Factors associated with prognosis and staging of intrahepatic cholangiocarcinoma
Abstract
Intrahepatic cholangiocarcinoma (ICC) is a relatively rare but aggressive primary liver cancer with a poor prognosis. A number of established clinical and pathologic factors correlate with prognosis, and this is reflected in the American Joint Committee on Cancer (AJCC) 8th Edition staging manual. Researchers have identified areas for improvement in staging and prognostication of ICC using more nuanced tools, including serum biomarkers, molecular profiling, immunophenotyping, and multimodal prognostic scoring systems. These data have led to proposals of novel staging systems that attempt to improve the correlation between stage and prognosis. More accurate staging tools may aid in treatment decisions that are tailored to each individual patient, to maximize therapy for individuals most likely to benefit and to avoid unnecessary toxicity and decision regret in those for whom aggressive treatment is unlikely to alter outcomes. Artificial intelligence and machine learning may help researchers develop new models that predict outcomes with more accuracy and precision.
Keywords
INTRODUCTION
Intrahepatic cholangiocarcinoma (ICC) is a type of biliary tract cancer (BTC) that is a relatively uncommon aggressive primary liver cancer. Although it shares some similarities with hepatocellular carcinoma (HCC) and extrahepatic cholangiocarcinoma, ICC has unique clinicopathologic, molecular, radiologic, and immune features. The prognosis for ICC is generally poor and increased age, large tumor size, multifocal disease, lymph node metastasis, and vascular invasion are associated with worse overall survival (OS)[1]. The preferred treatment for localized ICC is upfront surgery and adjuvant capecitabine[2-4]. However, even after curative-intent resection, patients are at high risk for recurrence[5]. Chemotherapy, immunotherapy, and targeted therapies are utilized for advanced BTC with variable efficacy[6]. Local therapies, such as stereotactic body radiotherapy, transarterial chemoembolization, or radioembolization, may be used as adjuncts in the advanced setting or for poor operative candidates[3]. The current 8th Edition American Joint Committee on Cancer (AJCC) Cancer Staging Manual uses a tumor-node-metastasis (TNM) staging system for ICC[7]. Although it has evolved over time, the current stages do not always correlate with prognosis[8]. Thus, identification of novel prognostic factors, or refinement of the impact of known prognostic factors, is needed to make treatment decisions for patients with this complex disease.
CLINICOPATHOLOGIC FEATURES
Etiology and patient factors
A variety of risk factors have been identified, including hepatitis B, liver flukes (e.g., Chlonorchis sensis), choledochal cysts, primary sclerosing cholangitis (PSC), hepatolithiasis, and cirrhosis[9]. The prevalence of these risk factors depends heavily on geography, comorbidities, and other individual patient factors. The role of screening among these particular patient populations has not yet been widely adopted but is under active investigation[10]. Interestingly, patients with ICC arising secondary to hepatolithiasis have a worse prognosis but also derive the greatest benefit from adjuvant chemotherapy[11-13]. Data are conflicting regarding the prognosis of hepatitis B-associated ICC relative to other etiologies[14-16]. Malnutrition and frailty are also correlated with inferior outcomes. In particular, sarcopenia has been associated with worse survival outcomes among patients with ICC, including individuals with advanced disease and patients undergoing curative-intent surgery[17,18]. Preoperative nutritional status also impacts survival among patients with ICC. While individuals with lower prognostic nutritional index (PNI) tend to have more aggressive tumor biology with a higher likelihood of multifocal disease, PNI remains an independent predictor of survival on multivariate analysis even after controlling for these factors[19].
The influence of underlying liver disease can be substantial, including younger age, obesity, diabetes, and metabolic-associated liver disease (MALD)[20-24]. Metabolic-associated steatohepatitis, formerly known as nonalcoholic steatohepatitis or NASH, confers a considerably worse prognosis than MALD (previously known as nonalcoholic fatty liver disease or NAFLD); in one study using case-matched cohorts, NASH was present in 22.5% of ICC vs. 6.2% in matched cohorts[25]. Cirrhotic patients with ICC have a worse prognosis relative to non-cirrhotic[26,27]. However, combined HCC-ICC has the worst prognosis compared with either HCC or ICC alone, and likely represents a poorly differentiated, aggressive malignancy[28-30]. Higher Albumin-Bilirubin grade (ALBI) predicts improved overall survival[31]. Lower preoperative gamma-glutamyltransferase (GGT) levels are associated with better prognosis among patients with hepatitis
Unfortunately, no widely adopted screening guidelines or protocols have been identified/implemented to facilitate early detection of ICC[34]. In turn, many patients present with advanced-stage disease. The impact of the COVID-19 pandemic on access to healthcare highlights the need for early detection; patients diagnosed with ICC during COVID-19 were more likely to present at an advanced stage and had higher mortality compared with pre-pandemic cases[35].
TNM staging
T Category
The AJCC 8th edition staging manual assigns T categories based on tumor size, vascular invasion, number of tumors, and invasion through the liver capsule or into other structures[7]. Multifocal ICC correlates with worse survival, but sometimes it can be unclear if multifocal disease represents a single primary tumor with intrahepatic metastasis, or multiple primary de novo tumors arising in a field defect such as cirrhosis or PSC[36]. Central tumors are at higher risk as well; in particular, ICC extending below the second bile duct confluence and those abutting hilar structures have a worse prognosis[37,38]. These factors may influence the likelihood of margin-negative resection. Margin status (positive or negative), as well as the extent of margin width, impacts survival[39-42]. In particular, margins of at least 1 cm confer improved survival compared with margin-negative resection with a width of < 1 cm[39].
Pathologic features
Three distinct growth patterns have been described in ICC: periductal infiltrating, which has been associated with the worst prognosis, vs. mass-forming and intraductal growth, which have a better prognosis[43]. The degree of tumor necrosis and the presence of lymphovascular (LVI) and perineural invasion (PNI) also correlate with long-term outcomes[44-46]. Tumor grade and differentiation alone demonstrate a modest correlation with prognosis, but the combination of these factors, termed histologic glandular differentiation, discriminates high-risk from low-risk ICC better than either factor alone[47]. Additional histologic findings continue to emerge, such as the presence of small nerve fibers, sarcomatoid change, degree of lymphangiogenesis, and tumor vascularity, with hypervascular tumors conferring a better prognosis[48-51]. The role of the tumor microbiome is being actively studied in other disease sites and may play a role in the prognosis of ICC as well[52].
N category
Node involvement without distant metastasis represents stage IIIB disease[7]. Lymph node metastases, both on preoperative imaging and final pathologic evaluation, confer a worse prognosis[53,54]. Nodal involvement is classified as Nx, N0, or N1; the presence of three or more involved nodes, or any metastasis to nodes beyond station 12, is associated with a worse prognosis[55].
Performing a lymphadenectomy does not independently improve survival for patients with ICC[56,57]. However, the location and extent of nodal metastases correlate with prognosis, and it is recommended to harvest at least 6 nodes at the time of curative-intent operation for optimal staging[55]. Although 6 nodes have been established as the minimum required for accurate staging, the optimal nodal harvest may be dependent on tumor size. For example, in one study, patients with tumors < 3 cm in size had accurate staging with 7 nodes harvested, whereas individuals with tumors ≥ 3 cm required 11 nodes for accurate staging[58].
Novel staging systems
The AJCC 8th edition remains the primary staging system used in North America. Compared with the 7th edition staging system, the 8th edition performs better relative to prognostic accuracy[59]. Novel staging systems for ICC have been proposed with the goal of improving the correlation between stage and prognosis. One such example is the Clinical Staging system (CS-iCCA) that used the Eastern Cooperative Oncology Group (ECOG) score, number of tumors, tumor size, metastasis, albumin, and carbohydrate antigen 19-9 (CA 19-9) to stratify patients into four stages. This system performed better than the TNM staging system in the derivation cohort, but not in the validation cohort[60]. The MEGNA score incorporated multifocality, extent of tumor, grade, nodal status, and age and performed similarly to the AJCC 8th edition staging system for OS, but not for RFS[61].
SURGICAL FACTORS
Anatomic vs. non-anatomic resection
It is unclear if ICC grows along the portal tributaries in a similar way to HCC. As such, the benefit of anatomic vs. non-anatomic resection remains controversial. Anatomic resection has been retrospectively compared with non-anatomic resection and conferred survival benefit in a propensity score matched cohort[62]. Another study noted that major hepatectomy, which was correlated with an anatomic resection, was associated with a risk of recurrence[63]. However, this study was not matched using propensity scoring, so the results may reflect the prognostic influence of tumor size rather than the type of resection. Another study suggested that margin width matters more than anatomic vs. non-anatomic hepatectomy[64]. These findings should be carefully considered in an era of increasing use of non-anatomic hepatectomy, even for large or multifocal tumors.
Role of minimally invasive hepatectomy
In a nonrandomized study comparing minimally invasive (MIS) to open hepatectomy, MIS was associated with better long-term survival outcomes[65]. Among patients at risk for very early recurrence, minimally invasive surgery was associated with improved long-term outcomes[66]. These data may be related to patients being able to start adjuvant chemotherapy earlier after MIS compared with open hepatectomy. Minimally invasive major hepatectomy is best reserved for high-volume surgeons at high-volume centers[67,68]. Additionally, while debated, some data have suggested that minimally invasive approaches may be less likely to achieve adequate lymphadenectomy[69].
BIOMARKERS
Circulating biomarkers
Serum tumor markers
CA 19-9 is an established serum tumor marker in cholangiocarcinoma. Among patients with ICC, elevated preoperative CA 19-9 can predict lymph node metastasis, early recurrence, and worse overall survival
Biomarkers and their correlation with worse prognosis in intrahepatic cholangiocarcinoma
Patient factors | Tumor markers | Inflammatory markers | Somatic mutations | Immune markers |
Low ALBI | High CA 19-9 | High NLR | TP53 | High TMB |
Low PNI | High CEA | High PLR | KRAS | Low PD-1/PD-L1 |
CTC | High SII | ARID1A | Low TIL | |
High nardilysin | BRAF | Low TLS | ||
FGFR2 fusion |
Inflammatory biomarkers
Newer inflammatory biomarker scores, based on values captured during routine laboratory testing, are increasingly incorporated into predictive outcome models for ICC [Table 1]. These biomarkers are rooted in the principle of cancer-related inflammation in which low-level “smoldering” inflammation in the tumor microenvironment (TME) may be associated with progression and metastasis[73]. The neutrophil-to-lymphocyte ratio (NLR) is one such index and has been correlated with ICC prognosis[74-76]. Platelet-to-lymphocyte ratio (PLR) has also been studied among patients with ICC[77,78]. A newer index, termed the systemic immune-inflammation index (SII), is comprised of platelet count multiplied by NLR[79]. In a multi-institutional database study, SII was the strongest prognostic value on multivariate analysis compared with other inflammatory indices. High SII was associated with a 5-year OS of 37.7% vs. 46.6% (P < 0.001)[79]. These indices have been further combined with nutritional markers. A high fibrinogen-albumin ratio index was associated with reduced RFS[80]. A combination of ALBI, SII, NLR, and prognostic nutritional index, termed the Prognostic Immune-Inflammatory-Nutritional score (PIIN), stratified patients with ICC into low- and high-risk groups relative to OS and RFS[81].
Other immune-related biomarkers have been described, such as nardilysin, a metalloendopeptidase that plays a role in tumor immune response. Nardilysin is intriguing as a measurable serum marker for molecular changes in the TME. High nardilysin levels were correlated with inferior OS and DFS, which was associated with messenger RNA (mRNA) expression in resected ICC specimens[82]. With increasing interest in molecular detection of cancer in the blood, such as circulating tumor DNA (ctDNA), circulating tumor cell count has been correlated with long-term outcomes in patients with advanced ICC[83]. However, to date, ctDNA has not been well studied in ICC.
Molecular biomarkers
Correlation between driver mutations and disease behavior has been a topic of increased interest across several different cancer types. The molecular phenotype of ICC is variable [Table 1][84]. The most common mutations identified include tumor protein p53 (TP53), isocitrate dehydrogenase (IDH1 and IDH2), Kirsten rat sarcoma virus (KRAS), AT-rich interactive domain-containing protein 1A (ARID1A), hepatocyte growth factor receptor (C-MET), V-raf murine sarcoma viral oncogene homolog B (BRAF), and fibroblast growth factor receptor 2 (FGFR2) fusion[85]. At least one mutation can be identified in about 60% of BTC[86]. Identification of somatic mutations can influence treatment decisions, as the landscape of targeted agents continues to broaden[87].
Somatic mutations that confer the worst overall survival prognosis are TP53 and KRAS. These mutations have been associated with increased tumor mutational burden and advanced stage at diagnosis[88,89]. Co-mutation of TP53 and KRAS is associated with particularly poor prognosis and aggressive biology[90]. ICC with BRAF V600E mutation also tends to present at a more advanced stage and has been associated with chemotherapy resistance, though targeted therapies may be an option in the advanced setting[88,91,92]. The presence of FGFR2 fusion has a variable impact on prognosis, depending on the stage at presentation[93-95]. Multiple inhibitors of the FGFR2 fusion product have been developed, with generally favorable results in cholangiocarcinoma[96]. A multitude of novel molecular alterations have been described, as the search for druggable targets increases in a tumor-agnostic manner. Dysregulated angiogenesis and neovascularization and epigenetic changes associated with epithelial-to-mesenchymal transition can drive tumor growth and metastasis, and may be amenable to targeted therapies[97-99]. Interestingly, somatic mutations in IDH1 and IDH2, which are components of the tricarboxylic acid cycle, do not influence prognosis independently, implying that there are other metabolism-related changes at the cellular level that may influence tumor biology, such as an interplay between IDH1/2 and copper- or iron-mediated apoptosis[100-103].
Immune biomarkers
Interest in applying immune therapy to cancers originally thought to be immunologically “cold” has increased dramatically and has been investigated in a biomarker-driven manner[104]. In terms of immunogenicity and sensitivity to immune therapy, cholangiocarcinoma lies somewhere between highly immunogenic tumors such as melanoma and immunologically “cold” tumors such as pancreatic ductal adenocarcinoma. Indeed, several inflammatory-immune markers, as described above, have consistently demonstrated a correlation with disease behavior, response to therapy, and outcomes [Table 1]. Furthermore, immune therapy is now part of first-line standard systemic therapy for advanced biliary tract cancers[6].
Tumor mutational burden (TMB) correlates with poor differentiation, aggressive biology, and immunogenicity, and confers a worse prognosis in ICC[105]. Other molecular features of the TME have been correlated with prognosis, such as the immune checkpoint interaction of programmed death receptor 1 (PD-1) and programmed death ligand 1 (PD-L1). Increased PD-1/PD-L1 expression and the number and type of tumor-infiltrating lymphocytes (TIL) have been demonstrated to confer a better prognosis, whereas decreased TIL and decreased immune checkpoint expression represent an immune-privileged phenotype with a worse prognosis[106-109]. Other immune checkpoints such as B7-H3 have been more recently described and demonstrate a similar relationship with outcomes[110]. In addition to NLR, SII, and other indices, the lymphocyte to C-reactive protein (CRP) ratio may be able to infer immunophenotype based on serum markers[111].
Other components of the TME are important as well. For example, macrophage phenotype, in terms of
The role of cancer-associated fibroblasts (CAF) in the tumor stroma is under active investigation in cholangiocarcinoma as well as other cancers. In vitro studies have demonstrated that IL-6 secreted by CAFs prevents autophagy in the TME and allows for tumor growth and immune exclusion[122]. This finding was corroborated in a series of 70 patients with resected ICC in which IL-6 expression correlated with worse prognosis and chemotherapy resistance[123]. This finding may support a role for investigating autophagy-related agents such as resveratrol to treat ICC[124].
As interest in biomarker-driven therapy increases, these immune markers will become increasingly important. An RNA immune signature has been proposed as a predictor of response to chemo-immunotherapy[125,126]. Tumor stemness, based on the expression of ANO1, CD109, and CTNND2, correlates with immunophenotype in which lower stemness is associated with an immune exhausted phenotype with a TME rich in TIL, as well as increased sensitivity to chemotherapy including cisplatin and fluorouracil[127]. A histopathology-based immunoscore, expression of IL23R, and a myeloid-based nomogram originally derived in HCC have also been proposed as immune-related biomarkers[128-130].
IMAGING AND RADIOMICS
Standard imaging techniques
High-quality imaging is essential for the diagnosis and staging of ICC. Computed tomography (CT), magnetic resonance imaging (MRI), and ultrasound all have a role in diagnosing and staging ICC. Initially validated in HCC, the Liver Imaging Reporting and Data System (LI-RADS) can be applied to the imaging characteristics of ICC and has been associated with overall prognosis. For example, the LR-M category was independently associated with inferior RFS (HR 8.10, 95%CI: 1.1-58.9), whereas LR-4 and LR-5 were not associated with differences in RFS[131]. Positron emission tomography with 18-fluorodeoxyglucose
Radiomics
Given the considerable heterogeneity in the ability of imaging to risk-stratify cholangiocarcinoma, alternative approaches need to be investigated. Radiomics is an evolving area of study based on the use of machine learning to identify radiologic predictors of disease biology[135]. Radiomics may be able to predict early postoperative recurrence risk based on contrast-enhanced CT scans[136]. Pertinent features include tumor shape (e.g., border irregularity or contour) and intralesional heterogeneity[137-140]. Radiomics may also be used to predict features of the TME. In particular, the application of machine learning to MRI was able to identify PD-1/PD-L1 expression[141]. Another study used machine learning and CT imaging to predict the prevalence of tertiary lymphoid structures[142]. As the prevalence of artificial intelligence (AI) increases within medicine and society, these approaches are likely to undergo further study, though their application currently has practical limitations.
PREDICTIVE MODELS
Risk of recurrence
Even in stage I ICC, approximately half of patients will recur; most of these recurrences (85%) occur within 12 months. Risk factors for recurrence in stage I ICC include CEA, SII, tumor size, and omission of lymphadenectomy[5]. Further risk stratification of early-stage ICC is possible using CA 19-9, CEA, tumor size, grade and degree of differentiation, and T stage. Low-risk early-stage patients had an OS of over 5 years and high-risk patients had a median OS of 12 months and a RFS of approximately 6 months[143]. Prognostic risk models may be used to guide adjuvant therapy decisions[4,144]. The Prognostic Recurrence Score (PRS) uses American Society of Anesthesiology classification (1 point), nodal metastasis or unsampled lymph nodes (N1 or Nx, 1 point), margin positive resection (1 point), tumor grade 3 or 4 (1 point), CA 19-9 > 37 at time of recurrence (2 points), CEA > 5 at recurrence (2 points), bilateral recurrence (1 point), and early recurrence (1 point) to quantify risk of recurrence. A PRS score of 3 or greater classifies patients as high-risk, with a median RFS of 36% (compared with 63% in the low-risk group) [Table 2][145].
Validated scoring and staging systems for intrahepatic cholangiocarcinoma, their components, and predicted outcome(s), in order of appearance in the manuscript
Score/system | Patient | Tumor | Node | Metastasis | Biomarkers | Predicts |
AJCC8 TNM[7] | Size, number, vascular invasion, extrahepatic invasion | + | + | OS by stage | ||
CS-iCCA[60] | ECOG | Number, size | + | Albumin, | OS by stage | |
MEGNA[61] | Age | Extent, grade, number | + | OS by stage | ||
Guangzhou[143] | Size, grade, T stage | CA 19-9, CEA | OS, RFS | |||
PRS[145] | ASA | Margins, grade, bilateral at recurrence, DFI | + | CA 19-9, CEA | RFS | |
VER (Preop)[66] | Age, race, cirrhosis | Size, number | + | Risk of VER | ||
VER (Postop)[66] | Age, race | Size, number, vascular invasion, margins | + | Risk of VER | ||
Chengdu[152] | TBS | CA 19-9, CEA, NLR | ||||
Subtype[153] | TBS | CA 19-9, NLR | OS by common, proliferative, inflammatory subtype | |||
Discordance[155] | Size | + | CEA, NLR | Discordance between preop/postop risk |
It is important to identify patients at risk for very early recurrence (VER), defined as recurrence within
Multimodality scoring systems
The Tumor Burden Score (TBS) was originally described for HCC, but has been adopted in ICC and CRLM and has been demonstrated to be an independent predictor of prognosis[150]. TBS is calculated with the formula TBS2 = (maximum tumor size)2 + (number of tumors)2[151]. TBS has also been incorporated as a component of other scoring systems. Combining TBS with NLR, CA 19-9, and CEA predicted survival among patients with ICC[152]. When combining TBS with CA 19-9 and NLR, patients with ICC were grouped into common, proliferative, and inflammatory subtypes. The inflammatory subtype had the worst prognosis (median OS 22.9 months) and a higher risk of local and distant recurrence despite lower T and N stages; the common subtype had the best prognosis (median OS 72.0 months)[153]. In a separate analysis of patients with recurrent ICC within a multi-institutional database, hazard function analysis demonstrated that TBS, T stage, and N stage were the strongest predictors of recurrence, and that adjuvant chemotherapy delayed recurrence among individuals with involved or unsampled lymph nodes[154].
An unresolved issue in the assessment of staging and prognosis in ICC is the imprecise correlation between preoperative and postoperative risk assessment tools in surgically resectable patients. In a multi-institutional retrospective study by Moro et al., preoperative variables were used to develop a preoperative prognostic model, and pre- and postoperative variables were used to develop a postoperative prognostic model; these two models were compared to determine the degree of discordance[155]. On multivariate analysis, tumor size, NLR, CEA, and patients with clinically metastatic nodes on preoperative imaging were more likely to have discordance between pre- and postoperative assessment [Table 2]. These findings highlight the need for more accurate preoperative prognostic tools to aid treatment decisions[155].
CONCLUSION
It is difficult to predict prognosis accurately in patients with ICC. Until recently, much of the literature regarding prognostic ICC factors has been limited to a single focus, such as individual clinical or pathologic factors or particular molecular alterations. The integration of various factors across domains is increasingly used to describe prognosis and propose improvements in staging systems. The use of machine learning in some statistical models, like the random forest model, has been able to identify novel factors that predict early recurrence. The best role for these machine learning models is likely in integrating the most relevant prognostic factors in an accurate and proportional way; in one published machine learning model, the most important prognostic factors to predict early recurrence were TBS, low CA 19-9 (< 200 U/mL), microvascular invasion, perineural invasion, and node-positive or inadequately staged nodal status, all of which are known prognostic factors related to ICC[156]. This role for AI and machine learning continues to evolve, with the goal of identifying the most accurate clinicopathologic factors, serum biomarkers, and molecular/immune factors to predict disease prognosis[157]. Machine learning models may be able to prognosticate and group patients with ICC into more accurate groups based on a multitude of factors[158]. These prediction models are particularly important in terms of guiding therapy decisions when the benefit of high-risk interventions such as hepatectomy and adjuvant chemotherapy may be less clear. Aggressive therapy in patients at high risk for recurrence may increase the risk of decision regret, a source of distress for patients and clinicians alike[159,160]. More data are needed on the relationship between prognostic models, shared decision-making, unfavorable outcomes such as very early recurrence, and decision regret.
The use of large data sets, particularly when informed by AI tools such as natural language processing, may be able to uncover additional prognostic factors or associations that may not be intuitive. There are innumerable factors that could influence disease-specific and treatment outcomes in ICC, some of which may be surprising. For example, patients on calcium channel blockers for cardiovascular indications had improved outcomes compared with those not on these medications[161]. However, novel AI and machine learning tools should be applied with caution, as this approach may be subject to a new set of biases[162].
The studies outlined herein are interesting and thought-provoking, but the practical application of these data, particularly in terms of prognostic calculators and incorporation into widely used staging manuals, is not clearly defined. Multiple studies have demonstrated that a simple TNM classification system is inadequate for ICC[8]. Given that several important prognostic factors are omitted from the TNM system, the incorporation of other factors such as tumor markers, molecular features, immune phenotype, and radiomic data could improve the existing staging systems. This approach has been adopted in breast cancer. For example, the AJCC 8th edition staging manual for breast cancer incorporates hormone receptor status, ERBB2/HER2 status, and tumor grade, as well as preoperative and postoperative TNM staging, to provide a more accurate prognosis based on stage[163]. However, it may be prohibitively challenging to incorporate more nuanced techniques such as radiomics and immune phenotypes into staging algorithms that are intended to be universally used. Accurate staging remains a challenge in ICC, evidenced by the variety of available literature. Given the heterogeneity of ICC and the multimodality of prognostic factors, AI and machine learning may assist in synthesizing a coherent and broadly applicable prognostic and staging model.
DECLARATIONS
Authors’ contributions
Made substantial contributions to the conception and design of the study and performed data analysis and interpretation: Chick RC, Ruff SM, Pawlik TM
Availability of data and materials
Not applicable.
Financial support and sponsorship
None.
Conflicts of interest
All authors declared that there are no conflicts of interest.
Ethical approval and consent to participate
Not applicable.
Consent for publication
Not applicable.
Copyright
© The Authors 2024.
REFERENCES
1. Mavros MN, Economopoulos KP, Alexiou VG, Pawlik TM. Treatment and prognosis for patients with intrahepatic cholangiocarcinoma: systematic review and meta-analysis. JAMA Surg 2014;149:565-74.
2. Ruff SM, Pawlik TM. Clinical management of intrahepatic cholangiocarcinoma: surgical approaches and systemic therapies. Front Oncol 2024;14:1321683.
3. NCCN guidelines version: biliary tract cancers. National comprehensive cancer network. Available from: https://www.nccn.org/professionals/physician_gls/pdf/btc.pdf [Last accessed on 8 Jul 2024].
4. Primrose JN, Fox RP, Palmer DH, et al. Capecitabine compared with observation in resected biliary tract cancer (BILCAP): a randomised, controlled, multicentre, phase 3 study. Lancet Oncol 2019;20:663-73.
5. Kawashima J, Sahara K, Shen F, et al. Predicting risk of recurrence after resection of stage I intrahepatic cholangiocarcinoma. J Gastrointest Surg 2024;28:18-25.
6. Oh DY, Ruth He A, Qin S, et al. Durvalumab plus gemcitabine and cisplatin in advanced biliary tract cancer. NEJM Evid 2022;1:EVIDoa2200015.
7. Amin MB, American Joint Committee on Cancer, American Cancer Society. AJCC cancer staging manual. 8th ed. Berlin: Springer; 2017.
8. Zhang XF, Xue F, He J, et al. Proposed modification of the eighth edition of the AJCC staging system for intrahepatic cholangiocarcinoma. HPB 2021;23:1456-66.
9. Maithel SK, Gamblin TC, Kamel I, Corona-Villalobos CP, Thomas M, Pawlik TM. Multidisciplinary approaches to intrahepatic cholangiocarcinoma. Cancer 2013;119:3929-42.
10. Thinkhamrop K, Khuntikeo N, Chamadol N, Suwannatrai AT, Phimha S, Kelly M. Associations between ultrasound screening findings and cholangiocarcinoma diagnosis in an at-risk population. Sci Rep 2022;12:13513.
11. Li Q, Chen C, Su J, et al. Recurrence and prognosis in intrahepatic cholangiocarcinoma patients with different etiology after radical resection: a multi-institutional study. BMC Cancer 2022;22:329.
12. Zhang XF, Chakedis J, Bagante F, et al. Implications of intrahepatic cholangiocarcinoma etiology on recurrence and prognosis after curative-intent resection: a multi-institutional study. World J Surg 2018;42:849-57.
13. Zhu Y, Zhu Y, Cai F, Zhao J, Liu F. Prognostic risk factors associated with recurrence and metastasis after radical resection in patients with hepatolithiasis complicated by intrahepatic cholangiocarcinoma. Cell Biochem Biophys 2015;73:455-60.
14. Xu XB, Hu C, Yang HJ, Zheng SS. Isolated anti-HBc is an independent risk factor for tumor recurrence in intrahepatic cholangiocarcinoma after curative resection. Hepatob Pancreat Dis Int 2022;21:472-8.
15. Wu ZF, Wu XY, Zhu N, et al. Prognosis after resection for hepatitis B virus-associated intrahepatic cholangiocarcinoma. World J Gastroenterol 2015;21:935-43.
16. Li Z, Gao Q, Wu Y, et al. HBV infection effects prognosis and activates the immune response in intrahepatic cholangiocarcinoma. Hepatol Commun 2024;8:e0360.
17. Taniai T, Haruki K, Yanagaki M, et al. Osteosarcopenia predicts poor prognosis for patients with intrahepatic cholangiocarcinoma after hepatic resection. Surg Today 2023;53:82-9.
18. Yugawa K, Itoh S, Kurihara T, et al. Skeletal muscle mass predicts the prognosis of patients with intrahepatic cholangiocarcinoma. Am J Surg 2019;218:952-8.
19. Akgül Ö, Bagante F, Olsen G, et al. Preoperative prognostic nutritional index predicts survival of patients with intrahepatic cholangiocarcinoma after curative resection. J Surg Oncol 2018;118:422-30.
20. Kim JH, Cheon YK, Lee TY, Lee SH, Chung H. Effect of age on the prognosis of intrahepatic cholangiocarcinoma. Korean J Intern Med 2023;38:39-47.
21. Lurje I, Uluk D, Pavicevic S, et al. Body composition is associated with disease aetiology and prognosis in patients undergoing resection of intrahepatic cholangiocarcinoma. Cancer Med 2023;12:17569-80.
22. Merath K, Mehta R, Hyer JM, et al. Impact of body mass index on tumor recurrence among patients undergoing curative-intent resection of intrahepatic cholangiocarcinoma- a multi-institutional international analysis. Eur J Surg Oncol 2019;45:1084-91.
23. Yu Q, Lei Z, Ma W, et al. Postoperative prognosis of non-alcoholic fatty liver disease-associated intrahepatic cholangiocarcinoma: a multi-center propensity score matching analysis. J Gastrointest Surg 2023;27:2403-13.
24. Fu K, Yang X, Wu H, Gong J, Li X. Diabetes and PKM2 affect prognosis in patients with intrahepatic cholangiocarcinoma. Oncol Lett 2020;20:265.
25. De Lorenzo S, Tovoli F, Mazzotta A, et al. Non-alcoholic steatohepatitis as a risk factor for intrahepatic cholangiocarcinoma and its prognostic role. Cancers 2020;12:3182.
26. Fei Y, Pan X, Wei F. The impact of cirrhosis on the prognosis of intrahepatic cholangiocarcinoma after surgery. Asian J Surg 2023;46:1361-3.
27. Zhang JX, Li P, Chen Z, et al. Impact of liver fibrosis score on prognosis after common therapies for intrahepatic cholangiocarcinoma: a propensity score matching analysis. BMC Cancer 2020;20:556.
28. Amory B, Goumard C, Laurent A, et al. Combined hepatocellular-cholangiocarcinoma compared to hepatocellular carcinoma and intrahepatic cholangiocarcinoma: different survival, similar recurrence: report of a large study on repurposed databases with propensity score matching. Surgery 2024;175:413-23.
29. Song P, Midorikawa Y, Nakayama H, et al. Patients’ prognosis of intrahepatic cholangiocarcinoma and combined hepatocellular-cholangiocarcinoma after resection. Cancer Med 2019;8:5862-71.
30. Jung DH, Hwang S, Kim KH, et al. Clinicopathological features and post-resection prognosis of double primary hepatocellular carcinoma and intrahepatic cholangiocarcinoma. World J Surg 2017;41:825-34.
31. Kaneko S, Kurosaki M, Tsuchiya K, et al. Prognosis of intrahepatic cholangiocarcinoma stratified by albumin-bilirubin grade. Hepatol Res 2021;51:902-8.
32. Zhang B, Liu S, Zhou B, et al. High serum gamma-glutamyl transpeptidase concentration associates with poor postoperative prognosis of patients with hepatitis B virus-associated intrahepatic cholangiocarcinoma. Ann Transl Med 2021;9:17.
33. Lu Z, Liu S, Yi Y, et al. Serum gamma-glutamyl transferase levels affect the prognosis of patients with intrahepatic cholangiocarcinoma who receive postoperative adjuvant transcatheter arterial chemoembolization: a propensity score matching study. Int J Surg 2017;37:24-8.
34. Association for the Study of the Liver. Electronic address: easloffice@easloffice.eu, European association for the study of the liver. EASL-ILCA clinical practice guidelines on the management of intrahepatic cholangiocarcinoma. J Hepatol 2023;79:181-208.
35. Geh D, Watson R, Sen G, et al. COVID-19 and liver cancer: lost patients and larger tumours. BMJ Open Gastroenterol 2022;9:e000794.
36. Turner KM, Delman AM, Kharofa J, et al. A national assessment of T2 staging for intrahepatic cholangiocarcinoma and the poor prognosis associated with multifocality. Ann Surg Oncol 2022;29:5094-102.
37. Sohn HJ, Kim H, Kim JR, et al. Predicting prognosis and evaluating the benefits of adjuvant chemotherapy depending on the tumor location in intrahepatic cholangiocarcinoma: focusing on the involvement of below 2nd bile duct confluence. Ann Surg Treat Res 2022;102:248-56.
38. Zhang XF, Bagante F, Chen Q, et al. Perioperative and long-term outcome of intrahepatic cholangiocarcinoma involving the hepatic hilus after curative-intent resection: comparison with peripheral intrahepatic cholangiocarcinoma and hilar cholangiocarcinoma. Surgery 2018;163:1114-20.
39. Spolverato G, Yakoob MY, Kim Y, et al. The impact of surgical margin status on long-term outcome after resection for intrahepatic cholangiocarcinoma. Ann Surg Oncol 2015;22:4020-8.
40. Li MX, Bi XY, Li ZY, et al. Impaction of surgical margin status on the survival outcome after surgical resection of intrahepatic cholangiocarcinoma: a systematic review and meta-analysis. J Surg Res 2016;203:163-73.
41. Jiang JH, Fang DZ, Hu YT. Influence of surgical margin width on survival rate after resection of intrahepatic cholangiocarcinoma: a systematic review and meta-analysis. BMJ Open 2023;13:e067222.
42. Wu L, Tsilimigras DI, Paredes AZ, et al. Trends in the incidence, treatment and outcomes of patients with intrahepatic cholangiocarcinoma in the USA: facility type is associated with margin status, use of lymphadenectomy and overall survival. World J Surg 2019;43:1777-87.
43. Bagante F, Spolverato G, Weiss M, et al. Impact of morphological status on long-term outcome among patients undergoing liver surgery for intrahepatic Cholangiocarcinoma. Ann Surg Oncol 2017;24:2491-501.
44. Tsilimigras DI, Ejaz A, Cloyd J, et al. Tumor necrosis impacts prognosis of patients undergoing resection for T1 intrahepatic cholangiocarcinoma. Ann Surg Oncol ;2022:4326-34.
45. Bartsch F, Heuft LK, Baumgart J, et al. Influence of lymphangio (L), vascular (V), and perineural (Pn) invasion on recurrence and survival of resected intrahepatic cholangiocarcinoma. J Clin Med 2021;10:2426.
46. Shao C, Chen J, Shi J, Huang L, Qiu Y. Histological classification of microvascular invasion to predict prognosis in intrahepatic cholangiocarcinoma. Int J Clin Exp Pathol 2017;10:7674-81.
47. Kosaka H, Ishida M, Ueno M, et al. Impact of trinal histological glandular differentiation grade on the prognosis of patients with intrahepatic cholangiocarcinoma: a multicenter retrospective study. J Gastrointest Surg 2023;27:2780-6.
48. Bednarsch J, Tan X, Czigany Z, et al. The presence of small nerve fibers in the tumor microenvironment as predictive biomarker of oncological outcome following partial hepatectomy for intrahepatic cholangiocarcinoma. Cancers 2021;13:3661.
49. Wang T, Kong J, Yang X, Shen S, Zhang M, Wang W. Clinical features of sarcomatoid change in patients with intrahepatic cholangiocarcinoma and prognosis after surgical liver resection: a propensity score matching analysis. J Surg Oncol 2020;121:524-37.
50. Sha M, Jeong S, Wang X, et al. Tumor-associated lymphangiogenesis predicts unfavorable prognosis of intrahepatic cholangiocarcinoma. BMC Cancer 2019;19:208.
51. Türkoğlu MA, Yamamoto Y, Sugiura T, et al. The favorable prognosis after operative resection of hypervascular intrahepatic cholangiocarcinoma: a clinicopathologic and immunohistochemical study. Surgery 2016;160:683-90.
52. Xin HY, Zou JX, Sun RQ, et al. Characterization of tumor microbiome and associations with prognosis in intrahepatic cholangiocarcinoma. J Gastroenterol 2024;59:411-23.
53. Wang J, Shu M, Peng H, et al. The influence of the extent of lymph node metastasis on the prognosis for patients with intrahepatic cholangiocarcinoma. Ann Surg Treat Res 2023;104:258-68.
54. Bartsch F, Hahn F, Müller L, et al. Relevance of suspicious lymph nodes in preoperative imaging for resectability, recurrence and survival of intrahepatic cholangiocarcinoma. BMC Surg 2020;20:75.
55. Zhang XF, Xue F, Dong DH, et al. Number and station of lymph node metastasis after curative-intent resection of intrahepatic cholangiocarcinoma impact prognosis. Ann Surg 2021;274:e1187-95.
56. Li F, Jiang Y, Jiang L, et al. Effect of lymph node resection on prognosis of resectable intrahepatic cholangiocarcinoma: a systematic review and meta-analysis. Front Oncol 2022;12:957792.
57. Zhu J, Liu C, Li H, et al. Adequate lymph node dissection is essential for accurate nodal staging in intrahepatic cholangiocarcinoma: a population-based study. Cancer Med 2023;12:8184-98.
58. Zhang R, Zhang J, Chen C, et al. The optimal number of examined lymph nodes for accurate staging of intrahepatic cholangiocarcinoma: a multi-institutional analysis using the nodal staging score model. Eur J Surg Oncol 2023;49:1429-35.
59. Spolverato G, Bagante F, Weiss M, et al. Comparative performances of the 7th and the 8th editions of the American Joint Committee on Cancer staging systems for intrahepatic cholangiocarcinoma. J Surg Oncol 2017;115:696-703.
60. Lozada ME, Zhang N, Jin W, et al. CS-iCCA, a new clinically based staging system for intrahepatic cholangiocarcinoma: establishment and external validation. Am J Gastroenterol 2023;118:2173-83.
61. Shaikh CF, Alaimo L, Moazzam Z, et al. Predicting overall and recurrence-free survival in patients with intrahepatic cholangiocarcinoma using the MEGNA score: a multi-institutional analysis. J Surg Oncol 2023;127:73-80.
62. Wang C, Ciren P, Danzeng A, et al. Anatomical resection improved the outcome of intrahepatic cholangiocarcinoma: a propensity score matching analysis of a retrospective cohort. J Oncol 2022;2022:4446243.
63. Langella S, Russolillo N, Ossola P, et al. Recurrence after curative resection for intrahepatic cholangiocarcinoma: how to predict the chance of repeat hepatectomy? J Clin Med 2021;10:2820.
64. Zhang XF, Bagante F, Chakedis J, et al. Perioperative and long-term outcome for intrahepatic cholangiocarcinoma: impact of major versus minor hepatectomy. J Gastrointest Surg 2017;21:1841-50.
65. Ratti F, Maina C, Clocchiatti L, et al. Minimally invasive approach provides oncological benefit in patients with high risk of very early recurrence (VER) after surgery for intrahepatic cholangiocarcinoma (iCCA). Ann Surg Oncol 2024;31:2557-67.
66. Tsilimigras DI, Sahara K, Wu L, et al. Very early recurrence after liver resection for intrahepatic cholangiocarcinoma: considering alternative treatment approaches. JAMA Surg 2020;155:823-31.
67. Carpenter EL, Thomas KK, Adams AM, et al. Modern trends in minimally invasive versus open hepatectomy for colorectal liver metastasis: an analysis of ACS-NSQIP. Surg Endosc 2023;37:5591-602.
68. Vreeland TJ, Collings AT, Ozair A, et al. SAGES/AHPBA guidelines for the use of minimally invasive surgery for the surgical treatment of colorectal liver metastases (CRLM). Surg Endosc 2023;37:2508-16.
69. Martin SP, Drake J, Wach MM, et al. Laparoscopic approach to intrahepatic cholangiocarcinoma is associated with an exacerbation of inadequate nodal staging. Ann Surg Oncol 2019;26:1851-7.
70. Yamada T, Nakanishi Y, Okamura K, et al. Impact of serum carbohydrate antigen 19-9 level on prognosis and prediction of lymph node metastasis in patients with intrahepatic cholangiocarcinoma. J Gastroenterol Hepatol ;2018:1626-33.
71. Bergquist JR, Ivanics T, Storlie CB, et al. Implications of CA19-9 elevation for survival, staging, and treatment sequencing in intrahepatic cholangiocarcinoma: a national cohort analysis. J Surg Oncol 2016;114:475-82.
72. He C, Zhang Y, Song Y, et al. Preoperative CEA levels are supplementary to CA19-9 levels in predicting prognosis in patients with resectable intrahepatic cholangiocarcinoma. J Cancer 2018;9:3117-28.
73. Mantovani A, Allavena P, Sica A, Balkwill F. Cancer-related inflammation. Nature 2008;454:436-44.
74. Choi WJ, Perez FM, Gravely A, et al. Preoperative neutrophil-to-lymphocyte ratio is prognostic for early recurrence after curative intrahepatic cholangiocarcinoma resection. Ann Hepatobiliary Pancreat Surg 2023;27:158-65.
75. Omichi K, Cloyd JM, Yamashita S, et al. Neutrophil-to-lymphocyte ratio predicts prognosis after neoadjuvant chemotherapy and resection of intrahepatic cholangiocarcinoma. Surgery 2017;162:752-65.
76. Chen Q, Yang LX, Li XD, et al. The elevated preoperative neutrophil-to-lymphocyte ratio predicts poor prognosis in intrahepatic cholangiocarcinoma patients undergoing hepatectomy. Tumour Biol 2015;36:5283-9.
77. Huh G, Ryu JK, Chun JW, et al. High platelet-to-lymphocyte ratio is associated with poor prognosis in patients with unresectable intrahepatic cholangiocarcinoma receiving gemcitabine plus cisplatin. BMC Cancer 2020;20:907.
78. Chen Q, Dai Z, Yin D, et al. Negative impact of preoperative platelet-lymphocyte ratio on outcome after hepatic resection for intrahepatic cholangiocarcinoma. Medicine 2015;94:e574.
79. Tsilimigras DI, Moris D, Mehta R, et al. The systemic immune-inflammation index predicts prognosis in intrahepatic cholangiocarcinoma: an international multi-institutional analysis. HPB 2020;22:1667-74.
80. Liu H, Qiu G, Hu F, Wu H. Fibrinogen/albumin ratio index is an independent predictor of recurrence-free survival in patients with intrahepatic cholangiocarcinoma following surgical resection. World J Surg Oncol 2021;19:218.
81. Zhu J, Wang D, Liu C, et al. Development and validation of a new prognostic immune-inflammatory-nutritional score for predicting outcomes after curative resection for intrahepatic cholangiocarcinoma: a multicenter study. Front Immunol 2023;14:1165510.
82. Yoh T, Hatano E, Kasai Y, et al. Serum nardilysin, a surrogate marker for epithelial-mesenchymal transition, predicts prognosis of intrahepatic cholangiocarcinoma after surgical resection. Clin Cancer Res 2019;25:619-28.
83. Jiang S, Tang W, Huang B. Longitudinal change of circulating tumour cell count and its relation to prognosis in advanced intrahepatic cholangiocarcinoma patients. Scand J Clin Lab Invest 2023;83:234-40.
84. Zhu AX, Borger DR, Kim Y, et al. Genomic profiling of intrahepatic cholangiocarcinoma: refining prognosis and identifying therapeutic targets. Ann Surg Oncol 2014;21:3827-34.
85. Andraus W, Tustumi F, de Meira Junior JD, et al. Molecular profile of intrahepatic cholangiocarcinoma. Int J Mol Sci 2023;25:461.
86. Weinberg BA, Xiu J, Lindberg MR, et al. Molecular profiling of biliary cancers reveals distinct molecular alterations and potential therapeutic targets. J Gastrointest Oncol 2019;10:652-62.
87. Tomczak A, Springfeld C, Dill MT, et al. Precision oncology for intrahepatic cholangiocarcinoma in clinical practice. Br J Cancer 2022;127:1701-8.
88. Robertson S, Hyder O, Dodson R, et al. The frequency of KRAS and BRAF mutations in intrahepatic cholangiocarcinomas and their correlation with clinical outcome. Hum Pathol 2013;44:2768-73.
89. Peng J, Fang S, Li M, et al. Genetic alterations of KRAS and TP53 in intrahepatic cholangiocarcinoma associated with poor prognosis. Open Life Sci 2023;18:20220652.
90. Guo C, Liu Z, Yu Y, et al. TP53/KRAS Co-mutations create divergent prognosis signatures in intrahepatic cholangiocarcinoma. Front Genet 2022;13:844800.
91. Xin HY, Sun RQ, Zou JX, et al. Association of BRAF variants with disease characteristics, prognosis, and targeted therapy response in intrahepatic cholangiocarcinoma. JAMA Netw Open 2023;6:e231476.
92. Subbiah V, Lassen U, Gasal E, Burgess P, Wainberg ZA. Dabrafenib plus trametinib in patients with BRAF(V600E)-mutated biliary tract cancer - Authors’ reply. Lancet Oncol 2020;21:e516.
93. Boerner T, Drill E, Pak LM, et al. Genetic determinants of outcome in intrahepatic cholangiocarcinoma. Hepatology 2021;74:1429-44.
94. Toshida K, Itoh S, Yugawa K, et al. Prognostic significance for recurrence of fibroblast growth factor receptor 2 in intrahepatic cholangiocarcinoma patients undergoing curative hepatic resection. Hepatol Res 2023;53:432-9.
95. Abou-Alfa GK, Bibeau K, Schultz N, et al. Effect of FGFR2 alterations on overall and progression-free survival in patients receiving systemic therapy for intrahepatic cholangiocarcinoma. Target Oncol 2022;17:517-27.
96. Bekaii-Saab TS, Valle JW, Van Cutsem E, et al. FIGHT-302: first-line pemigatinib vs gemcitabine plus cisplatin for advanced cholangiocarcinoma with FGFR2 rearrangements. Future Oncol 2020;16:2385-99.
97. Chen L, He Y, Han Z, et al. The impact of decreased expression of SVEP1 on abnormal neovascularization and poor prognosis in patients with intrahepatic cholangiocarcinoma. Front Genet 2022;13:1127753.
98. Tang Z, Yang Y, Zhang Q, Liang T. Epigenetic dysregulation-mediated COL12A1 upregulation predicts worse outcome in intrahepatic cholangiocarcinoma patients. Clin Epigenetics 2023;15:13.
99. Wu L, Yang J, Ke RS, et al. Impact of lncRNA SOX9-AS1 overexpression on the prognosis and progression of intrahepatic cholangiocarcinoma. Clin Res Hepatol Gastroenterol 2022;46:101999.
100. Bi C, Liu M, Rong W, et al. High Beclin-1 and ARID1A expression corelates with poor survival and high recurrence in intrahepatic cholangiocarcinoma: a histopathological retrospective study. BMC Cancer 2019;19:213.
101. Yao X, Chen B, Wang M, et al. Exploration and validation of a novel ferroptosis-related gene signature predicting the prognosis of intrahepatic cholangiocarcinoma. Acta Biochim Biophys Sin 2022;54:1376-85.
102. Sarcognato S, Sacchi D, Fabris L, et al. Ferroptosis in intrahepatic cholangiocarcinoma: IDH1(105GGT) single nucleotide polymorphism is associated with its activation and better prognosis. Front Med 2022;9:886229.
103. Ren H, Liu C, Zhang C, et al. A cuproptosis-related gene expression signature predicting clinical prognosis and immune responses in intrahepatic cholangiocarcinoma detected by single-cell RNA sequence analysis. Cancer Cell Int 2024;24:92.
104. Marabelle A, Le DT, Ascierto PA, et al. Efficacy of pembrolizumab in patients with noncolorectal high microsatellite instability/mismatch repair-deficient cancer: results from the phase II keynote-158 study. J Clin Oncol 2020;38:1-10.
105. Song JP, Liu XZ, Chen Q, Liu YF. High tumor mutation burden indicates a poor prognosis in patients with intrahepatic cholangiocarcinoma. World J Clin Cases 2022;10:790-801.
106. Deng M, Li SH, Fu X, et al. Relationship between PD-L1 expression, CD8+ T-cell infiltration and prognosis in intrahepatic cholangiocarcinoma patients. Cancer Cell Int 2021;21:371.
107. Tian L, Ma J, Ma L, et al. PD-1/PD-L1 expression profiles within intrahepatic cholangiocarcinoma predict clinical outcome. World J Surg Oncol 2020;18:303.
108. Dong Z, Liao B, Shen W, Sui C, Yang J. Expression of programmed death ligand 1 is associated with the prognosis of intrahepatic cholangiocarcinoma. Dig Dis Sci 2020;65:480-8.
109. Zhu Y, Wang XY, Zhang Y, et al. Programmed death ligand 1 expression in human intrahepatic cholangiocarcinoma and its association with prognosis and CD8+ T-cell immune responses. Cancer Manag Res 2018;10:4113-23.
110. Cheng R, Chen Y, Zhou H, Wang B, Du Q, Chen Y. B7-H3 expression and its correlation with clinicopathologic features, angiogenesis, and prognosis in intrahepatic cholangiocarcinoma. J Pathol Microbiol Immunol 2018;126:396-402.
111. Miyazaki K, Morine Y, Imura S, et al. Preoperative lymphocyte/C-reactive protein ratio and its correlation with CD8+ tumor-infiltrating lymphocytes as a predictor of prognosis after resection of intrahepatic cholangiocarcinoma. Surg Today 2021;51:1985-95.
112. Yang H, Yan M, Li W, Xu L. SIRPα and PD1 expression on tumor-associated macrophage predict prognosis of intrahepatic cholangiocarcinoma. J Transl Med 2022;20:140.
113. Xu L, Yan M, Long J, Liu M, Yang H, Li W. Identification of macrophage correlated biomarkers to predict the prognosis in patients with intrahepatic cholangiocarcinoma. Front Oncol 2022;12:967982.
114. Sun D, Luo T, Dong P, et al. CD86+/CD206+ tumor-associated macrophages predict prognosis of patients with intrahepatic cholangiocarcinoma. PeerJ 2020;8:e8458.
115. Oishi K, Sakaguchi T, Baba S, Suzuki S, Konno H. Macrophage density and macrophage colony-stimulating factor expression predict the postoperative prognosis in patients with intrahepatic cholangiocarcinoma. Surg Today 2015;45:715-22.
116. Xu Y, Li Z, Zhou Y, et al. Using immunovascular characteristics to predict very early recurrence and prognosis of resectable intrahepatic cholangiocarcinoma. BMC Cancer 2023;23:1009.
117. Ding GY, Ma JQ, Yun JP, et al. Distribution and density of tertiary lymphoid structures predict clinical outcome in intrahepatic cholangiocarcinoma. J Hepatol 2022;76:608-18.
118. Doi N, Ino Y, Fuse M, Esaki M, Shimada K, Hiraoka N. Correlation of vein-rich tumor microenvironment of intrahepatic cholangiocarcinoma with tertiary lymphoid structures and patient outcome. Mod Pathol 2024;37:100401.
119. Hu ZQ, Zhou ZJ, Luo CB, et al. Peritumoral plasmacytoid dendritic cells predict a poor prognosis for intrahepatic cholangiocarcinoma after curative resection. Cancer Cell Int 2020;20:582.
120. Fukuda Y, Asaoka T, Eguchi H, et al. Endogenous CXCL9 affects prognosis by regulating tumor-infiltrating natural killer cells in intrahepatic cholangiocarcinoma. Cancer Sci 2020;111:323-33.
121. Zhu C, Ma J, Zhu K, et al. Spatial immunophenotypes predict clinical outcome in intrahepatic cholangiocarcinoma. JHEP Rep 2023;5:100762.
122. Thongchot S, Vidoni C, Ferraresi A, et al. Dihydroartemisinin induces apoptosis and autophagy-dependent cell death in cholangiocarcinoma through a DAPK1-BECLIN1 pathway. Mol Carcinog 2018;57:1735-50.
123. Thongchot S, Vidoni C, Ferraresi A, et al. Cancer-associated fibroblast-derived IL-6 determines unfavorable prognosis in cholangiocarcinoma by affecting autophagy-associated chemoresponse. Cancers 2021;13:2134.
124. Thongchot S, Ferraresi A, Vidoni C, et al. Preclinical evidence for preventive and curative effects of resveratrol on xenograft cholangiocarcinogenesis. Cancer Lett 2024;582:216589.
125. Liu Z, Weng S, Xu H, et al. Computational recognition and clinical verification of TGF-β-derived mirna signature with potential implications in prognosis and immunotherapy of intrahepatic cholangiocarcinoma. Front Oncol 2021;11:757919.
126. Zeng TM, Pan YF, Yuan ZG, Chen DS, Song YJ, Gao Y. Immune-related RNA signature predicts outcome of PD-1 inhibitor-combined GEMCIS therapy in advanced intrahepatic cholangiocarcinoma. Front Immunol 2022;13:943066.
127. Yue Y, Tao J, An D, Shi L. Exploring the role of tumor stemness and the potential of stemness-related risk model in the prognosis of intrahepatic cholangiocarcinoma. Front Genet 2022;13:1089405.
128. Tian MX, Zhou YF, Qu WF, et al. Histopathology-based immunoscore predicts recurrence for intrahepatic cholangiocarcinoma after hepatectomy. Cancer Immunol Immun 2019;68:1369-78.
129. Zhang LT, Yang YF, Chen XM, Wang SB, Tong GL. IL23R as an indicator of immune infiltration and poor prognosis in intrahepatic cholangiocarcinoma: a bioinformatics analysis. Transl Cancer Res 2023;12:2461-76.
130. Liang J, Zhou H, Huang XQ, et al. A myeloid signature-based nomogram predicts the postoperative recurrence of intrahepatic cholangiocarcinoma. Front Mol Biosci 2021;8:742953.
131. Hwang JA, Lee S, Lee JE, Yoon J, Choi SY, Shin J. LI-RADS Category on MRI is associated with recurrence of intrahepatic cholangiocarcinoma after surgery: a multicenter study. J Magn Reson Imaging 2023;57:930-8.
132. Nishioka E, Tsurusaki M, Kozuki R, et al. Comparison of conventional imaging and 18F-fluorodeoxyglucose positron emission tomography/computed tomography in the diagnostic accuracy of staging in patients with intrahepatic cholangiocarcinoma. Diagnostics 2022;12:2889.
133. Lin Y, Chong H, Song G, et al. The influence of 18F-fluorodeoxyglucose positron emission tomography/computed tomography on the N- and M-staging and subsequent clinical management of intrahepatic cholangiocarcinoma. Hepatobiliary Surg Nutr 2022;11:684-95.
134. Ikeno Y, Seo S, Iwaisako K, et al. Preoperative metabolic tumor volume of intrahepatic cholangiocarcinoma measured by 18F-FDG-PET is associated with the KRAS mutation status and prognosis. J Transl Med 2018;16:95.
135. Brunese MC, Fantozzi MR, Fusco R, et al. Update on the applications of radiomics in diagnosis, staging, and recurrence of intrahepatic cholangiocarcinoma. Diagnostics 2023;13:1488.
136. Wakiya T, Ishido K, Kimura N, et al. CT-based deep learning enables early postoperative recurrence prediction for intrahepatic cholangiocarcinoma. Sci Rep 2022;12:8428.
137. Chen B, Mao Y, Li J, et al. Predicting very early recurrence in intrahepatic cholangiocarcinoma after curative hepatectomy using machine learning radiomics based on CECT: a multi-institutional study. Comput Biol Med 2023;167:107612.
138. Bo Z, Chen B, Yang Y, et al. Machine learning radiomics to predict the early recurrence of intrahepatic cholangiocarcinoma after curative resection: a multicentre cohort study. Eur J Nucl Med Mol Imaging 2023;50:2501-13.
139. Jolissaint JS, Wang T, Soares KC, et al. Machine learning radiomics can predict early liver recurrence after resection of intrahepatic cholangiocarcinoma. HPB 2022;24:1341-50.
140. Hao X, Liu B, Hu X, et al. A radiomics-based approach for predicting early recurrence in intrahepatic cholangiocarcinoma after surgical resection: a multicenter study. Annu Int Conf IEEE Eng Med Biol Soc 2021;2021:3659-62.
141. Zhang J, Wu Z, Zhang X, et al. Machine learning: an approach to preoperatively predict PD-1/PD-L1 expression and outcome in intrahepatic cholangiocarcinoma using MRI biomarkers. ESMO Open 2020;5:e000910.
142. Xu Y, Li Z, Yang Y, et al. A CT-based radiomics approach to predict intra-tumoral tertiary lymphoid structures and recurrence of intrahepatic cholangiocarcinoma. Insights Imaging 2023;14:173.
143. Wang J, Huang M, Shen J, et al. Development and external validation of a prognosis model to predict outcomes after curative resection of early-stage intrahepatic cholangiocarcinoma. Front Surg 2023;10:1102871.
144. Li Q, Zhang J, Chen C, et al. A nomogram model to predict early recurrence of patients with intrahepatic cholangiocarcinoma for adjuvant chemotherapy guidance: a multi-institutional analysis. Front Oncol 2022;12:896764.
145. Tsilimigras DI, Endo Y, Guglielmi A, et al. Recurrent intrahepatic cholangiocarcinoma: a 10-point score to predict post-recurrence survival and guide treatment of recurrence. Ann Surg Oncol 2024;31:4427-35.
146. Nassar A, Tzedakis S, Sindayigaya R, et al. Factors of early recurrence after resection for intrahepatic cholangiocarcinoma. World J Surg 2022;46:2459-67.
147. Choi WJ, Williams PJ, Claasen MPAW, et al. Systematic review and meta-analysis of prognostic factors for early recurrence in intrahepatic cholangiocarcinoma after curative-intent resection. Ann Surg Oncol ;2022:4337-53.
148. Zhang XF, Beal EW, Bagante F, et al. Early versus late recurrence of intrahepatic cholangiocarcinoma after resection with curative intent. Br J Surg 2018;105:848-56.
149. Akaoka M, Haruki K, Furukawa K, et al. Analysis of preoperative risk factors for early recurrence in patients after hepatic resection for intrahepatic cholangiocarcinoma. Am Surg 2024;90:1148-55.
150. Li H, Liu R, Qiu H, et al. Tumor burden score stratifies prognosis of patients with intrahepatic cholangiocarcinoma after hepatic resection: a retrospective, multi-institutional study. Front Oncol 2022;12:829407.
151. Tsilimigras DI, Moris D, Hyer JM, et al. Hepatocellular carcinoma tumour burden score to stratify prognosis after resection. Br J Surg 2020;107:854-64.
152. Lv Y, Liu H, He P, et al. A novel model for predicting the prognosis of postoperative intrahepatic cholangiocarcinoma patients. Sci Rep 2023;13:19267.
153. Alaimo L, Moazzam Z, Endo Y, et al. Long-term recurrence-free and overall survival differ based on common, proliferative, and inflammatory subtypes after resection of intrahepatic cholangiocarcinoma. Ann Surg Oncol 2023;30:1392-403.
154. Alaimo L, Moazzam Z, Brown ZJ, et al. Application of hazard function to investigate recurrence of intrahepatic cholangiocarcinoma after curative-intent liver resection: a novel approach to characterize recurrence. Ann Surg Oncol 2023;30:1340-9.
155. Moro A, Paredes AZ, Farooq A, et al. Discordance in prediction of prognosis among patients with intrahepatic cholangiocarcinoma: a preoperative vs postoperative perspective. J Surg Oncol 2019;120:946-55.
156. Alaimo L, Lima HA, Moazzam Z, et al. Development and validation of a machine-learning model to predict early recurrence of intrahepatic cholangiocarcinoma. Ann Surg Oncol 2023;30:5406-15.
157. Buettner S, Galjart B, van Vugt JLA, et al. Performance of prognostic scores and staging systems in predicting long-term survival outcomes after surgery for intrahepatic cholangiocarcinoma. J Surg Oncol 2017;116:1085-95.
158. Alaimo L, Pawlik TM. ASO author reflections: use of machine learning to predict early recurrence after resection of intrahepatic cholangiocarcinoma. Ann Surg Oncol 2023;30:5416-7.
159. Bagante F, Spolverato G, Cucchetti A, et al. Defining when to offer operative treatment for intrahepatic cholangiocarcinoma: a regret-based decision curves analysis. Surgery 2016;160:106-17.
160. Wilson A, Winner M, Yahanda A, Andreatos N, Ronnekleiv-Kelly S, Pawlik TM. Factors associated with decisional regret among patients undergoing major thoracic and abdominal operations. Surgery 2017;161:1058-66.
161. Kodama K, Kawaoka T, Kosaka M, et al. Calcium channel blockers improve the prognosis of patients with intrahepatic cholangiocarcinoma after resection. J Gastroenterol 2022;57:676-83.
162. Yang Y, Lin M, Zhao H, Peng Y, Huang F, Lu Z. A survey of recent methods for addressing AI fairness and bias in biomedicine. J Biomed Inform 2024;154:104646.
Cite This Article
How to Cite
Chick, R. C.; Ruff S. M.; Pawlik T. M. Factors associated with prognosis and staging of intrahepatic cholangiocarcinoma. J. Cancer. Metastasis. Treat. 2024, 10, 22. http://dx.doi.org/10.20517/2394-4722.2024.47
Download Citation
Export Citation File:
Type of Import
Tips on Downloading Citation
Citation Manager File Format
Type of Import
Direct Import: When the Direct Import option is selected (the default state), a dialogue box will give you the option to Save or Open the downloaded citation data. Choosing Open will either launch your citation manager or give you a choice of applications with which to use the metadata. The Save option saves the file locally for later use.
Indirect Import: When the Indirect Import option is selected, the metadata is displayed and may be copied and pasted as needed.
About This Article
Copyright
Data & Comments
Data
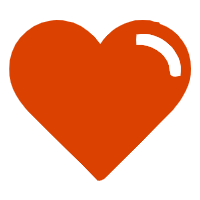
Comments
Comments must be written in English. Spam, offensive content, impersonation, and private information will not be permitted. If any comment is reported and identified as inappropriate content by OAE staff, the comment will be removed without notice. If you have any queries or need any help, please contact us at support@oaepublish.com.