Advances in lower extremity peripheral nerve surgery
Abstract
Peripheral nerve injury (PNI) is a common source of pain and disability in patients. While many patients are affected by PNI, peripheral nerve surgery advancements in the lower extremity have lagged behind the upper extremity. Subsequently, principles that have demonstrated success in the upper extremity have been implemented in the lower extremity. Interventions with recent advances include the advent of novel nerve transfers in the lower extremity and using stem cells and electrical stimulation (ES) for nerve regeneration. This article focuses on advances in nerve transfers for lower extremity PNI and provides details on the basic science and clinical applications of newer interventions.
Keywords
INTRODUCTION
Patients with traumatic injuries may experience pain and disability due to PNI. One recent study found that 1.2% of patients with lower extremity trauma experience PNI, and these patients are more likely to experience chronic pain and require physical and occupational therapy[1]. Therefore, proper diagnosis and treatment are crucial for improving outcomes in these patients. Historically, more attention has been paid to peripheral nerve reconstruction of the upper extremity, with less attention focused on the lower extremity. The longer distances between nerves in the lower extremity make nerve transfers in the leg more challenging. The increased distance required for regeneration can also lead to worse outcomes, as the target muscle(s) may be atrophied by the time of regeneration[2]. Research into nerve regeneration and factors that improve outcomes is crucial for overcoming these obstacles.
There are various options for repair of lower extremity nerve injuries depending on the extent of nerve damage and subsequent nerve gap. Direct repair is the preferred treatment modality in cases where a tensionless repair is possible with a neglectable nerve gap[3]. However, in cases of severe nerve damage, nerve conduits are preferred for gaps less than 3 cm, and auto- or allografts are used for gaps of more than 3 cm[3]. However, the capacity for nerve regeneration and functionality can be limited after reconstruction by scar formation, hemostasis, and infection[3]. Interventions have been proposed to improve nerve regeneration, including adipose-derived stem cells (ADSCs)[4-7], and electrical stimulation[8-10]. Although these interventions have demonstrated potential for improving axonal regeneration and functional nerve recovery, their use in clinical settings remains unclear.
In cases with significant scarring preventing nerve graft surgery, nerve transfers may be viable interventions for restoring muscle function. Nerve transfers have the possibility of earlier reinnervation with restoration of function[11]. Anatomical and clinical studies have investigated new sites for nerve transfers and reported promising results in traumatic cases[12-14] and patients with acute flaccid myelitis[15,16]. The variability of lower extremity nerve injuries requires a personalized approach and understanding of each therapy’s unique advantages and disadvantages. In this article, we will focus on the recent advances in nerve transfers and provide additional details regarding interventions to improve axonal regeneration.
NERVE TRANSFER
In upper extremity injuries, nerve transfers have been increasingly performed to restore motor function[11]. Nerve transfers allow the surgeon to avoid operating in the zone of injury, which may have scarring[11]. Another advantage is the potential for faster recovery, due to a nerve coaptation site closer to the target. Developments in nerve transfers for the lower extremity have lagged behind the upper extremity due to inherent anatomical challenges, such as increased distance for nerve regeneration and fewer nerve branches to serve as donor nerves following spinal cord injuries[2]. Other advantages of nerve transfer surgery in the lower extremity over nerve grafts arise because these injuries often require long nerve grafts, leading to a degeneration of the target distal motor endplate before reinnervation can occur[17]. Ambulation, as well as bowel and bladder control, are priorities for lumbosacral plexus injuries[2]. Examples of promising advances have been reported and are discussed below [Table 1].
Examples of the published articles on the use of nerve transfer for lower extremity nerve injuries
References | Study design Population characteristics Mean age ± SD, F; M ratio | Clinical outcome |
Femoral nerve repair | ||
Campbell et al. (2010)[18] | ● Case report ● 45-year-old female with a retroperitoneal schwannoma involving lumbar plexus | ● Excellent functional recovery and significant quadriceps functions at 2 years after the operation |
Goubier et al. (2012)[20] | ● Cadaveric study ● Investigated the anatomical feasibility of obturator-to-femoral nerve transfer in 5 cadavers (10 thighs) | ● Confirmed that obturator-to-femoral nerve transfer is anatomically possible and may have clinical implications |
Tung et al. (2012)[19] | ● Cadaveric study ● Evaluated the efficacy of obturator nerve transfer to the femoral nerve in both human and cadaveric subjects | ● Obturator-to-femoral nerve transfer is a safe and efficient procedure for the treatment of high femoral nerve injuries |
Karagiannis et al. (2015)[53] | ● Case report ● 49-year-old man with right-sided femoral nerve palsy undergoing dual gracilis and adductor longus to quadriceps muscles | ● Significant functional recovery at 3 years post-operation |
Inaba et al. (2018)[54] | ●Case report ● Partial obturator nerve transfer was done for the repair of an excised femoral nerve after resection of a retroperitoneal schwannoma | ● Significant quadriceps recovery with 4/5 knee extension and normal gait |
Meng et al. (2018)[55] | ● Animal study (rat) ● Investigate the efficacy and feasibility of obturator nerve transfer for repair of injured femoral nerve in rat models | ● A significant functional recovery and increase in quadriceps muscle mass in rat models after nerve transfer was observed |
Rastrelli et al. (2018)[56] | ● Case report ● Anterior branch of the obturator nerve was transferred to the femoral nerve at thigh level in a 19-year-old female | ● Obturator-to-femoral neve transfer is a feasible option when the nerve gap is considerable (≥ 6 cm) |
Doi et al. (2019)[15] | ● Case report ● Contralateral obturator nerve transfer to the left femoral nerve due to acute flaccid myelitis | ● At 14 months post-op, favorable functional outcome with full knee extension was achieved |
Graham et al. (2020)[57] | ● Case report ● A modified obturator-to-femoral neve transfer with cable grafting for a 49-year-old woman with iatrogenic injury to the femoral nerve | ● At 4 years post-op, patient recovered knee extension (4/5) and mobilization was successful |
Cao et al. (2020)[14] | ● Case report ● Contralateral obturator nerve transfer to femoral nerve after extensive lumbar plexus injury in a 30-year-old male | ● Contralateral obturator nerve transfer to femoral nerve is an alternative procedure when the ipsilateral obturator nerve is damaged |
Chen et al. (2020)[21] | ● Cadaveric study ● Evaluate the safety and feasibility of sciatic nerve transfer to the femoral nerve in cadavers | ● Suggested that the muscle branches of sciatic nerve may be a reasonable candidate for femoral nerve repair |
Nicholas et al. (2021)[13] | ● Case report ● Reported two cases of extensive lumbosacral plexus injury accompanied with root avulsion which underwent contralateral obturator-to-femoral neve | ● At the last follow-up, patients had 3/5 and 2/5 knee extension, representing this nerve transfer as a therapeutic option for extensive plexal injuries |
Peters et el. (2021)[12] | ● Retrospective case-series ● Reported the functional outcome of 14 patients with femoral nerve palsy that underwent femoral nerve decompression and nerve transfer | ● Post-operatively, a significant improvement in knee extension muscle power and pain compared with pre-operation (P-value = 0.001) |
Lubelski et al. (2021)[16] | ● Case-series ● Demonstrated sciatic-to-femoral nerve transfer using a fascicle of the proximal tibial nerve as the donor for pediatric patients with acute flaccid paralysis | ● Sciatic-to-femoral nerve transfer is a feasible option for repair of extensive lumbar plexus damage. However, the clinical outcome of patients are not available |
Donaldson et al. (2022)[58] | ● Case-series ● Two patients with femoral nerve injuries underwent concomitant gracilis muscle transfer and obturator-to-femoral neve (adductor longus nerve branch) | ● At 6 months post-op, one patient regained significant knee flexion and full knee extension with grade 4/5 power. ● At 18 months post-op, patient 2 had full knee flexion and extension with grade 5/5 muscle power |
Obturator nerve repair | ||
Spiliopoulos et al. (2011)[27] | ● Case report ● Femoral-to-obturator nerve transfer was done for a female patient with a iatrogenic obturator nerve injury after excision of a gynecologic tumor | ● At 1 year post-op, patient gained full limb adduction and full recovery was observed |
Tibial nerve repair | ||
Koshima et al. (2003)[28] | ● Case report ● First description of nerve transfer for repair of tibial nerve using the deep peroneal nerve | ● Significant improvement in patient’s functional outcome. Both patients were able to walk at the last follow up |
Yin et al. (2015)[29] | ● Case-series ● Evaluated the safety and efficacy of ipsilateral obturator-to-tibial nerve transfer in 5 consecutive patients with sacral plexus injury | ● Significant symptom resolution was observed following the transfer ● Obturator-to-tibial nerve transfer is a feasible option when direct nerve repair is not plausible |
Moore et al. (2017)[17] | ● Case-series and cadaveric study ● Investigated the distal femoral-to-sciatic nerve transfer for proximal nerve injuries | ● Efficient and safe transfer procedure for treatment of proximal tibial nerve injuries |
Agarwal et al. (2018)[59] | ● Prospective case-series ● Saphenous nerve transfer to the posterior tibial nerve was carried out for 21 patients with loss of sensation at the sole | ● At 6 months follow up, significant improvement in sensory perception was observed in most of sole territories |
Meng et al. (2018)[33] | ● Cadaveric study ● Investigated the efficacy and safety of femoral nerve transfer to peroneal and tibial nerves for high sciatic nerve injury | ● Femoral-to-sciatic nerve transfer is a feasible option for restoring muscle and sensory function for sciatic nerve and its branches |
Namazi et al. (2019)[60] | ● Cadaveric study ● Evaluated the safety and feasibility of obturator to tibial nerve transfer with saphenous nerve graft | ● This technique is feasible for patients with sacral nerve root avulsion injury. However, no clinical outcomes are available |
Peroneal nerve repair | ||
Ferris et al. (2017)[31] | ● Case-series ● Partial tibial nerve transfer was carried out for 9 patients with traumatic peroneal nerve injury | ● Excellent functional outcomes were observed for 7/9 patients. the study recommended nerve transfer as an alternative therapeutic option |
Nath et al. (2017)[32] | ● Retrospective case-series ● Investigated the surgical outcomes of 21 patients with foot drop undergoing nerve transfer | ● The results of the study showed significant improvement in functional outcome after the operation |
Meng et al. (2018)[33] | ● Cadaveric study ● Investigated the efficacy and safety of femoral nerve transfer to peroneal and tibial nerves for high sciatic nerve injury | ● Femoral-to-sciatic nerve transfer is a feasible option for restoring muscle and sensory function for sciatic nerve and its branches |
Flores et al. (2013)[34] | ● Retrospective case-series ● Investigated the efficacy and outcome of 13 patients with foot drop undergoing tibial-to-peroneal nerve transfer | ● Nerve transfer from the soleus muscle to the deep peroneal nerve is not recommended due to unfavorable patients outcomes |
Femoral nerve repair
Femoral nerve is the major branch of the L2-L4 lumbar plexus and innervates the hip flexor and knee extensor muscles. It also controls the sensory processing of the anteromedial thighs to the medial compartment of the legs and feet. Injuries to the femoral nerve may result in significant functional impairments depending on the anatomic location of the damaged nerve. Generally, femoral nerve injuries at the pelvis level are classified as high femoral nerve injuries. The first successful employment of nerve transfer for repair of high femoral nerve injury was reported in the study by Campbell et al. in 2010[18]. They transferred the ipsilateral obturator nerve to the injured femoral nerve, which was damaged due to a schwannoma[18]. The impressive restoration of functional outcomes after this nerve transfer was the inspiration for future surgeons to utilize nerve transfer for femoral nerve injuries when a direct repair is not possible due to a considerable nerve gap. Nevertheless, performing a transfer for an injured femoral nerve at the pelvis can be challenging. Goubier and Tung assessed the anatomical feasibility of obturator-to-femoral nerve transfer and confirmed that this nerve transfer is anatomically possible[19,20]. Since then, a few modifications have been made to the femoral nerve transfer to maximize axonal regeneration and nerve viability.
One of the most robust data on nerve transfer for femoral nerve repair came from the study by Peters et al.[12]. They previously reported success in treating high femoral nerve palsy using the motor branches of the anterior obturator nerve to the gracilis, adductor longus, and adductor brevis muscles and the sartorius motor branches to improve quadriceps function[12]. Successful reinnervation to all four quadriceps muscles has been reported in cases where the zone of injury was inaccessible, such as after hip surgery[12]. In this example, femoral nerve decompression was offered in conjunction with nerve transfer surgery as an adjunct therapy for neuropathic pain[12].
In patients with multilevel lumbosacral plexus injuries with concomitant ipsilateral nerve damages, restoration of knee extension has been reported using the contralateral obturator to the femoral nerve transfer[13,14]. This approach has also successfully restored knee extension in a pediatric patient with acute flaccid myelitis[15]. In cases of femoral nerve injury accompanied by bilateral obturator nerve damage, a cadaveric study by Chen et al. suggested the muscle branches of the sciatic nerve may be a reasonable candidate for femoral nerve repair[21]. Lubelski et al. demonstrated sciatic-to-femoral nerve transfer using a fascicle of the proximal tibial nerve as the donor for pediatric patients with acute flaccid paralysis[16]. However, the clinical implications and functional outcomes of this nerve transfer remain unclear.
Other proposed donors in cases of femoral nerve injury include the nerve to semitendinosus[21] and the S1 nerve root[22], as well as the intercostal, ilioinguinal, and iliohypogastric nerves[23]. Overall, the main indication of nerve transfer for repair of a high femoral nerve injury is reserved for patients for whom direct nerve repair or nerve graft surgery is not plausible.
Obturator nerve injury
The obturator nerve originates from the L2-L4 nerve roots and innervates the medial compartment of the thigh, which are responsible for adduction and external rotation of the thigh, as well as sensory processing of medial thigh. It enters the thigh after passing across the pelvis and through the obturator foramen. Obturator nerve injuries are rare and occur most commonly from complications during pelvic surgery. Injury to the obturator nerve results in weakness in thigh adduction and external rotation and sensory loss in the medial thigh. Given the surgical setting of these injuries, nerve repair is often performed intraoperatively with direct repair or nerve grafting[24-26]. Nerve transfers are less common interventions in obturator nerve injuries presenting postoperatively, with a conservative approach being preferred. However, one study reported full restoration of hip adduction and medial thigh sensation after nerve transfer of a branch of the femoral nerve to the obturator nerve[27].
Tibial nerve repair
The tibial nerve is a distal branch of the sciatic nerve (L4-S3 nerve roots) and is responsible for motor and sensory innervations to the posterior leg compartment, as well as foot and toe flexor muscles. Injuries to the tibial nerve may result in significant gait disturbance, impaired foot plantar flexion, and sensory losses. In cases of sciatic nerve injury, repair of the tibial nerve is given priority to ensure plantarflexion strength for walking and protective plantar sensation[17]. The first description of nerve transfer for repair of the tibial nerve was in the study by Koshima et al. in 2003[28]. They successfully used the deep peroneal nerve to restore sensory functions of the injured tibial nerve. Moore et al. described a novel approach for performing nerve transfer of the terminal branches of the femoral nerve supplying vastus medialis and vastus lateralis to the medial and lateral branches of the tibial nerve in cases of tibial and common peroneal nerve palsies after sciatic nerve injury[17]. Obturator nerve transfer to the tibial nerve to the medial head of the gastrocnemius has also been successful in restoring knee and ankle flexion[27]. One cadaver study found feasible targets for restoring tibial nerve function using transfers of the vastus medialis nerve branch to the medial gastrocnemius nerve branch[29]. There is a paucity of data on the utilization of nerve transfer for tibial nerve repair in the current literature. Nevertheless, all published articles reported significant improvements in functional outcomes. Nerve transfer should be taken into consideration as an alternative option, particularly for proximal sciatic nerve injuries.
Peroneal nerve repair
The common peroneal nerve is another major branch of the sciatic nerve, and it provides the motor and sensory processing of anterolateral compartment of legs to the dorsal aspect of feet and toes. The common peroneal nerve is at high risk of injury due to its superficial anatomical course, and it is the most common source of mononeuropathy in the lower extremity[30]. Peroneal nerve palsies arise from trauma, compression, or iatrogenic causes and are classically associated with “foot drop”, which results in gait disturbance and can lead to falls[30]. In cases where conservative management fails to improve nerve function after 4 months, surgical treatment may be required with nerve decompressions, direct nerve repair, nerve or tendon transfers, or ankle fusion[30].
Nerve transfers can restore function for patients with peroneal nerve palsy. Ferris et al. demonstrated improvement in active dorsiflexion in patients with traumatic common peroneal nerve injuries who underwent partial tibial nerve transfer to the motor branches of tibialis anterior[31]. Another study reports successful outcomes in patients with foot drop who undergo superficial peroneal nerve or tibial nerve fascicles transfer to the motor branch of the tibialis anterior and the deep peroneal nerve[32] [Figure 1]. Feasible nerve transfers have been reported in cadaver studies by transferring the vastus lateralis nerve branch to the deep peroneal nerve branch[33]. While there is potential for nerve transfers to help patients, not all nerve transfers have excellent outcomes. Poor outcomes have been reported in the nerve of the soleus muscle to the deep peroneal nerve transfer[34].
Figure 1. Nerve transfer for common peroneal nerve palsy in a 77-year-old female. A: The tibial nerve branch to lateral gastrocnemius (blue star) and the peroneal nerve branch to tibialis anterior nerve (white star) were identified. B: Nerve transfer of the tibial nerve branch to lateral gastrocnemius to the peroneal nerve branch to tibialis anterior.
These novel techniques demonstrate the innovation required to treat patients with PNI in the lower extremity. Nerve transfers have the potential to restore function in cases where other treatments, such as nerve grafting, are not feasible. To optimize outcomes in nerve transfers, the donor activation focused rehabilitation approach has been suggested in upper extremity nerve transfers[35]. Given the success of these interventions in the upper extremity, advances in the lower extremity are promising.
SURGICAL NEUROLYSIS
Neurolysis is another therapeutic option for patients with intractable pain that are not responsive to conventional treatments. Surgical neurolysis refers to the procedure of releasing the entrapped nerves from the adjacent tissues enabling them to decompress and repair. Pess et al. in 1987, described a case of femoral nerve compression following a total hip replacement that was successfully treated with surgical nerve decompression and neurolysis[36]. Since then, the implications of neurolysis for the treatment of lower extremity neuropathic pain have been discussed in the literature with favorable patient outcomes [Table 2]. The main role of neurolysis for lower extremity neuropathic pain is for patients with nerve entrapment. Decompressing the affected nerve from the adjacent fibrous tissues would lead to better functional recovery, symptom relief, and axonal regeneration. Surgical neurolysis is considered a safe and feasible option for nerve decompression [Figure 2]. Complications of surgical neurolysis have been reported rarely, and it mainly depends on surgical technique and the degree of nerve adhesions to the surrounding structures.
Examples of published articles on the implications of nerve neurolysis in the lower extremities
References | Study description | Clinical outcome |
Pess et al. (1987)[36] | ● Case report ● A patient underwent nerve decompression and neurolysis after femoral neuropathy following the use of pressurized cement in total hip arthroplasty | N/A |
Montgomery et al. (2005)[61] | ● Case report ● Late surgical neurolysis for a female patient with sciatic nerve injury after total hip arthroplasty | ● Full functional recover and pain alleviation after the procedure |
Volpi et al. (2005)[62] | ● Case report ● Laparoscopic sciatic nerve neurolysis in a 37-year-old female due to nerve entrapment after endometriosis | ● Significant improvement at the last follow up |
Possover et al. (2007)[63] | ● Case series ● Laparoscopic neurolysis of proximal sciatic nerve and sacral plexus due to endometriosis infiltration | ● Laparoscopic neurolysis is a feasible option for sciatic nerve entrapment |
Ramanan et al. (2011)[64] | ● Retrospective case-series ● Evaluated 20 patients with commo peroneal injury that underwent surgical neurolysis | ● Functional recovery was observed in 74 % and 68% of patients with motor and sensory dysfunction, respectively |
Kyriacou et al. (2013)[65] | ● Prospective cross-sectional ● Investigated the functional outcome of 56 patients with sciatic nerve palsy after hip arthroplasty that underwent surgical neurolysis | ● The mean VAS score decreased significantly after neurolysis ● Surgical neurolysis is associated with improved functional outcome in patients with sciatic nerve injury |
Maalla et al. (2013)[66] | ● Retrospective case-series ● Investigated the role of surgical neurolysis for patients with common peroneal nerve entrapment | ● Excellent outcome in 9 (60.0%) patients after neurolysis |
Aboulfetouh et al. (2014)[67] | ● Case-series ● Evaluate the safety and efficacy of neurolysis for treatment of sciatic nerve entrapment in 11 patients with sciatic nerve injury | ● At 1-year follow up, 10 patients (90.9%) had significant motor and sensory improvement ● Sciatic nerve neurolysis is a safe and efficient option for neuropathic pain without the risk of major complications |
Andrade et al. (2015)[68] | ● Case report ● A 38-year-old female with femoral nerve involvement by endometriosis underwent laparoscopic neurolysis | ● Laparoscopic neurolysis could be the first approach for treatment of femoral nerve endometrial infiltration |
Ham et al. (2018)[69] | ● Retrospective case-series ● Investigated the outcome of patients with deep gluteal syndrome that underwent endoscopic sciatic nerve neurolysis | ● Significant functional outcome with satisfactory pain reduction |
Ilizaliturri et al. (2018)[70] | ● Prospective case-series ● Endoscopic sciatic nerve exploration and neurolysis for 15 patients with deep gluteal syndrome | ● Excellent functional outcome with significant pain alleviation post-operation |
Broekx et al. (2018)[71] | ● Retrospective case-series ● Evaluated the outcome of peroneal nerve neurolysis in patients with foot drop after weight loss | ● External neurolysis is a safe and efficient procedure for foot drop with a success rate of 85% |
Tarabay et al. (2019)[72] | ● Case-series ● 14 patients underwent surgical neurolysis due to common peroneal nerve entrapment | ● 13 out of 14 patients reported significant motor functional recovery after decompression |
Park et al. (2019)[73] | ● Comparative study ● Compared functional outcome of patients undergoing neurolysis after acetabular fracture vs. deep gluteal syndrome | ● Neurolysis was associate with favorable outcomes in both groups; however, patients with deep gluteal syndrome were associated with better outcomes |
Figure 2. Femoral nerve neurolysis. A 39-year-old patient experienced traumatic neuropathic pain and a 2/5 Medical Research Council (MRC) score in knee extension. The patient had improvement in knee extension to MRC 4 function and resolution of pain following femoral nerve (black arrow) neurolysis.
STEM CELL THERAPY FOR NERVE REGENERATION
Nerve transfers may provide a definitive surgical resolution to many cases of lower extremity PNI. Alternative therapies to promote axonal regeneration and improve nerve function may be required in cases where nerve transfers are not possible. Stem cells have been investigated as a therapy for PNI due to their potential to regenerate neurons, support glial cells, and release factors to promote nerve regeneration[4]. Schwann cells, in particular, play a vital role in the regenerative response, although there are challenges associated with harvesting autologous Schwann cells[37]. Schwann cells are procured by harvesting donor nerves and cell culturing, requiring the loss of a functional nerve[37]. For this reason, there have been significant advances in lower extremity nerve regeneration using stem cells and the results have been satisfactory.
Embryonic stem cells
Embryonic stem cells (ESC) are pluripotent cells and can be extracted from the inner embryonic blastocyte layer. They can actively differentiate into almost all cell lineages including neurons and glial cells, which accounts for their regenerative effects[38]. An animal experiment by Cui et al. demonstrated that after the transplantation of ESC-derived neural progenitor cells at the site of sciatic injury, the stem cells differentiated into myelin-producing cells[38]. The transplanted progenitor cells can potentially replace the injured neuron and improve functional outcomes[38]. In another animal study, genetically modified human ESC overexpressing fibroblast growth factor 2 (FGF2) was successfully employed for sciatic nerve injury, which was associated with both sensory and motor resolution[39]. Almost all experimental studies on the use of ESCs for the treatment of lower extremity nerve damage have pointed to their potential regenerative effects [Table 3]. However, a few ethical concerns are limiting the use of ESC in human subjects. The main controversy is the potential moral status of the embryo that prohibits ESC harvesting from the inner blastocytes cell line[40].
Key papers regarding the implications of stem cell therapy for lower extremity nerve regeneration
References/Title | Study design | Clinical outcome |
ESC | ||
Cui et al. | ● Animal study (rat) ● Investigated rat ESC-NPCs’ efficacy in repairing severe sciatic nerve injury | ● Transplanted ESC can differentiate into myelin-producing cells after cell induction and have the potential to repair damaged peripheral nerve injuries |
Mozafari et al. (2018)[39] | ● Animal study (rat) ● Investigating the efficacy of modified ESC with overexpressing FGF-2 for sciatic nerve injury in rat models | ● Significant motor and sensory recovery were observed after modified ESC at the damaged sciatic neuron |
Jones et al. (2018)[74] | ● Animal study (rat) ● Explorated the results of hESC-derived neural crest in the sciatic nerve regeneration | ● In-vivo transplantation of hESC-derived neural crest was suggestive of significant regeneration at the site of sciatic injury |
Chen et al. (2020)[75] | ● Animal study (rat) ● Described the possible role of hESC-NPCs in the regeneration of sciatic nerve | ● hESC-NPCs and their microvesicles have the potential to promote sciatic nerve regeneration |
iPSC | ||
Wang et al. (2011)[42] | ●Animal study (rat) ● Reported electrophysiological results of sciatic nerve injury following NCSC derived from iPSC and ESCs | ● Combination of engineered scaffolds and multipotent stem cells has a higher therapeutic potential for nerve regeneration |
Huang et al. (2017)[43] | ● Animal study (rat) ● Investigate the regenerative effects of various differential stages of human fibroblast-derived iPSCs in the function of transected sciatic nerve | ● iPSC-derived NCSCs were associated with much better short and long-term sciatic nerve regeneration compared with the induced adult Schwann cells |
Xia et al. | ● Animal study (rat) ● Investigated the combination therapy of LIPUS with iPSC-NCSC | ● A combination of LIPUS treatment with iPSC-NCSC, GDF5, and PFTBA can provide a satisfactory outcome for sciatic nerve regeneration |
Lv et al. | ● Animal study (rat) ● Investigating the efficacy of LIPUS with iPSC-NCSC for regeneration of transected sciatic nerve in animal models | ● Results reported a higher rate of regenerated neurofilaments and vasculature with LIPUS stimulation following iPSCs-NCSC seeding |
Yokoi et al. (2018)[78] | ● Animal study (rat) ● Comparing sciatic nerve regeneration in young and old-aged mice following iPSC-derived neurospheres | ● Sciatic nerve regeneration was much slower in old-aged mice compared to younger ages. ● Adding the iPSC-derived neurospheres to the nerve conduit was associated with better axonal regeneration |
Pepper et al. (2017)[79] | ● Animal study (rat) ● Investigating whether motor neurons derived from human iPSCs have the potential to engraft into animal sciatic nerve | ● Although EMG studies reported no signs of functional recovery, the motor neurons in 40.6% of rat models had successful engrafted to the denervated muscles |
BMMSCs | ||
Dezawa et al. (2001)[45] | ● Animal study (rat) ● Efficacy of BMMSCs from rat models for regeneration of injured sciatic nerve | ● Significant nerve fiber regeneration following administration of genetically engineered BMMSC to the end of transected sciatic nerve |
Chen et al. (2006)[46] | ● Animal study (rat) ● Bone marrow-harvested MSCs were genetically engineered and transplanted at the nerve regeneration chamber | ● The experiment resulted in an increase in regenerative nerve fibers after differentiation of BMMSCs to Schwann-like cells |
Raoofi et al. (2021)[80] | ● Animal study (rat) ● BMMSCs were extracted from axotomy rat models and added to the neve conduit loaded with PCL | ● Better nerve regeneration with BMMSC conditioned medium provides satisfactory results for nerve regeneration |
Zheng et al. (2018)[81] | ● Animal study (rat) ● Denervated Schwann cells were co-cultured with neurons induced from BMMSCs in vitro ● The induced neurons were added to the crushed sciatic nerves in rat models (in vivo) | ● Co-culturing was associated with rapid denervated SC proliferation and enhancing the myelination process |
Fernandes et al. (2018)[82] | ● Animal study (rat) ● Compared BMMSC vs. ADSC for regeneration of lesioned sciatic nerve in rat models | ● Nerve regeneration was not satisfactory for both groups when using Matrigel as a conductor |
Cai et al. | ● In vitro and in vivo (animal study) ● Schwann-like cells were derived from human BMMSCs and used for sciatic nerve regeneration | ● Bone marrow derived SC-like cells has a potential for satisfactory axonal regeneration and augmented myelination |
ADSCs | ||
Karakol et al. (2022)[84] | ● Animal study (rat) ● Investigating the effects of epineural tubulization (ENT) with/without ADSCs for sciatic nerve transection | ● Satisfactory axonal regeneration and outcome following ENT+ intratubal ADSC |
Soto et al. (2021)[85] | ●Animal study (rat) ● ADSCs were magnetically recruited to the traumatic sciatic nerve for regenartion | ● A safe delivery method for neuronal regeneration with satisfactory outcomes |
Bucan et al. (2019)[86] | ● Animal study (rat) ● Effects of ADSC-derived exosomes on sciatic nerve remyelination | ● Satisfactory axonal regeneration and outcome |
Fernandes et al. (2018)[82] | ● Animal study (rat) ● Compared BMMSC vs. ADSC for regeneration of lesioned sciatic nerve in rat models | ● Nerve regeneration was not satisfactory for both groups when using Matrigel as a conductor |
Allbright et al. (2018)[87] | ● Animal study (rat) ● Investigate the role of ADSC with PCL delivery system for repair of sciatic nerve injury | ● Enhanced sciatic nerve regeneration and facilitated muscle reinnervation were observed |
Luca et al. (2017)[88] | ● Animal study (rat) ● Evaluated the efficacy of ADSCs with a fibrin gel delivery loaded with laminin | ● Implantation with laminin was associated with satisfactory axonal regeneration |
Induced pluripotent stem cells
To avoid these ethical concerns, Takahashi et al. were the first to induce pluripotent stem cells from animal (mouse) embryonic or human fibroblasts using transcription factors[41]. Their study was one of the first steps toward pluripotency control in somatic cells and providing a safe method for patient-specific stem cell generation. The first use of iPSC for lower extremity nerve regeneration was the study by Wang et al., in which they used iPSCs and ESCs to derive natural crest stem cells (NCSC) for the regeneration of sciatic nerve damage in rat models[42]. They observed that NCSCs can promote nerve myelination and regeneration[42]. Huang et al. conducted an experiment to investigate the regenerative effects of various differential stages of human fibroblast-derived iPSCs in the function of transected sciatic nerve[43]. They observed that the iPSC-derived NCSCs were associated with much better short and long-term sciatic nerve regeneration than the induced adult Schwann cells[43]. That said, there is one major concern regarding the employment of iPSCs and their derivatives in human subjects. Compared with ESCs, iPSCs and their derivations are susceptible to oncogenic transformations due to the pluripotency induction and overexpression of oncogenic factors[44]. Although various strategies have been introduced to diminish the potential tumorigenicity, their use has been limited to animal models only. A summary of the implications of iPSC for lower extremity nerve regeneration is obtained in [Table 3].
Bone marrow-derived mesenchymal stem cell
Bone marrow-derived mesenchymal stem cells (BMMSCs) are another source of pluripotent cells that are located in the stromal bone marrow compartment. Under specific experimental conditions, they have the potential to differentiate into mesenchymal lineages, which accounts for their extensive application in cell-based therapies. The first use of BMMSCs for lower limb nerve regeneration was described by Dezawa et al. in 2001[45]. They observed significant nerve fiber regeneration following administration of genetically engineered BMMSC to the end of transected sciatic nerve[45]. The same observation was made by Chen et al. using BMMSCs from rat models for regeneration of injured sciatic nerve[46]. The experiment resulted in an increase in regenerative nerve fibers after differentiation of BMMSCs to Schwann-like cells. Regardless of their potential beneficial effects, they are limited by their harvesting difficulties, which are usually quite painful. In addition, bone marrow aspirations provide low amounts of stem cells, most of which may get lost due to unsuitable post-translational microenvironment.
Adipose-derived stem cells
Contrary to BMMSCs, adipose-derived stem cells (ADSCs) are the preferred method due to the ease of harvest in great numbers[37]. However, ADSCs are acquired after 2-3 weeks of cell culturing[5]. To address this problem, a stromal vascular fraction (SVF), which is obtained by treating subcutaneous adipose tissue with collagenase, has emerged as a potential source of ADSCs that are immediately available and do not require cell culturing [Figure 3][5].
In 2020, Mathot et al. demonstrated enhanced neoangiogenesis of decellularized sciatic nerve graft defects with ADSCs in rats[48]. Notably, their protocol for cell harvesting is approved for harvesting ADSCs from patients as part of a future clinical trial[48]. ADSCs have also shown potential for improving nerve regeneration in rat studies when delivered to fibrin[6] and nerve conduits[7]. Furthermore, in another study by Shimizu et al., both ADSCs and SVF were shown to have excellent effects on nerve regeneration in a rat model with nerve conduits[5].
Clinical trials investigating the use of ADSCs are limited by the Food and Drug Association, which has not approved using ADSCs that have been enzymatically altered[49]. These federal regulations limit the availability of ADSCs for research, warranting further investigation into alternative sources[37]. One target source of mesenchymal stem cells is the olfactory nerve[50]. An additional intervention showing potential is the use of fat grafting as a source of ADSCs[37]. This is an evolving field of research, and more studies are needed to investigate patient outcomes[49].
ELECTRICAL STIMULATION
An additional intervention to encourage nerve regeneration after PNI is pulsatile ES, which has shown potential as an adjunct therapy by accelerating axonal regeneration and promoting recovery[10]. Keane et al. found that ES accelerated functional recovery when applied at the time of nerve graft surgery in rats[8]. Immunohistochemistry of the harvested nerve revealed increased axonal regeneration and macrophage accumulation[8]. Furthermore, Jo et al. found that ES improved nerve regeneration in a rat model and was comparable to the changes seen with systemic tacrolimus administration[9].
One challenge in the translation of ES at the time of surgery to a clinical setting is that the current protocol tested is one-hour in duration, which adds a significant time and cost burden for clinical trials[10]. Roh et al. found a possible solution to this problem by investigating the benefit of a 10-minute ES session in a rat model[10]. They found accelerated recovery in both the 10- and 60-minute ES groups compared to the control group, with evidence of early axon regeneration in both groups[10]. While no clinical trials have been published investigating ES in PNI in the lower extremity, one recent clinical trial found improved outcomes in patients treated with adjuvant ES during surgery for severe cubital tunnel syndrome[39]. However, more clinical trials are needed to evaluate the clinical applications of electrical stimulation in the lower extremity.
ES can be provided at the time of nerve repair or as part of a long-term approach with a neuroprosthesis. For example, in a case series by Possover et al., 29 patients with spinal cord injuries had long-term low-frequency ES of the pelvic somatic nerves with a neuroprosthesis implanted laparoscopically at the time of surgery[51]. While some patients were reported to have improved sensory and motor function recovery, this study was limited by its design, and further studies are needed to confirm the benefits of ES[51]. Notably, one concern with implantable neuroprosthesis is an induced foreign body reaction, which can compromise benefits by provoking an inflammatory response[52]. However, systemic dexamethasone treatment for 2 weeks in rats was found to significantly attenuate the inflammatory response, demonstrating a potential adjuvant therapy to improve the function of neuroprostheses[52].
CONCLUSION
There have been many significant advancements in peripheral nerve surgery, though advances in the lower extremity have lagged behind the upper extremity. Nerve transfers have been successfully performed in the upper extremity and translated to the restoration of function in the lower extremity. Meanwhile, new targets are being evaluated for their anatomical feasibility through cadaver studies, with case reports of successful implementation in surgery. Nerve regeneration has been researched, primarily through basic science studies, as a critical step that can be improved through ADSCs and ES.
Given the impact of lower extremity PNI on patient well-being, there must be a concerted effort to investigate the benefit of the discussed interventions through continued research. While there are challenges in translating basic science research to the clinical setting, the proposed interventions can be optimized. One key challenge in using ADSCs is finding a source of cells that is readily available and complies with federal regulation, thereby leading to investigations into fat grafting[37]. In ES, the current protocol relies on an hour-long session at the time of surgery, leading to research into a possible shortened duration as a solution[10]. Innovative solutions like these can promote continued advancement; however, clinical trials are necessary before these interventions become standard practice.
DECLARATIONS
Authors’ contributionsMade substantial contributions to the conception and design of the review: Garbuzov A, Nichols DS,
Review of literature, manuscript writing and critical revisions: Shekouhi R
Availability of data and materialsNot applicable.
Financial support and sponsorshipNone.
Conflicts of interestAll authors declared that there are no conflicts of interest.
Ethical approval and consent to participateNot applicable.
Consent for publicationNot applicable.
Copyright© The Author(s) 2023.
REFERENCES
1. Padovano WM, Dengler J, Patterson MM, et al. Incidence of nerve injury after extremity trauma in the United States. Hand (NY) 2022;17:615-23.
2. Bazarek S, Brown JM. The evolution of nerve transfers for spinal cord injury. Exp Neurol 2020;333:113426.
3. Trejo JL. Advances in the ongoing battle against the consequences of peripheral nerve injuries. Anat Rec (Hoboken) 2018;301:1606-13.
4. Panagopoulos GN, Megaloikonomos PD, Mavrogenis AF. The Present and future for peripheral nerve regeneration. Orthopedics 2017;40:e141-56.
5. Shimizu M, Matsumine H, Osaki H, et al. Adipose-derived stem cells and the stromal vascular fraction in polyglycolic acid-collagen nerve conduits promote rat facial nerve regeneration. Wound Repair Regen 2018;26:446-55.
6. Di Summa PG, Schiraldi L, Cherubino M, et al. Adipose derived stem cells reduce fibrosis and promote nerve regeneration in rats. Anat Rec (Hoboken) 2018;301:1714-21.
7. Carriel V, Garrido-Gómez J, Hernández-Cortés P, et al. Combination of fibrin-agarose hydrogels and adipose-derived mesenchymal stem cells for peripheral nerve regeneration. J Neural Eng 2013;10:026022.
8. Keane GC, Pan D, Roh J, et al. The effects of intraoperative electrical stimulation on regeneration and recovery after nerve isograft repair in a rat model. Hand (NY) 2022;17:540-8.
9. Jo S, Pan D, Halevi AE, et al. Comparing electrical stimulation and tacrolimus (FK506) to enhance treating nerve injuries. Muscle Nerve 2019;60:629-36.
10. Roh J, Schellhardt L, Keane GC, et al. Short-duration, pulsatile, electrical stimulation therapy accelerates axon regeneration and recovery following tibial nerve injury and repair in rats. Plast Reconstr Surg 2022;149:681e-90e.
12. Peters BR, Ha AY, Moore AM, Tung TH. Nerve transfers for femoral nerve palsy: an updated approach and surgical technique. J Neurosurg 2022;136:856-66.
13. Nichols DS, Chim H. Contralateral obturator to femoral nerve branch transfer for multilevel lumbosacral plexus avulsion injury. Plast Reconstr Surg Glob Open 2021;9:e3997.
14. Cao Y, Li Y, Zhang Y, et al. Contralateral obturator nerve transfer for femoral nerve restoration: a case report. Br J Neurosurg 2021;35:35-9.
15. Doi K, Sem SH, Hattori Y, Sakamoto S, Hayashi K, Maruyama A. Contralateral Obturator nerve to femoral nerve transfer for restoration of knee extension after acute flaccid myelitis: a case report. JBJS Case Connect 2019;9:e0073.
16. Lubelski D, Pennington Z, Tuffaha S, Moore A, Belzberg AJ. Sciatic-to-femoral nerve end-to-end coaptation for proximal lower extremity function in patients with acute flaccid myelitis: technical note and review of the literature. Oper Neurosurg (Hagerstown) 2021;21:20-6.
17. Moore AM, Krauss EM, Parikh RP, Franco MJ, Tung TH. Femoral nerve transfers for restoring tibial nerve function: an anatomical study and clinical correlation: a report of 2 cases. J Neurosurg 2018;129:1024-33.
18. Campbell AA, Eckhauser FE, Belzberg A, Campbell JN. Obturator nerve transfer as an option for femoral nerve repair: case report. Neurosurgery 2010;66:375; discussion 375.
19. Tung TH, Chao A, Moore AM. Obturator nerve transfer for femoral nerve reconstruction: anatomic study and clinical application. Plast Reconstr Surg 2012;130:1066-74.
20. Goubier JN, Teboul F, Yeo S. Transfer of two motor branches of the anterior obturator nerve to the motor portion of the femoral nerve: an anatomical feasibility study. Microsurgery 2012;32:463-5.
21. Chen H, Meng D, Xie Z, Yin G, Hou C, Lin H. Transfer of sciatic nerve motor branches in high femoral nerve injury: a cadaver feasibility study and clinical case report. Oper Neurosurg (Hagerstown) 2020;19:E244-50.
22. Zhu L, F Zhang, Yang D, Chen A. The effect of severing a normal S1 nerve root to use for reconstruction of an avulsed contralateral lumbosacral plexus: a pilot study. Bone Joint J 2015;97-B:358-65.
23. Toreih AA, Sallam AA, Ibrahim CM, Maaty AI, Hassan MM. Intercostal, ilioinguinal, and iliohypogastric nerve transfers for lower limb reinnervation after spinal cord injury: an anatomical feasibility and experimental study. J Neurosurg Spine 2018;30:268-78.
24. Menderes G, Vilardo N, Schwab CL, Azodi M. Incidental injury and repair of obturator nerve during laparoscopic pelvic lymphadenectomy. Gynecol Oncol 2016;142:208.
25. Andan C, Bakır MS, Şen S, Aksin Ş. Concurrent primary repair of obturator nerve transection during pelvic lymphadenectomy procedure via laparoscopical approach. Int J Surg Case Rep 2018;53:394-6.
26. Yıkılmaz TN, Öztürk E, Hamidi N, Başar H, Yaman Ö. Management of obturator nevre injury during pelvic lymph node dissection. Turk J Urol 2019;45:S26-9.
27. Spiliopoulos K, Williams Z. Femoral branch to obturator nerve transfer for restoration of thigh adduction following iatrogenic injury. J Neurosurg 2011;114:1529-33.
28. Koshima I, Nanba Y, Tsutsui T, Takahashi Y. Deep peroneal nerve transfer for established plantar sensory loss. J Reconstr Microsurg 2003;19:451-4.
29. Yin G, Chen H, Hou C, Xiao J, Lin H. Obturator nerve transfer to the branch of the tibial nerve innervating the gastrocnemius muscle for the treatment of sacral plexus nerve injury. Neurosurgery 2016;78:546-51.
30. Kihm CA, Camasta CA. Review of drop hallux: assessment and surgical repair. J Foot Ankle Surg 2017;56:103-7.
31. Ferris S, Maciburko SJ. Partial tibial nerve transfer to tibialis anterior for traumatic peroneal nerve palsy. Microsurgery 2017;37:596-602.
32. Nath RK, Somasundaram C. Gait improvements after peroneal or tibial nerve transfer in patients with foot drop: a retrospective study. Eplasty 2017:17.
33. Meng D, Chen H, Lin Y, Lin H, Hou C. Transferring of femoral nerve motor branches for high-level sciatic nerve injury: a cadaver feasibility study. Acta Neurochir (Wien) 2019;161:279-86.
34. Flores LP, Martins RS, Siqueira MG. Clinical results of transferring a motor branch of the tibial nerve to the deep peroneal nerve for treatment of foot drop. Neurosurgery 2013;73:609-15; discussion 615.
35. Kahn LC, Moore AM. Donor activation focused rehabilitation approach: maximizing outcomes after nerve transfers. Hand Clin 2016;32:263-77.
36. Pess G, Lusskin R, Waugh T, Battista A. Femoral neuropathy secondary to pressurized cement in total hip replacement: treatment by decompression and neurolysis. Report of a case. JBJS ;69:623-5.
37. Dehdashtian A, Bratley JV, Svientek SR, et al. Autologous fat grafting for nerve regeneration and neuropathic pain: current state from bench-to-bedside. Regen Med 2020;15:2209-28.
38. Cui L, Jiang J, Wei L, et al. Transplantation of embryonic stem cells improves nerve repair and functional recovery after severe sciatic nerve axotomy in rats. Stem Cells 2008;26:1356-65.
39. Mozafari R, Kyrylenko S, Castro MV, Ferreira RS Jr, Barraviera B, Oliveira ALR. Combination of heterologous fibrin sealant and bioengineered human embryonic stem cells to improve regeneration following autogenous sciatic nerve grafting repair. J Venom Anim Toxins Incl Trop Dis 2018;24:11.
40. Bioethics PsCo. Monitoring stem cell research: a report of the president’s council on bioethics. President’s council on bioethics; 2004. Available from: https://bioethicsarchive.georgetown.edu/pcbe/reports/stemcell/ [Last accessed on 7 Mar 2023].
41. Takahashi K, Yamanaka S. Induction of pluripotent stem cells from mouse embryonic and adult fibroblast cultures by defined factors. Cell 2006;126:663-76.
42. Wang A, Tang Z, Park IH, et al. Induced pluripotent stem cells for neural tissue engineering. Biomaterials 2011;32:5023-32.
43. Huang CW, Huang WC, Qiu X, et al. The differentiation stage of transplanted stem cells modulates nerve regeneration. Sci Rep 2017;7:17401.
44. Ben-David U, Benvenisty N. The tumorigenicity of human embryonic and induced pluripotent stem cells. Nat Rev Cancer 2011;11:268-77.
45. Dezawa M, Takahashi I, Esaki M, Takano M, Sawada H. Sciatic nerve regeneration in rats induced by transplantation of
46. Chen X, Wang XD, Chen G, Lin WW, Yao J, Gu XS. Study of
47. Zhang J, Liu Y, Chen Y, et al. Adipose-derived stem cells: current applications and future directions in the regeneration of multiple tissues. Stem Cells Int 2020;2020:8810813.
48. Mathot F, Rbia N, Bishop AT, Hovius SER, Shin AY. Adipose derived mesenchymal stem cells seeded onto a decellularized nerve allograft enhances angiogenesis in a rat sciatic nerve defect model. Microsurgery 2020;40:585-92.
49. Saffari TM, Saffari S, Vyas KS, Mardini S, Shin AY. Role of adipose tissue grafting and adipose-derived stem cells in peripheral nerve surgery. Neural Regen Res 2022;17:2179-84.
50. Brann JH, Firestein SJ. A lifetime of neurogenesis in the olfactory system. Front Neurosci 2014;8:182.
51. Possover M. Ten-year experience with continuous low-frequency pelvic somatic nerves stimulation for recovery of voluntary walking in people with chronic spinal cord injury: a prospective case series of 29 consecutive patients. Arch Phys Med Rehabil 2021;102:50-7.
52. la Oliva N, Navarro X, Del Valle J. Dexamethasone reduces the foreign body reaction to intraneural electrode implants in the peripheral nerve of the rat. Anat Rec (Hoboken) 2018;301:1722-33.
53. Karagiannis P, Ferris SI. Dual nerve transfer of gracilis and adductor longus nerves in restoration of complete femoral nerve palsy. ANZ J Surg 2018;88:E91-2.
54. Inaba N, Sato K, Suzuki T, et al. Partial obturator nerve transfer for femoral nerve injury: A case report. J Orthop Sci 2018;23:202-4.
55. Meng D, Zhou J, Lin Y, et al. Transfer of obturator nerve for femoral nerve injury: an experiment study in rats. Acta Neurochir (Wien) 2018;160:1385-91.
56. Rastrelli M, Tocco-Tussardi I, Tropea S, Rossi CR, Rizzato S, Vindigni V. Transfer of the anterior branch of the obturator nerve for femoral nerve reconstruction and preservation of motor function: a case report. Int J Surg Case Rep 2018;51:58-61.
57. Graham DJ, Sivakumar BS, Lawson R. Modification of obturator to femoral nerve transfer for femoral nerve palsy. Ann R Coll Surg Engl 2020;102:e70-2.
58. Donaldson EK, Chandler RM, Clark TA, Hayakawa TEJ, Giuffre JL. Reconstruction of quadriceps function using a single functional gracilis muscle transfer with an adductor longus nerve to femoral nerve branch of the rectus femoris nerve transfer. Ann Plast Surg 2022;89:419-30.
59. Agarwal P, Shukla P, Sharma D. Saphenous nerve transfer: a new approach to restore sensation of the sole. J Plast Reconstr Aesthet Surg 2018;71:1704-10.
60. Namazi H, Kiani M, Gholamzadeh S, Dehghanian A, Fatemeh DN. Obturator to tibial nerve transfer via saphenous nerve graft for treatment of sacral plexus root avulsions: a cadaveric study. Orthop Traumatol Surg Res 2020;106:291-5.
61. Montgomery AS, Birch R, Malone A. Sciatic neurostenalgia: caused by total hip arthroplasty, cured by late neurolysis. J Bone Joint Surg Br 2005;87:410-1.
62. Volpi E, Seinera P, Ferrero A, Dompè D. Laparoscopic neurolysis of the pelvic sciatic nerve in a case of catamenial footdrop. J Minim Invasive Gynecol 2005;12:525-7.
63. Possover M, Baekelandt J, Flaskamp C, Li D, Chiantera V. Laparoscopic neurolysis of the sacral plexus and the sciatic nerve for extensive endometriosis of the pelvic wall. Minim Invasive Neurosurg 2007;50:33-6.
65. Kyriacou S, Pastides PS, Singh VK, Jeyaseelan L, Sinisi M, Fox M. Exploration and neurolysis for the treatment of neuropathic pain in patients with a sciatic nerve palsy after total hip replacement. Bone Joint J 2013;95-B:20-2.
66. Maalla R, Youssef M, Sebai M, Essadam H. Peroneal nerve entrapment at the fibular head: outcomes of neurolysis. Orthop Traumatol Surg Res 2013;99:719-722.
67. Aboulfetouh I, Saleh A. Neurolysis for secondary sciatic nerve entrapment: evaluation of surgical feasibility and functional outcome. Acta Neurochir (Wien) 2014;156:1979-86.
68. Andrade C, Barata S, António F, Alho C, Calhaz-Jorge C, Osório F. Laparoscopic neurolysis of deep endometriosis infiltrating left femoral nerve: case report. Surg Technol Int 2015;27:163-168.
69. Ham DH, Chung WC, Jung DU. Effectiveness of endoscopic sciatic nerve decompression for the treatment of deep gluteal syndrome. Hip Pelvis 2018;30:29-36.
70. Ilizaliturri VM Jr, Arriaga R, Villalobos FE, Suarez-Ahedo C. Endoscopic release of the piriformis tendon and sciatic nerve exploration. J Hip Preserv Surg 2018;5:301-6.
71. Broekx S, Weyns F. External neurolysis as a treatment for foot drop secondary to weight loss: a retrospective analysis of 200 cases. Acta Neurochir (Wien) 2018;160:1847-56.
72. Tarabay B, Abdallah Y, Kobaiter-Maarrawi S, Yammine P, Maarrawi J. Outcome and Prognosis of Microsurgical Decompression in Idiopathic Severe Common Fibular Nerve Entrapment: Prospective Clinical Study. World Neurosurg 2019;126:e281-7.
73. Park MS, Jeong SY, Yoon SJ. Endoscopic sciatic nerve decompression after fracture or reconstructive surgery of the acetabulum in comparison with endoscopic treatments in idiopathic deep gluteal syndrome. Clin J Sport Med 2019;29:203-8.
74. Jones I, Novikova LN, Novikov LN, et al. Regenerative effects of human embryonic stem cell-derived neural crest cells for treatment of peripheral nerve injury. J Tissue Eng Regen Med 2018;12:e2099-109.
75. Chen X, Ye K, Yu J, et al. Regeneration of sciatic nerves by transplanted microvesicles of human neural stem cells derived from embryonic stem cells. Cell Tissue Bank 2020;21:233-48.
76. Xia B, Chen G, Zou Y, Yang L, Pan J, Lv Y. Low-intensity pulsed ultrasound combination with induced pluripotent stem cells-derived neural crest stem cells and growth differentiation factor 5 promotes sciatic nerve regeneration and functional recovery. J Tissue Eng Regen Med 2019;13:625-36.
77. Lv Y, Nan P, Chen G, Sha Y, Xia B, Yang L. In vivo repair of rat transected sciatic nerve by low-intensity pulsed ultrasound and induced pluripotent stem cells-derived neural crest stem cells. Biotechnol Lett 2015;37:2497-506.
78. Yokoi T, Uemura T, Takamatsu K, et al. Bioabsorbable nerve conduits coated with induced pluripotent stem cell-derived neurospheres enhance axonal regeneration in sciatic nerve defects in aged mice. J Biomed Mater Res B Appl Biomater 2018;106:1752-8.
79. Pepper JP, Wang TV, Hennes V, Sun SY, Ichida JK. Human induced pluripotent stem cell-derived motor neuron transplant for neuromuscular atrophy in a mouse model of sciatic nerve injury. JAMA Facial Plast Surg 2017;19:197-205.
80. Raoofi A, Sadeghi Y, Piryaei A, et al. Bone marrow mesenchymal stem cell condition medium loaded on pcl nanofibrous scaffold promoted nerve regeneration after sciatic nerve transection in male rats. Neurotox Res 2021;39:1470-86.
81. Zheng Y, Huang C, Liu F, et al. Reactivation of denervated Schwann cells by neurons induced from bone marrow-derived mesenchymal stem cells. Brain Res Bull 2018;139:211-23.
82. Fernandes M, Valente SG, Sabongi RG, et al. Bone marrow-derived mesenchymal stem cells versus adipose-derived mesenchymal stem cells for peripheral nerve regeneration. Neural Regen Res 2018;13:100-4.
83. Cai S, Tsui YP, Tam KW, et al. Directed differentiation of human bone marrow stromal cells to fate-committed schwann cells. Stem Cell Reports 2017;9:1097-108.
84. Karakol P, Kapi E, Karaöz E, Tunik S, Bozkurt M. Comparison of the effects of intratubal injection of adipose-derived mesenchymal stem cells in a rat sciatic nerve transection: an experimental study. Ann Plast Surg 2022;88:460-6.
85. Soto PA, Vence M, Piñero GM, et al. Sciatic nerve regeneration after traumatic injury using magnetic targeted adipose-derived mesenchymal stem cells. Acta Biomater 2021;130:234-47.
86. Bucan V, Vaslaitis D, Peck CT, Strauß S, Vogt PM, Radtke C. Effect of exosomes from rat adipose-derived mesenchymal stem cells on neurite outgrowth and sciatic nerve regeneration after crush injury. Mol Neurobiol 2019;56:1812-24.
87. Allbright KO, Bliley JM, Havis E, et al. Delivery of adipose-derived stem cells in poloxamer hydrogel improves peripheral nerve regeneration. Muscle Nerve 2018;58:251-60.
Cite This Article
How to Cite
Garbuzov, A.; Shekouhi, R.; Nichols, D. S.; Chim, H. Advances in lower extremity peripheral nerve surgery. Plast. Aesthet. Res. 2023, 10, 9. http://dx.doi.org/10.20517/2347-9264.2022.51
Download Citation
Export Citation File:
Type of Import
Tips on Downloading Citation
Citation Manager File Format
Type of Import
Direct Import: When the Direct Import option is selected (the default state), a dialogue box will give you the option to Save or Open the downloaded citation data. Choosing Open will either launch your citation manager or give you a choice of applications with which to use the metadata. The Save option saves the file locally for later use.
Indirect Import: When the Indirect Import option is selected, the metadata is displayed and may be copied and pasted as needed.
About This Article
Special Issue
Copyright
Data & Comments
Data
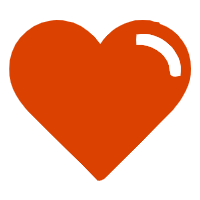
Comments
Comments must be written in English. Spam, offensive content, impersonation, and private information will not be permitted. If any comment is reported and identified as inappropriate content by OAE staff, the comment will be removed without notice. If you have any queries or need any help, please contact us at support@oaepublish.com.