Screening of genetic loci predisposing to herpes simplex virus infection on mouse chromosome 17
Abstract
Aim: The herpes simplex virus (HSV), one of the most common viruses infecting humans, is featured by a high infection rate and usually causes complex disorders difficult to diagnose and treat. Disease progression is always combined with the specific interaction between organism and environment, but genetic factors play a decisive role in most pathogenic processes. Like most human disorders, individual difference has also been involved in the pathogenesis of HSV infection. The present study aimed to screen the potential gene loci that regulates human predisposition to HSV infection.
Methods: With reference to previous studies, inbred mouse lines with significantly distinct predisposition to HSV infection were chosen for gene loci screening. Gene sites on mouse chromosome 17 associated with susceptibility to HSV infection were then identified by correlation analysis and genome-wide scanning technique.
Results: Genes affecting the vulnerability of mice to HSV infection were mapped to three regions on the 17th mouse chromosome, D17MIT51.1, D17MIT39.1 and the region between D17MIT180.1 and D17MIT184.
Conclusion: The results suggest that the mouse genetic background plays an important role in its susceptibility to HSV-1 infection, which might be regulated by multiple predisposing quantitative trait loci.
Keywords
Introduction
As an infection relapse could confer severe consequence in the pathogenicity of herpes simplex virus (HSV) infection, avoiding infection and preventing recurrence after treatment is of great importance. Individual differences involved in the pathogenesis of HSV infection in mice have been long studied. Several reports related to HSV infection susceptibility further pointed to the role of genetic background in the HSV infection process.[1-5] To further analyze the susceptibility to HSV infection in different inbred mouse lines, we scanned the 17th mouse chromosome for gene sites associated with it using the correlation analysis and genome-wide scanning technique.
Methods
Genomic DNA extraction
Firstly, 50-100 frozen tissue samples were weighed, grinded into powder in liquid nitrogen using a grinding bowel and pestle, and then immediately mixed with 1 mL Tripure. Tissue sample powder was then further homogenized 10 times using a homogenizing drill on ice for 20 min until no tissue fluid particles was visible, centrifuged at the speed of 12,000 g at 4 ℃, for 10 min. The homogenate was then kept at room temperature for 5 min to make sure the nuclear protein was totally separated. Each milliliter homogenate was mixed with 0.3 mL chloroform and shaken vigorously at 4 ℃ for 15 s, kept at room temperature for 2-15 min, then Centrifuged at 12,000 g at 4 ℃, for 15 min. To get high quality DNA, the upper layer of colorless aqueous liquid after centrifugation was removed. Each milliliter homogenate was mixed with 0.2 mL 100% ethanol which was stored at 4 ℃, mixed completely by rotating the bottle upside down several times and kept at room temperature for 2-3 min for DNA precipitation. After centrifuging at 2,000 g for 5 min at 4 ℃, the upper layer liquid was removed with a pipette carefully. Each milliliter sample solution was then mixed with 1 mL of 0.1 mol/L sodium citrate dissolved in 10% ethanol, kept at room temperature for 30 min with frequent mixing, and centrifuged at 4 ℃ for 5 min at 2,000 g again. The upper layer liquid was collected, mixed with 75% ethanol, and kept at room temperature for 30 min with frequent mixing. Then, after centrifuging at 4 ℃ and 2,000 g for 5 min, the upper layer liquid was removed and the DNA sample was dried in the air or vacuum for 5-10 min. Finally, we dissolved the DNA with 50 uL TE solution, pipetted out 1 uL sample for color comparison and another 10 uL for electrophoresis, and the residual was stored at -20 ℃ for further analysis. DNA samples were diluted with 90 uL MQ water and analyzed with ultraviolet spectrophotometer. The OD260 value, OD280 value and OD260/280 value were used for calculating the concentration of DNA.
Primer design and synthesis
Primer design referenced information from the mouse genome program (detailed information can be viewed on the website of Jackson Laboratory), which was designed by Shanghai Jikang Biotechnology Limited Company [Table 1]. The detailed information about PCR reaction system and PCR reaction condition can be seen in Table 2.
Primer sequences for scanning microsatellite loci on the 17th chromosome of mouse genome
Loci name | Primer sequence | Genetic distance (cM) | |
---|---|---|---|
D17MIT245.1 | Forward | FAM-TGTGCTCTGGCTAGGGAGTT | 3 |
Reverse | CACATTCATATGTACACACACATGC | ||
D17MIT143.2 | Forward | FAM-CTTACAAGCATCCTGTGGAACTC | 5 |
Reverse | GAGGACCAACAGTCAAACATAGC | ||
D17MIT46 | Forward | FAM-TCCACCCCACTACCTGACTC | 11.7 |
Reverse | CCCTTCTGATGACCACAGGT | ||
D17MIT146.1 | Forward | FAM-CTGTCAGCAGAACGTTCCTTAGT | 17.1 |
Reverse | CCAACTCAAGCCTTACATAGTGG | ||
D17MIT51.1 | Forward | FAM-TCTGCCCTGTAACAGGAGCT | 22.9 |
Reverse | CTTCTGGAATCAGAGGATCCC | ||
D17MIT10.1 | Forward | FAM-TGCACTTGCATAAGGAAAAC | 24.5 |
Reverse | GACTTTGGGGCCTACTTATG | ||
D17MIT180.1 | Forward | FAM-AGACACTGTCTAAAAACACAAGATGG | 29.4 |
Reverse | TTGTGTTCATATGCATGTGTGC | ||
D17MIT20.1 | Forward | FAM-AGAACAGGACACCGGACATC | 34.3 |
Reverse | TCATAAGTAGGCACACCAATGC | ||
D17MIT184 | Forward | FAM-TGCACTACCCAAACATGCAT | 38.5 |
Reverse | ACTTCTGACAGGAAGCATCCA | ||
D17MIT93.1 | Forward | FAM-TGTCCTTCGAGTGTTTGTGTG | 44.5 |
Reverse | TCCCCGGTGAATGAGTTATC | ||
D17MIT39.1 | Forward | FAM-CCTCTGAGGAGTAACCAAGCC | 45.3 |
Reverse | CACAGAGTTCTACCTCCAACCC | ||
D17MIT122.1 | Forward | FAM-TCTCTTCACTGCAATGGAACA | 51.9 |
Reverse | GAACCTATAGGCTCTTGAATAGATGG |
PCR reaction system and PCR reaction condition
PCR reaction system (total volume: 10 uL) | |
---|---|
Non-enzyme water | 5.4 μL |
10 × PCR buffer | 1.0 uL |
Mg2+ (25 mmol/L) | 0.5 uL |
dNTP (each 2.5 mmol/L) | 1.0 μL |
P1 (5 pM) | 0.5 uL |
P2 (5 pM) | 0.5 uL |
Template DNA (30-50 ng/uL) | 1.0 uL |
Ex-Taq enzyme (5 U/μL) | 0.1 μL |
PCR reaction condition | |
95°C | 5 min |
94°C | 30 s |
Time | 30 s |
72°C | 30 s |
Repeat the 2nd to 4th steps for totally 38 cycles | |
72°C | 10 min |
Store at 4°C |
Microsatellite loci detection
First, 1 mL Hi-Di Formamide was mixed with 50 uL GeneScan -500 LIZ Size Standard, then mixed with 9.5 uL polymerase chain reaction (PCR) product, which was diluted 20 times. Tubes containing the above solution were placed in the PCR instrument for degeneration at 95 ℃ for 5 min, kept on ice for more than 5 min. Using an ABI PRISMTM 310 genetic analyzer of the ABI Company for electrophoresis, the voltage was set to 15 KV and run at 60 ℃ for 28 min. The samples were then collected for further analysis.
Electrophoresis data processing
By using the software Genescan (311) and Genetyper (3.7), we could get the detected fragment size. The equipment was provided by ABI Company; the PCR amplification reagents by Baosheng Bioengineering Limited Company; and the fluorescent primers by Shanghai Jikang Biotechnology Limited Company.
Results
Scanning 12 microsatellite spots on the 17th chromosome of two mouse lines
The differences of 12 microsatellite loci between two inbred mouse lines BALB/C and C57BL/6 were first scanned. Among the loci scanned, seven of them were significantly different: D17MIT245.1, D17MIT46, D17MIT51.1, D17MIT180.1, D17MIT20. 1, D17MIT184 and D17MIT39.1 [Table 3; Figure 1].
Microsatellite loci scanning using BALB/C and C57BL/6 inbred mice
Microsatellite loci | BALB/C susceptible | C57BL/6 tolerant |
---|---|---|
D17MIT245.1 | 194 | 202 |
D17MIT143.2 | 112 | 112 |
D17MIT46 | 218 | 236 |
D17MIT146.1 | 166 | 166 |
D17MIT51.1 | 152 | 154 |
D17MIT10.1 | 155 | 155 |
D17MIT180.1 | 139 | 137 |
D17MIT20.1 | 163 | 175 |
D17MIT184 | 126 | 128 |
D17MIT93.1 | 155 | 155 |
D17MIT39.1 | 86 | 104 |
D17MIT122.1 | 141 | 141 |
Scanning microsatellite loci on the 17th chromosome using three inbred mouse lines
To minimize false-positives among the above seven sites obtained using the two inbred mouse lines, we further searched the literature and found that another inbred mouse line, DBA-2, had similar susceptibility to HSV infection as BALB/C mice. Therefore, scanning these three inbred mouse lines for microsatellite loci led to the exclusion of two of the above seven loci, D17MIT245.1 and D17MIT46. Our updated scanning results showed that D17MIT51.1, D17MIT39.1 and the genomic region between D17MIT180.1 and D17MIT184 were mouse microsatellite regions affecting susceptibility to HSV infection [Table 4; Figure 2].
Microsatellite loci scanning using BALB/C, DBA-2 and C57BL/6 inbred mice
Microsatellite loci | Susceptible | Tolerant | |
---|---|---|---|
BALB/C | DBA-2 | C57BL/6 | |
D17MIT245.1 | 194 | 200 | 202 |
D17MIT143.2 | 112 | 112 | 112 |
D17MIT46 | 218 | 208 | 236 |
D17MIT146.1 | 166 | 166 | 166 |
D17MIT51.1 | 152 | 152 | 154 |
D17MIT10.1 | 155 | 149 | 155 |
D17MIT180.1 | 139 | 139 | 137 |
D17MIT20.1 | 163 | 163 | 175 |
D17MIT184 | 126 | 126 | 128 |
D17MIT93.1 | 155 | 169 | 155 |
D17MIT39.1 | 86 | 86 | 104 |
D17MIT122.1 | 141 | 123 | 141 |
Bioinformatic analysis of genes in the HSV infection susceptibility regions on chromosome 17
For identifying potential genes involved in the susceptibility of mice to HSV infection, we used bioinformatics to analyze the genes localized in these regions. Based on the above results, bioinformatic analysis found approximately 140 genes in the positive sites D17MIT51.1, D17MIT39.1 and the region between D17MIT180.1 and D17MIT184 [Tables 5-7]. Among those genes, there were about 33 human homologous genes that showed some of the following characteristics: (1) containing many quantitative trait loci, such as epididymal fat pad weight quantitative trait loci (QTL) 3, subcutaneous fat pad weight QTL 4, spleen weight QTL 9, etc.; (2) containing some genes related to the important physiological functions of the body such as Mut methylmalonyl-Coenzyme A mutase; (3) containing genes related to the developmental and physiological function such as early growth adjusted QTL 2, early growth QTL 5, etc.; (4) containing genes associated with some diseases such as the Down syndrome critical region gene 1-like 1, MSM lymphoma resistance 1, etc.; (5) containing mouse tissue associated antigen H-2.
Bioinformatics of genes in microsatellite loci D17MIT51.1 region
No. | Mouse genome | Corresponding human genes | Functions |
---|---|---|---|
1 | Epididymal fat pad weight QTL 3 | QTL | |
2 | Subcutaneous fat pad weight QTL 4 | QTL | |
3 | Spleen weight QTL 9 | QTL | |
4 | Early growth adjusted QTL 2 | QTL | |
5 | Early growth QTL 5 | QTL | |
6 | Pulmonary adenoma susceptibility 12 | QTL | |
7 | Weight 6 weeks QTL 11 | QTL | |
8 | Weight 10 weeks QTL 12 | QTL | |
9 | DNA segment, Chr 17, Hunter 19 | ||
10 | DNA segment, Chr 17, Hunter 20 | ||
11 | DNA segment, Chr 17, Hunter 21 | ||
12 | Down syndrome critical region gene 1-like 1 | DSCR1L1 | |
13 | Fat pad 7 | QTL | |
14 | Mandibular morphogenesis 1 | QTL | |
15 | MSM lymphoma resistance 1 | QTL | |
16 | Bystin-like | BYSL | |
17 | DNA segment, Chr 17, ERATO Doi 191, expressed | ||
18 | DNA segment, Chr 17, ERATO Doi 763, expressed | ||
17 | Methylmalonyl-Coenzyme A mutase | MUT | |
18 | Neuroscience mutagenesis facility, 318 | ||
19 | Cysteine-rich secretory protein 2 | CRISP2 | |
20 | DNA segment, Chr 17, Tubingen 37 | ||
21 | DNA segment, Chr 17, Tubingen 16 | ||
22 | Ventral midbrain iron content 9 | QTL | |
23 | Soft tissue heal 11 | QTL | |
24 | DNA segment, Chr 17, Tubingen 37 | ||
25 | Gastritis type A susceptibility locus 4 | QTL | |
26 | H2 (histocompatibility-2, MHC) | Complex/cluster/region | |
27 | Histocompatibility 2, Q region | Complex/cluster/region | |
28 | Long bones 10 | QTL | |
29 | Lymphoma latency acceleration | QTL | |
30 | Leishmaniasis resistance 1 | QTL | |
31 | Locomotor activity 2 | QTL | |
32 | T cell receptor beta variable 4, control 1 | QTL | |
33 | T-cell receptor induced activation 3 | QTL | |
34 | Modifier of Odc1 | QTL | |
35 | UVB induced immunosuppression 2 | QTL | |
36 | Cleidocranial dysplasia | Complex/cluster/region detail | |
37 | Ectonucleotide Pyrophosphatase/phosphodiesterase 5 | ENPP5 | |
38 | T-complex-associated testis expressed 1 | TCTE1 | |
39 | Xenotropic murine leukemia virus 57 |
Bioinformatics of genes in microsatellite loci D17MIT39.1 region on the 17 mouse chromosome
No. | Mouse genome | Corresponding human genes | Functions |
---|---|---|---|
1 | Laminin receptor 9 | ||
2 | Proteoglycan induced arthritis 20 | QTL detail | |
3 | Ribosomal protein L19, related sequence 8 | pseudogene | |
4 | T-cell integration locus | ||
5 | Xanthine dehydrogenase | XDH | xanthine dehydrogenase |
6 | Sine oculis-related homeobox 2 homolog (Drosophila) | SIX2 | |
7 | Sine oculis-related homeobox 3 homolog (Drosophila) | SIX3 | |
8 | MutS homolog 2 (E. coli) | MSH2 | DNA mismatch repair protein, eukaryotic MSH2 type |
9 | Carcass protein in high growth mice 3 | QTL | |
10 | DNA segment, Chr 17, XREFdb 57 |
Bioinformatics of genes in microsatellite loci from D17MIT180.1 to D17MIT184 region
No. | Mouse genome | Corresponding human genes | Functions |
---|---|---|---|
1 | High mobility group nucleosomal binding domain 1, related sequence 8 | ||
2 | Cyclin D3 | CCND3 | |
3 | Ecotropic viral integration site 14 | ||
4 | High mobility group nucleosomal binding domain 2. related sequence 4 | ||
5 | DNA segment, Chr 17, Roswell Park 11, expressed | ||
6 | Transplantation-specific integration cluster 1 | ||
7 | Body weight 2 | ||
8 | DNA segment, Chr 17, CEPH 9 | ||
9 | DNA segment, Chr 17, Le Roy 1 | ||
10 | DNA segment, Chr 17, Tubingen 40 | ||
11 | P300/CBP-associated factor | PCAF | |
12 | Progastricsin (pepsinogen C) | PGC | |
13 | Thymus specific insertion locus | ||
14 | Meprin 1 alpha ;MGI:96963 | MEP1A | |
15 | DNA segment, Chr 17, Seldin 7 | ||
16 | Macrophage migration inhibitory factor, pseudogene 8 | Pseudogene | |
17 | Cerebellar cAMP 8 | QTL | |
18 | DNA segment, Chr 17, John C. Schimenti 39 | ||
19 | DNA segment, Chr 17, National Cardiovascular Center, Shionogi 7 | ||
20 | DNA segment, Chr 17, National Cardiovascular Center, Shionogi 34 | ||
21 | DNA segment, Chr 17, XREFdb 556 | ||
22 | abdominal fat weight 3 | QTL | |
23 | DNA segment, Chr 17, Abbott 3 | ||
24 | DNA segment, Chr 17, Birkenmeier 8 | ||
25 | DNA segment, Chr 17, Tubingen 20 | ||
26 | Heligmosomoides polygyrus nematode resistance 7 | QTL | |
27 | Heligmosomoides polygyrus nematode resistance 7 | QTL | |
28 | Obesity and body weight QTL 4 | QTL | |
29 | RAB5A, member RAS oncogene family | RAB5A | |
30 | Skin tumor susceptibility 10 | QTL | |
31 | DNA segment, Chr 17, Brigham Young University 2 | ||
32 | High density lipoprotein (HDL) level 4 | QTL | |
33 | Vav 1 oncogene | VAV1 | |
34 | Nrtn ;neurturin; | NRTN | |
35 | Creatine kinase, brain, related sequence 2 | ||
36 | Epstein-Barr virus induced gene 3 | EBI3 | |
37 | DNA segment, Chr 17, Hunter 24 | ||
38 | Ephrin A5 | EFNA5 | |
39 | RAS-like, family 2, locus 3 | ||
40 | Protein tyrosine phosphatase, receptor type, S | PTPRS | |
41 | Abdominal fat percentage 1 | QTL | |
42 | P. chabaudi malaria resistance QTL 7 | ||
43 | Caseinolytic peptidase, ATP-dependent, proteolytic subunit homolog (E. coli) | CLPP | |
44 | Plasmacytoma susceptibility 5 | ||
45 | Ribosomal protein L32, related sequence 7 | Pseudogene | |
46 | Sulfotransferase family, cytosolic, 1C, member 1 | ||
47 | DNA segment, Chr 17, Tubingen 23 | ||
48 | Complement component 3 | C3 | |
49 | CD86 expression in activated macrophages | QTL | |
50 | DNA segment, Chr 17, Hunter 15 | ||
51 | DNA segment, Chr 17, University of California at Los Angeles 2 | ||
52 | EGF-like module containing, mucin-like, hormone receptor-like sequence 1 | EMR1 | |
53 | EGF-like module containing, mucin-like, hormone receptor-like sequence 4 | EMR4 | |
54 | Modifier of obesity 4 | QTL | |
55 | Fer (fms/fps related) protein kinase, testis specific 1 | ||
56 | tubulin, beta 4 | TUBB4 | |
57 | DNA segment, Chr 17, ERATO Doi 599, expressed | ||
58 | DNA segment, Chr 17, Hunter 16 | ||
59 | SH3-domain GRB2-like 1 | SH3GL1 | |
60 | Thin fur | ||
61 | Abdominal fat percent QTL 6 | QTL | |
62 | Early somite stage arrest 15a | ||
63 | HDL QTL 29 | QTL | |
64 | KH-type splicing regulatory protein | KHSRP | |
65 | Regulatory factor X, 2 (influences HLA class II expression) | RFX2 | |
66 | Skeletal muscle weight 5 | QTL | |
67 | DNA segment, Chr 17, Wayne State University 104, expressed | C19orf10 | |
68 | DNA segment, Chr 17, XREFdb 173 | ||
69 | Immune response 5 | ||
70 | Feminization 1 homolog a (C. elegans) | FEM1A | |
71 | DNA segment, Chr 17, Indiana University Medical 1 | ||
72 | DNA segment, Chr 17, John C. Schimenti 20 | ||
73 | DNA segment, Chr 17, XREFdb 181 | ||
74 | Laminin, alpha 1 | LAMA1 | |
75 | RalA binding protein 1 | RALBP1 | |
76 | Twisted gastrulation homolog 1 (Drosophila) | TWSG1 | |
77 | Protein tyrosine phosphatase, receptor type, M | PTPRM | |
78 | Age related hearing loss 3 | QTL | |
79 | Stathmin 1, related sequence 2 | pseudogene | |
80 | Abdominal fat weight QTL 7 | QTL | |
81 | DNA segment, Chr 17, Birkenmeier 9 | ||
82 | DNA segment, Chr 17, Brigham and Women’s Genetics 1496 expressed |
Discussion
It has been widely observed that different species or even individuals of the same species show differences in response to infection, but the explanation for this phenomenon is still rather controversial. There have been reports suggesting that the genetic background might play an important role.[1,6-8] The causative factors for different responses to infection, the possible ways of intervention, the revolutionary changes of infection prevention, and the control that resulted from those changes have aroused great interest among the scientific community. In this study, we analyzed the genetic background that contributes to the HSV infection susceptibility.
The Herpes virus genus (Herpesviridae) is among the enveloped, linear, double-stranded DNA viruses that widely exist in nature. Approximately 100 HSV species have already been identified or partially identified. Among them, two HSV species, SV-1 and HSV-2, that share 50% homology, have been closely associated with humans. According to statistics provided by the WHO, approximately 70% of the total population worldwide carries HSV antibodies and more than one-third suffers from recurrent HSV infection. Along with its high prevalence rate, a variety of human diseases are closely related with HSV infection, including human herpes labialis, herpes conjunctivitis, 20 herpes zoster encephalitis and other diseases causing great harm to human health. HSV encephalitis is the most common, sporadic, viral encephalitis, accounting for 10-20% of acute, viral, encephalitis and 60-80% of natural mortality. Understanding the complex and specific characteristics of HSV infection-related diseases has been a scientifically and socially pressing need that has led to overcoming the recent difficulties in diagnosis and treatment.
As a viral disease seriously affecting human health with an increasing incidence in recent years, herpes simplex virus 1 (HSV-1) infection typically generates uncomfortable, watery blisters on the skin or mucous membranes of the mouth and lips,[9,10] potentially leads to encephalitis with remarkable sequelae, or vesicle eruption on genital organs.[11] More importantly, the eruption of these blisters and vesicles are frequently attributed to the long-term latent infection of HSV-1 in the nervous system.[12] Although the human HSV infection rate is very high, it is difficult to fully attract people’s attention, so it’s difficult for us to associate HSV infection with genetic background. Therefore, most of the previous HSV infection studies focused on the acquired immune response after infection, showing that T cell-mediated immune response plays an important role in resisting HSV-1 infection,[13,14] and immune suppressed or immune deficient individuals are vulnerable to opportunistic herpes virus infection.[15] Furthermore, recent studies[16,17] suggested that innate immune response plays a key role in limiting the spread of the virus. The development of innate immune germ line occurs earlier than acquired immune response system,[18] and these two mechanisms function differently. This is undoubtedly an important point that genetic backgrounds play an important role in HSV infection. Meanwhile, the clinical symptoms of acute infection, as well as the long-term pathologic processes induced by recurrent latent infection, have been shown to closely correlate with the complex viral genome structures and the molecular mechanism of viral gene transcriptional regulation and replication.[19,20]
In fact, as early as 1975, Lopez[1] reported that there are significant differences in the genetic backgrounds of inbred mice that had significantly different reactions to the same or similar HSV infection, which undoubtedly suggested that genetic background might be an important factor for susceptibility to infection. This mechanism revealed by Lopez has also been confirmed by other studies.[2,3] Also, there was a follow-up study on the relationship between the genetic background and susceptibility to HSV infection. Zawatzky et al.[21] showed that compared with susceptible DBA/2 mice, the relatively tolerant C57BL/6 mice could produce more interferons in the immune response when it comes to HSV-1 infection. But Brenner et al.[22] showed that there is no significant difference in the immune response to HSV infection among those two mouse lines. If the findings in mortality after infection phenotype, Simmons et al.[23] reported that only one gene loci functions in this process, while Kastrukoff et al.[24] reported that there were two loci separated in the role. We are inclined to believe that from the viewpoint of a gene associated with genetic background, it is undoubtedly that genetic background plays an important role in the phenotypic susceptibility to HSV infection. Since the genetic background is polygenic, and HSV has no apparent genetic background, the susceptibility of HSV infection itself is very likely regulated by more than one gene controlling quantitative traits.
By bioinformatics analysis, our results suggest that approximately 140 genes were found in the area of D17MIT51.1, D17MIT39.1 and the genomic region between D17MIT180.1 and D17MIT184, and functions of the majority of those genes are not fully revealed. There is a possibility that the above sites are related to the susceptibility to HSV infection, especially the growth-related genes which are highly suggestive of the importance of genetic background. Among these 140 genes, there were about 33 genes homologous to human genes. Their main functions include binding with other partners, regulating a variety of physiological processes, and the modulation of the phosphorylation process of various enzymes and coenzymes.
Based on our experimental results and bioinformatics analysis, the genetic background might play an important role in susceptibility to HSV infection, which is also consistent with most previous studies. It is worth noting that our finding is likely to be a quantitative trait locus, and may not be a particular system or population-specific mechanism. It is just a hint of this phenomenon in a particular system or population which has a relative higher or lower incidence in another race or ethnic groups. This is not consistent with some previous research.
In summary, the biological information and related data analysis suggest that these genes have important physiological and pathological functions. However, up to now, their associations with HSV susceptibility infection have not been reported, suggesting that they could be potential candidate genes that contributed to the different susceptibility to HSV infection.
Because HSV infection phenotypes have not been clearly defined yet, some issues are far from a consensus. For instance, whether the differences in response to HSV infection really exist and whether the genetic background plays a role in it. All these issues will undoubtedly limit the objectivity of this research. It is also necessary to validate the results in the present study by expanding the sample size, further investigating the role of the regulatory regions in regulating susceptibility to HSV infection. Furthermore, the functions of genes near these microsatellite loci, as well as their functions in regulating susceptibility, deserve further investigation.
Financial support and sponsorship
Nil.
Conflicts of interest
There are no conflicts of interest.
Patient consent
There is no patient involved.
Ethics approval
Ethics approval was obtained prior to the commencement of the study.
REFERENCES
1. Lopez C. Genetics of natural resistance to herpesvirus infections in mice. Nature 1975;258:152-3.
2. Kirchner H, Kochen M, Munk K, Hirt HM, Mergenhagen SE, Rosenstreich DL. Differences in susceptibility to herpes simplex virus infection of inbred strains of mice. IARC Sci Publ 1978;(24 Pt 2):783-8.
3. Lopez C. Resistance to herpes simplex virus - type 1 (HSV-1). Curr Top Microbiol Immunol 1981;92:15-24.
4. Cantin E, Tanamachi B, Openshaw H, Mann J, Clarke K. Gamma interferon (IFN-gamma) receptor null-mutant mice are more susceptible to herpes simplex virus type 1 infection than IFN-gamma ligand null-mutant mice. J Virol 1999;73:5196-200.
5. Umene K, Yamanaka F, Oohashi S, Koga C, Kameyama T. Detection of differences in genomic profiles between herpes simplex virus type 1 isolates sequentially separated from the saliva of the same individual. J Clin Virol 2007;39:266-70.
6. McLeod R, Buschman E, Arbuckle LD, Skamene E. Immunogenetics in the analysis of resistance to intracellular pathogens. Curr Opin Immunol 1995;7:539-52.
7. Smith MW, Dean M, Carrington M, Winkler C, Huttley GA, Lomb DA, Goedert JJ, O'Brien TR, Jacobson LP, Kaslow R, Buchbinder S, Vittinghoff E, Vlahov D, Hoots K, Hilgartner MW, O'Brien SJ. Contrasting genetic influence of CCR2 and CCR5 variants on HIV-1 infection and disease progression. Hemophilia Growth and Development Study (HGDS), Multicenter AIDS Cohort Study (MACS), Multicenter Hemophilia Cohort Study (MHCS), San Francisco City Cohort (SFCC), ALIVE Study. Science 1997;277:959-65.
8. Lagrange PH, Abel L. The genetic susceptibility to leprosy in humans. Acta Leprol 1996;10:11-27. (in French)
9. Xu F, Sternberg MR, Kottiri BJ, McQuillan GM, Lee FK, Nahmias AJ, Berman SM, Markowitz LE. Trends in herpes simplex virus type 1 and type 2 seroprevalence in the United States. JAMA 2006;296:964-73.
10. Fatahzadeh M, Schwartz RA. Human herpes simplex virus infections: epidemiology, pathogenesis, symptomatology, diagnosis, and management. J Am Acad Dermatol 2007;57:737-63; quiz 764-6.
12. Thompson RL, Sawtell NM. The herpes simplex virus type 1 latency-associated transcript gene regulates the establishment of latency. J Virol 1997;71:5432-40.
13. Nash AA, Cambouropoulos P. The immune response to herpes simplex virus. Semin Virol 1993;4:181-6.
14. Rouse BT, Babiuk LA. Mechanisms of recovery from Herpesvirus infections - a review. Can J Comp Med 1978;42:414-27.
15. Posavad CM, Koelle DM, Shaughnessy MF, Corey L. Severe genital herpes infections in HIV-infected individuals with impaired herpes simplex virus-specific CD8+ cytotoxic T lymphocyte responses. Proc Natl Acad Sci U S A 1997;94:10289-94.
16. Biron CA. Initial and innate responses to viral infections -- pattern setting in immunity or disease. Curr Opin Microbiol 1999;2:374-81.
17. Pien GC, Nguyen KB, Malmgaard L, Satoskar AR, Biron CA. A unique mechanism for innate cytokine promotion of T cell responses to viral infections. J Immunol 2002;169:5827-37.
18. Kimbrell DA, Beutler B. The evolution and genetics of innate immunity. Nat Rev Genet 2001;2:256-67.
19. Artusi S, Nadai M, Perrone R, Biasolo MA, Palu G, Flamand L, Calistri A, Richter SN. The Herpes Simplex Virus-1 genome contains multiple clusters of repeated G-quadruplex: Implications for the antiviral activity of a G-quadruplex ligand. Antiviral Res 2015;118:123-31.
21. Zawatzky R, Hilfenhaus J, Marcucci F, Kirchner H. Experimental infection of inbred mice with herpes simplex virus type 1. I. Investigation of humoral and cellular immunity and of interferon induction. J Gen Virol 1981;53:31-8.
22. Brenner GJ, Cohen N, Moynihan JA. Similar immune response to nonlethal infection with herpes simplex virus-1 in sensitive (BALB/c) and resistant (C57BL/6) strains of mice. Cell Immunol 1994;157:510-24.
23. Simmons A. H-2-linked genes influence the severity of herpes simplex virus infection of the peripheral nervous system. J Exp Med 1989;169:1503-7.
Cite This Article

How to Cite
Chen, X. Y.; Tang, W. J.; Zuo, X. Z.; Wang, G.; Wang, H. X.; Xie, P.; Huang, W. Screening of genetic loci predisposing to herpes simplex virus infection on mouse chromosome 17. Neurosciences. 2016, 3, 274-82. http://dx.doi.org/10.20517/2347-8659.2016.21
Download Citation
Export Citation File:
Type of Import
Tips on Downloading Citation
Citation Manager File Format
Type of Import
Direct Import: When the Direct Import option is selected (the default state), a dialogue box will give you the option to Save or Open the downloaded citation data. Choosing Open will either launch your citation manager or give you a choice of applications with which to use the metadata. The Save option saves the file locally for later use.
Indirect Import: When the Indirect Import option is selected, the metadata is displayed and may be copied and pasted as needed.
About This Article
Special Issue
Copyright
Data & Comments
Data
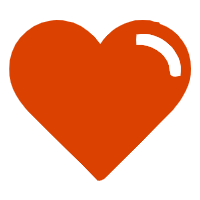
Comments
Comments must be written in English. Spam, offensive content, impersonation, and private information will not be permitted. If any comment is reported and identified as inappropriate content by OAE staff, the comment will be removed without notice. If you have any queries or need any help, please contact us at support@oaepublish.com.