSA) and society for healthcare epidemiology of America (SHEA): 2021 focused update guidelines on management of clostridioides difficile infection in adults. Clin Infect Dis 2021;73:755-7.
10. Mullish BH, Quraishi MN, Segal JP, et al. The use of faecal microbiota transplant as treatment for recurrent or refractory Clostridium difficile infection and other potential indications: joint British Society of Gastroenterology (BSG) and Healthcare Infection Society (HIS) guidelines. Gut 2018;67:1920-41.
11. Prehn J, Reigadas E, Vogelzang EH, et al; Guideline Committee of the European Study Group on Clostridioides difficile. European Society of Clinical Microbiology and Infectious Diseases: 2021 update on the treatment guidance document for Clostridioides difficile infection in adults. Clin Microbiol Infect 2021;27 Suppl 2:S1-S21.
12. Xue L, Deng Z, Luo W, He X, Chen Y. Effect of fecal microbiota transplantation on non-alcoholic fatty liver disease: a randomized clinical trial. Front Cell Infect Microbiol 2022;12:759306.
13. Craven L, Rahman A, Nair Parvathy S, et al. Allogenic fecal microbiota transplantation in patients with nonalcoholic fatty liver disease improves abnormal small intestinal permeability: a randomized control trial. Am J Gastroenterol 2020;115:1055-65.
14. Chauhan A, Kumar R, Sharma S, et al. Fecal microbiota transplantation in hepatitis B e antigen-positive chronic hepatitis b patients: a pilot study. Dig Dis Sci 2021;66:873-80.
15. Bajaj JS, Kassam Z, Fagan A, et al. Fecal microbiota transplant from a rational stool donor improves hepatic encephalopathy: a randomized clinical trial. Hepatology 2017;66:1354-5.
16. Bajaj JS, Salzman NH, Acharya C, et al. Fecal microbial transplant capsules are safe in hepatic encephalopathy: a phase 1, randomized, placebo-controlled trial. Hepatology 2019;70:1690-703.
17. Cabrera R, Nelson DR. Review article: the management of hepatocellular carcinoma. Aliment Pharmacol Ther 2010;31:461-76.
19. Schwabe RF, Greten TF. Gut microbiome in HCC-mechanisms, diagnosis and therapy. J Hepatol 2020;72:230-8.
20. Zhang HL, Yu LX, Yang W, et al. Profound impact of gut homeostasis on chemically-induced pro-tumorigenic inflammation and hepatocarcinogenesis in rats. J Hepatol 2012;57:803-12.
21. Das BK. Altered gut microbiota in hepatocellular carcinoma: Insights into the pathogenic mechanism and preclinical to clinical findings. APMIS 2022;130:719-40.
22. Ni J, Huang R, Zhou H, et al. Analysis of the relationship between the degree of dysbiosis in gut microbiota and prognosis at different stages of primary hepatocellular carcinoma. Front Microbiol 2019;10:1458.
23. Deng T, Li J, He B, et al. Gut microbiome alteration as a diagnostic tool and associated with inflammatory response marker in primary liver cancer. Hepatol Int 2022;16:99-111.
24. Lai MW, Chu YD, Hsu CW, Chen YC, Liang KH, Yeh CT. Multi-omics analyses identify signatures in patients with liver cirrhosis and hepatocellular carcinoma. Cancers 2022;15:210.
25. Liu B, Zhou Z, Jin Y, et al. Hepatic stellate cell activation and senescence induced by intrahepatic microbiota disturbances drive progression of liver cirrhosis toward hepatocellular carcinoma. J Immunother Cancer 2022;10:e003069.
26. Chen T, Ding R, Chen X, et al. Firmicutes and blautia in gut microbiota lessened in chronic liver diseases and hepatocellular carcinoma patients: a pilot study. Bioengineered 2021;12:8233-46.
27. Lapidot Y, Amir A, Nosenko R, et al. Alterations in the gut microbiome in the progression of cirrhosis to hepatocellular carcinoma. mSystems 2020;5:e00153-20.
28. Ren Z, Li A, Jiang J, et al. Gut microbiome analysis as a tool towards targeted non-invasive biomarkers for early hepatocellular carcinoma. Gut 2019;68:1014-23.
29. Liu Q, Li F, Zhuang Y, et al. Alteration in gut microbiota associated with hepatitis B and non-hepatitis virus related hepatocellular carcinoma. Gut Pathog 2019;11:1.
30. Jia X, Lu S, Zeng Z, et al. Characterization of gut microbiota, bile acid metabolism, and cytokines in intrahepatic cholangiocarcinoma. Hepatology 2020;71:893-906.
31. Saab M, Mestivier D, Sohrabi M, et al. Characterization of biliary microbiota dysbiosis in extrahepatic cholangiocarcinoma. PLoS One 2021;16:e0247798.
32. Rao BC, Zhang GZ, Zou YW, et al. Alterations in the human oral microbiome in cholangiocarcinoma. Mil Med Res 2022;9:62.
33. Chen B, Fu SW, Lu L, Zhao H. A preliminary study of biliary microbiota in patients with bile duct stones or distal cholangiocarcinoma. Biomed Res Int 2019;2019:1092563.
34. Pujic P, Beaman BL, Ravalison M, Boiron P, Rodríguez-nava V. Nocardia and actinomyces. Molecular medical microbiology. Elsevier; 2015. pp. 731-52.
35. Zhang Q, Ma C, Duan Y, et al. Gut microbiome directs hepatocytes to recruit MDSCs and promote cholangiocarcinoma. Cancer Discov 2021;11:1248-67.
36. Bajaj JS, Heuman DM, Hylemon PB, et al. Altered profile of human gut microbiome is associated with cirrhosis and its complications. J Hepatol 2014;60:940-7.
37. Shen F, Zheng RD, Sun XQ, Ding WJ, Wang XY, Fan JG. Gut microbiota dysbiosis in patients with non-alcoholic fatty liver disease. Hepatobiliary Pancreat Dis Int 2017;16:375-81.
38. Fox JG, Feng Y, Theve EJ, et al. Gut microbes define liver cancer risk in mice exposed to chemical and viral transgenic hepatocarcinogens. Gut 2010;59:88-97.
39. Dapito DH, Mencin A, Gwak GY, et al. Promotion of hepatocellular carcinoma by the intestinal microbiota and TLR4. Cancer Cell 2012;21:504-16.
40. Yoshimoto S, Loo TM, Atarashi K, et al. Obesity-induced gut microbial metabolite promotes liver cancer through senescence secretome. Nature 2013;499:97-101.
41. Zhang X, Coker OO, Chu ES, et al. Dietary cholesterol drives fatty liver-associated liver cancer by modulating gut microbiota and metabolites. Gut 2021;70:761-74.
42. Petrick JL, Florio AA, Znaor A, et al. International trends in hepatocellular carcinoma incidence, 1978-2012. Int J Cancer 2020;147:317-30.
43. Yeh SH, Chen PJ. Gender disparity of hepatocellular carcinoma: the roles of sex hormones. Oncology 2010;78 Suppl 1:172-9.
44. Nevola R, Tortorella G, Rosato V, et al. Gender differences in the pathogenesis and risk factors of hepatocellular carcinoma. Biology 2023;12:984.
45. Kalra M, Mayes J, Assefa S, Kaul AK, Kaul R. Role of sex steroid receptors in pathobiology of hepatocellular carcinoma. World J Gastroenterol 2008;14:5945-61.
46. Markle JG, Frank DN, Mortin-Toth S, et al. Sex differences in the gut microbiome drive hormone-dependent regulation of autoimmunity. Science 2013;339:1084-8.
47. Yurkovetskiy L, Burrows M, Khan AA, et al. Gender bias in autoimmunity is influenced by microbiota. Immunity 2013;39:400-12.
48. Ridlon JM, Ikegawa S, Alves JM, et al. Clostridium scindens: a human gut microbe with a high potential to convert glucocorticoids into androgens. J Lipid Res 2013;54:2437-49.
49. Xie G, Wang X, Zhao A, et al. Sex-dependent effects on gut microbiota regulate hepatic carcinogenic outcomes. Sci Rep 2017;7:45232.
50. Zhou J, Tripathi M, Sinha RA, Singh BK, Yen PM. Gut microbiota and their metabolites in the progression of non-alcoholic fatty liver disease. Hepatoma Res 2021;7:11.
51. Sousa P, Machado MV. MAFLD under the lens: the role of gut microbiota. Metab Target Organ Damage 2022;2:14.
52. Huang R, Li T, Ni J, et al. Different sex-based responses of gut microbiota during the development of hepatocellular carcinoma in liver-specific Tsc1-knockout mice. Front Microbiol 2018;9:1008.
53. Fattovich G, Stroffolini T, Zagni I, Donato F. Hepatocellular carcinoma in cirrhosis: incidence and risk factors. Gastroenterology 2004;127:S35-50.
54. Meriggi F, Graffeo M. Clinical characterisation and management of the main treatment-induced toxicities in patients with hepatocellular carcinoma and cirrhosis. Cancers 2021;13:584.
55. Chen Y, Yang F, Lu H, et al. Characterization of fecal microbial communities in patients with liver cirrhosis. Hepatology 2011;54:562-72.
56. Bajaj JS, Fagan A, White MB, et al. Specific gut and salivary microbiota patterns are linked with different cognitive testing strategies in minimal hepatic encephalopathy. Am J Gastroenterol 2019;114:1080-90.
57. Bajaj JS, Shamsaddini A, Fagan A, et al. Distinct gut microbial compositional and functional changes associated with impaired inhibitory control in patients with cirrhosis. Gut Microbes 2021;13:1953247.
58. Bajaj JS, Ridlon JM, Hylemon PB, et al. Linkage of gut microbiome with cognition in hepatic encephalopathy. Am J Physiol Gastrointest Liver Physiol 2012;302:G168-75.
59. Ho AHY, Wong S, Lui R. Topic: nutrition and the gut-liver-brain axis. Curr Hepatology Rep 2022;21:99-110.
60. Jackson R, Psarelli EE, Berhane S, Khan H, Johnson P. Impact of viral status on survival in patients receiving sorafenib for advanced hepatocellular cancer: a meta-analysis of randomized phase III trials. J Clin Oncol 2017;35:622-8.
61. Kudo M, Finn RS, Qin S, et al. Lenvatinib versus sorafenib in first-line treatment of patients with unresectable hepatocellular carcinoma: a randomised phase 3 non-inferiority trial. Lancet 2018;391:1163-73.
62. El-Serag HB, Marrero JA, Rudolph L, Reddy KR. Diagnosis and treatment of hepatocellular carcinoma. Gastroenterology 2008;134:1752-63.
63. Finn RS, Qin S, Ikeda M, et al. IMbrave150 Investigators. Atezolizumab plus bevacizumab in unresectable hepatocellular carcinoma. N Engl J Med 2020;382:1894-905.
64. Abou-alfa GK, Lau G, Kudo M, et al. Tremelimumab plus durvalumab in unresectable hepatocellular carcinoma. NEJM Evidence 2022;1:EVIDoa2100070.
65. Yau T, Kang YK, Kim TY, et al. Efficacy and safety of nivolumab plus ipilimumab in patients with advanced hepatocellular carcinoma previously treated with sorafenib: the checkmate 040 randomized clinical trial. JAMA Oncol 2020;6:e204564.
66. Finn RS, Ikeda M, Zhu AX, et al. Phase Ib study of lenvatinib plus pembrolizumab in patients with unresectable hepatocellular carcinoma. J Clin Oncol 2020;38:2960-70.
67. Alexander JL, Wilson ID, Teare J, Marchesi JR, Nicholson JK, Kinross JM. Gut microbiota modulation of chemotherapy efficacy and toxicity. Nat Rev Gastroenterol Hepatol 2017;14:356-65.
68. Jin M, Kong L, Han Y, Zhang S. Gut microbiota enhances the chemosensitivity of hepatocellular carcinoma to 5-fluorouracil
69. Inukai Y, Yamamoto K, Honda T, et al. Differences in the intestinal microbiome associated with diarrhea during lenvatinib treatment for hepatocellular carcinoma. Dig Dis 2023;41:138-47.
70. Yamamoto K, Kuzuya T, Honda T, et al. Relationship between adverse events and microbiomes in advanced hepatocellular carcinoma patients treated with sorafenib. Anticancer Res 2020;40:665-76.
71. El-Khoueiry AB, Sangro B, Yau T, et al. Nivolumab in patients with advanced hepatocellular carcinoma (CheckMate 040): an open-label, non-comparative, phase 1/2 dose escalation and expansion trial. Lancet 2017;389:2492-502.
72. Zhu AX, Finn RS, Edeline J, et al. KEYNOTE-224 investigators. Pembrolizumab in patients with advanced hepatocellular carcinoma previously treated with sorafenib (KEYNOTE-224): a non-randomised, open-label phase 2 trial. Lancet Oncol 2018;19:940-52.
73. Zheng Y, Wang T, Tu X, et al. Gut microbiome affects the response to anti-PD-1 immunotherapy in patients with hepatocellular carcinoma. J Immunother Cancer 2019;7:193.
74. Chung MW, Kim MJ, Won EJ, et al. Gut microbiome composition can predict the response to nivolumab in advanced hepatocellular carcinoma patients. World J Gastroenterol 2021;27:7340-9.
75. Wu H, Zheng X, Pan T, et al. Dynamic microbiome and metabolome analyses reveal the interaction between gut microbiota and anti-PD-1 based immunotherapy in hepatocellular carcinoma. Int J Cancer 2022;151:1321-34.
76. Routy B, Le Chatelier E, Derosa L, et al. Gut microbiome influences efficacy of PD-1-based immunotherapy against epithelial tumors. Science 2018;359:91-7.
77. Davar D, Dzutsev AK, McCulloch JA, et al. Fecal microbiota transplant overcomes resistance to anti-PD-1 therapy in melanoma patients. Science 2021;371:595-602.
78. Lee PC, Wu CJ, Hung YW, et al. Gut microbiota and metabolites associate with outcomes of immune checkpoint inhibitor-treated unresectable hepatocellular carcinoma. J Immunother Cancer 2022;10:e004779.
79. Finn RS, Ryoo BY, Merle P, et al. KEYNOTE-240 investigators. Pembrolizumab as second-line therapy in patients with advanced hepatocellular carcinoma in KEYNOTE-240: a randomized, double-blind, phase III trial. J Clin Oncol 2020;38:193-202.
80. Kelley RK, Sangro B, Harris W, et al. Safety, efficacy, and pharmacodynamics of tremelimumab plus durvalumab for patients with unresectable hepatocellular carcinoma: randomized expansion of a phase I/II study. J Clin Oncol 2021;39:2991-3001.
81. Yau T, Park J, Finn R, et al. CheckMate 459: a randomized, multi-center phase III study of nivolumab (NIVO)
82. Abou-alfa GK, Chan SL, Kudo M, et al. Phase 3 randomized, open-label, multicenter study of tremelimumab (T) and durvalumab (D) as first-line therapy in patients (pts) with unresectable hepatocellular carcinoma (uHCC): HIMALAYA. J Clin Oncol 2022;40:379.
83. Costante F, Airola C, Santopaolo F, Gasbarrini A, Pompili M, Ponziani FR. Immunotherapy for nonalcoholic fatty liver disease-related hepatocellular carcinoma: lights and shadows. World J Gastrointest Oncol 2022;14:1622-36.
84. Li Z, Zhang Y, Hong W, et al. Gut microbiota modulate radiotherapy-associated antitumor immune responses against hepatocellular carcinoma via STING signaling. Gut Microbes 2022;14:2119055.
85. McIlwain DR, Lang PA, Maretzky T, et al. iRhom2 regulation of TACE controls TNF-mediated protection against Listeria and responses to LPS. Science 2012;335:229-32.
86. Bian CF, Wang Y, Yu A, et al. Gut microbiota changes and biological mechanism in hepatocellular carcinoma after transarterial chemoembolization treatment. Front Oncol 2022;12:1002589.
87. Gulati M, Singh SK, Corrie L, Kaur IP, Chandwani L. Delivery routes for faecal microbiota transplants: available, anticipated and aspired. Pharmacol Res 2020;159:104954.
88. Baxter M, Ahmad T, Colville A, Sheridan R. Fatal aspiration pneumonia as a complication of fecal microbiota transplant. Clin Infect Dis 2015;61:136-7.
89. Kelly CR, Khoruts A, Staley C, et al. Effect of fecal microbiota transplantation on recurrence in multiply recurrent clostridium difficile infection: a randomized trial. Ann Intern Med 2016;165:609-16.
90. Kao D, Roach B, Silva M, et al. Effect of oral capsule-
91. Bloom PP, Donlan J, Torres Soto M, Daidone M, Hohmann E, Chung RT. Fecal microbiota transplant improves cognition in hepatic encephalopathy and its effect varies by donor and recipient. Hepatol Commun 2022;6:2079-89.
92. Hvas CL, Dahl Jørgensen SM, Jørgensen SP, et al. Fecal microbiota transplantation is superior to fidaxomicin for treatment of recurrent clostridium difficile infection. Gastroenterology 2019;156:1324-1332.e3.
93. Juul FE, Garborg K, Bretthauer M, et al. Fecal microbiota transplantation for primary clostridium difficile infection. N Engl J Med 2018;378:2535-6.
94. Haifer C, Paramsothy S, Kaakoush NO, et al. Lyophilised oral faecal microbiota transplantation for ulcerative colitis (LOTUS): a randomised, double-blind, placebo-controlled trial. Lancet Gastroenterol Hepatol 2022;7:141-51.
95. Sokol H, Landman C, Seksik P, et al. Saint-Antoine IBD Network. Fecal microbiota transplantation to maintain remission in Crohn's disease: a pilot randomized controlled study. Microbiome 2020;8:12.
96. Costello SP, Hughes PA, Waters O, et al. Effect of fecal microbiota transplantation on 8-week remission in patients with ulcerative colitis: a randomized clinical trial. JAMA 2019;321:156-64.
97. Wang H, Cui B, Li Q, et al. The safety of fecal microbiota transplantation for Crohn's disease: findings from a long-term study. Adv Ther 2018;35:1935-44.
98. Paramsothy S, Kamm MA, Kaakoush NO, et al. Multidonor intensive faecal microbiota transplantation for active ulcerative colitis: a randomised placebo-controlled trial. Lancet 2017;389:1218-28.
99. Baruch EN, Youngster I, Ben-Betzalel G, et al. Fecal microbiota transplant promotes response in immunotherapy-refractory melanoma patients. Science 2021;371:602-9.
100. Ianiro G, Rossi E, Thomas AM, et al. Faecal microbiota transplantation for the treatment of diarrhoea induced by tyrosine-kinase inhibitors in patients with metastatic renal cell carcinoma. Nat Commun 2020;11:4333.
101. Kakihana K, Fujioka Y, Suda W, et al. Fecal microbiota transplantation for patients with steroid-resistant acute graft-versus-host disease of the gut. Blood 2016;128:2083-8.
102. Zhao Y, Li X, Zhou Y, et al. Safety and efficacy of fecal microbiota transplantation for grade IV steroid refractory GI-GvHD patients: interim results from FMT2017002 trial. Front Immunol 2021;12:678476.
103. Dai M, Liu Y, Chen W, et al. Rescue fecal microbiota transplantation for antibiotic-associated diarrhea in critically ill patients. Crit Care 2019;23:324.
104. Ding X, Li Q, Li P, et al. Long-term safety and efficacy of fecal microbiota transplant in active ulcerative colitis. Drug Saf 2019;42:869-80.
105. Zhang T, Lu G, Zhao Z, et al. Washed microbiota transplantation
106. Lu G, Wang W, Li P, Wen Q, Cui B, Zhang F. Washed preparation of faecal microbiota changes the transplantation related safety, quantitative method and delivery. Microb Biotechnol 2022;15:2439-49.
107. Xiang Z, Wu J, Li J, Zheng S, Wei X, Xu X. Gut microbiota modulation: a viable strategy to address medical needs in hepatocellular carcinoma and liver transplantation. Engineering 2023:online ahead of print.
108. Shen YC, Lee PC, Kuo YL, et al. An exploratory study for the association of gut microbiome with efficacy of immune checkpoint inhibitor in patients with hepatocellular carcinoma. J Hepatocell Carcinoma 2021;8:809-22.
109. Mao J, Wang D, Long J, et al. Gut microbiome is associated with the clinical response to anti-PD-1 based immunotherapy in hepatobiliary cancers. J Immunother Cancer 2021;9:e003334.
110. Sharma A, Roy A, Premkumar M, et al. Fecal microbiota transplantation in alcohol-associated acute-on-chronic liver failure: an openlabel clinical trial. Hepatol Int 2022;16:433-46.
Cite This Article
How to Cite
Dai, M.; Lui R. N.; Lau L. H. S. The role of gut microbiome and fecal microbiota transplantation in liver cancer and related complications: mechanisms and therapeutic potentials. Hepatoma. Res. 2023, 9, 39. http://dx.doi.org/10.20517/2394-5079.2023.33
Download Citation
Export Citation File:
Type of Import
Tips on Downloading Citation
Citation Manager File Format
Type of Import
Direct Import: When the Direct Import option is selected (the default state), a dialogue box will give you the option to Save or Open the downloaded citation data. Choosing Open will either launch your citation manager or give you a choice of applications with which to use the metadata. The Save option saves the file locally for later use.
Indirect Import: When the Indirect Import option is selected, the metadata is displayed and may be copied and pasted as needed.
About This Article
Copyright
Data & Comments
Data
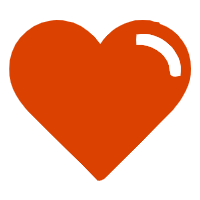
Comments
Comments must be written in English. Spam, offensive content, impersonation, and private information will not be permitted. If any comment is reported and identified as inappropriate content by OAE staff, the comment will be removed without notice. If you have any queries or need any help, please contact us at support@oaepublish.com.