Neuropathy and pain in Fabry disease
Abstract
Fabry disease (FD) is a multiorgan lysosomal storage disorder caused by mutations in the alfa-galactosidase A
Keywords
INTRODUCTION
Fabry disease (FD) is an X-linked hereditary lysosomal storage disorder with an estimated prevalence of 1 in 1,368 to 1 in 8,882[1]. FD is caused by genetic alterations in the gene encoding alfa-galactosidase A (GLA), which is located on chromosome Xq21 and consists of five exons. To date, over 1000 GLA mutations[2] have been identified, which may lead to an impairment or complete loss of GLA enzyme activity. The degree of GLA impairment is defined by the pathogenicity of the respective genetic mutation, which may range from apathogenic to pathogenic[3]. Since GLA is a key enzyme involved in the cleavage of sphingolipids, impairment in its function leads to the accumulation of Gb3 in lysosomes within cells of different tissues. An increase in cellular Gb3 load is pathognomonic for FD; however, the role of Gb3 in disease development remains unclear. Current concepts highlight lysosomal dysfunction as the main driver of FD pathophysiology rather than the accumulation of Gb3[4]. Despite X-chromosomal inheritance, women are also affected by FD and may reach all levels of disease severity[5,6]. Intravenous enzyme replacement therapy (ERT) via recombinant agalsidase alfa or agalsidase beta has been available for FD-specific treatment since 2001[7,8] and the chaperone migalastat as the first oral treatment since 2016[9]. Pain is one of the earliest FD symptoms and can begin as early as childhood[10,11]. While the exact mechanisms of pain pathophysiology in FD are incompletely understood, impairment of the small caliber thinly-myelinated A-delta and unmyelinated C nerve fibers is a key factor[12,13]. In this review, we summarize current knowledge on the clinical phenotype, pathophysiology, and treatment options of FD-associated neuropathy and pain, and provide an outlook into novel approaches to improve scientific insight and patient management.
NEUROPATHY IN FD
In FD, mainly the thinly-myelinated A-delta and unmyelinated C nerve fibers, i.e. the small nerve fibers, are affected[14,15]. Neuropathy of the large nerve fibers in terms of polyneuropathy with a sensorimotor clinical phenotype is rare and rather occurs as a complication of advanced nephropathy in FD patients suffering from chronic kidney disease and uremia[16,17]. In FD patients with small fiber neuropathy (SFN), the clinical phenotype is characterized by thermal hypoesthesia. Sensory profiles of FD patients, e.g., established using quantitative sensory testing (QST), mainly show an impairment in cold sensation[15,18,19]. In a long-term follow-up study of over 5 years, treatment-independent progress of thermal hypoesthesia was observed using QST in men with FD[15]. Another aspect of FD-associated SFN is the autonomic dysfunction regularly observed in FD[17,20-22]. Here, hypo- to anhidrosis is the main symptom that may also trigger and enhance heat intolerance which is frequent in FD. FD patients may further suffer from gastrointestinal complaints due to autonomic dysfunction of small caliber nerve fibers, such as dysmotility, diarrhea, and dyspepsia[23]. Autonomic dysfunction may further include cardiac dysautonomia, which may be relevant when deciding on medication with cardiac effects.
FD-ASSOCIATED PAIN
Pain is one of the earliest, most frequent symptoms in FD that may start in childhood[24] and compromises patients’ health-related quality of life[25,26] up to the development of depressive symptoms[26,27]. FD-associated pain is mostly episodic and triggerable by fever, heat, and physical activity. Patients describe it as a burning pain, primarily located at soles, toes, palms, and fingertips; however, it can affect any part of the body, including causing abdominal pain[28]. FD pain can be categorized into four pain subgroups[29]: (1) Pain attacks are the most frequent variant, with sudden and intensive pain upon triggers. Pain attacks remain during a trigger and typically resolve upon trigger removal; (2) Pain crises are severe pain attacks that spread over the body and may be so intense that patients need to be hospitalized. Pain crises may occur with and without triggers and are challenging to treat; (3) Evoked pain is often reported by FD patients in the extremities upon contact with hot or cold objects; and (4) Chronic pain, i.e., persistent pain, is rarely experienced by FD patients. As reported in a previous study, FD-associated pain may resolve over time independent of FD-specific treatment[29].
Pain in FD is mostly categorized as neuropathic pain due to its clinical characteristics (burning) and the impairment of small nerve fibers (QST, skin punch biopsy). It is, however, intriguing that FD patients report analgesic effects using non-steroidal anti-inflammatory drugs (NSAID). While NSAID are classically inefficient in neuropathic pain, they are the first-line treatment of nociceptive pain. Together with findings pointing to a systemic pro-inflammatory profile of FD patients[30-32], these data support the presence of a nociceptive component in FD pain. Further factors, such as spontaneous ectopic firing or central nervous system sensitization, are discussed.
Lyso-Gb3 and FD-associated pain
Globotriaosylsphingosine (lyso-Gb3) is a deacetylated form of Gb3 and its levels may be elevated in the plasma of FD patients[33]. Injection of lyso-Gb3 into the hind paws of mice caused acute pain-associated behavior upon mechanical stimulation, which completely resolved after 24 h. Additionally, mouse DRG showed an increase in intracellular calcium levels together with an increase in voltage-gated calcium channel current densities upon treatment with lyso-Gb3. These findings suggest that lyso-Gb3 may be involved in FD-associated pain by increasing intracellular calcium levels and causing hyperexcitation of nociceptors[34]. If lyso-Gb3 has a pathophysiological role in FD-associated pain is yet to be elucidated. Data are scarce and contradictory. While increased levels of lyso-Gb3 at baseline may serve as a risk factor for adverse outcomes in FD[35] and in one study, baseline plasma lyso-Gb3 levels correlated with baseline pain in male FD patients, no correlation was found between changes in plasma lyso-Gb3 levels and pain scores after treatment with migalastat[36].
DIFFERENTIAL DIAGNOSES OF FD-ASSOCIATED PAIN
The main distinguishing characteristic of FD-associated pain is its manifestation in early childhood as pain triggerable by stimuli such as fever, heat, or physical activity. Moreover, its location, mainly at soles and palms, is an important hallmark in patients’ medical history. One differential diagnosis is erythromelalgia (hereditary or secondary), which may manifest as triggerable pain in single extremities accompanied by local redness, swelling, and severe allodynia. Patients with SFN of other origin (e.g., diabetic, genetic, autoimmune) or idiopathic SFN may also present with acral burning pain. In patients with SFN, the lack of a family history and multiorgan involvement typical of FD can be informative. It is not recommended to conduct unselected genetic testing for FD in all patients with SFN unless typical hallmarks are present[37].
DIAGNOSING PATIENTS WITH FD NEUROPATHY AND PAIN
The main pillar of the diagnostic workup in patients with FD-associated pain is the detailed interview focusing on triggerable and spontaneous pain. Pain location, triggers, and characteristics are crucial information to be collected. Additionally, factors alleviating pain (e.g., drugs, non-pharmacological measures) need to be recorded. The use of FD-pain questionnaires is recommended to systematically cover all pain aspects that are important in FD[38,39]. The clinical assessment should contain a complete neurological examination with a focus on the sensory system. A sensory profile should be recorded, e.g., using QST, which may show thermal hyposensitivity as a finding typical for FD. Nerve conduction studies will help to detect hints of large nerve fiber impairment, if present. For the morphological investigation of the small nerve fibers, a skin punch biopsy is recommended to determine the intraepidermal nerve fiber density (IENFD). The IENFD is typically reduced early in FD. Skin punch biopsy should be performed at the baseline visit and can be repeated at follow-up if the patient reports new sensory symptoms and/or pain[40].
FD-SPECIFIC TREATMENT AND PAIN
It is crucial not to miss the FD diagnosis, since causative treatment is available. While there is currently no cure for FD, ERT or chaperone therapy are efficient symptomatic treatment options that may slow down the progress of FD when treatment is initiated early in the disease course[41,42]. The effect of ERT or chaperone is presented inconsistently in literature. Some studies have documented a relevant reduction in patients’ pain ratings[7,18,43-45], while others have found no such effect[46-48]. The personal experience of the authors (N.Ü.) regularly seeing patients at a large FD center is that only a subgroup of patients experience sufficient and sustained analgesia under FD-specific treatment and that symptomatic treatment is usually necessary. ERT is the most common treatment for FD patients with classic phenotypes. Since FD patients have low levels of GLA enzyme, replacement of this enzyme with recombinant protein shows beneficial effects. Two forms of the recombinant, agalsidase alfa and agalsidase beta, are commercially available. Treatment with both is clinically safe and efficient for FD patients. Agalsidase alfa is a glycosylated protein and structurally identical to GLA. It binds to the mannose-6-receptor on the cell surface and is then eventually transported to lysosomes, where it cleaves Gb3. Agalsidase beta also exhibits similar biochemical properties as agalsidase alfa; however, agalsidase beta shows higher glycosylation than agalsidase alfa[49].
Agalsidase alfa
Agalsidase alfa is administered intravenously every two weeks and the recommended dosage is 0.2 mg/kg body weight. The first clinical trial with agalsidase alfa showed sustained reduction in neuropathic pain and improvement in health-related quality of life of FD patients[8]. Subsequent long-term follow-up studies also supported this notion[50] and also showed improvement in cold and warm thresholds[18]. However, results from other long-term studies and Fabry Outcome Surveys reported no improvement in pain in FD after treatment with agalsidase alfa[51,52]. In a Japanese study, both male and female patients showed improvement in pain scores after treatment with agalsidase alfa and health-related quality of life remained stable during the course of treatment[53]. However, the non-interventional observational post-marketing surveillance from Japan revealed that there was no improvement in pain or health-related quality of life in patients who received ERT. Still, some patients experienced a reduction in pain at times, but they also received additional analgesics during ERT. Hence, it remains inconclusive if the reduction in pain was an effect of agalsidase alfa or the analgesics[46].
Agalsidase beta
Agalsidase beta is administered intravenously every two weeks and the recommended dosage is 1 mg/kg body weight. In early clinical trials, FD patients showed improvement in pain after treatment with agalsidase beta[7]. Treatment with agalsidase beta also showed improvement in the function of C-, Aδ-, and Aβ-nerve fibers in FD patients[45]. When young male and female FD patients ≤ 30 years were treated with agalsidase beta, male patients exhibited a reduction in both chronic peripheral pain and acute pain crises, but not abdominal pain after short-term follow-up (≥ 0.5 year). In contrast, women experienced a reduction solely in acute pain crises. Upon long-term follow-up (≥ 2.5 years), men with FD demonstrated a reduction in all types of pain, whereas women showed improvement only in abdominal pain[44].
Migalastat
Migalastat is the first oral drug for the treatment of FD[54]. Chemically, it is 1-deoxygalactonojirimycin and is an analog of the terminal galactose of Gb3. Oral therapy with migalastat is possible only in patients with so-called “amenable” GLA mutations, i.e., in cases where the rest of GLA activity is still preserved. The majority of these mutations are missense mutations, where the GLA enzyme is misfolded and thus migalastat functions as a chaperone by reversibly binding to the active site of GLA and improving its transportation to lysosomes[9]. Migalastat is administered orally once every other day at a dosage of
SYMPTOMATIC ANALGESIC TREATMENT OF FD PAIN
Symptomatic analgesic treatment is necessary for the majority of FD patients experiencing pain, since FD-specific treatment often does not sufficiently lead to FD pain alleviation. With FD being a rare disease, no randomized and controlled trials are available on pain treatment in FD patients. Hence, clinical management of FD pain follows national and international treatment guidelines for neuropathic pain[56] and expert opinion recommendations[57]. FD pain treatment covers the management of acute episodic FD-associated pain and the long-term analgesic treatment in patients with permanent FD pain, or as prophylaxis to reduce episodic FD pain[29]. As first non-pharmacological measures, FD patients regularly try to avoid any known triggers. Many patients also report pain relief upon cooling the aching extremities. If pain persists, often NSAIDS and non-opioid analgesics are used by FD patients. This underscores a potential inflammatory pain component. One major challenge is the cardiac and renal comorbidity of many patients, which makes careful selection and dosing mandatory. Since FD pain is often local, topically applicable analgesics such as lidocaine or capsaicin may be further options. In patients with extreme pain intensities such as in FD crises, opioid treatment may be indicated[57]. Furthermore, the application of earlier-generation anti-convulsant drugs such as phenytoin or carbamazepine may be beneficial in individual cases[57]. In extreme cases of FD crises, hospitalization for intravenous analgesic pain management may become necessary. In patients experiencing persistent FD-associated pain and aiming to reduce episodic FD pain frequency and intensity, prophylactic use of analgesics recommended as first-line treatment for neuropathic pain is also the current approach in FD patients. As an example, gabapentinoids and tricyclic antidepressants may be used, however, with caution for renal and cardiac contraindications[57].
INSIGHTS FROM FABRY ANIMAL MODELS
Rodent models of FD
To elucidate the pathophysiology of FD neuropathy, extensive work has been done in animal models. In mice, GLA knockout models (KO mice) are frequently used to understand the impact of Gb3 accumulation on different organs[58]. This mouse model shows age-dependent accumulation of Gb3 in different tissues including the dorsal root ganglia (DRG)[59]. Further studies have shown that KO mice, particularly males, display pain-associated behavior such as mechanical and thermal hypersensitivity starting at a very young age and turning to warm and cold hyposensitivity[59].
When investigating potential mechanisms that may contribute to neuropathy and pain in FD, Gb3-dependent alterations in mouse DRG neuron expression and functioning of voltage-gated sodium channels were found[59] in KO mice. Additionally, it was shown that altered expression of immune-related genes may lead to changes in the expression of pain-associated ion channels, contributing to pain in FD[60]. Further, macrophages in the DRG of KO mice have an increased expression of CD68 and CD163[61], which may contribute to pain in FD. GLA KO mice also show elevated expression of transient receptor potential cation channel subfamily V member 1 (TRPV1) in skin and DRG, potentially leading to thermal sensitivity[62] in contrast to thermal hyposensitivity seen in FD patients.
The impact of Gb3 accumulation on the density of peripheral nerve fibers was also investigated in GLA KO rats[63]. The findings revealed a decrease in myelinated nerve fiber density in the saphenous and distal tibial nerves, while no notable difference was observed in the proximal tibial nerves. Additionally, a reduction in unmyelinated nerve fiber density was seen in the saphenous nerve, but not in the femoral motor branches of GLA KO rats. Consistent with findings in KO rat DRG, larger axon diameters were noted in the proximal, but not distal parts of the tibial nerve in KO rats. Furthermore, prominent osmophilic accumulations were found in the myelinated axons of the proximal tibial nerve in KO rats. To determine the nature of these accumulations, immunofluorescence staining was performed using antibodies targeting lysosomes or Gb3 deposits. The results revealed that 2% of myelinated nerves in KO rats exhibited lysosomal accumulation of Gb3, a percentage still higher than that in WT rats. However, a significant amount of Gb3 staining did not colocalize with the axonal marker. Further, it is predicted that these accumulations could be a result of immune cell infiltration or Gb3 accumulation in non-neuronal cells such as Schwann cells.
KO rats displayed increased mechanical sensitivity which progressed with age. Further, their mechanical sensitivity was linked to the sensitization of the transient receptor potential ankyrin 1 (TRPA1) ion channel[64].
Various types of non-neuronal cells, such as keratinocytes and Schwann cells, play pivotal roles in transmitting noxious stimuli to sensory neurons[65-67]. Moreover, these cells may contribute to the manifestation of pain phenotypes observed in patients with FD. A recent study has revealed that cultured DRG from KO rats exhibits hyperexcitability when stimulated with cultured media from Schwann cells[68]. Furthermore, it has been demonstrated that Schwann cells from KO rats secrete p11, a protein that induces spontaneous activity and hyperexcitability in cultured DRG. Notably, upon removal of p11, the observed hyperexcitability was attenuated.
Since there is evidence from human FD studies that FD patients have systemic pro-inflammatory profiles[31,69], a potential immune-mediated component contributing to neuropathy and pain in FD was investigated. In line with results from FD patients, it was also observed that, the KO rats showed elevated levels of blood pro-inflammatory cytokines[70].
Mitochondrial dysfunction has been associated with inflammation, sensory disturbances, and pain in different animal models. Mitochondrial protein, ATP synthase c subunit lysine N-methyltransferase (ATPSc-KMT) acts as a switch for transforming pain from acute to chronic in mice models of chronic pain[71]. It was also found that macrophages transfer mitochondria to sensory neurons in order to resolve pain[72]. Furthermore, the expression of the ATPSc-KMT gene was also reduced in subchondral bone in patients suffering from osteoarthritis[73]. Although these findings seem to be exciting, their role in mitigating pain in FD has not yet been investigated.
Defects in autophagy and autophagosome maturation have been reported in different cells from FD patients, such as fibroblasts[74], peripheral blood mononuclear cells (PBMCs)[75], and conjunctival epithelia[76]. Additionally, renal biopsies from FD patients show impairment in autophagy with the presence of vacuoles in podocytes and mesangial cells[74]. Labeling for autophagy marker microtubule-associated protein 1A/1B-light chain 3 (LC3) in these biopsies revealed that the vacuoles are autophagic in nature. In mouse models of FD, impairment in the autophagy-lysosomal pathway was found in different brain regions[77]. Studies in animal models of peripheral nerve injury have shown a context-dependent role of autophagy in either suppressing[78,79] or enhancing[80,81] neuropathic pain. However, the role of autophagy in sensory neurons, especially small fiber degeneration, and its role in conferring pain in FD is yet to be understood.
Drosophila model of FD
Drosophila (Drosophila melanogaster) is an invertebrate and non-traditional model for studying nociception. Flies consist of sensory neurons[82] and respond to noxious stimuli by showing pain-like behavior[83]. Due to a short life cycle and ease of developing several mutant lines, drosophila can be employed to generate FD-relevant transgenic lines and to investigate nociception in FD. A transgenic fly line expressing human TRPV1 was recently developed to study pain mechanisms and effects of analgesic treatment in drosophila[84]. A drosophila model was also developed to study FD pathophysiology[85]. Transgenic fly lines with two different mutations in the GLA gene were generated: A156V and A285D. In these flies, the GLA enzyme is misfolded and remains trapped in the endoplasmic reticulum. The study also followed the lifespan of flies expressing the two different GLA mutations. The mutant GLA mutations resulted in premature death compared to wild-type GLA flies. Further, treatment with migalastat prolonged the lifespan of flies expressing the A156V mutation and showed little effect on flies expressing the A285D mutation.
Zebrafish model of FD
Zebrafish (Dania rerio) is a highly utilized model for pharmacological and toxicological studies[86]. Due to their genetic homology to humans, exploitable genetics and low maintenance costs, zebrafish present as an attractive model for studying nociception in FD. They respond to a variety of noxious stimuli like temperature[87] and pharmacological agents such as allyl-isothiocyanate[88], formalin[89], and acetic acid[90]. Since zebrafish lack the Gb3 synthase, they cannot produce Gb3, and hence, this model is very useful for studying FD pathomechanisms independent of Gb3 accumulation. They also exhibit robust nocifensive behavior, which is restored upon treatment with analgesics. Most recently, a zebrafish model was described that exhibited reduced GLA activity and impairment in glomerular filtration, as seen in human FD patients[91].
NOVEL PERSPECTIVES
iPSC- and CRISPR/Cas9-derived sensory neurons
Recognizing the limitations of animal models to fully recapitulate human disease, attention has turned toward induced pluripotent stem cell (iPSC)-based cell culture models, which hold promise as attractive alternatives for FD research[92]. With FD caused by pathogenic mutations in the GLA gene, developing animal models for all mutations is not feasible. iPSC-derived cell lines, originating from patient biomaterial, present an opportunity to closely mirror phenotypic characteristics observed in FD patients[92].
In two separate studies, the GLA gene was edited using CRISPR/CAS9 technology: once in an immortalized rat DRG cell line[93] and once in human embryonic stem cells[94] to develop sensory neurons relevant to FD. In the rat DRG cell line, the edited cells showed a reduction in GLA levels and activity and an increase in Gb3 accumulation, consistent with the FD phenotype. In human embryonic stem cells, the introduction of FD-related genetic mutations also resulted in decreased levels and activity of GLA and increased Gb3 accumulation. However, no quantification of GLA or Gb3 was performed in iPSC-derived sensory neurons. Both the rat DRG cell line and iPSC-derived sensory neurons showed expression of ion channels such as TRPV1, NaV 1.7, and NaV 1.8, indicating nociceptive properties. However, the functional characteristics of these sensory neurons were not investigated in either of the studies.
To study pain mechanisms in FD, iPSC lines were generated from skin fibroblasts of patients with FD suffering from pain. These cell lines were then differentiated into sensory neurons (SN) using a small molecule-based method. Interestingly, these SN showed an accumulation of Gb3 in both the soma and the neurites. Furthermore, increased calcium activity upon thermal stimulation, a common pain trigger in FD patients, was observed in these SN. In addition, the study showed that these SN exhibited reduced fiber diameter and impaired mitochondrial trafficking, which may be related to pain in FD[95].
In another study, SN were generated from iPSC using fibroblasts derived from a male subject harboring an S126G mutation in the GLA gene, which is classified as a variant of unknown significance (VUS)[96]. No difference in GLA activity was found in these cells, and there were also no Gb3 accumulations. In addition, electrophysiological properties such as rheobase and threshold potential were comparable to those of the control group. This study underscores the importance of recognizing different GLA mutations and determining their pathogenicity, since not all GLA mutations lead to clinically relevant GLA impairment.
iPSC-derived endothelial cells
Endothelial cell (EC) dysfunction is closely associated with FD. ECs are involved in vascular angiogenesis and the application of tube formation assays is one of the quantitative and sensitive methods used to determine angiogenesis[97]. It has been consistently reported that iPSC-derived ECs from FD patients show reduced tube formation ability compared to ECs from healthy individuals[98,99], indicating impaired angiogenesis. Apart from Gb3 accumulation hypoxia and mitochondrial dysfunction[99], increase in expression of thrombospondin 1[98], oxidative stress[100], increased release of heparanases and pro-inflammatory cytokines[101], and degradation of calcium-activated potassium channel KCa3.1[102] may contribute to EC dysfunction. Impairment in EC function leads to hypovascularization, which may also contribute to the pathophysiology of pain in FD.
Outlook
High-throughput screening of drugs using automated electrophysiological methods provides an opportunity to discover new therapeutic compounds using cell cultures[103]. iPSC-based cell cultures may serve as perfectly relevant model for developing new and repurposing existing analgesics to treat pain in FD. Recent studies have shown that this approach could be one future way of discovering novel analgesics[104].
However, it should be noted that these drug discovery methods rely on strong phenotypic readouts such as electrophysiological output. Additionally, iPSC- derived cell lines come with some caveats such as batch variability, clone variability, and heterogenous populations. Further research to identify reliable readouts and establish screening platforms is crucial for treating pain, not only in FD.
CONCLUSION
Although extensive clinical data on pain characteristics in FD is available, the correlation between genotype and pain phenotype is not yet clear. Animal models have provided some clues about pathological manifestations in FD. Understanding these mechanisms using patient-specific models will be crucial for treating pain and also developing pain-alleviating therapeutics for FD patients. With new treatment perspectives such as gene therapy and substrate reduction therapy for FD, hopes also remain high for more efficient analgesia in patients suffering from FD-associated pain.
DECLARATIONS
Authors’ contributions
Composed and edited the manuscript, literature research on the topic: Medala VK, Üçeyler N
Availability of data and materials
Not applicable.
Financial support and sponsorship
None.
Conflicts of interest
Both authors declared that there are no conflicts of interest.
Ethical approval and consent to participate
Not applicable.
Consent for publication
Not applicable.
Copyright
© The Author(s) 2024.
REFERENCES
1. Platt FM, D'Azzo A, Davidson BL, Neufeld EF, Tifft CJ. Lysosomal storage diseases. Nat Rev Dis Primers 2018;4:27.
2. Saito S, Ohno K, Sakuraba H. Fabry-database.org: database of the clinical phenotypes, genotypes and mutant α-galactosidase a structures in Fabry disease. J Hum Genet 2011;56:467-8.
3. Effraimidis G, Rasmussen ÅK, Dunoe M, et al. Systematic cascade screening in the Danish Fabry disease centre: 20 years of a national single-centre experience. PLoS One 2022;17:e0277767.
4. Bertoldi G, Caputo I, Driussi G, et al. Biochemical mechanisms beyond glycosphingolipid accumulation in Fabry disease: might they provide additional therapeutic treatments? J Clin Med 2023;12:2063.
5. Izhar R, Borriello M, La Russa A, et al. Fabry disease in women: genetic basis, available biomarkers, and clinical manifestations. Genes 2023;15:37.
6. Faro DC, Losi V, Rodolico MS, et al. Sex differences in anderson-Fabry cardiomyopathy: clinical, genetic, and imaging analysis in women. Genes 2023;14:1804.
7. Eng CM, Banikazemi M, Gordon RE, et al. A phase 1/2 clinical trial of enzyme replacement in fabry disease: pharmacokinetic, substrate clearance, and safety studies. Am J Hum Genet 2001;68:711-22.
8. Schiffmann R, Kopp JB, Austin HA 3rd, et al. Enzyme replacement therapy in Fabry disease: a randomized controlled trial. JAMA 2001;285:2743-9.
9. Germain DP, Hughes DA, Nicholls K, et al. Treatment of Fabry's disease with the pharmacologic chaperone migalastat. N Engl J Med 2016;375:545-55.
10. Li Q, Wang J, Tian M, et al. Clinical features and enzyme replacement therapy in 10 children with Fabry disease. Front Pediatr 2023;11:1084336.
11. Morand O, Johnson J, Walter J, et al. Symptoms and quality of life in patients with Fabry disease: results from an international patient survey. Adv Ther 2019;36:2866-80.
12. Politei JM, Durand C, Schenone AB. Small Fiber neuropathy in Fabry disease: a review of pathophysiology and treatment. J Inborn Errors Metab Scr 2016;4:232640981666135.
13. Üçeyler N, Kahn AK, Kramer D, et al. Impaired small fiber conduction in patients with Fabry disease: a neurophysiological case-control study. BMC Neurol 2013;13:47.
14. Scott LJ, Griffin JW, Luciano C, et al. Quantitative analysis of epidermal innervation in Fabry disease. Neurology 1999;52:1249-54.
15. Üçeyler N, He L, Schönfeld D, et al. Small fibers in Fabry disease: baseline and follow-up data under enzyme replacement therapy. J Peripher Nerv Syst 2011;16:304-14.
16. Arnold R, Pianta TJ, Issar T, et al. Peripheral neuropathy: an important contributor to physical limitation and morbidity in stages 3 and 4 chronic kidney disease. Nephrol Dial Transplant 2022;37:713-9.
17. Burlina AP, Sims KB, Politei JM, et al. Early diagnosis of peripheral nervous system involvement in Fabry disease and treatment of neuropathic pain: the report of an expert panel. BMC Neurol 2011;11:61.
18. Schiffmann R, Floeter MK, Dambrosia JM, et al. Enzyme replacement therapy improves peripheral nerve and sweat function in Fabry disease. Muscle Nerve 2003;28:703-10.
19. Maag R, Binder A, Maier C, et al. Detection of a characteristic painful neuropathy in Fabry disease: a pilot study. Pain Med 2008;9:1217-23.
20. Hilz MJ. Evaluation of peripheral and autonomic nerve function in Fabry disease. Acta Paediatr Suppl 2002;91:38-42.
21. Møller AT, Bach FW, Feldt-Rasmussen U, et al. Autonomic skin responses in females with Fabry disease. J Peripher Nerv Syst 2009;14:159-64.
22. Dutra-Clarke M, Tapia D, Curtin E, et al. Variable clinical features of patients with Fabry disease and outcome of enzyme replacement therapy. Mol Genet Metab Rep 2021;26:100700.
23. Bar N, Karaa A, Kiser K, Kuo B, Zar-Kessler C. Gastrointestinal sensory neuropathy and dysmotility in Fabry disease: presentations and effect on patient's quality of life. Clin Transl Gastroenterol 2023;14:e00633.
24. Hopkin RJ, Bissler J, Banikazemi M, et al. Characterization of Fabry disease in 352 pediatric patients in the Fabry Registry. Pediatr Res 2008;64:550-5.
25. Burand AJ Jr, Stucky CL. Fabry disease pain: patient and preclinical parallels. Pain 2021;162:1305-21.
26. Winter Y, Hilz M, Beuschlein F, et al. Screening for health-related quality of life and its determinants in Fabry disease: a cross-sectional multicenter study. Mol Genet Metab 2023;140:107692.
27. Körver S, Geurtsen GJ, Hollak CEM, et al. Depressive symptoms in Fabry disease: the importance of coping, subjective health perception and pain. Orphanet J Rare Dis 2020;15:28.
28. Radulescu D, Crisan D, Militaru V, et al. Gastrointestinal manifestations and treatment options in Fabry disease patients. a systematic review. J Gastrointestin Liver Dis 2022;31:98-106.
29. Üçeyler N, Ganendiran S, Kramer D, Sommer C. Characterization of pain in Fabry disease. Clin J Pain 2014;30:915-20.
30. Rosa Neto NS, Bento JCB, Caparbo VF, Pereira RMR. Increased serum interleukin-6 and tumor necrosis factor alpha levels in fabry disease: correlation with disease burden. Clinics 2021;76:e2643.
31. Laffer B, Lenders M, Ehlers-Jeske E, Heidenreich K, Brand E, Köhl J. Complement activation and cellular inflammation in Fabry disease patients despite enzyme replacement therapy. Front Immunol 2024;15:1307558.
32. Üçeyler N, Urlaub D, Mayer C, Uehlein S, Held M, Sommer C. Tumor necrosis factor-α links heat and inflammation with Fabry pain. Mol Genet Metab 2019;127:200-6.
33. Aerts JM, Groener JE, Kuiper S, et al. Elevated globotriaosylsphingosine is a hallmark of Fabry disease. Proc Natl Acad Sci USA 2008;105:2812-7.
34. Choi L, Vernon J, Kopach O, et al. The Fabry disease-associated lipid Lyso-Gb3 enhances voltage-gated calcium currents in sensory neurons and causes pain. Neurosci Lett 2015;594:163-8.
35. Nowak A, Beuschlein F, Sivasubramaniam V, Kasper D, Warnock DG. Lyso-Gb3 associates with adverse long-term outcome in patients with Fabry disease. J Med Genet 2022;59:287-93.
36. Bichet DG, Aerts JM, Auray-Blais C, et al. Assessment of plasma lyso-Gb3 for clinical monitoring of treatment response in migalastat-treated patients with Fabry disease. Genet Med 2021;23:192-201.
37. de Greef BT, Hoeijmakers JG, Wolters EE, et al. No Fabry disease in patients presenting with isolated small fiber neuropathy. PLoS One 2016;11:e0148316.
38. Magg B, Riegler C, Wiedmann S, Heuschmann P, Sommer C, Üçeyler N. Self-administered version of the Fabry-associated pain questionnaire for adult patients. Orphanet J Rare Dis 2015;10:113.
39. Üçeyler N, Magg B, Thomas P, Wiedmann S, Heuschmann P, Sommer C. A comprehensive Fabry-related pain questionnaire for adult patients. Pain 2014;155:2301-5.
40. Interdisziplinäre leitlinie für die diagnose und therapie des morbus Fabry. 2022. Available from: https://dgn.org/leitlinie/interdisziplinare-leitlinie-fur-die-diagnose-und-therapie-des-morbus-fabry [Last accessed on 2 Jul 2024].
41. Ezgu F, Alpsoy E, Bicik Bahcebasi Z, et al. Expert opinion on the recognition, diagnosis and management of children and adults with Fabry disease: a multidisciplinary Turkey perspective. Orphanet J Rare Dis 2022;17:90.
42. Wanner C, Arad M, Baron R, et al. European expert consensus statement on therapeutic goals in Fabry disease. Mol Genet Metab 2018;124:189-203.
43. Müntze J, Lau K, Cybulla M, et al. Patient reported quality of life and medication adherence in Fabry disease patients treated with migalastat: a prospective, multicenter study. Mol Genet Metab 2023;138:106981.
44. Hopkin RJ, Cabrera GH, Jefferies JL, et al. Clinical outcomes among young patients with Fabry disease who initiated agalsidase beta treatment before 30 years of age: an analysis from the Fabry registry. Mol Genet Metab 2023;138:106967.
45. Hilz MJ, Brys M, Marthol H, Stemper B, Dütsch M. Enzyme replacement therapy improves function of C-, Aδ-, and Aβ-nerve fibers in Fabry neuropathy. Neurology 2004;62:1066-72.
46. Sasa H, Nagao M, Kino K. Safety and effectiveness of enzyme replacement therapy with agalsidase alfa in patients with Fabry disease: post-marketing surveillance in Japan. Mol Genet Metab 2019;126:448-59.
47. Lenders M, Nordbeck P, Kurschat C, et al. Treatment of Fabry disease management with migalastat-outcome from a prospective 24 months observational multicenter study (FAMOUS). Eur Heart J Cardiovasc Pharmacother 2022;8:272-81.
48. Riccio E, Zanfardino M, Ferreri L, et al. Switch from enzyme replacement therapy to oral chaperone migalastat for treating Fabry disease: real-life data. Eur J Hum Genet 2020;28:1662-8.
49. Lee K, Jin X, Zhang K, et al. A biochemical and pharmacological comparison of enzyme replacement therapies for the glycolipid storage disorder Fabry disease. Glycobiology 2003;13:305-13.
50. Hoffmann B, Garcia de Lorenzo A, Mehta A, Beck M, Widmer U, Ricci R. FOS European Investigators. Effects of enzyme replacement therapy on pain and health related quality of life in patients with Fabry disease: data from FOS (Fabry Outcome Survey). J Med Genet 2005;42:247-52.
51. Sergi B, Conti G, Paludetti G. Inner ear involvement in Anderson-Fabry disease: long-term follow-up during enzyme replacement therapy. Acta Otorhinolaryngol Ital 2010;30:87-93.
52. Hughes DA, Barba Romero MÁ, Hollak CE, Giugliani R, Deegan PB. Response of women with Fabry disease to enzyme replacement therapy: comparison with men, using data from FOS-the Fabry outcome survey. Mol Genet Metab 2011;103:207-14.
53. Tsuboi K, Yamamoto H. Efficacy and safety of enzyme-replacement-therapy with agalsidase alfa in 36 treatment-naïve Fabry disease patients. BMC Pharmacol Toxicol 2017;18:43.
54. Germain DP, Giugliani R, Hughes DA, et al. Safety and pharmacodynamic effects of a pharmacological chaperone on α-galactosidase a activity and globotriaosylceramide clearance in Fabry disease: report from two phase 2 clinical studies. Orphanet J Rare Dis 2012;7:91.
55. Hughes DA, Nicholls K, Shankar SP, et al. Oral pharmacological chaperone migalastat compared with enzyme replacement therapy in Fabry disease: 18-month results from the randomised phase III ATTRACT study. J Med Genet 2017;54:288-96.
56. Finnerup NB, Attal N, Haroutounian S, et al. Pharmacotherapy for neuropathic pain in adults: a systematic review and meta-analysis. Lancet Neurol 2015;14:162-73.
57. Politei JM, Bouhassira D, Germain DP, et al. Pain in Fabry disease: practical recommendations for diagnosis and treatment. CNS Neurosci Ther 2016;22:568-76.
58. Ohshima T, Murray GJ, Swaim WD, et al. α-galactosidase a deficient mice: a model of Fabry disease. Proc Natl Acad Sci USA 1997;94:2540-4.
59. Hofmann L, Hose D, Grießhammer A, et al. Characterization of small fiber pathology in a mouse model of Fabry disease. Elife 2018;7:e39300.
60. Spitzel M, Wagner E, Breyer M, et al. Dysregulation of immune response mediators and pain-related ion channels is associated with pain-like behavior in the GLA KO mouse model of Fabry disease. Cells 2022;11:1730.
61. Choconta JL, Labi V, Dumbraveanu C, Kalpachidou T, Kummer KK, Kress M. Age-related neuroimmune signatures in dorsal root ganglia of a Fabry disease mouse model. Immun Ageing 2023;20:22.
62. Lakomá J, Rimondini R, Ferrer Montiel A, Donadio V, Liguori R, Caprini M. Increased expression of Trpv1 in peripheral terminals mediates thermal nociception in Fabry disease mouse model. Mol Pain 2016;12:174480691666372.
63. Waltz TB, Burand AJ Jr, Sadler KE, Stucky CL. Sensory-specific peripheral nerve pathology in a rat model of Fabry disease. Neurobiol Pain 2021;10:100074.
64. Miller JJ, Aoki K, Moehring F, et al. Neuropathic pain in a Fabry disease rat model. JCI Insight 2018;3:99171.
65. Talagas M, Lebonvallet N, Leschiera R, et al. Keratinocytes communicate with sensory neurons via synaptic-like contacts. Ann Neurol 2020;88:1205-19.
66. Mikesell AR, Isaeva E, Schulte ML, et al. Keratinocyte piezo1 drives paclitaxel-induced mechanical hypersensitivity. bioRxiv 2023.
67. Erbacher C, Britz S, Dinkel P, et al. Interaction of human keratinocytes and nerve fiber terminals at the neuro-cutaneous unit. Elife 2024;13:e77761.
68. Waltz TB, Chao D, Prodoehl EK, et al. Schwann cell release of p11 induces sensory neuron hyperactivity in Fabry disease. bioRxiv 2023.
69. Yogasundaram H, Nikhanj A, Putko BN, et al. Elevated inflammatory plasma biomarkers in patients with Fabry disease: a critical link to heart failure with preserved ejection fraction. J Am Heart Assoc 2018;7:e009098.
70. Kanack AJ, Aoki K, Tiemeyer M, Dahms NM. Platelet and myeloid cell phenotypes in a rat model of Fabry disease. FASEB J 2021;35:e21818.
71. Willemen HLDM, Santos Ribeiro PS, Broeks M, et al. Inflammation-induced mitochondrial and metabolic disturbances in sensory neurons control the switch from acute to chronic pain. Cell Rep Med 2023;4:101265.
72. van der Vlist M, Raoof R, Willemen HLDM, et al. Macrophages transfer mitochondria to sensory neurons to resolve inflammatory pain. Neuron 2022;110:613-26.e9.
73. Palada V, Siddiqah Ahmed A, Hugo A, Radojčić MR, Svensson CI, Kosek E. Expression of mitochondrial TSPO and FAM173B is associated with inflammation and symptoms in patients with painful knee osteoarthritis. Rheumatology 2021;60:1724-33.
74. Chévrier M, Brakch N, Céline L, et al. Autophagosome maturation is impaired in Fabry disease. Autophagy 2010;6:589-99.
75. Ivanova MM, Changsila E, Iaonou C, Goker-Alpan O. Impaired autophagic and mitochondrial functions are partially restored by ERT in Gaucher and Fabry diseases. PLoS One 2019;14:e0210617.
76. Marenco M, Segatto M, Sacchetti M, Mangiantini P, Giovannetti F, Plateroti R. Autophagy-lysosome pathway alteration in ocular surface manifestations in Fabry disease patients. Orphanet J Rare Dis 2022;17:291.
77. Nelson MP, Tse TE, O'Quinn DB, et al. Autophagy-lysosome pathway associated neuropathology and axonal degeneration in the brains of alpha-galactosidase a-deficient mice. Acta Neuropathol Commun 2014;2:20.
78. Chen H, Hu Y, Xie K, et al. Effect of autophagy on allodynia, hyperalgesia and astrocyte activation in a rat model of neuropathic pain. Int J Mol Med 2018;42:2009-19.
79. Liu K, Yang Y, Zhou F, Xiao Y, Shi L. Inhibition of PI3K/AKT/mTOR signaling pathway promotes autophagy and relieves hyperalgesia in diabetic rats. Neuroreport 2020;31:644-9.
80. Zhang E, Yi MH, Ko Y, et al. Expression of LC3 and Beclin 1 in the spinal dorsal horn following spinal nerve ligation-induced neuropathic pain. Brain Res 2013;1519:31-9.
81. Chen W, Lu Z. Upregulated TLR3 promotes neuropathic pain by regulating autophagy in rat with L5 spinal nerve ligation model. Neurochem Res 2017;42:634-43.
82. Hehlert P, Zhang W, Göpfert MC. Drosophila mechanosensory transduction. Trends Neurosci 2021;44:323-35.
83. Petersen M, Tenedini F, Hoyer N, Kutschera F, Soba P. Assaying thermo-nociceptive behavior in drosophila larvae. Bio Protoc 2018;8:e2737.
84. Jang W, Oh M, Cho EH, Baek M, Kim C. Drosophila pain sensitization and modulation unveiled by a novel pain model and analgesic drugs. PLoS One 2023;18:e0281874.
85. Braunstein H, Papazian M, Maor G, Lukas J, Rolfs A, Horowitz M. Misfolding of lysosomal α-galactosidase a in a fly model and its alleviation by the pharmacological chaperone migalastat. Int J Mol Sci 2020;21:7397.
86. Bonan CD, Siebel AM. Editorial: zebrafish as a model for pharmacological and toxicological research. Front Pharmacol 2022;13:976970.
87. Lopez-Luna J, Al-Jubouri Q, Al-Nuaimy W, Sneddon LU. Impact of analgesic drugs on the behavioural responses of larval zebrafish to potentially noxious temperatures. Appl Anim Behav Sci 2017;188:97-105.
88. Prober DA, Zimmerman S, Myers BR, et al. Zebrafish TRPA1 channels are required for chemosensation but not for thermosensation or mechanosensory hair cell function. J Neurosci 2008;28:10102-10.
89. Magalhães FEA, de Sousa CÁPB, Santos SAAR, et al. Adult zebrafish (danio rerio): an alternative behavioral model of formalin-induced nociception. Zebrafish 2017;14:422-9.
90. Costa FV, Rosa LV, Quadros VA, Santos ARS, Kalueff AV, Rosemberg DB. Understanding nociception-related phenotypes in adult zebrafish: behavioral and pharmacological characterization using a new acetic acid model. Behav Brain Res 2019;359:570-8.
91. Elsaid HOA, Furriol J, Blomqvist M, et al. Reduced α-galactosidase a activity in zebrafish (Danio rerio) mirrors distinct features of Fabry nephropathy phenotype. Mol Genet Metab Rep 2022;31:100851.
92. Klein T, Grüner J, Breyer M, et al. Small fibre neuropathy in Fabry disease: a human-derived neuronal in vitro disease model. bioRxiv 2023.
93. Kaneski CR, Hanover JA, Schueler Hoffman UH. Generation of an in vitro model for peripheral neuropathy in Fabry disease using CRISPR-Cas9 in the nociceptive dorsal root ganglion cell line 50B11. Mol Genet Metab Rep 2022;31:100871.
94. Kaneski CR, Hanover JA, Schueler Hoffman UH. Generation of GLA-knockout human embryonic stem cell lines to model peripheral neuropathy in Fabry disease. Mol Genet Metab Rep 2022;33:100914.
95. Klein T, Grüner J, Breyer M, et al. Small fibre neuropathy in Fabry disease: a human-derived neuronal in vitro disease model and pilot data. Brain Commun 2024;6:fcae095.
96. Breyer M, Grüner J, Klein A, et al. In vitro characterization of cells derived from a patient with the GLA variant c.376A>G (p.S126G) highlights a non-pathogenic role in Fabry disease. Mol Genet Metab Rep 2024;38:101029.
97. DeCicco-Skinner KL, Henry GH, Cataisson C, et al. Endothelial cell tube formation assay for the in vitro study of angiogenesis. J Vis Exp 2014;91:e51312.
98. Do HS, Park SW, Im I, et al. Enhanced thrombospondin-1 causes dysfunction of vascular endothelial cells derived from Fabry disease-induced pluripotent stem cells. EBioMedicine 2020;52:102633.
99. Klug K, Spitzel M, Hans C, et al. Endothelial cell dysfunction and hypoxia as potential mediators of pain in Fabry disease: a human-murine translational approach. Int J Mol Sci 2023;24:15422.
100. Shen JS, Meng XL, Moore DF, et al. Globotriaosylceramide induces oxidative stress and up-regulates cell adhesion molecule expression in Fabry disease endothelial cells. Mol Genet Metab 2008;95:163-8.
101. Pollmann S, Scharnetzki D, Manikowski D, Lenders M, Brand E. Endothelial dysfunction in Fabry disease is related to glycocalyx degradation. Front Immunol 2021;12:789142.
102. Choi S, Kim JA, Na HY, et al. Globotriaosylceramide induces lysosomal degradation of endothelial KCa3.1 in Fabry disease. Arterioscler Thromb Vasc Biol 2014;34:81-9.
103. Liu PW, Zhang H, Werley CA, et al. A phenotypic screening platform for chronic pain therapeutics using all-optical electrophysiology. Pain 2024;165:922-40.
Cite This Article
How to Cite
Medala, V. K.; Üçeyler N. Neuropathy and pain in Fabry disease. Rare. Dis. Orphan. Drugs. J. 2024, 3, 20. http://dx.doi.org/10.20517/rdodj.2024.13
Download Citation
Export Citation File:
Type of Import
Tips on Downloading Citation
Citation Manager File Format
Type of Import
Direct Import: When the Direct Import option is selected (the default state), a dialogue box will give you the option to Save or Open the downloaded citation data. Choosing Open will either launch your citation manager or give you a choice of applications with which to use the metadata. The Save option saves the file locally for later use.
Indirect Import: When the Indirect Import option is selected, the metadata is displayed and may be copied and pasted as needed.
About This Article
Special Issue
Copyright
Data & Comments
Data
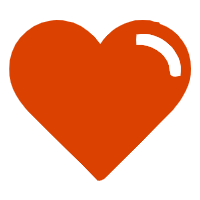
Comments
Comments must be written in English. Spam, offensive content, impersonation, and private information will not be permitted. If any comment is reported and identified as inappropriate content by OAE staff, the comment will be removed without notice. If you have any queries or need any help, please contact us at support@oaepublish.com.