Navigating immunotherapy for ovarian cancer: current landscape and future perspectives
Abstract
Ovarian cancer (OC) is the leading cause of death related to gynecologic malignancies, with recurrence occurring frequently despite significant advances in surgical interventions and chemotherapy. Therefore, novel therapies are necessary to improve the long-term prognosis of the disease. Immunotherapy holds promise in OC treatment by harnessing the potential of the immune system to combat neoplastic cells. The effectiveness of immunotherapy has been demonstrated in numerous cancers and subsequently integrated into clinical practice. However, despite initial preclinical findings suggesting an immunogenic microenvironment in OC, immune checkpoint inhibitors have not shown significant outcomes in clinical studies thus far. Further investigation is needed to fully understand the role of immunity in OC and to develop more effective therapeutic strategies, including combinatorial approaches and the identification of predictive biomarkers for more accurate patient selection for immunotherapy.
Keywords
INTRODUCTION
In the United States, approximately 22,000 patients receive an ovarian cancer (OC) diagnosis every year. This makes OC one of the most prevalent tumors among women and, specifically, the fifth most common cause of cancer-related fatalities among female individuals[1]. The focus of this manuscript is on epithelial OC, representing the most prevalent subtype, accounting for 90% of ovarian cancer cases. This decision is guided by the substantial clinical and pathological differences observed between epithelial and non-epithelial ovarian cancers[2]. The current protocol typically entails initial tumor reduction surgery succeeded by a regimen of platinum-based and taxane-based chemotherapy for ongoing management. After the initial treatment, cancer recurrence rates are high, affecting around 70% of patients with optimal debulking
Immune checkpoint inhibitors
One innovative approach to treating ovarian cancer (OC) is immune checkpoint inhibition, which targets molecules such as cytotoxic T-lymphocyte-associated protein 4 (CTLA-4) with its ligand CD80, and programmed death receptor-1 (PD-1) with its ligand PD-L1. These regulatory checkpoints play a crucial role in discerning between foreign pathogens and host cells. Upon interaction with a peripheral cell, a
ICI: monotherapy
Numerous clinical investigations have been undertaken in this context. For example, in a phase II study assessing nivolumab (an anti-PD-1 agent), the approximate best overall response rate (ORR) was 15%, with a disease control rate (DCR) of about 45%. Significantly, a sustained complete response rate was observed in patients undergoing treatment (UMIN Clinical Trials Registry UMIN000005714)[21]. In a very interesting phase II trial (KEYNOTE-100-NCT02674061), 376 OC patients were treated with pembrolizumab in a monotherapy regimen, yielding an ORR of solely 8%. It was then observed that higher expression of PD-L1, measured by a combined positive score (CPS) of 10 or more, led to an increased ORR (17.1%) compared to a CPS between 1 and less than 10 (10.2%) or less than 1 (5%)[22]. Overall, these trials demonstrated limited effectiveness of PD-1 blockade as a monotherapy in OC patients.
ICI: combination therapies
An avenue to improve the potency of PD-1 blockade involves combining it with other ICIs, which theoretically synergize with the immune system, thereby augmenting the clinical response. Some studies in the literature have shown that the association of PD-1/PD-L1 blockade and CTLA-4 blockade[23,24] is more effective as compared to PD-1/PD-L1 blockade alone in murine experimental systems of melanoma and OC. Specifically, the combination therapy of ipilimumab and nivolumab has demonstrated efficacy in treating metastatic melanoma and lung cancer, albeit with increased toxicity compared to using PD-1 blockade alone[25]. Consequently, researchers are exploring the potential of combining ipilimumab with nivolumab for the treatment of ovarian cancer patients[26]. In a randomized phase II trial conducted by Zamarin et al., which enrolled 100 OC patients, the combination of nivolumab and ipilimumab demonstrated a significantly higher response rate compared to the use of nivolumab alone[27]. One intriguing aspect is the rationale behind combining antiangiogenic agents with ICI, leveraging the capacity of antiangiogenics to improve T cell circulation into tumors[28]. Preclinical models have shown that inhibiting VEGF signaling can boost anti-neoplastic immunity and improve the capability of ICI[29], while combining anti-PD-L1 with anti-VEGF has demonstrated combined effects in vivo[30]. In a phase II clinical investigation involving 38 OC patients, the concurrent use of nivolumab (an anti-PD1) and bevacizumab (an anti-VEGF) was evaluated[31]. The combination achieved an overall response rate (ORR) of 28.9%, with differing rates between platinum-sensitive and platinum-resistant groups (40% vs. 16.7%). The median progression-free survival (PFS) was 9.4 months, with variations observed between sensitive and resistant patients (12.1 vs. 7.7 months). Notably, approximately 90% of participants experienced grade 3 or higher adverse events associated with the treatment. In a placebo-controlled randomized phase III trial conducted by Pignata et al., involving 1,300 individuals diagnosed with stage III-IV OC, the efficacy of atezolizumab in combination with standard carboplatin-paclitaxel-bevacizumab (experimental arm) was compared to placebo and standard treatment (control arm)[32]. The results indicated that the experimental arm did not demonstrate any prognostic advantage over the control arm in terms of PFS. However, another study highlighted a favorable trend toward atezolizumab in a subset of patients with higher PD-L1 expression. Furthermore, it has been shown that the combination of ICIs with PARP inhibitors could represent a promising strategy, as tumors with anomalies in DNA repair mechanisms (specifically, homologous recombination deficiency - HRD) may evade immune control[33] [Table 1]. PARP inhibitors can induce DNA damage that may stimulate an effective immune response and restore the TME[34]. Indeed, various experiments conducted in animal models have already suggested that the combination of PARP inhibitors and ICIs can be particularly effective and ensure a more favorable prognosis for OC patients[35-37]. In Table 2, we have listed all the ongoing phase III clinical trials investigating the efficacy of ICIs in combination with PARP inhibitors and/or antiangiogenic agents in OC patients, either when combined with chemotherapy or used as maintenance therapy.
Efficacy and toxicity of ICIs in both monotherapy and combination therapies
First author, year | Drugs | Study phase | No. of patients | Efficacy | Response rate | Toxicity* |
Hamanishi et al., 2015[21] | Nivolumab | II | 20 | Median PFS: 3.5 months (95%CI: 1.7 to 3.9 months); median OS: 20.0 months (95%CI: 7.0 months to not reached) | Two patients with durable complete responses (in the Best ORR 15.0% DCR: 45.0% | Grade 3/4 TRAEs: 40% (lymphocytopenia, anemia, albumin decreased, maculopapular rash, thyroiditis-induced fever and tachycardia) |
Nishio et al., 2020[22] | Pembrolizumab | II | 376 | NR | ORR: 8.0% (95%CI: 5.4-11.2) | All grades TRAEs: 61.9% (hyperthyroidism, hypothyroidism) Grade > 3: 23.8% (severe skin toxicity, nephritis) |
Zamarin et al., 2020[27] | Nivolumab vs. Nivolumab and Ipilimumab | II | 100 | Median PFS nivolumab group: 2 months, nivolumab plus ipilimumab group 3.9 months; HR for PFS (nivolumab plus ipilimumab vs. nivolumab alone): 0.53 (95%CI: 0.34- 0.82) | ORR within 6 months: nivolumab group: 12.2% nivolumab plus ipilimumab group: 31.4% | Grade ≥ 3 TRAEs: nivolumab group: 33% (pancreatic enzyme elevation) nivolumab plus ipilimumab group: 49% (pancreatitis, anemia, thrombocytopenia, acute kidney injury) No treatment-related deaths were reported |
Liu et al., 2019[31] | Nivolumab and Bevacizumab | II | 38 | Median PFS: 8.1 months (95%CI: 6.3-14.7 months) | ORR: 28.9% (95% exact binomial CI: 15.4%-45.9%) in platinum-sensitive participants: 40.0% (95%CI: 19.1%-64.0%) in platinum-resistant participants: 16.7% (95%CI: 3.6%-41.4%) | All grades TRAEs: 89.5% Grade > 3: 23.7% (serum lipase level increases) |
Pignata et al., 2023[32] | Atezolizumab vs. Placebo Both with Paclitaxel, Carboplatin, Bevacizumab | III | 1,301 | HR for OS in PD-L1-positive population: 0.83 (95%CI: HR for OS in ITT population: 0.92 (95%CI: 0.78-1.09), median OS: atezolizumab 50.5 months; placebo 46.6 months | Not indicated | Not indicated |
Kurtz et al., 2023[33] | Atezolizumab vs. Placebo Both with Platinum-based chemotherapy | III | 614 | HR for investigator-assessed PFS in the ITT population: 0.83 (95%CI: 0.69-0.99); median PFS: atezolizumab 13.5 months, placebo 11.3 months | Not indicated | Grade ≥ 3 TRAEs: atezolizumab-treated patients 13% (hypothyroidism, hyperthyroidism, hepatitis or transaminitis, colitis or severe diarrhea) placebo-treated patients 8% (hematologic) |
Ongoing phase III clinical trials of immunotherapy combined with other drugs in OC
Study, NCT | No. of patients | Treatment | Primary outcome |
AGO DUO NCT03737643 | 1,407 | Platinum-based chemotherapy plus bevacizumab ± durvalumab followed by maintenance bevacizumab ± durvalumab ± olaparib | PFS |
ATHENA NCT03522246 | 1,000 | Platinum-based chemotherapy followed by maintenance ± rucaparib in combination with ± nivolumab | PFS |
FIRST NCT03602859 | 1,402 | Carboplatin+paclitaxel plus bevacizumab in combination with | PFS |
ENGOT OV 43 NCT03740165 | 1,367 | Paclitaxel/carboplatin in combination with ± bevacizumab (investigator choice) ± pembrolizumab followed by maintenance ± bevacizumab ± pembrolizumab | PFS OS |
NRG-GY009 NCT02839707 | 443 | Pegylated liposomal doxorubicin hydrochlorid ± bevacizumab ± atezolizumab | DLT PFS OS |
ANITA NCT03598270 | 414 | Platinum-based chemotherapy ± atezolizumab followed by maintenance niraparib ± atezolizumab | PFS |
AGO-OVAR 2.29 NCT03353831 | 550 | Platinum-based chemotherapy plus bevacizumab in combination | OS PFS |
ATALANTE NCT02891824 | 614 | Platinum-based chemotherapy plus bevacizumab in combination with ± atezolizumab followed by maintenance bevacizumab ± atezolizumab | PFS |
KEYNOTE-B96/ENGOT-ov65 NCT05116189 | 616 | Pembrolizumab + paclitaxel ± bevacizumab and placebo comparator: placebo + paclitaxel ± bevacizumab | PFS |
NCT03914612 | 759 | Active comparator: arm I placebo, paclitaxel, carboplatin - Experimental: Arm II pembrolizumab, paclitaxel, carboplatin | PFS |
Vaccines
Numerous tumor-related antigens have been recognized in OC, including sialyl-Tn, NY-ESO-1 (also called Cancer testis antigen 1, or CTAG1B), mucin antigen 1, EGFR2/neu, amino enhancer of split protein, mesothelin, p53, mucin antigen 16/cancer antigen, folate-binding protein, human telomerase reverse transcriptase, and surviving[38-43]. These antigens could be valuable focal points for tumor vaccine development, but the molecular heterogeneity of the tumor has complicated the selection of an appropriate compound capable of eliciting a robust immunological response against tumors[44]. For instance, NY-ESO-1 emerges as one of the most intriguing targets due to its absence in healthy tissues beyond the gonad and its presence being detected in approximately 40% of tumors in a sample of 1,000 OC patients[45]. Several clinical studies have been carried out to examine the efficacy of NY-ESO-1-based vaccines, demonstrating their ability to extend patients’ overall survival no later than 2 years[46,47]. Nonetheless, doubts arise regarding the enduring effectiveness of these vaccines, which aim at a self-antigen that is wrongly expressed by cancerous cells. This skepticism arises from tumors’ inclination to develop escape strategies, such as losing antigens through immunoediting, thus avoiding detection by the immune system[48]. Consequently, further vaccine developments are necessary to overcome these evasion mechanisms. In particular, neoantigens emerge from DNA mutations in tumor cells and serve as a promising target for cancer vaccines because they are recognized as foreign by the immune system. Somatic mutations, which may be truncal or clonal, generate neoantigens expressed only by tumors. These antigens can trigger a robust and specific immune response against the tumor, contrasting with the weak immune response against normal body antigens[49]. Tumors with a high mutation load appear to respond better to immunotherapies, suggesting that a greater quantity of neoantigens may boost the immune system’s response against the tumor[50]. However, the private nature of neoantigens complicates the development of universal vaccines[51]. Furthermore, while it was previously believed that ovarian tumors lacked a sufficient mutation load for neoantigen-based therapy, a study revealed the presence of numerous neoantigens and specific T cells in OC patients[52]. Autologous vaccines might have a pivotal role in the advancement of cancer immunotherapy[53]. An exemplification of this strategy is autologous vaccines, engineered to enhance the immune response against tumor cells while concurrently counteracting their immune evasion mechanisms. A preliminary study assessed the efficacy of this association in six patients with advanced metastatic OC resistant to chemotherapy. The combined therapy included Ipilimumab, followed by surgical intervention and the infusion of TILs that have been multiplied outside the body before, along with IL-2 and Nivolumab. The outcomes were encouraging, as evidenced by one patient achieving a partial response and five patients achieving disease stabilization at the 12-month mark. These outcomes were compared to those obtained without Ipilimumab, demonstrating an increase in the efficacy of ex vivo expanded TILs, particularly an enhancement in CD8+ T cell activity[54]. This study underscores the potential benefits of combining personalized vaccines with ICI therapies. A different individualized immunogen, Vigil, aims to instruct T cells in identifying tumor-specific clonal neoantigens, representing a logical advancement. A phase I clinical trial assessed the association of Atezolizumab and Vigil in patients with recurrent OC. The optimal timing of administration emerged as crucial, as the findings not only demonstrate promising efficacy but also underscore the treatment’s safety profile. In particular, Vigil was administered first, followed by Atezolizumab. This sequence of administration was correlated with increased efficacy while also resulting in the advantage of causing fewer side effects. Median progression-free survival remained indeterminate in the Vigil-treated cohort and reached 10.8 months in the Atezolizumab-treated group (hazard ratio 0.33). These outcomes parallel prior research on Vigil, indicating potential enhanced clinical utility, particularly among BRCA wild-type patients[55]. The observed safety profile and clinical efficacy in this limited patient population underscore the necessity for additional investigation.
Oncolytic viruses
The approach with oncolytic viruses (OVs) represents an innovative perspective in the field of cancer therapy. These viruses are designed to specifically target tumor cells, sparing healthy ones, and can be genetically modified to enhance their efficacy in combating cancer[56]. Furthermore, the use of oncolytic viruses offers potential advantages over conventional therapies such as chemotherapy and radiotherapy, which can cause damage to surrounding healthy tissues and significant side effects[57]. On the other hand, OVs can be engineered to be highly selective in destroying tumor cells, while minimizing damage to healthy tissues. The combination of OVs with immunotherapy, such as ICIs, represents a promising area of research[58]. The synergistic effect of these two approaches can improve the immune response against cancer, thereby enhancing treatment efficacy. Additionally, ongoing preclinical and clinical studies are exploring further administration methods of OVs and new viral engineering strategies to optimize their antitumor activity and reduce the likelihood of developing resistance. In addition to OVs, other experimental treatments for OC include various viruses such as the reovirus, adenovirus, and others[59]. Some of these have shown antitumor activity in phase I and II studies. For example, it has been demonstrated that a herpes simplex virus engineered to express interleukin-12 (IL-12) effectively eradicates both murine and human ovarian cancer cell lines. This treatment also regulates ovarian cancer metastases and enhances survival rates in murine models when administered directly into the omentum and peritoneal cavity[60]. The concurrent application of intratumoral Newcastle disease virus (NDV) therapy alongside anti-CTLA-4 blockade has demonstrated therapeutic efficacy in preclinical animal models. This is substantiated by observable tumor regression and enhanced survival rates[61]. Nevertheless, notwithstanding these encouraging advancements, additional clinical investigations are imperative to elucidate the precise role of OVs in OC treatment and to warrant their regulatory approval.
Adoptive T cell therapy
An alternative cellular-based strategy involves the application of Adoptive Cell Therapy (ACT) employing TILs, known as TIL-ACT[62]. This individualized immunotherapeutic approach entails harvesting autologous TILs, expanding them ex vivo, and subsequently reintroducing them into the patient alongside IL-2 in high dosage to augment the in vivo T cell-mediated antitumor response. Prior to TIL-ACT administration, a nonmyeloablative lymphodepleting chemotherapy regimen is administered, inducing transient lymphopenia and leukopenia, crucial for ensuring successful engraftment of the transferred
CAR-T cell therapies
CAR-T cell therapies utilizing genetically engineered T cells expressing redirected TCRs or chimeric antigen receptors (CARs) have garnered significant attention. Specifically, CAR-T cells are patient-derived leukocytes genetically engineered to recognize surface antigens on tumor cells and initiate a targeted immune response[67]. Although CAR-T cell therapy has advanced in recent years and shown effectiveness in hematologic malignancies, comparable results have not been observed in solid tumors. The primary challenge lies in identifying tumor-specific antigens that are overexpressed while avoiding damage to healthy tissues[68]. In the realm of CAR-T therapy for OC, prevalent targets include mucin-16 (MUC16), folate receptor-α (FRα), and human epidermal growth factor receptor 2 (HER2)[69,70]. Noteworthy findings from Chekmasova et al. affirm the capacity of MUC16 CAR-T cells to significantly impede tumor progression in murine models, offering promising insights into potential therapeutic avenues[71]. FRα protein exhibits minimal expression in normal cells, particularly in OC[72]. Utilizing CAR technology to target folate receptor 1 (FOLR1, also known as FRα) has been explored as a potential strategy for OC therapy. Folate receptor 1 (FOLR1, also known as FRα) is identified as a promising target for cancer therapies due to its abnormal expression in various epithelial tumors, including OC, and its minimal expression in healthy tissues. FRα is inaccessible in normal tissues and remains unaffected by chemotherapy. In a preclinical study, high FRα expression was confirmed in primary OC samples, and CAR-T cells were engineered using plasmids encoding humanized single-chain variable fragments from clinical antibodies MORAb-003 and M9436A specific to FRα. These CAR-T cells demonstrated significant efficacy both
FUTURE PERSPECTIVES
The array of immunotherapeutic options presents intriguing prospects for managing advanced recurrent OC. Specifically, Figure 1 illustrates the latest approaches to immunotherapy in treating OC, as previously discussed.
With the ongoing expansion in drug choices and combinations, there is a growing need for conceptual frameworks and rational study designs to hasten clinical progress[77]. While much headway has been made in extending survival for recurrent OC, recent successes in immunotherapy foster optimism for discovering curative treatments. Priority in clinical development should be given to frontline scenarios, where the greatest benefits are anticipated.
The emergence of PARP inhibitors, coupled with promising evidence of their synergy with ICIs in tumors with homologous recombination deficiency (HRD), underscores the potential for personalized therapy and a significant impact in frontline care. In HRD cells, PARP inhibitors block the repair of single-strand DNA breaks, leading to the accumulation of double-strand breaks that cannot be efficiently repaired. This results in synthetic lethality due to the dual impairment of DNA repair pathways. Furthermore, this genomic instability increases the load of tumor neoantigens, enhancing the potential efficacy of ICI. Investigating the combination of PARP inhibitors with PD-1/PD-L1 blockade as a frontline strategy for these patients represents a paradigm shift that could yield profound and enduring responses, potentially replacing current chemotherapy regimens. Moreover, evidence indicating that hypoxia downregulates BRCA1 and RAD51 expression, consequently affecting the HR pathway and sensitizing hypoxic cancer cells to PARP inhibitors, suggests that antiangiogenic drugs could augment the efficacy of PARP inhibitors via contextual synthetic lethality[78-81]. This may enhance the response of tumors lacking BRCA mutations to PARP inhibitors, thereby providing a rationale for combining antiangiogenic drugs, PARP inhibitors, and ICIs in this clinical setting[82,83]. Considering the synergistic potential of both antiangiogenic agents and PARP inhibitors with ICB, exploring such combinations could yield significant advances in OC cases with proficient homologous recombination, warranting investigation in frontline settings if confirmed. Consolidation strategies have long been explored in OC due to the high rate of disease recurrence following initial treatments. Immunotherapy holds promise in augmenting both the rate of curative response and the survival duration for patients who retain residual disease following initial treatment. Combining vaccines, particularly those directed against neoepitopes, with immunomodulatory agents like low-dose cyclophosphamide or ICB may offer low-toxicity treatment options. Additionally, for individuals enduring persistent disease subsequent to initial treatment, the application of ACT involving TILs or targeted T cells might emerge as a promising alternative, considering its distinctive method of administration. Beyond the aforementioned strategies, there exists a compelling rationale for investigating pharmaceutical agents directed against prevalent immunosuppressive factors in OC, whether employed singly or in combination. Immunomodulatory substances such as transforming growth factor-beta (TGF-β), IL-10, the adenosine pathway, indoleamine 2,3-dioxygenase-1 (IDO-1), and proinflammatory mediators like TNF-α, IL-6, M2 macrophages, myeloid-derived suppressor cells (MDSCs), and others represent promising targets for therapeutic innovation and development[84-86].
Finally, in this context, it is essential to emphasize the PI3K pathway as a novel frontier for innovative treatments in epithelial OC. This pathway is often upregulated in OC and plays a crucial role in chemoresistance and the maintenance of genomic stability, as it is implicated in various processes related to DNA replication and cell cycle regulation. Inhibiting the PI3K pathway could potentially induce genomic instability and mitotic catastrophe by reducing the activity of the spindle assembly checkpoint protein Aurora kinase B, thus promoting the occurrence of lagging chromosomes during prometaphase and enhancing the efficacy of ICI[87].
Clinical advancement should involve validating early-phase adaptive study methodologies, enabling the efficient evaluation and selection of drug candidates for combination therapies, alongside the integration of biomarkers. The latter requirement entails acquiring tumor biopsy samples, a procedure that might be partially substituted by employing molecular imaging methods and liquid biopsies. Additionally, concurrent clinical trials that assess the identical drug association in various therapeutic, neoadjuvant, and surrogate tumor environments should promptly provide essential insights to accelerate clinical advancement.
CONCLUSION
While the immune system’s role in OC pathogenesis is crucial, the translation of immunotherapy into clinical practice for this cancer type has predominantly remained confined to preliminary investigations. These investigations have highlighted OC’s immunogenicity and the potential of antitumor immunity activation as a viable therapeutic approach for a disease prone to recurrence. Initial endeavors explored cytokine treatment in OC, yet failed to provide compelling data from phase III trials. Conversely, ICI has emerged as a significant immunostimulant increasingly utilized in oncology, leveraging OC’s immunological attributes for therapeutic intervention. Nevertheless, ICI monotherapy has demonstrated modest efficacy in pre-treated ovarian cancer patients, prompting the exploration of combination therapies to improve outcomes. Consequently, various strategies have been developed to sensitize OC to immunotherapy through combination with chemotherapy, antiangiogenics, PARP inhibitors, radiotherapy, and dual ICI. A primary concern remains the identification of optimal prognostic markers to enhance candidate selection for ICI regimen.
A deeper understanding of underlying biological mechanisms, alongside ongoing technological advancements, is imperative to expand the scope of immune therapies and achieve meaningful advancements in clinical outcomes for OC patients.
DECLARATIONS
Authors’ contributions
Conceptualization, writing - original draft, methodology, supervision, validation: Capuozzo M
Conceptualization, writing - original draft: Ferrara F
Writing - original draft: Cinque C
Writing - review & editing: Farace S
Supervision, validation: Lauritano D
Conceptualization, writing - original draft: Ottaiano A
Availability of data and materials
Full availability of data and materials. All stated data can be provided to the reader upon request.
Financial support and sponsorship
None.
Conflicts of interest
All authors declared that there are no conflicts of interest.
Ethical approval and consent to participate
Not applicable.
Consent for publication
Not applicable.
Copyright
© The Author(s) 2024.
REFERENCES
2. Saani I, Raj N, Sood R, et al. Clinical challenges in the management of malignant ovarian germ cell tumours. Int J Environ Res Public Health 2023;20:6089.
3. Bailey M, Morand S, Royfman R, et al. Targeted combination of poly(ADP-ribose) polymerase inhibitors and immune checkpoint inhibitors lacking evidence of benefit: focus in ovarian cancer. Int J Mol Sci 2024;25:3173.
4. Gupta S, Nag S, Aggarwal S, Rauthan A, Warrier N. Maintenance therapy for recurrent epithelial ovarian cancer: current therapies and future perspectives - a review. J Ovarian Res 2019;12:103.
5. Secord A, O’Malley DM, Sood AK, Westin SN, Liu JF. Rationale for combination PARP inhibitor and antiangiogenic treatment in advanced epithelial ovarian cancer: a review. Gynecol Oncol 2021;162:482-95.
6. Kurnit KC, Coleman RL, Westin SN. Using PARP inhibitors in the treatment of patients with ovarian cancer. Curr Treat Options Oncol 2018;19:1.
7. Capuozzo M, Celotto V, Santorsola M, et al. Emerging treatment approaches for triple-negative breast cancer. Med Oncol 2023;41:5.
8. Capuozzo M, Ferrara F, Santorsola M, Zovi A, Ottaiano A. Circulating tumor cells as predictive and prognostic biomarkers in solid tumors. Cells 2023;12:2590.
9. Salmaninejad A, Valilou SF, Shabgah AG, et al. PD-1/PD-L1 pathway: basic biology and role in cancer immunotherapy. J Cell Physiol 2019;234:16824-37.
10. Akinleye A, Rasool Z. Immune checkpoint inhibitors of PD-L1 as cancer therapeutics. J Hematol Oncol 2019;12:92.
11. Wakabayashi G, Lee YC, Luh F, Kuo CN, Chang WC, Yen Y. Development and clinical applications of cancer immunotherapy against PD-1 signaling pathway. J Biomed Sci 2019;26:96.
12. Hamanishi J, Mandai M, Iwasaki M, et al. Programmed cell death 1 ligand 1 and tumor-infiltrating CD8+ T lymphocytes are prognostic factors of human ovarian cancer. Proc Natl Acad Sci USA 2007;104:3360-5.
13. Maine CJ, Aziz NH, Chatterjee J, et al. Programmed death ligand-1 over-expression correlates with malignancy and contributes to immune regulation in ovarian cancer. Cancer Immunol Immunother 2014;63:215-24.
14. Santoiemma PP, Powell DJ Jr. Tumor infiltrating lymphocytes in ovarian cancer. Cancer Biol Ther 2015;16:807-20.
15. Sun J, Yan C, Xu D, et al. Immuno-genomic characterisation of high-grade serous ovarian cancer reveals immune evasion mechanisms and identifies an immunological subtype with a favourable prognosis and improved therapeutic efficacy. Br J Cancer 2022;126:1570-80.
16. Wilkinson AN, Chen R, Coleborn E, et al. Let-7i enhances anti-tumour immunity and suppresses ovarian tumour growth. Cancer Immunol Immunother 2024;73:80.
17. Pugh-Toole M, Nicolela AP, Nersesian S, Leung BM, Boudreau JE. Natural killer cells: the missing link in effective treatment for high-grade serous ovarian carcinoma. Curr Treat Options Oncol 2022;23:210-26.
18. Janardhanan M, Smitha NV, Rajalakshmi G, George A, Koyakutty M, Iyer S. Tumour microenvironment as a potential immune therapeutic target for tongue cancer management. J Oral Maxillofac Pathol 2023;27:382-9.
19. Asadi M, Zarredar H, Zafari V, et al. Immune features of tumor microenvironment: a genetic spotlight. Cell Biochem Biophys 2024;82:107-18.
20. Das A, Ghose A, Naicker K, et al. Advances in adoptive T-cell therapy for metastatic melanoma. Curr Res Transl Med 2023;71:103404.
21. Hamanishi J, Mandai M, Ikeda T, et al. Safety and antitumor activity of anti-PD-1 antibody, nivolumab, in patients with platinum-resistant ovarian cancer. J Clin Oncol 2015;33:4015-22.
22. Nishio S, Matsumoto K, Takehara K, et al. Pembrolizumab monotherapy in Japanese patients with advanced ovarian cancer: subgroup analysis from the KEYNOTE-100. Cancer Sci 2020;111:1324-32.
23. Duraiswamy J, Kaluza KM, Freeman GJ, Coukos G. Dual blockade of PD-1 and CTLA-4 combined with tumor vaccine effectively restores T-cell rejection function in tumors. Cancer Res 2013;73:3591-603.
24. Duraiswamy J, Freeman GJ, Coukos G. Dual blockade of PD-1 and CTLA-4 combined with tumor vaccine effectively restores T-cell rejection function in tumors-response. Cancer Res 2014;74:633-4.
25. Chen J, Li S, Yao Q, et al. The efficacy and safety of combined immune checkpoint inhibitors (nivolumab plus ipilimumab): a systematic review and meta-analysis. World J Surg Oncol 2020;18:150.
26. Shoushtari AN, Friedman CF, Navid-Azarbaijani P, et al. Measuring toxic effects and time to treatment failure for nivolumab plus ipilimumab in melanoma. JAMA Oncol 2018;4:98-101.
27. Zamarin D, Burger RA, Sill MW, et al. Randomized phase II trial of nivolumab versus nivolumab and ipilimumab for recurrent or persistent ovarian cancer: an NRG oncology study. J Clin Oncol 2020;38:1814-23.
28. Wang H, Franco F, Ho PC. Metabolic regulation of tregs in cancer: opportunities for immunotherapy. Trends Cancer 2017;3:583-92.
29. Gao X, McDermott DF. Combinations of bevacizumab with immune checkpoint inhibitors in renal cell carcinoma. Cancer J 2018;24:171-9.
30. Chen Y, Li F, Li D, Liu W, Zhang L. Atezolizumab and blockade of LncRNA PVT1 attenuate cisplatin resistant ovarian cancer cells progression synergistically via JAK2/STAT3/PD-L1 pathway. Clin Immunol 2021;227:108728.
31. Liu JF, Herold C, Gray KP, et al. Assessment of combined nivolumab and bevacizumab in relapsed ovarian cancer: a phase 2 clinical trial. JAMA Oncol 2019;5:1731-8.
32. Pignata S, Bookman M, Sehouli J, et al. Overall survival and patient-reported outcome results from the placebo-controlled randomized phase III IMagyn050/GOG 3015/ENGOT-OV39 trial of atezolizumab for newly diagnosed stage III/IV ovarian cancer. Gynecol Oncol 2023;177:20-31.
33. Kurtz JE, Pujade-Lauraine E, Oaknin A, et al. Atezolizumab combined with bevacizumab and platinum-based therapy for platinum-sensitive ovarian cancer: placebo-controlled randomized phase III ATALANTE/ENGOT-ov29 trial. J Clin Oncol 2023;41:4768-78.
34. Higuchi T, Flies DB, Marjon NA, et al. CTLA-4 blockade synergizes therapeutically with PARP inhibition in BRCA1-deficient ovarian cancer. Cancer Immunol Res 2015;3:1257-68.
35. Lorusso D, Ceni V, Daniele G, et al. Immunotherapy in gynecological cancers. Explor Target Antitumor Ther 2021;2:48-64.
36. Stewart RA, Pilié PG, Yap TA. Development of PARP and immune-checkpoint inhibitor combinations. Cancer Res 2018;78:6717-25.
37. Lee EK, Konstantinopoulos PA. Combined PARP and Immune checkpoint inhibition in ovarian cancer. Trends Cancer 2019;5:524-8.
38. Murray JL, Przepiorka D, Ioannides CG. Clinical trials of HER-2/neu-specific vaccines. Semin Oncol 2000;27:71-5.
39. IeM, Kurman RJ. Ovarian tumorigenesis: a proposed model based on morphological and molecular genetic analysis. Am J Pathol 2004;164:1511-8.
40. Ding J, Zhang Y, Che Y. Ovarian cancer stem cells: critical roles in anti-tumor immunity. Front Genet 2022;13:998220.
42. Capuozzo M, Santorsola M, Bocchetti M, et al. p53: from fundamental biology to clinical applications in cancer. Biology 2022;11:1325.
43. Hilliard TS. The impact of mesothelin in the ovarian cancer tumor microenvironment. Cancers 2018;10:277.
44. Vandin F. Computational methods for characterizing cancer mutational heterogeneity. Front Genet 2017;8:83.
45. Szender JB, Papanicolau-Sengos A, Eng KH, et al. NY-ESO-1 expression predicts an aggressive phenotype of ovarian cancer. Gynecol Oncol 2017;145:420-5.
46. Pavlick A, Blazquez AB, Meseck M, et al. Combined vaccination with NY-ESO-1 protein, Poly-ICLC, and montanide improves humoral and cellular immune responses in patients with high-risk melanoma. Cancer Immunol Res 2020;8:70-80.
47. Wada H, Isobe M, Kakimi K, et al. Vaccination with NY-ESO-1 overlapping peptides mixed with Picibanil OK-432 and montanide ISA-51 in patients with cancers expressing the NY-ESO-1 antigen. J Immunother 2014;37:84-92.
48. Odunsi K, Qian F, Matsuzaki J, et al. Vaccination with an NY-ESO-1 peptide of HLA class I/II specificities induces integrated humoral and T cell responses in ovarian cancer. Proc Natl Acad Sci USA 2007;104:12837-42.
49. Srivastava PK. Cancer neoepitopes viewed through negative selection and peripheral tolerance: a new path to cancer vaccines. J Clin Invest 2024;134:e176740.
50. Zhang Z, Lu M, Qin Y, et al. Neoantigen: a new breakthrough in tumor immunotherapy. Front Immunol 2021;12:672356.
51. Tu SM, Trikannad AK, Vellanki S, et al. Stem cell origin of cancer: clinical implications beyond immunotherapy for drug versus therapy development in cancer care. Cancers 2024;16:1151.
52. Morisaki T, Hikichi T, Onishi H, et al. Intranodal administration of neoantigen peptide-loaded dendritic cell vaccine elicits epitope-specific T cell responses and clinical effects in a patient with chemorefractory ovarian cancer with malignant ascites. Immunol Invest 2021;50:562-79.
53. Yu R, Zhao F, Xu Z, Zhang G, Du B, Shu Q. Current status and future of cancer vaccines: a bibliographic study. Heliyon 2024;10:e24404.
54. Kverneland AH, Pedersen M, Westergaard MCW, et al. Adoptive cell therapy in combination with checkpoint inhibitors in ovarian cancer. Oncotarget 2020;11:2092-105.
55. Rocconi RP, Stevens EE, Bottsford-Miller JN, et al. Proof of principle study of sequential combination atezolizumab and Vigil in relapsed ovarian cancer. Cancer Gene Ther 2022;29:369-82.
56. Chen L, Ma Z, Xu C, et al. Progress in oncolytic viruses modified with nanomaterials for intravenous application. Cancer Biol Med 2023;20:830-55.
57. Feola S, Russo S, Ylösmäki E, Cerullo V. Oncolytic ImmunoViroTherapy: a long history of crosstalk between viruses and immune system for cancer treatment. Pharmacol Ther 2022;236:108103.
58. Oh CM, Chon HJ, Kim C. Combination immunotherapy using oncolytic virus for the treatment of advanced solid tumors. Int J Mol Sci 2020;21:7743.
59. Jin KT, Du WL, Liu YY, Lan HR, Si JX, Mou XZ. Oncolytic virotherapy in solid tumors: the challenges and achievements. Cancers 2021;13:588.
60. Hu H, Zhang S, Cai L, et al. A novel cocktail therapy based on quintuplet combination of oncolytic herpes simplex virus-2 vectors armed with interleukin-12, interleukin-15, GM-CSF, PD1v, and IL-7 × CCL19 results in enhanced antitumor efficacy. Virol J 2022;19:74.
61. Huang F, Dai C, Zhang Y, Zhao Y, Wang Y, Ru G. Development of molecular mechanisms and their application on oncolytic newcastle disease virus in cancer therapy. Front Mol Biosci 2022;9:889403.
62. Krishna S, Lowery FJ, Copeland AR, et al. Stem-like CD8 T cells mediate response of adoptive cell immunotherapy against human cancer. Science 2020;370:1328-34.
63. Dudley ME, Wunderlich JR, Yang JC, et al. A phase I study of nonmyeloablative chemotherapy and adoptive transfer of autologous tumor antigen-specific T lymphocytes in patients with metastatic melanoma. J Immunother 2002;25:243-51.
64. Albarrán V, San Román M, Pozas J, et al. Adoptive T cell therapy for solid tumors: current landscape and future challenges. Front Immunol 2024;15:1352805.
65. Pedersen M, Westergaard MCW, Milne K, et al. Adoptive cell therapy with tumor-infiltrating lymphocytes in patients with metastatic ovarian cancer: a pilot study. Oncoimmunology 2018;7:e1502905.
66. Verdegaal EME, Santegoets SJ, Welters MJP, et al. Timed adoptive T cell transfer during chemotherapy in patients with recurrent platinum-sensitive epithelial ovarian cancer. J Immunother Cancer 2023;11:e007697.
67. Marofi F, Motavalli R, Safonov VA, et al. CAR T cells in solid tumors: challenges and opportunities. Stem Cell Res Ther 2021;12:81.
68. Li YR, Halladay T, Yang L. Immune evasion in cell-based immunotherapy: unraveling challenges and novel strategies. J Biomed Sci 2024;31:5.
69. Klampatsa A, Dimou V, Albelda SM. Mesothelin-targeted CAR-T cell therapy for solid tumors. Expert Opin Biol Ther 2021;21:473-86.
70. Schoutrop E, El-Serafi I, Poiret T, et al. Mesothelin-specific CAR T cells target ovarian cancer. Cancer Res 2021;81:3022-35.
71. Chekmasova AA, Rao TD, Nikhamin Y, et al. Successful eradication of established peritoneal ovarian tumors in SCID-Beige mice following adoptive transfer of T cells genetically targeted to the MUC16 antigen. Clin Cancer Res 2010;16:3594-606.
72. Frigerio B, Montermini M, Silvana C, Figini M. Role of antibody engineering in generation of derivatives starting from MOv19 MAb: 40 years of biological/therapeutic tools against folate receptor alfa. Antib Ther 2022;5:301-10.
73. Daigre J, Martinez-Osuna M, Bethke M, et al. Preclinical evaluation of novel folate receptor 1-directed CAR T cells for ovarian cancer. Cancers 2024;16:333.
74. Zuo S, Wen Y, Panha H, et al. Modification of cytokine-induced killer cells with folate receptor alpha (FRα)-specific chimeric antigen receptors enhances their antitumor immunity toward FRα-positive ovarian cancers. Mol Immunol 2017;85:293-304.
75. Han C, McNamara B, Bellone S, et al. The Poly (ADP-ribose) polymerase inhibitor olaparib and pan-ErbB inhibitor neratinib are highly synergistic in HER2 overexpressing epithelial ovarian carcinoma in vitro and in vivo. Gynecol Oncol 2023;170:172-8.
76. Jiang D, Im HJ, Sun H, et al. Radiolabeled pertuzumab for imaging of human epidermal growth factor receptor 2 expression in ovarian cancer. Eur J Nucl Med Mol Imaging 2017;44:1296-305.
77. Ma LS, Yan QI, Huang Y, Zhao W, Zhu YU. Downregulation of human epidermal growth factor receptor 2 by short hairpin RNA increases chemosensitivity of human ovarian cancer cells. Oncol Lett 2015;9:2211-7.
78. Ferrara F, Zovi A, Capuozzo M, Langella R. Atopic dermatitis: treatment and innovations in immunotherapy. Inflammopharmacology 2024;32:1777-89.
79. Seifeddine R, Dreiem A, Blanc E, et al. Hypoxia down-regulates CCAAT/enhancer binding protein-alpha expression in breast cancer cells. Cancer Res 2008;68:2158-65.
80. Altwerger G, Ghazarian M, Glazer PM. Harnessing the effects of hypoxia-like inhibition on homology-directed DNA repair. Semin Cancer Biol 2024;98:11-8.
81. Henning W, Stürzbecher HW. Homologous recombination and cell cycle checkpoints: Rad51 in tumour progression and therapy resistance. Toxicology 2003;193:91-109.
82. Zhuang Y, Liu K, He Q, Gu X, Jiang C, Wu J. Hypoxia signaling in cancer: implications for therapeutic interventions. MedComm 2023;4:e203.
83. Revythis A, Limbu A, Mikropoulos C, et al. Recent insights into PARP and immuno-checkpoint inhibitors in epithelial ovarian cancer. Int J Environ Res Public Health 2022;19:8577.
84. Giannone G, Ghisoni E, Genta S, et al. Immuno-metabolism and microenvironment in cancer: key players for immunotherapy. Int J Mol Sci 2020;21:4414.
85. Guerrouahen BS, Maccalli C, Cugno C, Rutella S, Akporiaye ET. Reverting immune suppression to enhance cancer immunotherapy. Front Oncol 2019;9:1554.
86. Wang Y, Wang Y, Ren Y, Zhang Q, Yi P, Cheng C. Metabolic modulation of immune checkpoints and novel therapeutic strategies in cancer. Semin Cancer Biol 2022;86:542-65.
Cite This Article
Export citation file: BibTeX | EndNote | RIS
OAE Style
Capuozzo M, Ferrara F, Cinque C, Farace S, Lauritano D, Ottaiano A. Navigating immunotherapy for ovarian cancer: current landscape and future perspectives. J Cancer Metastasis Treat 2024;10:20. http://dx.doi.org/10.20517/2394-4722.2024.38
AMA Style
Capuozzo M, Ferrara F, Cinque C, Farace S, Lauritano D, Ottaiano A. Navigating immunotherapy for ovarian cancer: current landscape and future perspectives. Journal of Cancer Metastasis and Treatment. 2024; 10: 20. http://dx.doi.org/10.20517/2394-4722.2024.38
Chicago/Turabian Style
Maurizio Capuozzo, Francesco Ferrara, Claudia Cinque, Stefania Farace, Domenico Lauritano, Alessandro Ottaiano. 2024. "Navigating immunotherapy for ovarian cancer: current landscape and future perspectives" Journal of Cancer Metastasis and Treatment. 10: 20. http://dx.doi.org/10.20517/2394-4722.2024.38
ACS Style
Capuozzo, M.; Ferrara F.; Cinque C.; Farace S.; Lauritano D.; Ottaiano A. Navigating immunotherapy for ovarian cancer: current landscape and future perspectives. J. Cancer. Metastasis. Treat. 2024, 10, 20. http://dx.doi.org/10.20517/2394-4722.2024.38
About This Article
Special Issue
Copyright
Data & Comments
Data
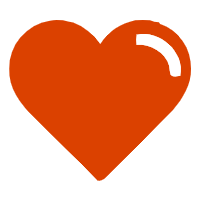
Comments
Comments must be written in English. Spam, offensive content, impersonation, and private information will not be permitted. If any comment is reported and identified as inappropriate content by OAE staff, the comment will be removed without notice. If you have any queries or need any help, please contact us at support@oaepublish.com.