Review on gene and cell therapies in prostate cancer treatment: prospects and challenges
Abstract
Gene and cell therapies have emerged as innovative therapeutic strategies, offering a new paradigm in the treatment of prostate cancer. This review explores the current state of gene and cell therapies, including their mechanisms, applications, and the challenges faced in clinical translation. The article selection was based on the 2020 preferred reporting items for systematic reviews and meta-analyses (PRISMA). Eighty-four (84) articles (published in English between 2018 and 2024) from standard electronic databases were extracted and reviewed. Key therapeutic approaches such as viral vector-mediated delivery, CRISPR-Cas9 gene editing, suicide genes, cell-based therapies like CAR T cell therapy, and dendritic cell vaccines that target specific tumor antigens were reviewed. These therapies promise to enhance therapeutic efficacy with few challenges, like off-target effects, limited delivery efficiency, and immune-related toxicities associated with delivery. Advances in CAR T cells, nanoparticle-based gene delivery systems, and epigenetic modulation are potential solutions to these challenges. Combination therapies involving gene and cell therapies with immunotherapies and oncolytic viruses hold the potential for synergistic treatment effects. Certain critical regulatory, ethical, and accessibility issues must be addressed to ensure the safe and equitable deployment of these therapies. Continued research and innovation in gene and cell therapies and streamlined regulatory pathways will be crucial for realizing their full potential in treating prostate cancer. Overcoming these challenges and fears confronting gene and cell therapies worldwide might facilitate a stream of personalized medicine in prostate oncology.
Keywords
BACKGROUND
Prostate cancer is one of the most common malignancies affecting men worldwide, particularly in older populations[1]. According to recent epidemiological studies, prostate cancer ranks as the second most frequently diagnosed cancer death in men, with its incidence rising significantly in regions such as North America, Europe, and parts of sub-Saharan Africa. Approximately 1 in 44 men died from prostate cancer, with a total of 35,250 deaths worldwide in 2024[1-3]. The risk factors for prostate cancer include age, family history, race, lifestyle, and mutation in the BRCA1 and BRCA2 genes. African-American men and Caribbean men of African descent have been found to present with higher incidence and mortality rates compared to other races, which is attributed to genetic predispositions and access to healthcare disparities[3]. Current treatment strategies for prostate cancer include a combination of surgery, radiotherapy, chemotherapy, and hormonal therapy, depending on the stage and aggressiveness of the disease and the diagnosis of definitive tumor markers[1]. Early-stage prostate cancer can be effectively managed with surgery or localized radiation; advanced cases present significant therapeutic challenges due to metastasis and resistance to conventional therapies[4] and, therefore, the need for more personalized and innovative approaches to managing the disease. Gene and cell therapies now represent groundbreaking approaches aimed at overcoming the limitations of conventional cancer treatments, unlike traditional methods that often target cancer tissues non-specifically. These therapies work at the molecular level, correcting the underlying genetic mutations or enhancing the immune system’s ability to recognize and destroy cancer cells. Gene and cell therapy have gained considerable attention due to their potential to provide long-term remission with fewer side effects[5-8]. Figure 1 illustrates gene therapy. Regulatory agencies should prioritize the accelerated approval of clinical trials and products. The article selection method for this review was based on the 2020 Preferred Reporting Items for Systematic Reviews and Meta-Analyses (PRISMA) standards. Eighty-four (84) articles from standard electronic databases published in English between 2018 and 2024 were extracted and reviewed.
Figure 1. Gene therapy for prostate cancer[8].
CURRENT THERAPEUTIC APPROACHES
Prostate cancer treatment has traditionally relied on well-established modalities, including surgery, radiotherapy, hormone therapy, and chemotherapy. The choice of treatment is typically guided by the stage of the cancer, the patient’s age and overall health, and whether the disease has spread beyond the prostate gland. For early-stage prostate cancer, localized treatments such as radical prostatectomy (surgical removal of the prostate) and external beam radiotherapy are highly effective in controlling the disease and improving long-term survival rates[9]. Hormone therapy, also known as androgen deprivation therapy (ADT), is commonly used in patients with advanced or metastatic prostate cancer. Prostate cancer cells rely on androgen to grow. ADT reduces androgen levels or blocks their effects, leading to a significant reduction in tumor size and slowing the progression of the disease[10]. Hormone therapy is not curative and may eventually lead to castration-resistant prostate cancer (CRPC) due to the cancer's negative response to hormonal manipulation. Chemotherapy was initially reserved for advanced-stage prostate cancer or when the disease becomes resistant to hormone therapy. However, it has now been extended to non-hormone-resistant cases. Drugs like docetaxel and cabazitaxel are used to kill rapidly dividing cancer cells but often come with severe side effects such as fatigue, hair loss, and weakened immune function[11]. Chemotherapy has been shown to prolong survival, but it does not offer a cure and is associated with significant toxicity.
Despite the effectiveness of these standard treatments, they come with notable limitations. Surgical interventions, though potentially curative in early-stage disease, carry risks such as urinary incontinence, erectile dysfunction, neuropathy, and other complications that significantly affect a patient’s quality of life[12]. The availability of da Vinci and other robotic systems in Uganda and other African countries has dramatically reduced surgical complications. More so, radiotherapy causes damage to surrounding tissues, leading to bowel, bladder, and sexual dysfunction. Conventional treatments often fall short in advanced prostate cancer due to the cancer’s ability to develop resistance. For instance, castration-resistant prostate cancer (CRPC) continues to grow despite androgen deprivation, rendering hormone therapy ineffective[13].
Once the cancer becomes resistant to hormone therapy, other options are limited, and the prognosis worsens[14]. Similarly, chemotherapy temporarily shrinks tumors but does not address the root cause of the disease, such as genetic mutations driving cancer progression and its associated systemic toxicity. These limitations underscore the need for more targeted and personalized approaches to prostate cancer treatment. This is where gene and cell therapies offer potential benefits to overcome the challenges of drug resistance and minimize damage to healthy tissues.
GENE THERAPY
Gene therapy involves the modification of the genetic material within cancer cells to inhibit tumor growth, induce apoptosis, or enhance immune recognition.
Targeted gene delivery systems
Gene therapy in prostate cancer treatment revolves around delivering therapeutic genes directly to cancer cells, altering their behavior to inhibit tumor growth or enhance the immune system's ability to combat the cancer. The two primary methods of gene delivery are viral and non-viral vectors. Viral vectors, such as adenoviruses, lentiviruses, and retroviruses, have been widely studied due to their high efficiency in transferring genetic material. These viruses are modified to carry therapeutic genes without causing disease[15]. Adenovirus vectors are frequently used to deliver genes that trigger apoptosis or suppress the activity of oncogenes in prostate cancer cells[16]. Non-viral vectors are composed of plasmid DNA and rely on synthetic materials like liposomes and nanoparticles to encapsulate and deliver the genetic material to the cancer cells. Although non-viral vectors are considered safer due to lower immune response risks, a few challenges in terms of transformation efficiency (how DNA enters cells via lysosomes or nanoparticles) and sustained gene expression are often encountered[17]. In prostate cancer, the development of nanoparticles that can target tumor-specific receptors and deliver therapeutic genes directly to the cancer site is a major area of ongoing research. Nanoparticles have emerged as a promising tool in gene therapy, offering versatile and transformative efficient ways of delivering genetic materials into cells. These tiny particles are engineered to target specific cells or tissues, reducing off-target effects experienced with older tools[18-20]. Different types of nanoparticles exist in gene therapy: liposome liquid-based nanoparticles, encapsulated genetic material[l9], polymeric nanoparticles made from biodegradable polymer[21], and metallic nanoparticles like gold, silver, and iron oxide[22]. These tools provide several advantages in the field of gene therapy with targeted delivery, improved efficiency, and bio-compatibility despite toxicity, immunogenicity, and scalability associated with the process[21].
Gene editing tools
The advent of CRISPR-Cas9 technology has revolutionized gene therapy, enabling precise editing of specific gene sequences. CRISPR-Cas9 is a tool used to knock out oncogenes or restore the function of tumor suppressor genes such as PTEN or TP53, which are frequently mutated in advanced prostate cancer[23]. See Figure 1. This technology holds significant promise in targeting genetic mutations that lead to resistance to conventional treatments, such as androgen receptor (AR) mutations that make prostate cancer cells less responsive to hormone therapy. By directly editing the AR gene, CRISPR-Cas9 could potentially reverse resistance and enhance the efficacy of existing therapies[24,25]. However, concerns about off-target effects and the potential for unintended genetic alterations remain a key challenge. Off-target effects include insertion and deletions of nucleotide at off-target sites, unintended substitution, chromosomal rearrangement (translocation and inversions), and often mixtures of edited and unedited cells within an individual (Mosaicism)[25], as presented in Figure 2.
Figure 2. Mechanism of the CRISPR/Cas9 gene editing system. The single guide RNA (sgRNA) directs the Cas9 nuclease to a complementary genomic sequence, where Cas9 induces a double-strand break (DSB). The target sequence must be adjacent to a 5′-NGG-3′ protospacer adjacent motif (PAM) for Cas9 activity. The DSB is repaired either by non-homologous end joining (NHEJ) or homology-directed repair (HDR), the latter of which can utilize a DNA repair template to introduce precise genetic modifications or exogenous sequences [25].
Immunogene therapy
Various gene transfer techniques have been explored in prostate cancer therapy, each with its own set of advantages and challenges. One promising approach is the transfer of tumor suppressor genes such as p53, which plays a crucial role in regulating cell division and apoptosis. In prostate cancer, the p53 gene is mutated or inactivated, allowing cancer cells to proliferate uncontrollably[26]. Delivering a functional p53 gene into prostate cancer cells can restore their ability to undergo programmed cell death, potentially reducing tumor size and preventing metastasis. Another approach is the use of oncolytic viruses, which are designed to target, infect, and kill cancer cells while sparing normal cells. These viruses destroy cancer cells directly and stimulate the immune system to recognize and attack the tumor. Oncolytic virotherapy is a scientific way of enhancing the body's natural immune response against prostate cancer, particularly in combination with other treatments such as checkpoint inhibitors[27].
Suicide gene
Suicide gene therapy is a promising approach in the treatment of prostate cancer. This technique involves introducing a gene that makes cancer cells vulnerable to a specific drug, causing them to die[28]. The process starts with the introduction of a suicide gene, typically via a viral vector, into the prostate cancer cells. This gene encodes an enzyme that converts a pro-drug into a toxic compound, selectively killing the cancer cells. Herpes simplex virus thymidine kinase (HSV-tk): This gene makes cancer cells sensitive to the antiviral drug ganciclovir, leading to cell death. Cytochrome P450: This gene converts a pro-drug into a toxic compound, selectively killing cancer cells[29]. Several clinical trials have investigated the safety and efficacy of suicide gene therapy for prostate cancer. A study published in 1996 reported the first use of suicide gene therapy in prostate cancer patients[30], and numerous studies have demonstrated the potential of this approach in treating prostate cancer. Overall, suicide gene therapy holds promise for the treatment of prostate cancer, and ongoing research aims to address the challenges and improve its efficacy[31-33].
CELL THERAPY
Cell therapy uses immune cells like T cells to fight cancer. In prostate cancer, chimeric antigen receptor (CAR) T cell therapy and dendritic cell vaccines have shown promise in early-phase clinical trials[34].
Chimeric antigen receptor T cell
This is one of the most advanced forms of cell therapy being explored for prostate cancer. This approach involves genetically modifying a patient’s T cells (a type of immune cell) to express CARs - synthetic receptors designed to recognize specific proteins on the surface of cancer cells. Once re-infused into the patient, these CAR T cells can recognize and destroy prostate cancer cells[34]. In prostate cancer, CAR T cell therapy primarily targets the prostate-specific membrane antigen (PSMA), a protein highly expressed on the surface of prostate cancer cells. By engineering T cells to target PSMA, researchers enhance the immune system's ability to eliminate cancerous cells[35]. Preclinical studies have shown promising results, with CAR T cells effectively recognizing and killing prostate cancer cells in laboratory models. However, there are significant challenges to the use of CAR T cell therapy in prostate cancer. One of the main concerns is the potential for off-tumor toxicity, where CAR T cells attack not only cancer cells but also healthy tissues that express low levels of PSMA or other targeted proteins[36]. Additionally, prostate cancer cells can develop resistance to CAR T cell therapy by downregulating the expression of PSMA, which allows them to evade immune detection. This highlights the need for further research to improve the specificity and durability of CAR T cell therapies for prostate cancer.
Dendritic cell vaccines
Dendritic cell vaccines are a promising cell-based therapeutic approach in the treatment of prostate cancer. Dendritic cells are Toll-like receptors-a- a type of antigen-presenting cell that plays a critical role in initiating immune responses. Dendritic cell vaccines are designed to activate the patient's immune system by introducing tumor-associated antigens (TAAs) that help the immune system recognize and target prostate cancer cells[37]. The most well-known dendritic cellular immunotherapy in prostate cancer treatment is sipuleucel-T (Provenge), the first cancer vaccine approved by the FDA for the treatment of metastatic castration-resistant prostate cancer (CRPC). Sipuleucel-T is made by isolating a patient’s dendritic cells, exposing them to a protein found in prostate cancer cells, and then re-infusing them into the patient to stimulate an immune response[38]. Clinical trials have demonstrated that sipuleucel-T can extend overall survival in patients with metastatic prostate cancer, although its effects on tumor shrinkage and progression-free survival have been limited. Despite their potential, dendritic cell vaccines face several hurdles. The manufacturing process is labor-intensive and expensive, making it difficult to produce large volumes of gene-edited products like cells, tissues, and organisms. Additionally, the immune responses generated by these vaccines are often weak and require combining with other immunotherapies like immune checkpoint inhibitors necessary to achieve more robust clinical outcomes[39].
Natural killer cells and adoptive cell transfer
Natural killer (NK) cells are another type of immune cell being explored for their potential use in prostate cancer treatment. NK cells are part of the body’s innate immune system and can recognize and kill tumor cells without prior sensitization[40]. Research has focused on enhancing the cytotoxicity of NK cells against prostate cancer cells by either populating them in the laboratory or genetically modifying them to enhance their activity. For example, NK cells can be engineered to express CARs similar to those used in CAR T cell therapy, allowing them to effectively target prostate cancer-specific antigens such as PSMA[41]. However, challenges remain in improving the persistence and trafficking of NK cells to the tumor site. CAR T cells, dendritic cell vaccines, and NK cell therapies hold several promises, but several challenges must be addressed before they can become widely adopted in clinical practice. One of the key issues is immune evasion by prostate cancer cells, which can modify their surface proteins or create an immunosuppressive microenvironment that limits the effectiveness of immune-based therapies[42]. Furthermore, significant immune-related adverse events, such as cytokine release syndrome (CRS), can lead to severe systemic inflammation and even death[43]. The cost of cell therapies is another major barrier to their widespread use. For instance, treatments with CAR T cell therapy and dendritic cell vaccines require personalized manufacturing processes, which drive up costs and limit accessibility. Efforts are ongoing to streamline production and develop off-the-shelf cell therapies, but these approaches are still in their early stages of development.
CLINICAL APPLICATIONS AND TRIALS IN PROSTATE CANCER TREATMENT
Gene and cell therapies for prostate cancer are at various stages of clinical trials, each designed to evaluate the safety, efficacy, and feasibility of these cutting-edge approaches. Many of the trials in phase I or II stages focus on determining the optimal dosing, safety profiles, and early signs of efficacy before progressing to larger-scale studies. One of the prominent gene therapy trials is evaluating the use of adenoviral vectors to deliver tumor suppressor genes like p53 directly to prostate tumors. These trials are investigating whether restoring the function of the p53 gene can halt tumor progression or lead to tumor shrinkage in patients with advanced prostate cancer. Early results from these trials have shown modest reductions in tumor size and stabilizations of the disease, although more extensive studies are needed to assess long-term outcomes[44]. See Figure 3.
Figure 3. Mechanism of Immunotherapy in prostate cancer[18].
Additionally, CRISPR-Cas9 technology is being tested in clinical settings to knock out specific mutations associated with resistance to androgen deprivation therapy (ADT), as presented in Figure 3. The potential for CRISPR to modify androgen receptor (AR) genes and restore sensitivity to ADT is a major focus in prostate cancer treatment. Preliminary data from phase I trials suggest that CRISPR-mediated gene editing has successfully reduced tumor burden in a small number of patients, though challenges such as off-target effects and efficient delivery of the CRISPR components need to be addressed before wider clinical implementation[45]. In terms of cell therapy, CAR T cell therapy targeting prostate-specific membrane antigen (PSMA) has entered phase I/II trials. These trials are investigating the safety and preliminary efficacy of CAR T cells in patients with metastatic castration-resistant prostate cancer (mCRPC) who have exhausted standard treatments. Early trial data suggest that CAR T cell therapy has led to partial tumor responses in some patients, though immune-related adverse events such as cytokine release syndrome (CRS) have been reported45[35]. These adverse effects are being closely monitored, with researchers working to refine CAR T cell designs to reduce toxicity and enhance therapeutic benefits. Cytokine release syndrome (CRS) is a potentially life-threatening condition that can occur after gene editing therapies, particularly those using CAR T cells or other immunotherapies. Signs and symptoms of CRS include high fever above 38 °C, fatigue, headaches, muscular pain, joint pain, diarrhea, Nausea and vomiting, and skin rash[46].
Given the limitations of gene and cell therapies when used alone, there is increasing interest in combining these therapies with existing treatment modalities such as chemotherapy, radiation, and immunotherapy. For example, sipuleucel-T, a dendritic cell-based vaccine, is used in combination with immune checkpoint inhibitors like PD-1 inhibitors to enhance the immune response against prostate cancer cells. Early-phase trials have shown that combining sipuleucel-T with checkpoint inhibitors can lead to enhanced immune activation and longer overall survival compared to sipuleucel-T alone[28]. Similarly, combining CAR T cell therapy with checkpoint inhibitors is being explored to overcome the immune exhaustion that sometimes occurs after CAR T cell infusion. By blocking inhibitory signals in the tumor microenvironment, checkpoint inhibitors can enhance the persistence and function of CAR T cells, potentially leading to more durable responses in prostate cancer[47]. Several challenges remain in translating gene and cell therapies into standard prostate cancer treatments. Safety concerns are a significant barrier, particularly with CAR T cell therapy, where adverse events like CRS and neurotoxicity can be life-threatening[48]. Efforts to mitigate these side effects, such as using suicide genes to control CAR T cell activity, have optimized safety profiles. Gene and cell therapies are expensive to develop and manufacture, with personalized treatments like CAR T cell therapy costing hundreds of thousands of dollars per patient. Scaling these treatments for widespread use will require advancements in manufacturing processes and the development of off-the-shelf therapies that can be administered without the need for individualized cell modifications[49].
The clinical application of gene and cell therapies also raises ethical and regulatory concerns. The use of CRISPR-Cas9 and other gene-editing technologies, in particular, has sparked debate over the potential for germline editing. While germline editing is not currently being pursued in prostate cancer trials, the rapid advancement of gene-editing technologies has led to calls for stricter regulatory oversight to ensure that these therapies are used responsibly[50]. The regulatory approval process for gene and cell therapies is complex, with agencies such as the FDA and EMA requiring extensive safety and efficacy data before granting approval. This requirement contributes to delays in bringing these therapies to market, though accelerated approval pathways are being considered for treatments that show significant promise in early-phase trials.
CHALLENGES AND LIMITATIONS OF GENE AND CELL THERAPY FOR PROSTATE CANCER
Safety concerns
One of the most significant challenges in gene and cell therapy for prostate cancer is safety. Even though these therapies hold tremendous potential, they can also introduce serious adverse effects. For example, CAR T cell therapy has been associated with severe cytokine release syndrome (CRS) and neurotoxicity, which can be life-threatening if not properly managed. CRS occurs when the infused CAR T cells activate the immune system to an extreme degree, resulting in a massive release of cytokines that cause systemic inflammation. Managing these side effects requires careful monitoring and, in some cases, administration of drugs like tocilizumab (an IL-6 receptor antagonist) to control the inflammatory response[48]. However, the risk of these side effects continues to limit the widespread use of CAR T cell therapy in prostate cancer treatment. In gene therapy, safety concerns are tied to the vectors that deliver genetic material. Viral vectors such as adenoviruses, commonly used in gene therapy, can cause immunogenic reactions or insert themselves into the wrong part of the genome, potentially leading to oncogenesis (development of new cancers). This risk necessitates long-term follow-up of patients undergoing gene therapy to monitor for any adverse events, including the development of secondary cancers[51].
Delivery challenges
A major limitation of gene and cell therapies is that they are not effective at the target site. For gene therapy, the challenge is ensuring that the therapeutic gene is delivered to prostate tumor cells without affecting healthy tissue. Most gene therapies rely on viral vectors to deliver the gene, but these vectors must be accurately targeted to avoid off-target effects and toxicity. Non-viral methods, such as nanoparticles or lipid-based systems, are being developed as alternatives but are still in the experimental stages and may not yet provide the same level of efficiency as viral vectors[52]. In cell therapies, particularly CAR T cell therapy, the challenge lies in getting the CAR T cells to infiltrate the tumor microenvironment. Solid tumors like prostate cancer have a highly immunosuppressive microenvironment, which makes it difficult for T cells to penetrate the tumor and exert their effects. Prostate cancer tumors also tend to express low levels of antigens, such as PSMA, making it harder for CAR T cells to recognize and attack the cancer cells; therefore, overcoming these delivery challenges is critical for improving the efficacy of both gene and cell therapies[53].
Tumor resistance and heterogeneity
Gene and cell therapies for prostate cancer face the challenge of tumor heterogeneity. Different regions of the tumor may have distinct genetic and molecular characteristics. This heterogeneity can make it difficult for a single therapy to target all the cancerous cells. CRISPR-Cas9-based gene therapies may be able to knock out a specific mutation in one part of the tumor, but other regions of the tumor may harbor different mutations that allow them to continue growing[54]. Similarly, tumors can develop resistance to therapies over time, leading to treatment failure. In CAR T cell therapy, tumors may downregulate the expression of the antigen that the CAR T cells are designed to target, often leading to immune escape and treatment failure. This has been observed in some patients undergoing CAR T cell therapy for prostate cancer, where a loss of PSMA expression allowed the tumor to evade the immune system and continue progressing[55]. However, endotoxins have been reported to increase the expression of PSMA, which counterbalances the problem associated with PSMA reduction. Developing strategies to overcome tumor resistance, such as engineering CAR T cells that target multiple antigens, is an area of active research.
Cost and accessibility
The high cost of gene and cell therapies is another major limitation, particularly the widespread clinical use. CAR T cell therapy involves an individualized manufacturing process where a patient’s T cells are harvested, genetically modified, expanded, and then re-infused. This process can cost hundreds of thousands of dollars per patient, making it inaccessible to many individuals and unsustainable for a larger healthcare system[56]. Gene therapies also involve high costs due to the complexity of developing and producing viral vectors and other delivery systems. These financial barriers limit the availability of gene and cell therapies to only a small patient population, particularly those in high-income countries. Efforts to reduce costs through automation, streamlining production, and the development of off-the-shelf cell therapies are ongoing, yet these solutions are still years away from becoming widely available[48].
Ethical concerns
Ethical issues surrounding gene and cell therapy also pose significant challenges. The use of CRISPR-Cas9 technology raises concerns about the potential for germline editing, where genetic changes made in a patient could be passed down to future generations. Although current clinical trials focus on somatic gene editing (which affects only the patient), the rapid advancements in gene-editing technology have sparked concerns about potential misuse, particularly for non-therapeutic purposes (e.g., designer babies)[39]. There are also ethical concerns regarding the fairness of access to these therapies, given their high cost and limited availability. Gene and cell therapies are currently only available at a few specialized medical centers, primarily in wealthier countries, despite trials/seeking of legislative approval ongoing in developing countries. This raises concerns about health equity as patients in low- and middle-income countries may not have access to these potentially life-saving treatments[56].
Regulatory hurdles
Gene and cell therapies face complex regulatory hurdles before they are approved for widespread use. Regulatory agencies such as the FDA and EMA require extensive safety and efficacy data sets, which can take years to collect in clinical trials. This long approval process delays the availability of new therapies and increases development costs[57]. There are also challenges in developing appropriate quality control measures for manufacturing gene and cell therapies. This is due to their complex and personalized nature. In response to these challenges, some regulatory agencies have introduced accelerated approval pathways for gene and cell therapies that show promising early results. However, these pathways still require rigorous post-market surveillance to ensure that these therapies are safe and effective in the long term. Therefore, there is a need for phase 4 clinical studies for all licensed gene editing and cell therapy modalities in the post-market setting.
FUTURE DIRECTIONS AND PROSPECTS FOR GENE AND CELL THERAPY IN PROSTATE CANCER
Emerging technologies
The future of gene and cell therapy in prostate cancer treatment will be shaped by several emerging technologies that promise to address current limitations and enhance therapeutic efficacy. Next-generation CAR T cell technology is focused on improving the specificity and safety of these therapies. One major approach is dual-targeted CAR T cells engineered to recognize multiple antigens in prostate cancer cells. This can potentially overcome antigen escape and enhance treatment response. CAR T cells targeting both PSMA and prostate stem cell antigen (PSCA) have shown promise in preclinical models by targeting a broader range of tumor cells and reducing the risk of antigen loss[58]. Moreover, ARM-CAR T cells are being explored to improve the persistence and functionality of CAR T cells within the hostile tumor microenvironment. These cells are designed with enhanced signaling domains that can sustain T cell activation and survival, potentially leading to more responses in patients[59].
Innovations in gene delivery systems are addressing the challenges associated with targeting and transformation efficiency. Nanoparticle-based delivery systems are being developed to deliver therapeutic genes with precision and reduced immunogenicity. Lipid nanoparticles and polymer-based carriers are non-viral vectors optimized for more effective gene transfer and reduced off-target effects. These systems can also be engineered to release their payloads in response to specific stimuli present in the tumor microenvironment, enhancing targeted delivery[60]. Epigenetic modulations are gaining traction as they can modify the expression of genes involved in tumor progression without altering the underlying DNA sequence. Small molecules and epigenetic editing tools are being investigated to reverse aberrant gene silencing or activation that contributes to prostate cancer development. For instance, drugs targeting DNA methylation and histone modification are showing potential in reactivating tumor suppressor genes and enhancing the efficacy of existing treatments[61].
Furthermore, combining gene and cell therapies with other modalities like oncolytic viruses continues to be a key area that selectively infects and kills cancer cells. This combined gene therapy enhances the oncolytic effect and promotes systemic antitumor immunity. For example, oncolytic viruses expressing therapeutic genes that stimulate immune responses or sensitize tumors to other treatments are showing promise in preclinical studies[62]. Integrating gene and cell therapies with immunotherapies such as immune checkpoint inhibitors is an active area of research. A combination of these approaches can potentially overcome immune evasion mechanisms and boost the overall effectiveness of treatment. Preliminary studies combining CAR T cells with checkpoint inhibitors can lead to improved clinical outcomes by enhancing T cell activity and prolonging their persistence in the tumor[63]. Streamlining regulatory processes to accelerate the development and approval of these therapies will ensure that patient safety is a priority. Regulatory agencies are exploring adaptive pathways and real-world evidence to expedite the approval process for promising therapies[57]. Ethically, there will be a need for ongoing discussions about equitable access to these advanced therapies. It is crucial to address health disparities and ensure that all patients, regardless of socioeconomic status, have access to these potentially life-saving treatments[56]. International collaboration and knowledge sharing are essential to advance gene and cell therapies for prostate cancer.
Collaborative efforts among academic institutions, pharmaceutical companies, and regulatory agencies can help standardize protocols, share data, and accelerate the development and dissemination of these therapies. More so, global initiatives and consortia focused on prostate cancer research and therapy development are likely to play a significant role in shaping the future landscape of treatment[49].
FUTURE PROSPECTS AND RESEARCH DIRECTIONS
The future of gene and cell therapies for prostate cancer appears promising, with ongoing research and technological advancements paving the way for more effective and personalized treatments. Continued development in next-generation CAR T cells, advanced gene delivery systems, and combination therapies is expected to enhance treatment outcomes and overcome current limitations. Therefore, addressing cost and accessibility issues, along with regulatory and ethical challenges, will be essential for translating these advancements into widespread clinical practice. Future research should focus on expanding clinical trials to include diverse patient populations and assessing long-term outcomes, as well as investigating the mechanisms of resistance and tumor heterogeneity to refine therapeutic approaches and technological innovation involved in the development and validation of new technologies for gene and cell therapy delivery. Health policies aim to ensure that advancements in gene and cell therapies are accessible to all patients, irrespective of socioeconomic status.
CONCLUSION
Gene and cell therapies represent a transformative shift in the treatment landscape for prostate cancer in Africa medical landscape, offering new hope for patients with advanced or refractory disease. This comprehensive review has explored the current state of these therapies, highlighting their potential benefits, emerging advancements, and ongoing challenges. Viral vector-mediated delivery of therapeutic genes and CRISPR-Cas9 gene editing have shown potential in targeting specific genetic mutations and enhancing the expression of tumor suppressor genes. CAR T cell therapy has demonstrated significant efficacy in targeting prostate cancer cells, although challenges related to safety and delivery remain. Innovations such as dual-targeted CAR T cells and ARM-CAR T cells aimed at improving efficacy and safety. Advances in nanoparticle-based and non-viral delivery systems hold promise for enhancing targeted delivery and reducing off-target effects. New approaches in epigenetic therapies also offer the potential for reversing aberrant gene expression associated with prostate cancer. Combination therapies such as oncolytic viruses and immunotherapies could enhance overall treatment efficacy. Addressing regulatory hurdles, ensuring equitable access, and navigating ethical issues are crucial for advancing these therapies.
DECLARATIONS
Authors’ contributions
Conceptualization, writing and editing, correspondence: Oboma YI
Drafting, revising and editing, proofreading: Ekpenyong B
Data extraction, article screening, and literature search: Charles M. A, Kenneth E. M
Availability of data and materials
Not applicable.
Financial support and sponsorship
Not applicable.
Conflicts of interest
All authors declared that there are no conflicts of interest.
Ethical approval and consent to participate
Not applicable.
Consent for publication
Not applicable.
Copyright
© The Author(s) 2025.
REFERENCES
1. Key statistics for prostate cancer. 2024. Available from: https://www.cancer.org/cancer/types/prostate-cancer/about/key-statistics.html [Last accessed on 20 Mar 2025].
3. Wang Y, Li X, You Z. Comparative analysis of the incidence, diagnosis and treatment of prostate cancer between China and the United States. Serican J Med. 2025:2.
4. Prostate Cancer Foundation. 2022. Available from: https://www.pcf.org [Last accessed on 20 Mar 2025].
5. Chen T, Wang M, Chen Y, Liu Y. Current challenges and therapeutic advances of CAR-T cell therapy for solid tumors. Cancer Cell Int. 2024;24:133.
6. Stefanoudakis D, Kathuria-Prakash N, Sun AW, et al. The potential revolution of cancer treatment with CRISPR technology. Cancers. 2023;15:1813.
7. Li YR, Halladay T, Yang L. Immune evasion in cell-based immunotherapy: unraveling challenges and novel strategies. J Biomed Sci. 2024;31:5.
8. Logotheti S, Papadaki E, Zolota V, et al. Lineage plasticity and stemness phenotypes in prostate cancer: harnessing the power of integrated “omics” approaches to explore measurable metrics. Cancers. 2023;15:4357.
9. Chen L, Li Q, Wang Y, Zhang Y, Ma X. Comparison on efficacy of radical prostatectomy versus external beam radiotherapy for the treatment of localized prostate cancer. Oncotarget. 2017;8:79854-63.
10. Desai K, McManus JM, Sharifi N. Hormonal therapy for prostate cancer. Endocr Rev. 2021;42:354-73.
11. Corn PG, Heath EI, Zurita A, et al. Cabazitaxel plus carboplatin for the treatment of men with metastatic castration-resistant prostate cancers: a randomised, open-label, phase 1-2 trial. Lancet Oncol. 2019;20:1432-43.
12. Michaelson MD, Cotter SE, Gargollo PC, Zietman AL, Dahl DM, Smith MR. Management of complications of prostate cancer treatment. CA Cancer J Clin. 2008;58:196-213.
13. Talkar SS, Patravale VB. Gene therapy for prostate cancer: a review. Endocr Metab Immune Disord Drug Targets. 2021;21:385-96.
14. Gudenkauf LM, Gray S, Gonzalez BD, Sachdeva A, Autio K. Balancing hormone therapy: mitigating adverse effects of androgen-deprivation therapy and exploring alternatives in prostate cancer management. Am Soc Clin Oncol Educ Book. 2024;44:e433126.
15. Dogbey DM, Torres VES, Fajemisin E, et al. Technological advances in the use of viral and non-viral vectors for delivering genetic and non-genetic cargos for cancer therapy. Drug Deliv Transl Res. 2023;13:2719-38.
16. Eade TN, Hanlon AL, Horwitz EM, Buyyounouski MK, Hanks GE, Pollack A. What dose of external-beam radiation is high enough for prostate cancer? Int J Radiat Oncol Biol Phys. 2007;68:682-9.
17. Osuchowski M, Osuchowski F, Latos W, Kawczyk-Krupka A. The use of upconversion nanoparticles in prostate cancer photodynamic therapy. Life. 2021;11:360.
18. Runcie KD, Dallos MC. Prostate Cancer immunotherapy-finally in from the cold? Curr Oncol Rep. 2021;23:88.
19. Kanu GA, Parambath JBM, Abu Odeh RO, Mohamed AA. Gold nanoparticle-mediated gene therapy. Cancers. 2022;14:5366.
20. Allami P, Heidari A, Rezaei N. The role of cell membrane-coated nanoparticles as a novel treatment approach in glioblastoma. Front Mol Biosci. 2022;9:1083645.
21. Beach MA, Nayanathara U, Gao Y, et al. Polymeric nanoparticles for drug delivery. Chem Rev. 2024;124:5505-616.
22. Bajpai S, Tiwary SK, Sonker M, et al. Recent advances in nanoparticle-based cancer treatment: a review. ACS Appl Nano Mater. 2021;4:6441-70.
23. Lee NH, You S, Taghizadeh A, Taghizadeh M, Kim HS. Cell membrane-cloaked nanotherapeutics for targeted drug delivery. Int J Mol Sci. 2022;23:2223.
24. Kadry MO, Abdel-Megeed RM. CRISPR-Cas9 genome and long non-coding RNAs as a novel diagnostic index for prostate cancer therapy via liposomal-coated compounds. Plos One. 2024;19:e0302264.
25. Zhang H, Qin C, An C, et al. Application of the CRISPR/Cas9-based gene editing technique in basic research, diagnosis, and therapy of cancer. Mol Cancer. 2021;20:126.
26. Tabibian M, Moghaddam FS, Motevaseli E, et al. Targeting mRNA-coding genes in prostate cancer using CRISPR/Cas9 technology with a special focus on androgen receptor signaling. Cell Commun Signal. 2024;22:504.
27. Kumari S, Sharma V, Tiwari R, Maurya JP, Subudhi BB, Senapati D. Therapeutic potential of p53 reactivation in prostate cancer: strategies and opportunities. Eur J Pharmacol. 2022;919:174807.
28. Belete TM. The current status of gene therapy for the treatment of cancer. Biol Targets Ther. 2021;2021:67-77.
29. Altanerova U, Jakubechova J, Benejova K, et al. Intracellular prodrug gene therapy for cancer mediated by tumor cell suicide gene exosomes. Int J Cancer. 2021;148:128-39.
30. Yanagisawa N, Satoh T, Tabata KI, et al. Cytopathic effects and local immune responses in repeated neoadjuvant HSV-tk + ganciclovir gene therapy for prostate cancer. Asian J Urol. 2021;8:280-8.
31. Dhankhar R, Kawatra A, Mohanty A, Gulati P. Microbial enzymes used in prodrug activation for cancer therapy: insights and future perspectives. Curr Protein Pept Sci. 2021;22:514-25.
32. Mani R, Martin CG, Balu KE, et al. A novel protozoa parasite-derived protein adjuvant is effective in immunization with cancer cells to activate the cancer-specific protective immunity and inhibit the cancer growth in a murine model of colorectal cancer. Cells. 2024;13:111.
33. Li J, Zeng H, Li L, Song M, Dong M. Biomembrane-wrapped gene delivery nanoparticles for cancer therapy. Front Bioeng Biotechnol. 2023;11:1211753.
34. Wang I, Song L, Wang BY, Rezazadeh Kalebasty A, Uchio E, Zi X. Prostate cancer immunotherapy: a review of recent advancements with novel treatment methods and efficacy. Am J Clin Exp Urol. 2022;10:210-33.
35. Wang F, Li Z, Feng X, et al. Advances in PSMA-targeted therapy for prostate cancer. Prostate Cancer Prostatic Dis. 2022;25:11-26.
36. Flugel CL, Majzner RG, Krenciute G, et al. Overcoming on-target, off-tumour toxicity of CAR T cell therapy for solid tumours. Nat Rev Clin Oncol. 2023;20:49-62.
37. Escudero-Lourdes C, Alvarado-Morales I, Tokar EJ. Stem cells as target for prostate cancer therapy: opportunities and challenges. Stem Cell Rev Rep. 2022;18:2833-51.
38. Hafron JM, Wilfehrt HM, Ferro C, Harmon M, Flanders SC, McKay RR. Real-world effectiveness of sipuleucel-T on overall survival in men with advanced prostate cancer treated with androgen receptor-targeting agents. Adv Ther. 2022;39:2515-32.
39. Zanotta S, Galati D, De Filippi R, Pinto A. Enhancing dendritic cell cancer vaccination: the synergy of immune checkpoint inhibitors in combined therapies. Int J Mol Sci. 2024;25:7509.
40. Perera Molligoda Arachchige AS. Human NK cells: from development to effector functions. Innate Immun. 2021;27:212-29.
41. Gong Y, Klein Wolterink RGJ, Wang J, et al. Chimeric antigen receptor natural killer (CAR-NK) cell design and engineering for cancer therapy. J Hematol Oncol. 2021;14:73.
42. Bou-Dargham MJ, Sha L, Sang QA, Zhang J. Immune landscape of human prostate cancer: immune evasion mechanisms and biomarkers for personalized immunotherapy. BMC Cancer. 2020;20:572.
43. Liu LL, Skribek M, Harmenberg U, Gerling M. Systemic inflammatory syndromes as life-threatening side effects of immune checkpoint inhibitors: case report and systematic review of the literature. J Immunother Cancer. 2023;11:e005841.
44. Hong B, van den Heuvel AP, Prabhu VV, Zhang S, El-Deiry WS. Targeting tumor suppressor p53 for cancer therapy: strategies, challenges and opportunities. Curr Drug Targets. 2014;15:80-9.
45. Han HA, Pang JKS, Soh BS. Mitigating off-target effects in CRISPR/Cas9-mediated in vivo gene editing. J Mol Med. 2020;98:615-32.
46. Shimabukuro-Vornhagen A, Gödel P, Subklewe M, et al. Cytokine release syndrome. J Immunother Cancer. 2018;6:56.
47. Kim TJ, Koo KC. Current status and future perspectives of checkpoint inhibitor immunotherapy for prostate cancer: a comprehensive review. Int J Mol Sci. 2020;21:5484.
48. Freyer CW, Porter DL. Cytokine release syndrome and neurotoxicity following CAR T-cell therapy for hematologic malignancies. J Allergy Clin Immunol. 2020;146:940-8.
49. Abou-el-Enein M, Elsallab M, Feldman SA, et al. Scalable manufacturing of CAR T cells for cancer immunotherapy. Blood Cancer Dis. 2021;2:408-22.
51. Pipe SW, Reddy KR, Chowdary P. Gene therapy: practical aspects of implementation. Haemophilia. 2022;28 Suppl 4:44-52.
52. Zu H, Gao D. Non-viral vectors in gene therapy: recent development, challenges, and prospects. AAPS J. 2021;23:78.
53. Weimin S, Abula A, Qianghong D, Wenguang W. Chimeric cytokine receptor enhancing PSMA-CAR-T cell-mediated prostate cancer regression. Cancer Biol Ther. 2020;21:570-80.
54. Fisher R, Pusztai L, Swanton C. Cancer heterogeneity: implications for targeted therapeutics. Br J Cancer. 2013;108:479-85.
55. Narayan V, Barber-Rotenberg JS, Jung IY, et al. PSMA-targeting TGFβ-insensitive armored CAR T cells in metastatic castration-resistant prostate cancer: a phase 1 trial. Nat Med. 2022;28:724-34.
56. Quinn C, Young C, Thomas J, Trusheim M. MIT NEWDIGS FoCUS Writing Group. Estimating the clinical pipeline of cell and gene therapies and their potential economic impact on the US healthcare system. Value Health. 2019;22:621-6.
58. Porter LH, Harrison SG, Risbridger GP, Lister N, Taylor RA. Left out in the cold: Moving beyond hormonal therapy for the treatment of immunologically cold prostate cancer with CAR T cell immunotherapies. J Steroid Biochem Mol Biol. 2024;243:106571.
59. Ohomoimen G, Oboma YI, Beredugo S, Beredugo L. MutS homolog 2 and MutS homolog 3 genes are equivocal in prostate disease diagnosis: a study from Niger Delta University Teaching Hospital (NDUTH) in Okolobiri, Bayelsa State, Nigeria. Acad J Health Sci. 2024;39:23.
60. Patra D. Polymer-based nanoparticles as efficient non-viral vectors for gene delivery in CAR-T cell therapy. Exon. 2024;1:87-96.
61. Patnaik S, Anupriya. Drugs targeting epigenetic modifications and plausible therapeutic strategies against colorectal cancer. Front Pharmacol. 2019;10:588.
62. Shalhout SZ, Miller DM, Emerick KS, Kaufman HL. Therapy with oncolytic viruses: progress and challenges. Nat Rev Clin Oncol. 2023;20:160-77.
Cite This Article

How to Cite
Download Citation
Export Citation File:
Type of Import
Tips on Downloading Citation
Citation Manager File Format
Type of Import
Direct Import: When the Direct Import option is selected (the default state), a dialogue box will give you the option to Save or Open the downloaded citation data. Choosing Open will either launch your citation manager or give you a choice of applications with which to use the metadata. The Save option saves the file locally for later use.
Indirect Import: When the Indirect Import option is selected, the metadata is displayed and may be copied and pasted as needed.
About This Article
Special Issue
Copyright
Data & Comments
Data
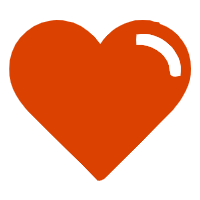
Comments
Comments must be written in English. Spam, offensive content, impersonation, and private information will not be permitted. If any comment is reported and identified as inappropriate content by OAE staff, the comment will be removed without notice. If you have any queries or need any help, please contact us at [email protected].