Longitudinal biomarker evaluation in Fabry disease patients receiving lentivirus-mediated gene therapy
Abstract
Aim: In 2016, a team of Canadian researchers initiated the world’s first gene therapy clinical trial for Fabry disease. The study, aiming to determine the safety and toxicity of lentivirus α-galactosidase A transduced autologous CD34+ cells in adult males with Fabry disease (n = 5), was conducted at three Canadian centers. The objective of the present work was to evaluate a profile of Fabry disease-related biomarkers in five participants during a 5-year surveillance period, as part of the evaluation of this novel therapy.
Methods: Sixteen globotriaosylceramide (Gb3) isoforms and eight globotriaosylsphingosine (lyso-Gb3) analogues were measured by LC-MS/MS in plasma and urine at numerous time points before and after gene therapy. Plasma and peripheral blood leukocyte α-galactosidase A activity were also measured.
Results: Levels of urine and plasma lyso-Gb3 and analogues, and urine Gb3 were lower after gene therapy and while treated with enzyme replacement therapy (ERT) compared to baseline (before gene therapy) in participants treated with ERT only. Three participants chose to cease ERT treatment at some point after gene therapy. Two of these patients had lower levels of urine and plasma lyso-Gb3 and analogues compared to baseline, whereas one participant had higher levels of these biomarkers compared to baseline. An increase in urine Gb3 was observed when ERT treatment ceased after gene therapy (P < 0.05) in all three participants.
Conclusion: The evaluation of this complete glycosphingolipid biomarker profile was useful in monitoring the biochemical response to gene therapy in this cohort of five patients with Fabry disease.
Keywords
INTRODUCTION
Fabry disease (OMIM #301500) is a genetic disorder caused by a mutation in the GLA gene located on the X chromosome (position Xq22)[1]. This leads to a deficiency of the lysosomal enzyme α-galactosidase A (EC3.2.1.22), which is involved in the catabolism of glycosphingolipids with terminal α-galactosyl moieties, and to their subsequent systemic accumulation[2]. It is noteworthy to mention that even though Fabry disease is an X-linked disorder, women may also be affected, and sometimes as severely as men[3]. The disease typically progresses gradually, starting in childhood or adolescence, with acroparesthesia, pain crises, hypohidrosis, gastrointestinal problems, proteinuria, and angiokeratomas[4,5]. In adulthood, a multisystemic disorder is on-going where cardiac (left ventricular hypertrophy, myocardial fibrosis, heart failure, arrythmias), renal (proteinuria, low glomerular filtration rate, end-stage renal failure) and neurological (ischemic lesions, transient ischemic attacks, stroke) involvement are also observed[6-9]. Life expectancy of untreated patients with Fabry disease is shortened by 20 years for men and 10 years for women[9], and quality of life is severely impaired. Treatments aimed at restoring enzyme activity are presently approved for Fabry disease. Enzyme replacement therapy (ERT) was approved in 2001 and consists of intravenous infusions of recombinant α-galactosidase A every two weeks, either with agalsidase alfa (Replagal™, Takeda Pharmaceuticals, 0.2 mg/kg body weight) or agalsidase beta (Fabrazyme®, Sanofi, 1.0 mg/kg body weight)[10,11]. Pegunigalsidase alfa (now branded Elfabrio, Protalix Biotherapeutics and Chiesi Global Rare Diseases, 1 mg/kg body weight) has been approved by the U.S. Food and Drug Administration (FDA) and the European Medicines Agency (EMA) to treat adults with Fabry disease[12,13] in 2023. The pharmacological chaperone migalastat (Galafold™, Amicus Therapeutics, 123 mg capsule) was approved in 2016. This medication is taken orally every other day and can be prescribed to patients having mutations amenable to increasing endogenous residual enzyme activity by stabilizing mutant forms of α-galactosidase A in the endoplasmic reticulum and assisting proper trafficking to the lysosome[14].
Research for novel Fabry disease therapies is on-going. A promising avenue is the ex vivo gene therapy approach, where hematopoietic stem cells are harvested from the patient, engineered to express
The objective of the present work was to evaluate this panel of Fabry disease biomarkers measured as part of the Canadian gene therapy clinical trial[16]. Gb3, lyso-Gb3, and their isoforms and analogues were measured in plasma and urine for all five Fabry participants at numerous time points as part of a surveillance and monitoring period of 5 years. The chemical structures of the biomarkers under study are shown in Figure 1. Enzyme activity levels of α-galactosidase A were also measured in plasma and peripheral blood leukocytes for the same duration. To our knowledge, this is the first time that a complete biochemical profile for biomarker evaluation has been performed as part of a gene therapy clinical trial for Fabry disease.
Figure 1. Chemical structures of globotriaosylceramide (Gb3) isoforms, globotriaosylsphingosine (lyso-Gb3), and related analogues. The exact positions of the hydroxyl group on the fatty acid of Gb3(C24:OH), and of the double bond on the fatty acids of Gb3(C16:1),
METHODS
Ethics approval
The biomarker study was conducted in agreement with the Declaration of Helsinki and approved by the Institutional Review Board at the Faculty of Medicine and Health Sciences at the Centre intégré universitaire de santé et de services sociaux de l’Estrie-Centre hospitalier universitaire de Sherbrooke (CIUSSS de l’Estrie-CHUS), where the biomarker analyses were performed (Project #2017-1575), authorization obtained on
Reagents
The internal standard (IS) of lyso-Gb3 and related analogues (N-glycinated lyso-Gb3, or lyso-Gb3-Gly) was synthesized in-house as previously described[29]. Lyso-Gb3, [Gb3(d18:1)(C17:0)], [Gb3(d18:1)(C18:0)D3], and [Gb3(d18:1)(C23:0)] were bought from Matreya LLC (State College, PA, USA). HPLC-grade acetonitrile, and methyl tert-butyl ether (MTBE) were obtained from MilliporeSigma (Darmstadt, Germany). Formic acid (99+% purity) was from Acros Organics (Morris Plains, NJ, USA), while A.C.S. grade o-phosphoric acid (85% purity), ammonium hydroxide (29%), and Optima LC/MS grade water and methanol were from Fisher Scientific (Fair Lawn, NJ, USA).
Study participants
Biomarkers were evaluated in all five participants from the Fabry disease gene therapy clinical trial (NCT02800070). Eligibility criteria at baseline were previously described in detail[16]. Briefly, recruitment was restricted to men aged 18 to 50 years with a confirmed classical Fabry disease phenotype (< 7.0% or absent α-galactosidase A activity in plasma or leukocytes) on ERT for at least 6 months and with adequate organ function. Fabry symptoms at baseline and treatment phase outcomes were previously described[16]. GLA mutations and age at transplant were the following: Participant #1: p.Gln321Arg, 48 years;
Conduct of the study and interventions
This gene therapy clinical trial was subdivided into five distinct phases that were previously described[16]. Briefly, ERT was stopped for a minimum of 30 days before gene therapy. Levels of α-galactosidase A activity were then assessed to establish baseline measurements. During the mobilization phase, peripheral blood CD34+ hematopoietic stem and progenitor cells (HSPCs) were mobilized at the regional stem cell center using filgrastim (Participants #1 and #5) or a combination of filgrastim and plerixafor (Participants #2, #3, and #4) as mobilizing agents, followed by leukapheresis. CD34+ selected cells were transduced with lentiviral α-galactosidase A and cryopreserved while waiting for the certificate of analysis approval to be obtained from Health Canada (approximately 30 days). The day before infusion of autologous CD34+ transduced cells, participants received melphalan IV (100 mg/m2). Safety review of participant data and a return to ERT were performed 1 month post-transplant, except for Participant #3, who chose not to restart ERT. ERT for the other participants was paused after at least 6 months post-transplant if certain clinical endpoints were reached and patients consented to this change in procedure. Participants were followed for a period of 5 years. Urine and plasma specimens were collected for biomarker evaluation at different time points as part of this follow-up period. Figure 2 shows the timeline for each participant, including the urine and plasma specimen collection time points, the periods where the participants were treated with ERT, and the type of ERT treatment. Participant #1 was treated with Replagal up to one month before transplant, then with Fabrazyme from 1 to 18 months post-transplant, and then ERT treatment was stopped. Participants #2 and #5 were treated with Fabrazyme up to one month before transplant and resumed Fabrazyme at 1 month post-transplant until the end of the 5-year follow-up. Participant #3 was treated with Replagal up to one month before transplant, and never resumed ERT after transplant. Finally, participant #4 was treated with Fabrazyme up to one month before transplant, and then with Fabrazyme from 1 to 7 months post-transplant when ERT treatment was stopped.
Sample collection
For each time point, 4 milliliters (mL) of blood was collected in a lavender (potassium EDTA) vacutainer blood collection tube and 15 mL of urine was collected in a urine specimen container. Immediately after collection, blood specimens were centrifuged for 10 min at 2 000× g (room temperature), and the plasma fraction was transferred into a polypropylene tube. Urine and plasma specimens were kept frozen
Biomarker analyses
Methylated and non-methylated Gb3 isoforms in urine
Regarding the analysis of Gb3 isoforms in urine, we used a quantitative assay that was devised according to the metabolomic study published by our group[26]. This urine assay was devised on a newer generation of mass spectrometer that allowed the analysis of more molecules simultaneously without compromising the sensitivity and reproducibility of the results. It is noteworthy to mention that after performing urinary metabolomic studies with a time-of-flight mass spectrometry system, we discovered different groups of Gb3 biomarkers[25,26] for Fabry disease. Among these groups, we detected methylated and non-methylated Gb3 isoforms. The main difference between these two groups is that methylated Gb3 isoforms (n = 7) have a chemical structure comprising different fatty-acid chains where the methylation is on the nitrogen atom [see Figure 1]. The exact position of the methyl group was determined by mass spectrometry[26]. In fact, we found that the isoform Gb3(d18:1)(C22:0)Me had fragments at m/z 296.3 on the methylated sphingosine, and at m/z 354.4 on the methylated fatty acid. These studies thus confirmed that the methylation process is on the nitrogen atom shared by the sphingosine and the fatty acid fragments[26]. Moreover, it has been reported in the literature that methyltransferases catalyze methylation for different biological processes[30-33], thus confirming these methylated molecules.
Seven methylated Gb3 isoforms [Gb3(d18:1)(C16:0)Me; Gb3 (d18:1)(C18:0)Me; Gb3(d18:1)(C20:0)Me;
Total Gb3 in plasma
Regarding the selection of plasma Gb3 isoforms, we used an assay that was already devised and validated in our laboratory, thus readily available, when the gene therapy clinical trial started. This specific assay was devised for the quantification of the most abundant Gb3 isoforms in plasma.
Six Gb3 isoforms [Gb3(d18:1)(C16:1); Gb3(d18:1)(C16:0); Gb3(d18:1)(C18:0); Gb3(d18:1)(C22:0);
Lyso-Gb3 and related analogues in urine and in plasma
Two previous metabolomic studies[23,24] led us to detect a total of 8 analogues of lyso-Gb3 in urine and plasma specimens of patients with Fabry disease. Lyso-Gb3 (-12) and lyso-Gb3 (+14) were only detected in urine specimens, while lyso-Gb3 (+18) was only detected in plasma specimens. It is noteworthy to mention that the abundance of these analogues varies significantly between these two matrices, as shown in Supplementary Figures 1 and 2.
Lyso-Gb3 and related analogues in urine
Lyso-Gb3 and seven related analogues (lyso-Gb3 -28 Da; lyso-Gb3 -12 Da; lyso-Gb3 -2 Da; lyso-Gb3 +14 Da; lyso-Gb3 +16 Da; lyso-Gb3 +34 Da; and lyso-Gb3 +50 Da) were analyzed in urine by UHPLC-MS/MS following a solid phase extraction (SPE) purification procedure as previously described[29,36]. Tandem mass spectrometry MRM transitions are shown in Supplementary Table 1. Standards of lyso-Gb3 and
Lyso-Gb3 and related analogues in plasma
Lyso-Gb3 and six related analogues (lyso-Gb3 -28 Da; lyso-Gb3 -2 Da; lyso-Gb3 +16 Da; lyso-Gb3 +18 Da; lyso-Gb3 +34 Da; and lyso-Gb3 +50 Da) were analyzed in plasma specimens by UHPLC-MS/MS following a SPE purification procedure as previously described[37]. Tandem mass spectrometry MRM transitions are shown in Supplementary Table 1. Standards of lyso-Gb3 and lyso-Gb3-Gly were used for calibration and IS, respectively. The reference values were “not detected”, except for lyso-Gb3 (≤ 2.4 nM), lyso-Gb3 -2 Da
α-galactosidase a enzyme assay
The α-galactosidase A assay uses a fluorescent artificial substrate 4-methylumbelliferyl-alpha-D-galactopyranoside based on the method previously described by Desnick et al.[38]. The normal range for
Statistical analyses
Statistical analyses and longitudinal follow-up graphs were generated using GraphPad Prism 9.5.1. (GraphPad Software, San Diego, CA, USA). Each biomarker was evaluated at two different periods: Enzyme Replacement Therapy-Gene Therapy (ERT-GT) and only Gene Therapy (GT) compared to the baseline period. The baseline value (BV) was defined as the mean of the biomarker values obtained at each time point in the baseline period, which is the pre-GT period when the participant was treated with ERT (-4 to
Deltas were defined as the difference between BV and biomarker values obtained at each time point collected during a specific period, expressed as a percentage of BV. Despite a small sample, normality of the population distribution was assumed, and therefore CI were based on a Student’s distribution. P-values were obtained using a two-sided one-sample Student’s t-test to conclude for an elevation, reduction, or stability (mean delta compared to the theoretical value of 0). For all analyses, statistical significance was established at P < 0.05.
RESULTS
The complete biomarker study dataset, including the individual measurements of the different Gb3 isoforms and lyso-Gb3 analogues, is available in Supplementary Table 2. The longitudinal follow-ups of individual lyso-Gb3 analogue measurements are shown for urine and plasma in Supplementary Figures 1 and 2, respectively. The longitudinal follow-ups of individual Gb3 isoforms are shown for plasma in
Mean deltas from baseline values (BV) for biomarkers
The mean deltas from baseline values (BV) for biomarkers are shown in Figure 3 for all Fabry participants.
Figure 3. Mean deltas from baseline value and 95% confidence intervals (CI) in the periods after transplant where participants were treated with enzyme replacement therapy (ERT-GT period) or not (GT period). The baseline value (BV) was defined as the mean of the biomarker values obtained at each time point in the baseline period, which is the pre-GT period when the participant was treated with ERT (-4 to -1 month). *P value < 0.05).
Longitudinal biomarker follow-up for 5 Fabry patients
Supplementary Figure 6 shows combined longitudinal results for all 5 patients for each biomarker under study.
The longitudinal follow-ups of the biomarkers under study over the gene therapy timeline, where transplant is day 0, are shown in Figure 4 (Participant 1), Figure 5 (Participant 2), Figure 6 (Participant 3), Figure 7 (Participant 4), and Figure 8 (Participant 5) and expressed as the %difference from the screening period, except for the enzyme activity measured in leukocytes and plasma which is expressed as nmol/hr/mg protein, and nmol/hr/mL, respectively. The period from 1-month pre-GT to 1-month post-GT will not be discussed, considering the medical procedures involved during this period which might have introduced more variability in the biomarker measurements.
Participant #1
Urine and plasma lyso-Gb3 and total analogues show similar profiles [Figure 4A-D]. As expected, the lowest levels were observed during the ERT-GT period [Figure 3], when participant #1 was treated with both Fabrazyme and gene therapy (n = 5 time points). During this period, mean urine lyso-Gb3 levels were at
Plasma and leukocyte α-galactosidase A activities are shown in Figure 4G. The plasma activity was
Participant #2
Urine and plasma lyso-Gb3 and total analogues show similar profiles [Figure 5A-D]. As expected, the lowest levels were observed during the ERT-GT period [Figure 3], when participant #2 was treated with both Fabrazyme and gene therapy (1-60 months post-GT, n = 9 time points). During this period, mean urine lyso-Gb3 levels were at -55.5% ± 25.4% (P < 0.050), and mean urine analogues were at
Plasma and leukocyte α-galactosidase A activities are shown in Figure 5G. The plasma activity was
Participant #3
Urine and plasma lyso-Gb3 and total analogues show similar profiles [Figure 6A-D]. It is noteworthy to mention that participant #3 chose not to resume ERT treatment after gene therapy [Figure 3]. During the period from 1-60 months post-GT (GT period, n = 10 time points), mean urine lyso-Gb3 levels were at
Plasma and leukocyte α-galactosidase A activities are shown in Figure 6G. The plasma activity was
Participant #4
Urine and plasma lyso-Gb3 and total analogues show similar profiles [Figure 7A-D]. As expected, the lowest levels were observed during the ERT-GT period, when participant #4 was treated with both Fabrazyme and gene therapy (3-6 months post-GT, n = 2 time points) [Figure 3]. During this period, mean urine lyso-Gb3 levels were at -28.0% ± 203.3% (P = 0.331), and mean urine analogues were at -24.0% ± 12.7% (P < 0.050) compared to BV (ERT only, n = 3 time points). On the other hand, mean plasma lyso-Gb3 levels were at
Plasma and leukocyte α-galactosidase A activities are shown in Figure 7G. The plasma activity was
Participant #5
Urine and plasma lyso-Gb3 and total analogues show similar profiles [Figure 8A-D]. As expected, the lowest levels were observed during the ERT-GT period, when participant #5 was treated with both Fabrazyme and gene therapy (3-60 months post-GT, n = 8 time points) [Figure 3]. During this period, mean urine lyso-Gb3 levels were at -75.5% ± 18.7% (P < 0.001), and mean urine analogues were at -57.9% ± 17.3% (P < 0.001) compared to BV (ERT only, n = 4 time points). On the other hand, mean plasma lyso-Gb3 levels were at
Plasma and leukocyte α-galactosidase A activities are shown in Figure 8G. The plasma activity was
DISCUSSION
A longitudinal biomarker follow-up was performed in a cohort of five participants who were treated as part of the world’s first gene therapy clinical trial for Fabry disease at three Canadian centers. Figure 3 summarizes the biomarker results obtained as part of this study. It is noteworthy to mention that, considering that the participants under study had classical mutations, most biomarkers remained above normal reference values, even after treatment with ERT and/or gene therapy (see Supplementary Table 2). Supplementary Figure 1 shows that the analogues are more elevated than lyso-Gb3 itself in urine specimens. We would like to emphasize that the evaluation of the complete profile of lyso-Gb3 and analogues is particularly important in this matrix.
The levels of urine and plasma lyso-Gb3 and analogues, as well as urine Gb3, were lower compared to baseline (ERT alone) when participants were treated with both ERT and gene therapy
Biomarker levels were also evaluated in three participants who discontinued ERT treatment at some point after gene therapy (Participant #1, Participant #3, and Participant #4). When ERT treatment was stopped post-GT, the levels of urine and plasma lyso-Gb3 and analogues remained lower than baseline (ERT alone) for Participant #1 (not statistically significant for urine lyso-Gb3 and analogues, P < 0.050 for plasma lyso-Gb3 and analogues) and Participant #3 (P < 0.050). For Participant #4, urine and plasma lyso-Gb3 and analogues were either stable (urine lyso-Gb3, P > 0.050), or elevated compared to baseline values while treated with ERT alone (P ≤ 0.050). This is consistent with the fact that the diminution of biomarker levels was less important for this participant when treated with both ERT and gene therapy. Plasma Gb3 values remained stable (P > 0.050) for Participants #1 and #4, while a statistically significant diminution was observed for Participant #3 (-12.9% ± 9.6%, P < 0.050). In all three participants who stopped ERT treatment post-GT, an important augmentation of urine Gb3 was observed compared to baseline values
The reason for the augmentation of urine Gb3 in these participants is unclear. We do know from previous studies that Gb3 accumulates in virtually all renal cell types, with particularly important accumulations in podocytes and distal tubular epithelial cells[39]. The exfoliation of kidney tubular cells contributes to the presence of Gb3 in voided urine specimens[40]. Urine Gb3 might also originate from the shedding of podocytes or from the leakage of circulating Gb3 through renal glomeruli[41]. It would thus be plausible that the augmentation of urine Gb3 reflects an increased accumulation of Gb3 in different renal cell types following treatment switch from ERT to gene therapy in the present study. In fact, it was previously shown that Gb3 was cleared or decreased in several renal cell types following the administration of ERT for a period of 11 months[39]. A potential increased accumulation of Gb3 in podocytes would also exacerbate their detachment into the urine (podocyturia)[42,43]. This phenomenon would be in accordance with an increased measured level of urine Gb3. Nevertheless, the prognostic value of urine Gb3 as a biomarker for kidney disease progression is debatable. Shiga et al. found higher levels of urine Gb3 in patients with Fabry disease showing renal events (proteinuria, elevated serum creatinine, or decreased eGFR) and suggested closely monitoring patients without any renal symptoms but with high levels of urinary Gb3 to prevent kidney function deterioration[44]. On the other hand, other researchers did not find any correlations between urinary Gb3 levels and renal function parameters such as albuminuria, eGFR, serum creatinine, and proteinuria[45,46]. Regarding the participants of the present study, the increased levels of urine Gb3 were not associated with a decrease in eGFR. Nevertheless, this finding warrants further investigations and close monitoring of renal function with known predictors of kidney disease progression (proteinuria, albuminuria, and serum creatinine) in the participants who stopped ERT after gene therapy treatment. In fact, the clinical outcomes at 5 years for the five participants from this gene therapy clinical trial and their relationship with biomarker changes are currently under evaluation and will provide more answers regarding these increased urinary Gb3 levels and kidney disease status.
Nowak et al. have found that serum lyso-Gb3 levels were associated with kidney function, renal replacement therapy, cardiomyopathy, and stroke/transient ischemic attacks and that it was a significant risk factor associated with important clinical events[47,48]. Considering that lyso-Gb3 levels tend to increase with disease severity[27,47-49], it can be hypothesized that the stability or decrease of this biomarker and its analogues in plasma and urine after discontinuation of ERT is important and shows the benefits and success of gene therapy treatment. In fact, toxic effects of lyso-Gb3 exposure were previously reported and include alteration of pathways involved in protein translation and folding[50], induction of necroptosis via autophagy[51], release promotion of secondary mediators of glomerular injury[52], and sensitization of peripheral nociceptive neurons[53]. Moreover, van der Veen et al. recently showed that, in untreated patients with Fabry disease, plasma lyso-Gb3 eventually reached a stable level during childhood and that this level was associated with the course of disease progression[54]. Ouyang et al. also found that lyso-Gb3 measurements could improve the prediction of kidney disease progression in patients with Fabry disease[55]. Our group showed the association of lyso-Gb3 and some related analogues with the LVMI and MSSI in patients with a late-onset IVS4+919G>A cardiac variant mutation in Taiwan[27]. It was previously shown that serum lyso-Gb3 and lyso-Gb3 analogues varied according to the type of mutations and related phenotype[56,57]. A group of experts recently recommended routine monitoring of plasma lyso-Gb3 at baseline and every 6 to 12 months as part of treatment initiation/switch for longitudinal level evaluations, considering that the reduction in lyso-Gb3 levels seems important[58]. Participants #1 and #3 had lower levels of plasma and urine lyso-Gb3 and analogues when treated with gene therapy compared to previous levels under ERT treatment alone. It was not the case for participant #4, for whom an increase was observed after ERT treatment discontinuation. Considering the lowest levels of urine and plasma lyso-Gb3 and analogues obtained under both ERT and gene therapy treatments, it might be hypothesized that the combination of these two treatments gives the best outcomes in patients affected with Fabry disease. This observation warrants further investigation.
The strength of this study is the analysis of a unique and extensive profile of sixteen Gb3 isoforms in urine, six Gb3 isoforms in plasma, six lyso-Gb3 analogues in plasma, and seven lyso-Gb3 analogues in the urine of participants. These mass spectrometry analyses were performed using validated methodologies for maximum accuracy and precision. This complete profile of Fabry disease-related glycosphingolipids was never reported before in adults with Fabry disease as part of gene therapy treatment. One limitation of this study was the low number of participants included in the research. The variability in ERT treatments before and after gene therapy for each participant was also a confounding variable. As shown in Figure 2, some participants were treated with Fabrazyme, and others with Replagal at baseline. This also implies different dosages between these two ERT treatments. Participant #1 was on Replagal before gene therapy and resumed ERT with Fabrazyme, while Participant #2 never stopped ERT after gene therapy, and
In summary, high sensitivity and specificity mass spectrometry assays enabled the analysis of a complete biochemical profile of Fabry disease-related biomarkers in urine and plasma, leading to better monitoring and follow-up of these treated patients as part of a precision medicine approach. The biochemical profile also helped learn more about the complex pathophysiology involved in Fabry patients. Future perspectives will focus on correlation studies between these biomarkers and the clinical manifestations of the disease.
DECLARATIONS
Acknowledgments
The authors would like to thank Fabry disease patients who participated in this study. We are also grateful to Waters Corp. for their scientific support and partnership through the Waters Centre of Innovation, directed by Christiane Auray-Blais. We would like to acknowledge the work of Michel Boutin, Mohamed Gamrani, and Georges Kabala Ntumba for their technical and scientific expertise. We thank Cindy Yau, Rupi Mangat, Sarah Young, and Pam Degendorfer from Ozmosis Research Inc. for their support of the trial.
Authors’ contributions
Conceptualization, funding acquisition, resources: Medin JA, Auray-Blais C
Methodology, visualization: Martineau T, Lavoie P
Validation, formal analysis, investigation: Auray-Blais C, Martineau T, Lavoie P
Data curation: Auray-Blais C, Martineau T, Lavoie P, Medin JA
Writing original draft preparation: Lavoie P
Writing-review: Auray-Blais C, Lavoie P, Martineau T
Supervision, project administration, technical and material support: Auray-Blais C
Editing: Auray-Blais C, Lavoie P, Martineau T, Rupar CA, Barber DL, Keating A, Foley R, Khan A, West ML, Medin JA
All authors have read and agreed to the published version of the manuscript.
Availability of data and materials
Mass spectrometry data supporting these findings are provided in Supplementary Material and are stored into secured data repositories at the Faculty of Medicine and Health Sciences at the Université de Sherbrooke, Sherbrooke, Quebec. All enzyme activity results were stored in Dr T Rupar’s office in London, ON.
Financial support and sponsorship
This research study was funded by the Canadian Institutes of Health Research (CIHR, grant number 119187), The Kidney Foundation of Canada, AVROBIO, Inc., and research funds from Auray-Blais C.
Conflicts of interest
All authors declared that there are no conflicts of interest.
Ethical approval and consent to participate
The biomarker study was conducted in agreement with the Declaration of Helsinki and approved by the Institutional Review Board at the Faculty of Medicine and Health Sciences at the Centre intégré universitaire de santé et de services sociaux de l’Estrie-Centre hospitalier universitaire de Sherbrooke (CIUSSS de l’Estrie-CHUS), where the biomarker analyses were performed (Project #2017-1575), authorization obtained on November 14th, 2016). The trial (NCT02800070) was approved by Health Canada on April 26th, 2016, and conducted in compliance with local institutional and/or university Human Experimentation Committee requirements. Research Ethics Board (REB) approval was provided by University Health Network REB, Alberta Health Services REB, Capital Health Services REB, Hamilton Integrated Research Ethics, and the Medical College of Wisconsin Institutional Review Board.
Consent for publication
Informed consent was obtained from all subjects involved in the study.
Copyright
© The Author(s) 2024.
Supplementary Materials
REFERENCES
1. Kok K, Zwiers KC, Boot RG, Overkleeft HS, Aerts JMFG, Artola M. Fabry disease: molecular basis, pathophysiology, diagnostics and potential therapeutic directions. Biomolecules 2021;11:271.
2. Ryckman AE, Brockhausen I, Walia JS. Metabolism of glycosphingolipids and their role in the pathophysiology of lysosomal storage disorders. Int J Mol Sci 2020;21:6881.
3. Perretta F, Antongiovanni N, Jaurretche S. Major organic involvement in women with fabry disease in argentina. Sci World J 2018;2018:6515613.
4. Ramaswami U, Whybra C, Parini R, et al. Clinical manifestations of Fabry disease in children: data from the Fabry outcome survey. Acta Paediatr 2006;95:86-92.
5. Caputo F, Lungaro L, Galdi A, et al. Gastrointestinal involvement in anderson-Fabry disease: a narrative review. Int J Environ Res Public Health 2021;18:3320.
6. Pieroni M, Moon JC, Arbustini E, et al. Cardiac involvement in Fabry disease: JACC review topic of the week. J Am Coll Cardiol 2021;77:922-36.
7. Silva CAB, Moura-Neto JA, Dos Reis MA, Vieira Neto OM, Barreto FC. Renal manifestations of Fabry disease: a narrative review. Can J Kidney Health Dis 2021;8:2054358120985627.
8. Mishra V, Banerjee A, Gandhi AB, et al. Stroke and Fabry disease: a review of literature. Cureus 2020;12:e12083.
10. Kant S, Atta MG. Therapeutic advances in Fabry disease: the future awaits. Biomed Pharmacother 2020;131:110779.
12. EMA. Elfabrio. Available from: https://www.ema.europa.eu/en/medicines/human/EPAR/elfabrio [Last accessed on 21 Jun 2024].
13. FDA. Available from: https://www.accessdata.fda.gov/drugsatfda_docs/label/2023/761161s000lbl.pdf [Last accessed on 21 Jun 2024].
15. Felis A, Whitlow M, Kraus A, Warnock DG, Wallace E. Current and investigational therapeutics for Fabry disease. Kidney Int Rep 2020;5:407-13.
16. Khan A, Barber DL, Huang J, et al. Lentivirus-mediated gene therapy for Fabry disease. Nat Commun 2021;12:1178.
17. Simonetta I, Tuttolomondo A, Daidone M, Pinto A. Biomarkers in anderson-Fabry disease. Int J Mol Sci 2020;21:8080.
18. Carnicer-Cáceres C, Arranz-Amo JA, Cea-Arestin C, et al. Biomarkers in Fabry disease. Implications for clinical diagnosis and follow-up. J Clin Med 2021;10:1664.
19. Bengtsson BA, Johansson JO, Hollak C, Linthorst G, FeldtRasmussen U. Enzyme replacement in anderson-Fabry disease. Lancet 2003;361:352.
20. Schiffmann R, Kopp JB, Austin HA 3rd, et al. Enzyme replacement therapy in Fabry disease: a randomized controlled trial. JAMA 2001;285:2743-9.
21. Eng CM, Guffon N, Wilcox WR, et al. Safety and efficacy of recombinant human alpha-galactosidase a replacement therapy in Fabry’s disease. N Engl J Med 2001;345:9-16.
22. Aerts JM, Groener JE, Kuiper S, et al. Elevated globotriaosylsphingosine is a hallmark of Fabry disease. Proc Natl Acad Sci USA 2008;105:2812-7.
23. Dupont FO, Gagnon R, Boutin M, Auray-Blais C. A metabolomic study reveals novel plasma lyso-Gb3 analogs as Fabry disease biomarkers. Curr Med Chem 2013;20:280-8.
24. Auray-Blais C, Boutin M, Gagnon R, Dupont FO, Lavoie P, Clarke JT. Urinary globotriaosylsphingosine-related biomarkers for Fabry disease targeted by metabolomics. Anal Chem 2012;84:2745-53.
25. Manwaring V, Boutin M, Auray-Blais C. A metabolomic study to identify new globotriaosylceramide-related biomarkers in the plasma of Fabry disease patients. Anal Chem 2013;85:9039-48.
26. Auray-Blais C, Boutin M. Novel Gb3 isoforms detected in urine of Fabry disease patients: a metabolomic study. Curr Med Chem 2012;19:3241-52.
27. Auray-Blais C, Lavoie P, Boutin M, et al. Biomarkers associated with clinical manifestations in Fabry disease patients with a late-onset cardiac variant mutation. Clin Chim Acta 2017;466:185-93.
28. Auray-Blais C, Blais CM, Ramaswami U, et al. Urinary biomarker investigation in children with Fabry disease using tandem mass spectrometry. Clin Chim Acta 2015;438:195-204.
29. Lavoie P, Boutin M, Auray-Blais C. Multiplex analysis of novel urinary lyso-Gb3-related biomarkers for Fabry disease by tandem mass spectrometry. Anal Chem 2013;85:1743-52.
30. Finkelstein JD. Metabolic regulatory properties of S-adenosylmethionine and S-adenosylhomocysteine. Clin Chem Lab Med 2007;45:1694-9.
31. Lohse B, Kristensen JL, Kristensen LH, et al. Inhibitors of histone demethylases. Bioorg Med Chem 2011;19:3625-36.
32. Bai HW, Shim JY, Yu J, Zhu BT. Biochemical and molecular modeling studies of the O-methylation of various endogenous and exogenous catechol substrates catalyzed by recombinant human soluble and membrane-bound catechol-O-methyltransferases. Chem Res Toxicol 2007;20:1409-25.
33. Patti GJ, Yanes O, Shriver LP, et al. Metabolomics implicates altered sphingolipids in chronic pain of neuropathic origin. Nat Chem Biol 2012;8:232-4.
34. Abaoui M, Boutin M, Lavoie P, Auray-Blais C. High-risk screening of Fabry disease: analysis of fifteen urinary methylated and non-methylated Gb3 isoforms using tandem mass spectrometry. Curr Protoc Hum Genet 2016;91:17.24.1-11.
35. Abaoui M, Boutin M, Lavoie P, Auray-Blais C. Tandem mass spectrometry multiplex analysis of methylated and non-methylated urinary Gb3 isoforms in Fabry disease patients. Clin Chim Acta 2016;452:191-8.
36. Lavoie P, Boutin M, Abaoui M, Auray-Blais C. Fabry disease biomarkers: analysis of urinary lyso-Gb3 and seven related analogs using tandem mass spectrometry. Curr Protoc Hum Genet 2016;90:17.22.1-12.
37. Boutin M, Lavoie P, Abaoui M, Auray-Blais C. Tandem mass spectrometry quantitation of lyso-Gb3 and six related analogs in plasma for Fabry disease patients. Curr Protoc Hum Genet 2016;90:17.23.1-9.
38. Desnick RJ, Allen KY, Desnick SJ, Raman MK, Bernlohr RW, Krivit W. Fabry’s disease: enzymatic diagnosis of hemizygotes and heterozygotes. Alpha-galactosidase activities in plasma, serum, urine, and leukocytes. J Lab Clin Med 1973;81:157-71.
39. Thurberg BL, Rennke H, Colvin RB, et al. Globotriaosylceramide accumulation in the Fabry kidney is cleared from multiple cell types after enzyme replacement therapy. Kidney Int 2002;62:1933-46.
40. Chatterjee S, Gupta P, Pyeritz RE, Kwiterovich PO Jr. Immunohistochemical localization of glycosphingolipid in urinary renal tubular cells in Fabry’s disease. Am J Clin Pathol 1984;82:24-8.
41. Schiffmann R, Waldek S, Benigni A, Auray-Blais C. Biomarkers of Fabry disease nephropathy. Clin J Am Soc Nephrol 2010;5:360-4.
42. Fall B, Scott CR, Mauer M, et al. Urinary podocyte loss is increased in patients with Fabry disease and correlates with clinical severity of Fabry nephropathy. PLoS One 2016;11:e0168346.
43. Najafian B, Tøndel C, Svarstad E, Gubler MC, Oliveira JP, Mauer M. Accumulation of Globotriaosylceramide in podocytes in Fabry nephropathy is associated with progressive podocyte loss. J Am Soc Nephrol 2020;31:865-75.
44. Shiga T, Tsukimura T, Namai Y, Togawa T, Sakuraba H. Comparative urinary globotriaosylceramide analysis by thin-layer chromatography-immunostaining and liquid chromatography-tandem mass spectrometry in patients with Fabry disease. Mol Genet Metab Rep 2021;29:100804.
45. Moura AP, Hammerschmidt T, Deon M, Giugliani R, Vargas CR. Investigation of correlation of urinary globotriaosylceramide (Gb3) levels with markers of renal function in patients with Fabry disease. Clin Chim Acta 2018;478:62-7.
46. Vedder AC, Linthorst GE, van Breemen MJ, et al. The Dutch Fabry cohort: diversity of clinical manifestations and Gb3 levels. J Inherit Metab Dis 2007;30:68-78.
47. Nowak A, Mechtler TP, Hornemann T, et al. Genotype, phenotype and disease severity reflected by serum LysoGb3 levels in patients with Fabry disease. Mol Genet Metab 2018;123:148-53.
48. Nowak A, Beuschlein F, Sivasubramaniam V, Kasper D, Warnock DG. Lyso-Gb3 associates with adverse long-term outcome in patients with Fabry disease. J Med Genet 2022;59:287-93.
49. Niemann M, Rolfs A, Störk S, et al. Gene mutations versus clinically relevant phenotypes: lyso-Gb3 defines Fabry disease. Circ Cardiovasc Genet 2014;7:8-16.
50. Nikolaenko V, Warnock DG, Mills K, Heywood WE. Elucidating the toxic effect and disease mechanisms associated with Lyso-Gb3 in Fabry disease. Hum Mol Genet 2023;32:2464-72.
51. Hwang AR, Park S, Woo CH. Lyso-globotriaosylsphingosine induces endothelial dysfunction via autophagy-dependent regulation of necroptosis. Korean J Physiol Pharmacol 2023;27:231-40.
52. Sanchez-Niño MD, Sanz AB, Carrasco S, et al. Globotriaosylsphingosine actions on human glomerular podocytes: implications for Fabry nephropathy. Nephrol Dial Transplant 2011;26:1797-802.
53. Choi L, Vernon J, Kopach O, et al. The Fabry disease-associated lipid Lyso-Gb3 enhances voltage-gated calcium currents in sensory neurons and causes pain. Neurosci Lett 2015;594:163-8.
54. van der Veen SJ, Sayed ME, Hollak CEM, et al. Early risk stratification for natural disease course in Fabry patients using plasma globotriaosylsphingosine levels. Clin J Am Soc Nephrol 2023;18:1272-82.
55. Ouyang Y, Zhang W, Zhao Z, et al. Globotriaosylsphingosine improves risk stratification of kidney progression in Fabry disease patients. Clin Chim Acta 2024;556:117851.
56. Duro G, Zizzo C, Cammarata G, et al. Mutations in the GLA gene and LysoGb3: is it really anderson-Fabry disease? Int J Mol Sci 2018;19:3726.
57. Ferreira S, Auray-Blais C, Boutin M, et al. Variations in the GLA gene correlate with globotriaosylceramide and globotriaosylsphingosine analog levels in urine and plasma. Clin Chim Acta 2015;447:96-104.
Cite This Article
Export citation file: BibTeX | EndNote | RIS
OAE Style
Auray-Blais C, Lavoie P, Martineau T, Rupar CA, Barber DL, Keating A, Foley R, Khan A, West ML, Medin JA. Longitudinal biomarker evaluation in Fabry disease patients receiving lentivirus-mediated gene therapy. Rare Dis Orphan Drugs J 2024;3:25. http://dx.doi.org/10.20517/rdodj.2024.14
AMA Style
Auray-Blais C, Lavoie P, Martineau T, Rupar CA, Barber DL, Keating A, Foley R, Khan A, West ML, Medin JA. Longitudinal biomarker evaluation in Fabry disease patients receiving lentivirus-mediated gene therapy. Rare Disease and Orphan Drugs Journal. 2024; 3(3): 25. http://dx.doi.org/10.20517/rdodj.2024.14
Chicago/Turabian Style
Christiane Auray-Blais, Pamela Lavoie, Tristan Martineau, C. Anthony Rupar, Dwayne L. Barber, Armand Keating, Ronan Foley, Aneal Khan, Michael L. West, Jeffrey A. Medin. 2024. "Longitudinal biomarker evaluation in Fabry disease patients receiving lentivirus-mediated gene therapy" Rare Disease and Orphan Drugs Journal. 3, no.3: 25. http://dx.doi.org/10.20517/rdodj.2024.14
ACS Style
Auray-Blais, C.; Lavoie P.; Martineau T.; Rupar CA.; Barber DL.; Keating A.; Foley R.; Khan A.; West ML.; Medin JA. Longitudinal biomarker evaluation in Fabry disease patients receiving lentivirus-mediated gene therapy. Rare. Dis. Orphan. Drugs. J. 2024, 3, 25. http://dx.doi.org/10.20517/rdodj.2024.14
About This Article
Special Issue
Copyright
Data & Comments
Data
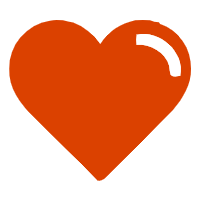
Comments
Comments must be written in English. Spam, offensive content, impersonation, and private information will not be permitted. If any comment is reported and identified as inappropriate content by OAE staff, the comment will be removed without notice. If you have any queries or need any help, please contact us at support@oaepublish.com.