The neurobiology of targeted muscle reinnervation for post-amputation pain
Abstract
Targeted muscle reinnervation (TMR) is a peripheral nerve procedure that can prevent and treat postamputation pain. The nerve transfer allows for organized nerve regeneration and repair after amputation surgery. The procedure can successfully prevent neuromas despite large size mismatches between the donor and recipient nerves. Here, we discuss the fundamentals of peripheral nerve injury and regeneration as it pertains to TMR. We propose axonal pruning to explain axon behavior when there are large size mismatches between transferred nerves. Given the increasing use of TMR for amputees, future studies should investigate the basic science of peripheral nerves in TMR. Advances in this field have the potential to significantly improve clinical outcomes for these patients.
Keywords
INTRODUCTION
More than 2 million amputees live in the United States, and approximately 185,000 amputations occur in the United States each year[1]. Pain is a significant problem affecting more than 70% of amputees[2]. Peripheral nerve injury and subsequent improper axon regeneration result in a disorganized bundle of nerve tissue, known as a neuroma[3]. Neuromas are a known cause of postamputation pain, which includes residual limb pain (RLP), phantom limb sensations (PLS), and phantom limb pain (PLP)[3]. RLP is pain at the site where amputation occurred, often due to nerve injury and neuroma formation[3]. PLS are non-painful sensations in the amputated limb, which may lead to PLP[3]. Meanwhile, PLP represents neuropathic pain localized to the lost limb[3]. Postamputation pain contains significant overlap, and patients with neuromas are significantly more likely to suffer from PLS and PLP[4,5]. Postamputation pain has a significant impact on patient outcomes, including prosthetic use, return to work, and overall quality of life[6].
Unfortunately, postamputation pain is complex and poorly understood, and treatment of postamputation pain remains difficult[4,7]. Targeted muscle reinnervation (TMR) is an emerging surgical procedure to manage nerves and treat pain in amputees. TMR involves transferring the proximal stump of transected major peripheral nerves to nearby motor nerves of muscles that lack function after amputation[8]. In surgery, the major peripheral nerve being managed with TMR is identified, dissected, and cut distally to healthy fascicles. The recipient motor nerve is identified using a nerve stimulator and cut just proximally to any areas of branching into muscle. The small recipient motor nerve is sutured to the center of the large donor nerve in an end-to-end fashion. Fibrin glue is used to reinforce the coaptation and prevent collateral axonal sprouting and neuroma formation. TMR was originally performed by Kuiken et al. in 2004 to improve myoelectric prosthetic control[9]. Incidentally, TMR was found to successfully treat and prevent neuroma pain in amputees, sparking an explosion of new research into TMR[4,7]. Recent literature shows the clinical success of TMR, the expansion of its use, and improvements in surgical technique[4,5,8]. However, there is a paucity of literature exploring the basic science of nerve regeneration pertaining to TMR and how the procedure actually prevents neuroma formation. Surprisingly, the procedure can be successful in preventing neuromata despite a large size discrepancy between donor and recipient nerves[4,10]. No known studies have explained a possible mechanism for axon behavior when there is a large size mismatch between transferred nerves. The purpose of this review is to attempt to explain the process of nerve regeneration in TMR for postamputation pain and to propose axonal pruning as a potential mechanism for axon behavior in the setting of a large size mismatch between coapted nerves.
PERIPHERAL NERVE ANATOMY
The main functions of the PNS are to send sensory information to the CNS, transmit motor commands to voluntary striated muscles in the body, and regulate autonomic functions such as blood pressure[11]. Therefore, the PNS contains motor, sensory, and autonomic nerve fibers that combine to form motor, sensory, or mixed nerves[11,12].
The PNS is myelinated by Schwann cells that increase the speed of action potential propagation[11]. Schwann cells are the supporting cells of the PNS that surround and protect axons[13]. In addition to myelin, peripheral nerves are surrounded by three well-organized connective tissue layers: endoneurium, perineurium, and epineurium[11] [Figure 1]. The endoneurium is the innermost compartment surrounding individual nerve fibers, and it forms the blood-nerve barrier[13]. The nerve fibers are grouped into fascicles, which are enveloped by concentrically arranged perineurium[13]. The epineurium is the outermost layer of peripheral nerves, containing several nerve fascicles and the nerve’s blood supply[13]. The well-organized peripheral nerves and their tissue are essential for proper neurotransmission, as well as normal nerve regeneration following peripheral nerve injury.
Figure 1. Schematic presentation of a peripheral nerve. Source: Nicholls et al.[14].
PERIPHERAL NERVE INJURY
Peripheral nerve injury (PNI) can have a significant impact on a patient’s quality of life[15]. PNI frequently develops into neuropathic pain, which is a complex form of pain that is modulated by both the PNS and CNS[15,16]. Sunderland et al. were the first to classify peripheral nerve injuries and offer a prognosis for nerve repair based on the degree of connective tissue disruption[16] [Figure 2].
Figure 2. Sunderland peripheral nerve injury classification. Source: Deumens et al.[17].
Since mature neurons are terminally differentiated cells that are incapable of mitosis, a nerve injury to the cell body cannot be repaired and results in apoptosis[13]. However, there are two main mechanisms for axonal regeneration: terminal sprouting from injured axons and collateral axonal branching from intact axons[13,18,19]. This is largely because Schwann cells, unlike neurons, can undergo mitosis if injured[13]. Understanding the fundamentals of peripheral nerve degeneration and regeneration after injury will help inform targeted muscle reinnervation for the treatment of postamputation pain.
In limb amputation, peripheral nerve injury is unavoidable[8,20]. Amputation requires nerve transection and can be considered a Sunderland grade 5 nerve injury, in which all connective tissue layers are disrupted and the distal target for nerve regeneration is removed[16] [Figure 2]. Injury begins the peripheral nerve degeneration cascade. Within hours, degeneration of the axon and myelin begins in both directions from the site of injury in a complex process known as Wallerian degeneration[13]. Wallerian degeneration is the rapid, vigorous process of degeneration of distal and some proximal segments of axon after nerve injury[13,17] [Figure 3]. The disruption of the axon plasma membrane causes the influx of extracellular calcium ions, which triggers proteolysis, fragmentation, and degradation of the myelin and axons[21]. Schwann cells dissociate from the axon and transition into repair cells to help digest myelin[21]. Schwann cells initially phagocytose debris before the macrophages are recruited and enter through the leaky blood-nerve barrier[21]. Macrophages are critical to Wallerian degeneration as they rapidly engulf and digest debris, clearing the path for nerve regeneration to occur[17,21]. Wallerian degeneration begins within 24 h of injury and completes after 3 weeks[22].
Figure 3. Overview of the mechanism of peripheral nerve injury and regeneration. Source: Yow et al.[23].
Wallerian degeneration traditionally refers to the degeneration of distal detached axon segments after nerve injury but has also been found to extend proximally from the site of injury[13]. Wallerian degeneration proceeds proximally for about two internodes before the axon is sealed within hours of injury[13]. This is thought to protect the intact axon and cell body from the extracellular environment and apoptosis while allowing retrograde chromatolysis to proceed[13,22].
The chromatolytic changes seen in PNI include disintegration of Nissl bodies (cisterns of the rough endoplasmic reticulum) in the cytoplasm, an eccentric nucleus, a prominent nucleolus, and an increase in RNA and protein synthesis[17]. In peripheral nerve injury, these chromatolytic changes reflect a shift of metabolic activity away from the synthesis of proteins for neurotransmission and towards proteins required for growth and axon sprouting[17]. Depending on the environment and degree of injury, retrograde chromatolysis may cause the neuron to produce apoptotic proteins, in which case nerve regeneration fails and the neuron undergoes programmed cell death[17]. If the neuron survives, the retrograde reaction peaks at 2-3 weeks after the nerve injury[13,17]. In this instance, nerve degeneration quickly transitions to nerve regeneration[17].
PERIPHERAL NERVE REGENERATION
Nerve regeneration begins when Schwann cells undergo mitosis and rapidly proliferate[17] [Figure 3]. After assisting with the initial degradation of the injured axon and myelin, Schwann cells align from both ends of the damaged nerve to form new endoneurial tubes across the injury site[17]. These are known as bands of Büngner, through which new axon sprouts can grow[17].
Once retrograde chromatolysis has peaked, regenerative terminal sprouting and collateral axonal branching can begin[17-19]. Axon sprouts grow along the bands of Büngner at an average rate of 1-3 mm/day towards their distal targets[17]. Distal nerve stumps and target tissues have attractive forces on axon sprouts that likely drive chemotaxis[17]. Since axon sprouting and growth cones require an organized endoneurial tube, the extent of endoneurial tube disruptions during injury determines the healthy regeneration of the nerve axons to their intended targets[16]. Depending on the distance the axon sprouts must travel, sprouts may take weeks to months to traverse the axon injury gap and re-enter appropriate endoneurial tubes[24]. Eventually, the axon sprouts extend through the distal target nerve with support from Schwann cells in the bands of Büngner[24].
Collateral axonal branching occurs via a different mechanism[18,19]. The de novo branches stem from the main axon of the injured neuron or from the axons of nearby uninjured neurons[18]. The outgrowths are formed by actin filament protrusions that become invaded by stable microtubules[18]. The microtubules allow the branches to mature and continue extending toward their target[18]. Collateral branches enter Schwann cell tubes, which guide reinnervation to their target in a similar way to terminal sprouting[25].
Successful nerve regeneration is established when neurotransmission through the injured neuron is restored[17,21]. Axon sprouts that successfully enter the distal target will become re-myelinated and grow stronger with use[13]. Once regeneration is complete, chromatolysis is reversed and cell function returns to normal[17].
NEUROMA FORMATION/FAILURE OF REGENERATION
Nerve regeneration can fail at any step in this process[17]. If the cell body is injured, retrograde chromatolysis may result in the production of apoptotic proteins and cell death[17,26]. If regenerative axon sprouts fail to cross the injury site due to large gaps, a physical barrier formed by scarring, or other factors, the axon sprouts will form a neuroma[17]. Aberrant sprouting may occur in limb amputation when nerves are not given a new target[10]. Neuromas are a common cause of postamputation pain and may negatively impact the function and quality of life of amputees[3,4].
Collateral axonal branching is frequently misdirected and can cause improper innervation of distal targets[27,28]. For instance, nearby sensory nerves can branch to reinnervate the distribution of an injured or cut motor neuron[27,28]. This can produce painful hyperalgesia that is often misinterpreted as neuroma pain[28]. The hyperalgesia due to the collateral branching of sensory axons produces a burning sensation and hypersensitivity to touch[28]. However, collateral branching does not contribute to neuroma formation and is not amenable to surgery. It can be treated with desensitization therapy[28].
PHANTOM LIMB SENSATIONS AND PAIN
PLS are any non-painful sensations that occur in the missing body part after amputation[29,30]. Over 90% of amputees experience PLS in the first 6 months[30]. Phantom sensations may include feelings of movement, touch, tingling, itching, or paresthesia in the missing limb[31].
The causes of phantom sensations are not well understood and are thought to involve both peripheral and central mechanisms. After amputation surgery, neuroma formation and abnormal spontaneous neuronal activity at the proximal end of the cut peripheral nerves may contribute to phantom sensations[32,33]. Neuromata have been shown to correlate with increased duration and intensity of phantom sensations and phantom limb pain[33]. Also, injured peripheral nerves have upregulated sodium channels, causing increased sensitivity to mechanical stimulation and abnormal firing[32,33]. The increased sensitivity of the injured nerves decreases the pressure pain threshold, which may explain why some amputees experience increased PLS and PLP with prosthetic use[32,33].
PLS are also thought to be modulated via central mechanisms. The somatosensory homunculus in the cortex of the brain receives sensory, positional, and movement information from peripheral nerves[34]. The cortical representation of an amputated limb likely persists for some time after limb amputation resulting in a phantom limb[34]. Reorganization of the somatosensory cortex may also underlie phantom sensations[35]. Neurons in the somatosensory cortex that previously responded to signals from the missing limb can begin to respond to signals from other nearby neurons[35]. As a result, stimulation of nerves in other parts of the body can be aberrantly received by the neurons of the amputated limb in the somatosensory cortex, causing sensations to be improperly perceived[35]. Phantom limbs usually change and fade over time, and most PLS disappear after 2 to 3 years[4]. However, PLS may become painful and develop into phantom limb pain in about 45% of patients[34,36].
Phantom limb pain is a complex interplay between neuromas and the central nervous system. PLP is a type of neuropathic pain that can include burning, throbbing, crushing, cramping, or sharp pain in the missing limb[36]. Cortical reorganization is a key component of PLP, and it is even more difficult than neuroma pain to prevent or reverse[4]. Therefore, treating neuromas may prevent cortical reorganization and further centralization of PLP[4,34]. In a prospective, multicenter, randomized clinical trial, Dumanian et al. found evidence that TMR significantly decreases phantom pain in major limb amputees compared to standard treatment at 1 year[4]. Therefore, TMR may prevent PLS and PLP by addressing the contribution of peripheral nerve injury to these experiences.
SIZE MISMATCH AND AXONAL PRUNING
TMR often involves a size mismatch between the donor and recipient nerves at the nerve coaptation site[37] [Figure 4]. The ideal nerve coaptation is a 1:1 diameter ratio and the recommended size ratio is less than 2:1[20]. However, the current practice is to accept large size mismatches in TMR[37]. Depending on the available anatomy, the physiological and clinical implications of the size mismatch are unknown, but Kim
A possible theory to explain why TMR works despite a large size discrepancy between transferred nerves is the process of axonal pruning. Axonal pruning is selective axon degeneration that removes unnecessary, misguided, or excessive axon sprouts while maintaining the integrity of the cell body[26,38,39] [Figure 5]. Singh et al. showed that during axon competition, active (i.e., winning) axons can eliminate less active, competing axons by axonal pruning[40]. Axonal pruning is well established in the development of the CNS and PNS[26,40]. Selective degradation of axons that unsuccessfully innervate their targets or are no longer necessary allows for optimal wiring of neural connections in the developing nervous system[26].
Figure 5. Schematic model illustrating axonal pruning for refining neural connections. Source: Fujita et al.[41].
Axonal pruning has previously been described in the context of nerve injury. Following an injury to a mixed peripheral nerve, regenerating motor axons will preferentially enter both sensory and motor Schwann cell tubes over regenerating sensory axons[42]. The preferential motor reinnervation of distal targets - known as preferential motor reinnervation (PMR) - is made possible by the pruning of sensory axon sprouts while maintaining motor axons[27,42] [Figure 6]. We believe axonal pruning may explain the elimination of misguided axonal sprouting in TMR, allowing for successful reinnervation despite large size mismatches between the donor and recipient nerves.
Figure 6. Preferential motor reinnervation. Following an injury to a mixed peripheral nerve, regenerating axons will preferentially reinnervate modality-matched targets driven by target signals. Regenerating axons that inappropriately innervate mismatched sensory or muscle targets undergo axonal pruning. Source: Mackinnon et al.[43].
Axonal pruning is distinct from Wallerian degeneration and neuronal apoptosis, although all three processes result in axon degeneration[26]. A key feature of axonal pruning is the selective, controlled degradation of axon fibers without inflammation or damage to the cell body[26]. As previously described, Wallerian degeneration is an inflammatory reaction that occurs in response to nerve injury[17], while apoptosis is the programmed death of the entire neuron, including the axon and cell body[26]. The apoptosis pathway is highly restricted after development as neurons cannot regenerate[44]. Axonal pruning, however, is critical to adult neural plasticity, as it permits the selective loss of specific axon sprouts while maintaining nearby axon segments and the integrity of the cell body[26]. Therefore, axonal pruning is the most likely mechanism to explain the behavior of axon sprouts at nerve coaptation sites in TMR.
DISCUSSION
Targeted muscle reinnervation is a major surgical advancement in the field of amputation surgery. Since its introduction in 2004, the use of TMR has been growing rapidly with the main goals of treating and preventing painful neuromas and improving the function of amputees. There has been an abundance of research into the clinical success of TMR and its growing indications for use. However, there is a gap in the basic scientific descriptions of peripheral nerve behavior in TMR. Our review aims to fill this gap and provide a better understanding of neurobiology as it pertains to TMR.
Injured peripheral nerves will always attempt to reach a distal target after injury. Amputation surgery requires the transection of nerves and eliminates a distal target for organized regeneration, creating a high-risk environment for neuroma formation[4,8]. TMR provides a physiologically appropriate environment for regenerating axons and establishes a new distal target, thus preventing neuroma formation. Advances in the understanding of peripheral nervous system pathophysiology can further improve TMR techniques and treatment for patients.
Based on the principles of peripheral nerve injury and regeneration, it is surprising that several clinical studies have found that TMR can successfully prevent neuromas despite large size mismatches between the transferred nerves[4,8]. This is unexpected given the many sprouting axons from the larger proximal peripheral nerve stump that are left without organized connective tissue or a distal target to guide normal regeneration. Here, we propose axonal pruning as a viable explanation for the process of eliminating misguided or unnecessary sprouts when there is a significant size mismatch between transferred nerves in TMR. Axonal pruning may explain why TMR prevents neuroma formation and allows an optimal highway to the new target to form. However, no studies have been performed to support this theory, and further study is necessary to determine the mechanism preventing neuroma formation when there is a large size mismatch between the transferred nerves in TMR.
There are several limitations to this review since the mechanisms behind TMR and postamputation pain are complex and have not been extensively studied. The information in this review was drawn from a diverse set of orthopedic, neurobiology, and pain studies to better understand the behavior of peripheral nerves in TMR. There is a strong need for basic scientific models of TMR and further investigation in this field. It is our hope to trigger future research to further identify the pathways through which TMR can be clinically effective.
TMR can be considered by surgeons performing amputations and for other indications such as neuroma management. Clinical practice momentum is shifting towards routinely offering TMR at the time of primary amputation. However, before recommending this widespread change in clinical practice, comprehensive high-level evidence to support this practice is needed. In this review, we attempt to bridge the gap between the literature on the peripheral nervous system and TMR.
CONCLUSION
In conclusion, TMR can be successful in treating neuromata and postamputation pain in amputees. Given the increasing use of TMR for amputees, further research should be done into the basic science of TMR. A better understanding of the mechanism of peripheral nerve injury can help surgeons improve treatments for amputation patients and develop new surgical techniques to prevent pain. Comprehensive, evidence-based knowledge of TMR has the potential to vastly improve the outcomes, function, and quality of life of amputees.
DECLARATIONS
Authors’ contributionsMade substantial contributions to the conception and design of the study, implementation of the research and interpretation: Tanner N,
Took the lead in writing the manuscript with input and critical feedback: Ayalon O, Tanner N
Discussed the findings and contributed to the final manuscript: Tanner N, Ayalon O
Availability of data and materialsNot applicable.
Financial support and sponsorshipNone.
Conflicts of interestBoth authors have declared that there are no conflicts of interest.
Ethical approval and consent to participateNot applicable.
Consent for publicationNot applicable.
Copyright© The Author(s) 2023.
REFERENCES
1. Limb Loss in the U.S.A. Amputee coalition. Available from: https://www.amputee-coalition.org/wp-content/uploads/2020/03/LLAM-Infographic-2020.pdf [Last accessed on 30 Mar 2023].
2. Hanley MA, Ehde DM, Jensen M, Czerniecki J, Smith DG, Robinson LR. Chronic pain associated with upper-limb loss. Am J Phys Med Rehabil 2009;88:742-51-quiz 752, 779.
3. Ephraim PL, Wegener ST, MacKenzie EJ, Dillingham TR, Pezzin LE. Phantom pain, residual limb pain, and back pain in amputees: results of a national survey. Arch Phys Med Rehabil 2005;86:1910-9.
4. Dumanian GA, Potter BK, Mioton LM, et al. Targeted muscle reinnervation treats neuroma and phantom pain in major limb amputees: a randomized clinical trial. Ann Surg 2019;270:238-46.
5. Valerio IL, Dumanian GA, Jordan SW, et al. Preemptive treatment of phantom and residual limb pain with targeted muscle reinnervation at the time of major limb amputation. J Am Coll Surg 2019;228:217-26.
6. Sheehan TP, Gondo GC. Impact of limb loss in the United States. Phys Med Rehabil Clin N Am 2014;25:9-28.
7. Woo SL, Kung TA, Brown DL, Leonard JA, Kelly BM, Cederna PS. Regenerative peripheral nerve interfaces for the treatment of postamputation neuroma pain: a pilot study. Plast Reconstr Surg Glob Open 2016;4:e1038.
8. Cheesborough JE, Souza JM, Dumanian GA, Bueno RA Jr. Targeted muscle reinnervation in the initial management of traumatic upper extremity amputation injury. Hand (N Y) 2014;9:253-7.
9. Kuiken TA, Dumanian GA, Lipschutz RD, Miller LA, Stubblefield KA. The use of targeted muscle reinnervation for improved myoelectric prosthesis control in a bilateral shoulder disarticulation amputee. Prosthet Orthot Int 2004;28:245-53.
10. Kim PS, Ko JH, O'shaughnessy KK, Kuiken TA, Pohlmeyer EA, Dumanian GA. The effects of targeted muscle reinnervation on neuromas in a rabbit rectus abdominis flap model. J Hand Surg Am 2012;37:1609-16.
11. Chalk C. Neuropathy: peripheral. Encyclopedia of neuroscience. Elsevier; 2009. p. 777-82. Available from: https://www.researchgate.net/publication/246711331_Encyclopedia_of_Neuroscience [Last accessed on 30 Mar 2023].
12. Chhabra A, Ahlawat S, Belzberg A, Andreseik G. Peripheral nerve injury grading simplified on MR neurography: As referenced to Seddon and Sunderland classifications. Indian J Radiol Imaging 2014;24:217-24.
13. Paulsen DF. Nerve tissue. In: Histology and cell biology: examination & board review. 6th ed. McGraw-Hill Education; 2022. Available from: https://www.amazon.com/Histology-Cell-Biology-Examination-Science/dp/0071476652 [Last accessed on 30 Mar 2023].
14. Nicholls K, Furness ND. Peripheral nerve compression syndromes of the upper limb. Surgery (Oxford) 2019;37:288-93.
15. Nicholson B, Verma S. Comorbidities in chronic neuropathic pain. Pain Med 2004;5 Suppl 1:S9-S27.
16. SUNDERLAND S. A classification of peripheral nerve injuries producing loss of function. Brain 1951;74:491-516.
17. Deumens R, Bozkurt A, Meek MF, et al. Repairing injured peripheral nerves: Bridging the gap. Prog Neurobiol 2010;92:245-76.
18. Gallo G. The cytoskeletal and signaling mechanisms of axon collateral branching. Dev Neurobiol 2011;71:201-20.
19. Konofaos P, Bassilios Habre S, Wallace RD. End-to-side nerve repair: current concepts and future perspectives. Ann Plast Surg 2018;81:736-40.
20. Daugherty THF, Mailey BA, Bueno RA Jr, Neumeister MW. Targeted muscle reinnervation in the hand: an anatomical feasibility study for neuroma treatment and prevention. J Hand Surg Am 2020;45:802-12.
22. Scheib J, Höke A. Impaired regeneration in aged nerves: clearing out the old to make way for the new. Exp Neurol 2016;284:79-83.
23. Yow YY, Goh TK, Nyiew KY, et al. Therapeutic potential of complementary and alternative medicines in peripheral nerve regeneration: a systematic review. Cells 2021;10:2194.
24. Craig A. Peripheral nerve injuries: mononeuropathy. In: Mitra R, ed. Principles of rehabilitation medicine. McGraw-Hill Education; 2019. Available from: https://accessmedicine.mhmedical.com/content.aspx?bookid=2550§ionid=206759548 [Last accessed on 30 Mar 2023].
25. Matsumoto M, Hirata H, Nishiyama M, Morita A, Sasaki H, Uchida A. Schwann cells can induce collateral sprouting from intact axons: experimental study of end-to-side neurorrhaphy using a Y-chamber model. J Reconstr Microsurg 1999;15:281-6.
26. Geden MJ, Romero SE, Deshmukh M. Apoptosis versus axon pruning: molecular intersection of two distinct pathways for axon degeneration. Neurosci Res 2019;139:3-8.
27. Brushart TM, Gerber J, Kessens P, Chen YG, Royall RM. Contributions of pathway and neuron to preferential motor reinnervation. J Neurosci 1998;18:8674-8681.
28. Hill EJR, Patterson JMM, Yee A, Crock LW, Mackinnon SE. What is operative? J Hand Surg Glob Online 2023;5:126-32.
30. Jensen TS, Krebs B, Nielsen J, Rasmussen P. Phantom limb, phantom pain and stump pain in amputees during the first 6 months following limb amputation. Pain 1983;17:243-56.
31. Schley MT, Wilms P, Toepfner S, et al. Painful and nonpainful phantom and stump sensations in acute traumatic amputees. J Trauma 2008;65:858-64.
32. Nikolajsen L, Ilkjaer S, Jensen TS. Relationship between mechanical sensitivity and postamputation pain: a prospective study. Eur J Pain 2000;4:327-34.
33. Kajander KC, Wakisaka S, Bennett GJ. Spontaneous discharge originates in the dorsal root ganglion at the onset of a painful peripheral neuropathy in the rat. Neurosci Lett 1992;138:225-8.
34. Flor H, Elbert T, Mühlnickel W, Pantev C, Wienbruch C, Taub E. Cortical reorganization and phantom phenomena in congenital and traumatic upper-extremity amputees. Exp Brain Res 1998;119:205-12.
35. Borsook D, Becerra L, Fishman S, et al. Acute plasticity in the human somatosensory cortex following amputation. Neuroreport 1998;9:1013-7.
36. Ahmed A, Bhatnagar S, Mishra S, Khurana D, Joshi S, Ahmad SM. Prevalence of phantom limb pain, stump pain, and phantom limb sensation among the amputated cancer patients in india: a prospective, observational study. Indian J Palliat Care 2017;23:24-35.
37. Fowler TP. Targeted muscle reinnervation in the hand: a technical roadmap. J Hand Surg Am 2022;47:287.e1-8.
38. Low LK, Cheng HJ. Axon pruning: an essential step underlying the developmental plasticity of neuronal connections. Philos Trans R Soc Lond B Biol Sci 2006;361:1531-44.
39. Luo L, O'Leary DD. Axon retraction and degeneration in development and disease. Annu Rev Neurosci 2005;28:127-56.
40. Singh KK, Park KJ, Hong EJ, et al. Developmental axon pruning mediated by BDNF-p75NTR-dependent axon degeneration. Nat Neurosci 2008;11:649-58.
41. Fujita Y, Yamashita T. Mechanisms and significance of microglia-axon interactions in physiological and pathophysiological conditions. Cell Mol Life Sci 2021;78:3907-19.
42. Fox IK, Brenner MJ, Johnson PJ, Hunter DA, Mackinnon SE. Axonal regeneration and motor neuron survival after microsurgical nerve reconstruction. Microsurgery 2012;32:552-62.
43. Mackinnon SE, Yee A. Chapter 1.11, Anatomy and Physiology for the Peripheral Nerve Surgeon. In: Nerve Surgery. Thieme; 2015. Available from: https://www.thieme-connect.de/products/ebooks/book/10.1055/b-003-106416 [Last accessed on 30 Mar 2023].
Cite This Article
How to Cite
Tanner, N.; Ayalon, O. The neurobiology of targeted muscle reinnervation for post-amputation pain. Plast. Aesthet. Res. 2023, 10, 11. http://dx.doi.org/10.20517/2347-9264.2022.95
Download Citation
Export Citation File:
Type of Import
Tips on Downloading Citation
Citation Manager File Format
Type of Import
Direct Import: When the Direct Import option is selected (the default state), a dialogue box will give you the option to Save or Open the downloaded citation data. Choosing Open will either launch your citation manager or give you a choice of applications with which to use the metadata. The Save option saves the file locally for later use.
Indirect Import: When the Indirect Import option is selected, the metadata is displayed and may be copied and pasted as needed.
About This Article
Special Issue
Copyright
Data & Comments
Data
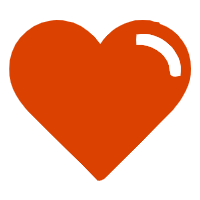
Comments
Comments must be written in English. Spam, offensive content, impersonation, and private information will not be permitted. If any comment is reported and identified as inappropriate content by OAE staff, the comment will be removed without notice. If you have any queries or need any help, please contact us at support@oaepublish.com.