The landscape of current and future therapeutic opportunities for Fabry disease
Abstract
Fabry disease is a rare genetic disorder classified as a lysosomal storage disease. It is an X-linked disease, caused by the mutation of the GLA gene, leading to the deficit or absence of function of the enzyme α-galactosidase A. It is a multi-organ and progressive disease characterized by systemic involvement primarily affecting the cardiac, renal and neurological systems. Current treatment options include established therapies such as two enzyme replacement therapies (agalsidase α, agalsidase β), one chaperone treatment (migalastat), and a recently approved enzyme replacement therapy targeting pegunigalsidase α. New drugs are being developed, including substrate reduction therapy, mRNA therapy, and genetic therapy. These emerging treatments have the potential to address the limitations of current therapies and ensure more effective and personalized treatment. This review explores and analyzes the diverse therapeutic strategies available for treating this complex and intriguing disease.
Keywords
INTRODUCTION
Fabry disease (FD) is caused by mutations in the GLA gene, located on the X chromosome, which encodes
FD exhibits a wide range of clinical manifestations influenced by specific mutations in the GLA gene and gender. Due to its X-linked inheritance, males typically experience more severe symptoms and earlier onset of complications compared to females[3].
FD presents a diverse range of manifestations that progress through different life stages. During childhood and adolescence, patients typically experience chronic or episodic acroparesthesia, angiokeratomas, ophthalmologic abnormalities, auditory neuropathy, disorders of sweating, increased albuminuria, and gastrointestinal disturbances. Young adulthood is marked by more pronounced angiokeratomas, selective proteinuria, fever, gastrointestinal symptoms, and early-onset cardiac abnormalities. In later adulthood
In males, the distinction between “classical” and “non-classical” forms of FD is determined by the residual activity of α-GAL A enzyme, preferably evaluated in leukocytes, along with the presence of characteristic clinical manifestations. The classic form of the disease is characterized by negligible or minimal (< 1%) activity of the α-GalA enzyme, which results in early-onset and widespread multi-organ complications. On the other hand, the attenuated or later-onset variant appears later in life, with symptoms that vary depending on the residual levels of α-GalA activity[6]. Heterozygous females exhibit variable clinical manifestations, and enzyme activity alone does not consistently distinguish between different phenotypes. It is hypothesized that severe disease in females heterozygous for an X-linked disorder results from unfavorable skewing during Lyonization, leading to biased inactivation of the wild-type allele in the majority of tissues. As a result, in females, classification relies on genetic mutation analysis, family history, and comprehensive clinical and biochemical evaluations[7].
Current therapeutic strategies for FD include enzyme replacement therapy (ERT) and oral chaperone treatment. The ERT category includes agalsidase α, agalsidase β and pegunigalsidase α, recombinant α-Gal A proteins that are effective in symptom control and in decelerating or arresting disease progression[8,9]. Chaperone therapy with Migalastat works by stabilizing the residual endogenous enzyme, correcting its misfolding, and thereby restoring the degradation of glycolipids[10]. Numerous other molecules are currently under investigation[11]. A novel form of ERT, moss-αGal, derived from plants has been shown to be effective, safe, and well-tolerated in early studies so far[12].
An additional therapeutic strategy involves substrate reduction therapy (SRT), which is designed to attenuate the production of metabolic products that cannot be degraded due to the inherent enzymatic deficiency. In this context, Venglustat and Lucerastat inhibit glucosylceramide synthase (GCS) to limit the amount of metabolites not degradable due to the lack of α-GalA enzyme[13,14].
Further advancements in therapeutic approaches for FD are represented by gene therapy. This strategy is designed to direct the therapy toward circulating hematopoietic cells using a recombinant vector capable of transducing and correcting the target cells. These cells would then acquire the ability to produce significant amounts of α-galactosidase A, both intracellularly and in their secretions[15,16].
Beyond genetic interventions, systemic messenger RNA (mRNA)-based therapeutics are under development for FD. This approach aims to enhance endogenous protein synthesis, thereby improving the conformational maturation, glycosylation, and distribution of α-GAL A in cells[17].
Finally, in vitro studies have explored strategies to reduce intra-lysosomal storage by enhancing the efflux of stored substances from the lysosomal vesicle and facilitating its subsequent expulsion from the cell[18,19] [Figure 1].
This review aims to comprehensively evaluate all current and emerging therapeutic strategies for FD.
CURRENTLY AVAILABLE THERAPY FOR FABRY DISEASE
Enzyme replacement therapy
Currently available Enzyme replacement therapy (ERT) for FD include agalsidase α (Replagal, Takeda), produced in human fibroblasts and administered at a dose of 0.2 mg/kg biweekly, and agalsidase β (Fabrazyme, Sanofi Genzyme), produced in Chinese hamster ovary cells and administered at a dose of
Pegunigalsidase α is a new ERT, produced by Protalix Biotherapeutics in Israel. This drug, recently approved by the FDA, has improved stability and half-life thanks to PEGylation.
Agalsidase β
Numerous clinical studies have investigated the effects of ERT in FD patients and many studies conducted over the years have demonstrated the efficacy of agalsidase β[25]. Agalsidase β has been shown to slow the progression of renal, cardiac, and cerebrovascular complications in advanced FD patients compared to placebo, with early treatment initiation being associated with better outcomes[26].
Weidemann et al. showed that ERT with agalsidase β improved myocardial function in FD patients by increasing peak systolic strain rate. These findings suggest that early ERT may mitigate left ventricular hypertrophy[27].
A small observational study confirmed that agalsidase β reduced lyso-Gb3 levels more effectively than agalsidase α[28]. Given the strong correlation between plasma lyso-Gb3 levels and disease severity, targeting this biomarker is crucial for assessing treatment outcomes[29-31].
Messalli et al. reported significant improvements in cardiac MRI parameters, including reduced myocardial T2 relaxation time, maximal myocardial thickness, and total left ventricular mass, after 48 months of agalsidase β treatment[32].
Agalsidase β facilitates the clearance of Gb3 deposits, as assessed in renal biopsy studies. The clearance is time- and dose-dependent, becoming evident after just 5 months of therapy with agalsidase β compared to placebo, and affects the cells most resistant to treatment, such as podocytes and distal tubular epithelium[33,34].
Both agalsidase β and agalsidase α are effective in slowing the progression of FD and are considered safe[35]. Arends et al. compared agalsidase α and agalsidase β, finding no difference in complication rates. However, patients treated with agalsidase β exhibited a more pronounced reduction in lyso-Gb3 concentrations and a more favorable impact on left ventricular mass, particularly in those with LVMI < 75 g/m2[36].
Severe anaphylaxis is rare with ERT, and infusion-related reactions are typically manageable with pre-infusion antipyretic drugs and low-dose corticosteroids.
The development of anti-drug antibodies (ADAs), typically within 3 to 6 months from starting therapy, can diminish ERT efficacy by reducing lyso-Gb3 clearance[37]. Agalsidase β appears to be associated with a higher incidence of neutralizing antibodies, possibly due to differences in the manufacturing process[7].
Agalsidase α
The efficacy of agalsidase α has also been widely demonstrated. In the study by Beck et al., which compared patients in the Fabry Outcome Survey (FOS) treated with agalsidase α to cohorts of untreated patients, a slower decline in renal function was observed in treated patients compared to untreated ones[38]. The same study also demonstrated a later onset of renal, cardiac and neurological complications, as well as delayed mortality in patients treated with agalsidase α[38].
A retrospective analysis conducted by Kampmann et al. at the University Children’s Hospital in Mainz, Germany, involved prospective data collection from medical records of FD patients under their care[39]. After ten years of treatment, heart failure improved in 22 of 42 patients, and angina scores remained stable or improved in 41 patients, highlighting the treatment’s significance for cardiomyopathy symptoms[39].
Due to a global shortage of agalsidase β between 2009 and 2012, many patients were switched to agalsidase
In a randomized, double-blind, placebo-controlled clinical trial, Hughes et al. demonstrated the efficacy of agalsidase α in reversing hypertrophic cardiomyopathy in FD patients[41]. MRI studies revealed a reduction in left ventricular mass after 6 months of treatment with agalsidase α compared to placebo, accompanied by a mean 20% decrease in myocardial Gb3 content, as evidenced by multiple myocardial biopsies[41].
Pegunigalsidase α
Pegunigalsidase α (PRX-102) is a chemically cross-linked and PEGylated α-Gal A generated within the cells of the tobacco plant. It is added with a molecule of polyethylene glycol (PEG) to enhance the enzyme’s stability and extend its half-life. Since this enzyme is plant-derived, it does not present M6P on its surface glycans, indicating a different mechanism of cellular uptake and potentially leading to a distinct biodistribution profile compared to agalsidase α and agalsidase β[42,43].
In vitro studies using human plasma and in vivo studies using a mouse model have demonstrated its greater steadiness. The murine model showed superior biodistribution of pegunigalsidase α relative to agalsidase α, with significant reductions in Gb3 deposits in heart and kidney tissues[43].
An open-label phase I/II trial demonstrated that pegunigalsidase α has a significantly longer plasma half-life of 80 h, compared to less than 1 h for agalsidase β in 16 patients, thanks to its distinct pharmacokinetics and low immunogenicity. Additionally, a reduction in Gb3 by 84% was observed in kidney biopsies following treatment[12].
The 2-year BALANCE study, a randomized phase III trial, evaluated the non-inferiority of pegunigalsidase α compared to agalsidase β in adult patients with FD who had received agalsidase β for at least one year and exhibited an annualized estimated glomerular filtration rate (eGFR) decline of more than
The BRIDGE trial, another important phase III open-label study, assessed the safety and efficacy of pegunigalsidase α (1 mg/kg every 2 weeks) in adults with FD who had previously been treated with agalsidase α (0.2 mg/kg every 2 weeks) for at least 2 years. The results demonstrated a reduction in the slope of eGFR in patients treated with pegunigalsidase α compared to previous treatment with agalsidase α and no adverse impact of anti-pegunigalsidase α antibodies on eGFR decline[45].
The results from the phase III, open-label, BRIGHT study indicated that patients with FD receiving ERT every other week can successfully switch to pegunigalsidase α (2 mg/kg every 4 weeks), demonstrating that this regimen could be an effective maintenance therapy with a favorable safety profile[46].
PEGylation appears to conceal certain epitopes from recognition by the immune system, which leads to a reduction in immunogenicity. This process involves attaching polyethylene glycol (PEG) molecules to therapeutic proteins, effectively shielding them from immune cells that would otherwise trigger an immune response. Furthermore, research has demonstrated that pegunigalsidase can not only reduce the formation of anti-drug antibodies but also reverse existing ones. This action can promote immune tolerance, making the therapy more effective and less likely to cause adverse immune reactions[12,43].
Although rare cases of hypersensitivity reactions and a case of immune-complex-mediated glomerulonephritis have been reported, the drug has been approved at a recommended dose of 1 mg/kg every 2 weeks by the European Medicines Agency and the Food and Drug Administration. It represents a promising new therapeutic alternative for FD[47].
Chaperone therapy
Migalastat
Although ERTs are effective, they have several limitations: the need for intravenous administration, which may reduce patient compliance or negatively impact the quality of life; the inability to cross the blood-brain barrier; and the potential development of anti-drug antibodies.
An alternative treatment for FD is chaperone therapy, which aims to assist mutated α-GAL A proteins in correctly folding, thereby enhancing their stability and function within the lysosome. However, it is only effective for patients with specific “amenable” mutations in the GLA gene genetic variants that result in a qualitatively anomalous production of the misfolded, unstable enzymes. Amenable mutations can be responsible for both classic and late-onset phenotypes[48].
Migalastat, a representative chaperone drug, binds to a specific sugar molecule (iminosugar) within the catalytic domain of the mutant α-GAL A. This binding helps the enzyme fold correctly and facilitates its transport to the lysosome, where it can degrade the accumulated substrate (Gb3).
A mutation is considered responsive to migalastat if α-Gal A activity increases by at least 1.20 times the baseline level, with an absolute increase of at least 3.0% in wild-type α-Gal A activity when exposed to
Migalastat is a potent inhibitor of α-GAL A at high doses. However, at lower doses, it paradoxically increases enzyme activity for specific GLA variants[49]. This seemingly contradictory effect is believed to occur because iminosugar initially binds to the enzyme, promoting proper folding. Once the enzyme reaches the lysosome, the competitive inhibitor (iminosugar) is displaced by the natural substrate, allowing α-GAL A to function normally. Migalastat is an oral medication administered at a dose of 123 mg every other day. It was approved by the European Medicines Agency (EMA) in 2016 for the treatment of FD patients aged 16 years and above, with an eGFR ≥ 30 mL/min/1.73 m2 and mutations amenable to chaperone therapy[50].
The FACETS trial compared chaperone therapy (Migalastat) with placebo in ERT-naïve patients with Migalastat-responsive GLA mutations. After 6 months of treatment, Migalastat significantly reduced plasma lyso-Gb3 levels and the number of Gb3 inclusions in kidney interstitial capillaries compared to placebo. Additionally, the first 6 months of Migalastat treatment resulted in a decrease in the total Gb3 inclusion volume per podocyte in kidney biopsies, which correlated with a reduction in the mean podocyte volume[51]. Furthermore, a significant reduction in the mean LVMI was observed at the 24-month mark compared to baseline[5].
The ATTRACT trial evaluated the effectiveness of Migalastat in ERT-experienced patients with amenable GLA mutations. Patients were randomized to either switch to Migalastat or continue ERT. After 18 months, Migalastat significantly reduced the mean left ventricular mass index compared to the ERT group, which showed no significant change. However, the design of this study has some limitations. The initial cohort included patients with non-amenable mutations in the Migalastat arm and a higher discontinuation rate in the ERT arm. As a result, only a small number of ERT patients (16 patients) remained evaluable by the study’s conclusion, which may potentially influence the reliability of the results[52].
Hughes et al. conducted a study to assess the long-term efficacy and safety of Migalastat[53]. They studied patients enrolled in the phase III clinical trial, FACET and ATTRACT, and they found that 97 patients treated with Migalastat for up to 8.6 years showed a low incidence of FACEs (renal, cardiac and neurological events), in both naïve and ERT-experienced patients[53].
While it remains uncertain whether Migalastat can cross the blood-brain barrier in humans, studies in a mouse model of FD have shown that Migalastat increases α-Gal A activity and reduces Gb3 levels in most tissues, including the brain[54,55].
NEW EMERGING THERAPIES
New potential therapeutic drugs are being explored in studies, including second-generation enzymes, substrate reduction therapies, and gene- and mRNA-based therapeutic strategies [Table 1].
Characteristics of emerging therapies
New therapy | Administration | Advantages | Trials |
Moss-galactosidase alfa | • Repeated administration | ◆ Safety and tolerability ◆ Prolonged reduction of Gb3 excretion ◆ Expression of the mannose receptor on kidney cells | • Phase 1 clinical trial (NCT02995993) |
Venglustat | • 15 mg once daily | ◆ Oral administration ◆ Linear pharmacokinetics ◆ Rapid absorption ◆ Favorable safety and tolerability profile ◆ Ability to pass the blood-brain barrier | • 3-year open-label phase 2 trials (NCT02228460 - NCT02489344) • PERIDOT (randomized, double-blind, placebo-controlled, 12-month Phase 3 study NCT05206773) • CARAT (phase III clinical trial NCT05280548) |
Lucerastat | • 100, 300, 500, 1,000 mg once daily • 200, 500, 1,000 mg b.i.d. | ◆ Oral administration ◆ Favorable safety and tolerability profile ◆ Ability to pass the blood-brain barrier | • SAD (single-ascending dose study NCT02944487) • MAD (multiple-ascending dose study NCT02944474) • MODIFY (multicenter, double-blind, randomized, placebo-controlled phase III study NCT03425539) |
Gene therapy | • Single administration | ◆ Long-term treatment ◆ All damaged cells and tissues involved | • Open-label phase I/II trial (NCT04519749) |
mRNA-based therapy | • Repeated administration | ◆ No immunogenicity ◆ Long-term treatment ◆ Endogenous α-Gal expression by the targeted tissues ◆ No risk of insertional mutagenesis ◆ Prolonged half-lives and duration | • Preclinical studies |
Enzyme replacement therapy
Moss α-galactosidase
Moss-α-galactosidase (moss-αGAL) is a plant-derived variant of α-galactosidase engineered to possess a
A phase 1 clinical trial confirmed the safety and tolerability of a single dose of moss-αGAL, accompanied by a sustained reduction in Gb3 excretion. Leveraging the mannose receptor, which is expressed on macrophages, endothelial cells, and kidney cells, moss-αGAL shows promise for further development. The positive results from phase 1 trials have paved the way for ongoing phase 2/3 clinical trials[57].
Substrate reduction therapies
The substrate reduction therapy (SRT) aims to decrease the deposits due to the accumulation of metabolites caused by the enzymatic defect, by blocking glucosylceramide synthase (GCS), thereby decreasing the synthesis of precursors to Gb3. The primary advantages of SRT are its oral administration and its ability to cross the blood-brain barrier. In patients with residual galactosidase-α activity (non-classical mutations), SRT may effectively reduce substrate levels to a point where the remaining enzyme can manage the metabolic load. Alternatively, SRT can be combined with ERT if α-GalA activity is below 1%[11,58].
Indeed, data from Fabry mice studies showed that adding substrate reduction to ERT may offer both complementary and synergistic benefits in the management of FD[59].
It is essential to operate with precaution in dosing, because complete inhibition of a single enzyme can disrupt cellular homeostasis, influencing processes such as apoptosis, cell growth, and differentiation.
Two molecules were developed for Fabry disease: Venglustat, produced by Sanofi Genzyme and Lucerastat, produced by Idorsia Pharmaceuticals, both of which inhibit glucosylceramide synthase (GCS).
Venglustat
Venglustat is a ceramide-based small molecule designed to inhibit GCS. By blocking GCS, Venglustat reduces the production of glucosylceramide, a precursor to glycosphingolipids.
Phase 1 studies have demonstrated its linear pharmacokinetics, rapid absorption, and a favorable safety and tolerability profile in healthy volunteers[60].
Data from a 26-week open-label Phase 2a study (NCT02228460) and a 130-week extension trial (NCT02489344) demonstrated the safety and efficacy of Venglustat in treatment-naïve adult males with FD (15 mg once daily oral dose)[61].
Patients treated with Venglustat exhibited decreased levels of key glycosphingolipid pathway markers. While proximal markers showed a rapid decline, more distal markers such as plasma Gb3 and globotriaosylsphingosine demonstrated a gradual reduction. Importantly, no biochemical or histological evidence of disease progression was observed over three years of follow-up.
However, the efficacy and safety of Venglustat need to be confirmed in larger studies.
A phase III clinical trial, CARAT, is currently underway to evaluate the effect of Venglustat on left ventricular mass index compared to traditional therapies[62].
There is another ongoing trial, the PERIDOT trial, that aims to evaluate the efficacy of Venglustat in reducing neuropathic and abdominal pain in treatment-naïve patients with FD[63].
The drug is also being evaluated for the treatment of other diseases, including Parkinson’s disease associated with GBA mutations, Gaucher disease type 3, GM2 gangliosidosis, and autosomal dominant polycystic kidney disease[60].
Lucerastat
Lucerastat, or N-butyldeoxygalactonojirimycin, is a soluble, low-molecular-weight molecule derived from galactose. It is administered orally and functions as a GCS inhibitor, restoring the normal balance of glycosphingolipid (GSL) synthesis, breakdown, and cellular transport[64].
Two separate randomized, double-blind, placebo-controlled studies evaluated the safety, tolerability, and pharmacokinetics of oral Lucerastat in healthy male volunteers.
In the single-ascending dose trial (SAD), 31 males got placebo or Lucerastat (a single oral dose of 100, 300, 500, or 1,000 mg). Other 8 subjects took 1,000 mg Lucerastat or placebo bis in die (two doses separated by 12 h).
In the multiple-ascending dose trial (MAD), 37 volunteers received either placebo or 200, 500, or 1,000 mg Lucerastat, twice daily for 7 consecutive days. To assess the impact of food on drug absorption, 6 participants in the 500 mg dose group received Lucerastat with or without food.
Data from both the SAD and MAD studies indicated that Lucerastat was well tolerated overall. These promising results have led to further investigations of SRT with Lucerastat in FD patients[64].
In a single-center, open-label, randomized study, patients were randomized to receive Lucerastat in addition to standard care, including ERT (10 patients), or ERT alone (4 patients). The Lucerastat treatment group showed a significant reduction in plasma concentrations of Gb3 and its precursors, glucosylceramide and lactosylceramide. However, no clinical improvement in cardiac and renal functions was observed, likely due to the study’s short duration of only 12 weeks[65].
Additional studies are ongoing to further evaluate the efficacy and safety of Lucerastat in adult FD patients[66].
Gene and mRNA-based therapies
The primary goal of gene therapies is a long-term treatment strategy that addresses all damaged cells and tissues in patients with FD.
In recent years, numerous preclinical studies have been conducted both in vivo and ex vivo, utilizing a variety of viral vectors, such as retroviral, lentiviral, adenoviral, and non-viral vectors.
The single-arm study NCT02800070 enrolled five male patients with classic FD who received autologous CD34+ hematopoietic stem cells engineered to express α-galactosidase A using a lentiviral vector. Initial data assessed the safety and tolerability, showing a reduction in plasma and urine levels of Gb3 and
Studies using Fabry knockout mice have employed recombinant adenoviral vectors expressing human
Other phase 1/2 studies testing in vivo gene therapy are currently underway. Various biological agents utilizing adeno-associated virus vectors are under investigation, including the liver- and cardiac-targeted 4D-310[69], as well as the liver-targeted ST-920[70] and FLT190[71].
Preliminary data from these studies show an increase in α-Gal A activity and a reduction in Gb3 levels.
mRNA-based therapy is one of the promising future therapeutic strategies for FD, as it enables endogenous α-Gal A expression in the targeted tissues, offering long-term safety and no immunogenicity. Unlike DNA-based therapies, mRNA-based therapy does not carry the risk of insertional mutagenesis.
However, the effects of mRNA-based therapy are transient, thus requiring repeated administration.
A preclinical study evaluated the systemic delivery of mRNA encoding human α-Gal A in wild-type (WT) mice, α-Gal A-deficient mice, and WT non-human primates (NHPs). Data indicate a dose-dependent increase in protein activity and subsequent substrate reduction in GLA-deficient mice following a single administration of h-α-Gal A mRNA. Importantly, these effects were sustained, with prolonged half-lives and substrate reduction observed in tissues and plasma for up to 6 weeks. Repeated administrations of h-α-Gal A mRNA demonstrated continued efficacy, safety, and pharmacological activity in a Fabry mouse model[17].
SYMPTOMATIC TREATMENT
The specific therapy for FD should be integrated with adjunctive interventions to manage the cardiac, renal, neurological, and other complications arising from chronic tissue damage[72].
For renal management, sodium-glucose cotransporter-2 inhibitor (SGLT2i), ACE inhibitors (ACEI), or angiotensin receptor blockers (ARB) are recommended to control albuminuria and slow the progression of chronic kidney disease (CKD). Standard CKD management guidelines should be followed for the use of statins and the prevention of CKD-related mineral bone disorders (CKD-MBD)[73]. For patients progressing to renal failure, dialysis or kidney transplantation is required. Furthermore, living-related donors must be tested to ensure they are negative for FD.
Cardiac complications are typically managed by ACEI or ARB, while beta-blockers should be used cautiously. Amiodarone should be avoided, especially in the case of ERT, because of its possible suppressive impact on α-galactosidase (α-GAL) enzyme activity. For symptomatic bradycardia or significant AV conduction abnormalities, permanent pacemaker insertion may be indicated[74]. Patients with atrial fibrillation should receive lifelong anticoagulation, and in cases of malignant arrhythmias, implantable cardioverter-defibrillators (ICD) should be considered[75].
Cerebrovascular complications require stroke prophylaxis with antithrombotic agents (e.g., aspirin or clopidogrel) for secondary prevention. In patients with atrial fibrillation, anticoagulation with warfarin or newer agents is recommended[76].
Neuropathic pain is managed using anticonvulsants such as gabapentin or pregabalin as first-line agents, with opioid agonists considered for severe pain crises. Lifestyle modifications, including avoiding temperature extremes and maintaining proper hydration, are important to prevent pain exacerbations[77].
Gastrointestinal symptoms, such as delayed gastric emptying, can be treated with metoclopramide, while dyspepsia is managed with H2 blockers. Dietary adjustments, including increased fiber intake and smaller, more frequent meals, can help manage dysmotility and diarrhea[78,79].
For pulmonary involvement, bronchodilators are used to relieve airway obstruction[80].
Hearing loss is addressed with hearing aids or cochlear implants[81].
Dermatological issues, including angiokeratomas, have a limited response to laser or cosmetic treatments[82].
This multifaceted strategy is designed to address the diverse pathological manifestations of the disease, ultimately improving overall patient health and quality of life.
Considering the multi-organ involvement and the need for supportive therapy for each organ and system, the multidisciplinary management of the patient by a team of disease experts is essential[6,72,83].
CONCLUSIONS
Despite advancements in research and therapeutic approaches, currently approved treatments for Fabry disease remain limited to agalsidase α, agalsidase ß, pegunigalsidase α, and migalastat. Access to ERT and chaperone therapy varies significantly across different countries, depending on regional healthcare systems and resources.
Early diagnosis of both classic and non-classic forms of Fabry disease is crucial but remains challenging due to their rarity within an already rare condition. This underscores the need for collaboration among diverse healthcare professionals to ensure precise and timely care. Furthermore, it is essential to recognize not only the physical changes resulting from the genetic defect but also its profound impact on quality of life. Today, there is a growing emphasis on the comprehensive evaluation of individuals with rare diseases, driving scientific research toward personalized therapies tailored to meet the unique needs of each patient. These therapies aim not only to address the symptoms but also to improve their quality of life.
Certainly, the collaboration between established and emerging therapeutic options holds promise for favorable outcomes for the treatment of Fabry disease.
DECLARATIONS
Acknowledgments
This work was generated within the European Reference Network for Rare Kidney Diseases (ERKnet).
Authors’ contributions
Design of the study, literature search, writing, editing: Giliberti M
Literature search, writing: Robles S, Campilongo G, Serena Russo M, Di Leo V
Critical review of the manuscript: Gesualdo L
Availability of data and materials
Not applicable.
Financial support and sponsorship
None.
Conflicts of interest
Gesualdo L received grant support from Abionyx and Sanofi to his university department (DIMEPRE-1); he has been a member of advisory boards for AstraZeneca, Baxter, Chinook, GSK, Mundipharma, Novartis, Pharmadoc, Roche, Sanofi, Travere, and Vifor Pharma, and invited speaker at meetings supported by AstraZeneca, Astellas, Estor, Takeda, Amicus, Fresenius, Werfen, Medtronic, Travere, and GSK. Giliberti M has been a member of advisory boards for BioCryst and Kyowa Kirin and was invited speaker at meetings supported by Sanofi, Takeda, Amicus, Kyowa Kirin, Alnylam, Chiesi, and AstraZeneca. The other authors have declared that they have no conflicts of interest.
Ethical approval and consent to participate
Not applicable.
Consent for publication
Not applicable.
Copyright
© The Author(s) 2024.
REFERENCES
1. Askari H, Kaneski CR, Semino-Mora C, et al. Cellular and tissue localization of globotriaosylceramide in Fabry disease. Virchows Arch 2007;451:823-34.
2. Amodio F, Caiazza M, Monda E, et al. An overview of molecular mechanisms in Fabry disease. Biomolecules 2022;12:1460.
3. Desnick RJ. Chapter 38 - Fabry disease: α-galactosidase a deficiency; In: Rosenberg’s molecular and genetic basis of neurological and psychiatric disease, 5th edition; 2015. pp. 419-30.
4. Schiffmann R, Hughes DA, Linthorst GE, et al. Screening, diagnosis, and management of patients with Fabry disease: conclusions from a “Kidney disease: improving global outcomes” (KDIGO) controversies conference. Kidney Int 2017;91:284-93.
5. Germain DP, Hughes DA, Nicholls K, et al. Treatment of Fabry’s disease with the pharmacologic chaperone migalastat. N Engl J Med 2016;375:545-55.
6. Germain DP, Altarescu G, Barriales-Villa R, et al. An expert consensus on practical clinical recommendations and guidance for patients with classic Fabry disease. Mol Genet Metab 2022;137:49-61.
7. Arends M, Wanner C, Hughes D, et al. Characterization of classical and nonclassical Fabry disease: a multicenter study. J Am Soc Nephrol 2017;28:1631-41.
8. Ramaswami U, Beck M, Hughes D, et al. Cardio- renal outcomes with long- term agalsidase alfa enzyme replacement therapy: a 10- year Fabry outcome survey (FOS) analysis. Drug Des Devel Ther 2019;13:3705-15.
9. Bichet DG, Torra R, Wallace E, et al. Long-term follow-up of renal function in patients treated with migalastat for Fabry disease. Mol Genet Metab Rep 2021;28:100786.
10. Johnson FK, Mudd PN Jr, Bragat A, Adera M, Boudes P. Pharmacokinetics and safety of migalastat HCl and effects on agalsidase activity in healthy volunteers. Clin Pharmacol Drug Dev 2013;2:120-32.
11. Lerario S, Monti L, Ambrosetti I, et al. Fabry disease: a rare disorder calling for personalized medicine. Int Urol Nephrol 2024;56:3161-72.
12. Schiffmann R, Goker-Alpan O, Holida M, et al. Pegunigalsidase alfa, a novel PEGylated enzyme replacement therapy for Fabry disease, provides sustained plasma concentrations and favorable pharmacodynamics: a 1-year Phase 1/2 clinical trial. J Inherit Metab Dis 2019;42:534-44.
13. Cox TM. Innovative treatments for lysosomal diseases. Best Pract Res Clin Endocrinol Metab 2015;29:275-311.
15. Medin JA, Tudor M, Simovitch R, et al. Correction in trans for Fabry disease: expression, secretion and uptake of alpha-galactosidase A in patient-derived cells driven by a high-titer recombinant retroviral vector. Proc Natl Acad Sci USA 1996;93:7917-22.
16. Takenaka T, Qin G, Brady RO, Medin JA. Circulating alpha-galactosidase A derived from transduced bone marrow cells: relevance for corrective gene transfer for Fabry disease. Hum Gene Ther 1999;10:1931-9.
17. Zhu X, Yin L, Theisen M, et al. Systemic mRNA therapy for the treatment of Fabry disease: preclinical studies in wild-type mice, Fabry mouse model, and wild-type non-human primates. Am J Hum Genet 2019;104:625-37.
18. Puri V, Watanabe R, Dominguez M, et al. Cholesterol modulates membrane traffic along the endocytic pathway in sphingolipid-storage diseases. Nat Cell Biol 1999;1:386-8.
19. Schueler U, Kaneski C, Remaley A, et al. A Short Synthetic Peptide Mimetic of Apolipoprotein A1 Mediates Cholesterol and Globotriaosylceramide Efflux from Fabry Fibroblasts. In: Morava E, Baumgartner M, Patterson M, Rahman S, Zschocke J, Peters V, editors. Berlin: Springer Berlin Heidelberg; 2016. pp. 69-75.
20. Biegstraaten M, Arngrímsson R, Barbey F, et al. Recommendations for initiation and cessation of enzyme replacement therapy in patients with Fabry disease: the European Fabry Working Group consensus document. Orphanet J Rare Dis 2015;10:36.
21. Christensen EI, Zhou Q, Sørensen SS, et al. Distribution of α-galactosidase A in normal human kidney and renal accumulation and distribution of recombinant α-galactosidase A in Fabry mice. J Am Soc Nephrol 2007;18:698-706.
22. Prabakaran T, Nielsen R, Larsen JV, et al. Receptor-mediated endocytosis of α-galactosidase A in human podocytes in Fabry disease. PLoS One 2011;6:e25065.
23. Prabakaran T, Nielsen R, Satchell SC, et al. Mannose 6-phosphate receptor and sortilin mediated endocytosis of α-galactosidase A in kidney endothelial cells. PLoS One 2012;7:e39975.
24. Frustaci A, Verardo R, Scialla R, et al. Downregulation of mannose-6-phosphate receptors in Fabry disease cardiomyopathy: a potential target for enzyme therapy enhancement. J Clin Med 2022;11:5440.
25. Tøndel C, Bostad L, Larsen KK, et al. Agalsidase benefits renal histology in young patients with Fabry disease. J Am Soc Nephrol 2013;24:137-48.
26. Banikazemi M, Bultas J, Waldek S, et al. Agalsidase-beta therapy for advanced Fabry disease: a randomized trial. Ann Intern Med 2007;146:77-86.
27. Weidemann F, Breunig F, Beer M, et al. Improvement of cardiac function during enzyme replacement therapy in patients with Fabry disease: a prospective strain rate imaging study. Circulation 2003;108:1299-301.
28. van Breemen MJ, Rombach SM, Dekker N, et al. Reduction of elevated plasma globotriaosylsphingosine in patients with classic Fabry disease following enzyme replacement therapy. Biochim Biophys Acta 2011;1812:70-6.
29. Arends M, Wijburg FA, Wanner C, et al. Favourable effect of early versus late start of enzyme replacement therapy on plasma globotriaosylsphingosine levels in men with classical Fabry disease. Mol Genet Metab 2017;121:157-61.
30. Auray-Blais C, Lavoie P, Boutin M, et al. Biomarkers associated with clinical manifestations in Fabry disease patients with a late-onset cardiac variant mutation. Clin Chim Acta 2017;466:185-93.
31. Nowak A, Beuschlein F, Sivasubramaniam V, Kasper D, Warnock DG. Lyso-Gb3 associates with adverse long-term outcome in patients with Fabry disease. J Med Genet 2022;59:287-93.
32. Messalli G, Imbriaco M, Avitabile G, et al. Role of cardiac MRI in evaluating patients with Anderson-Fabry disease: assessing cardiac effects of long-term enzyme replacement therapy. Radiol Med 2012;117:19-28.
33. Tøndel C, Thurberg BL, DasMahapatra P, et al. Clinical relevance of globotriaosylceramide accumulation in Fabry disease and the effect of agalsidase beta in affected tissues. Mol Genet Metab 2022;137:328-41.
34. Germain DP, Waldek S, Banikazemi M, et al. Sustained, long-term renal stabilization after 54 months of agalsidase beta therapy in patients with Fabry disease. J Am Soc Nephrol 2007;18:1547-57.
35. Sirrs SM, Bichet DG, Casey R, et al. Outcomes of patients treated through the Canadian Fabry disease initiative. Mol Genet Metab 2014;111:499-506.
36. Arends M, Biegstraaten M, Wanner C, et al. Agalsidase alfa versus agalsidase beta for the treatment of Fabry disease: an international cohort study. J Med Genet 2018;55:351-8.
37. Mauhin W, Lidove O, Amelin D, et al. Deep characterization of the anti-drug antibodies developed in Fabry disease patients, a prospective analysis from the French multicenter cohort FFABRY. Orphanet J Rare Dis 2018;13:127.
38. Beck M, Hughes D, Kampmann C, et al. Long-term effectiveness of agalsidase alfa enzyme replacement in Fabry disease: a Fabry outcome survey analysis. Mol Genet Metab Rep 2015;3:21-7.
39. Kampmann C, Perrin A, Beck M. Effectiveness of agalsidase alfa enzyme replacement in Fabry disease: cardiac outcomes after 10 years’ treatment. Orphanet J Rare Dis 2015;10:125.
40. Tsuboi K, Yamamoto H. Clinical observation of patients with Fabry disease after switching from agalsidase beta (Fabrazyme) to agalsidase alfa (Replagal). Genet Med 2012;14:779-86.
41. Hughes DA, Elliott PM, Shah J, et al. Effects of enzyme replacement therapy on the cardiomyopathy of Anderson-Fabry disease: a randomised, double-blind, placebo-controlled clinical trial of agalsidase alfa. Heart 2008;94:153-8.
42. Azevedo O, Gago MF, Miltenberger-Miltenyi G, Sousa N, Cunha D. Fabry disease therapy: state-of-the-art and current challenges. Int J Mol Sci 2020;22:206.
43. Kizhner T, Azulay Y, Hainrichson M, et al. Characterization of a chemically modified plant cell culture expressed human α-Galactosidase-A enzyme for treatment of Fabry disease. Mol Genet Metab 2015;114:259-67.
44. Wallace EL, Goker-Alpan O, Wilcox WR, et al. Head-to-head trial of pegunigalsidase alfa versus agalsidase beta in patients with Fabry disease and deteriorating renal function: results from the 2-year randomised phase III BALANCE study. J Med Genet 2024;61:520-30.
45. Linhart A, Dostálová G, Nicholls K, et al. Safety and efficacy of pegunigalsidase alfa in patients with Fabry disease who were previously treated with agalsidase alfa: results from BRIDGE, a phase 3 open-label study. Orphanet J Rare Dis 2023;18:332.
46. Bernat J, Holida M, Longo N, et al. eP149: safety and efficacy of pegunigalsidase alfa, every 4 weeks, in Fabry disease: results from the phase 3, open-label, BRIGHT study. Genet Med 2022;24:S91-2.
47. Germain DP, Linhart A. Pegunigalsidase alfa: a novel, pegylated recombinant alpha-galactosidase enzyme for the treatment of Fabry disease. Front Genet 2024;15:1395287.
48. Nowak A, Huynh-Do U, Krayenbuehl PA, Beuschlein F, Schiffmann R, Barbey F. Fabry disease genotype, phenotype, and migalastat amenability: insights from a national cohort. J Inherit Metab Dis 2020;43:326-33.
49. Fan JQ, Ishii S, Asano N, Suzuki Y. Accelerated transport and maturation of lysosomal alpha-galactosidase A in Fabry lymphoblasts by an enzyme inhibitor. Nat Med 1999;5:112-5.
51. Mauer M, Sokolovskiy A, Barth JA, et al. Reduction of podocyte globotriaosylceramide content in adult male patients with Fabry disease with amenable GLA mutations following 6 months of migalastat treatment. J Med Genet 2017;54:781-6.
52. Hughes DA, Nicholls K, Shankar SP, et al. Oral pharmacological chaperone migalastat compared with enzyme replacement therapy in Fabry disease: 18-month results from the randomised phase III ATTRACT study. J Med Genet 2017;54:288-96.
53. Hughes DA, Bichet DG, Giugliani R, et al. Long-term multisystemic efficacy of migalastat on Fabry-associated clinical events, including renal, cardiac and cerebrovascular outcomes. J Med Genet 2023;60:722-31.
54. Young-Gqamana B, Brignol N, Chang HH, et al. Migalastat HCl reduces globotriaosylsphingosine (lyso-Gb3) in Fabry transgenic mice and in the plasma of Fabry patients. PLoS One 2013;8:e57631.
55. Nowicki M, Bazan-Socha S, Błażejewska-Hyżorek B, et al. Polish Fabry Disease Collaborative Group. A review and recommendations for oral chaperone therapy in adult patients with Fabry disease. Orphanet J Rare Dis 2024;19:16.
56. Shen JS, Busch A, Day TS, et al. Mannose receptor-mediated delivery of moss-made α-galactosidase A efficiently corrects enzyme deficiency in Fabry mice. J Inherit Metab Dis 2016;39:293-303.
57. Hennermann JB, Arash-Kaps L, Fekete G, Schaaf A, Busch A, Frischmuth T. Pharmacokinetics, pharmacodynamics, and safety of moss-aGalactosidase A in patients with Fabry disease. J Inherit Metab Dis 2019;42:527-33.
58. Palaiodimou L, Kokotis P, Zompola C, et al. Fabry disease: current and novel therapeutic strategies. A narrative review. Curr Neuropharmacol 2023;21:440-56.
59. Ashe KM, Budman E, Bangari DS, et al. Efficacy of enzyme and substrate reduction therapy with a novel antagonist of glucosylceramide synthase for Fabry disease. Mol Med 2015;21:389-99.
60. Peterschmitt MJ, Crawford NPS, Gaemers SJM, Ji AJ, Sharma J, Pham TT. Pharmacokinetics, pharmacodynamics, safety, and tolerability of oral venglustat in healthy volunteers. Clin Pharmacol Drug Dev 2021;10:86-98.
61. Deegan PB, Goker-Alpan O, Geberhiwot T, et al. Venglustat, an orally administered glucosylceramide synthase inhibitor: assessment over 3 years in adult males with classic Fabry disease in an open-label phase 2 study and its extension study. Mol Genet Metab 2023;138:106963.
62. A study to evaluate the effect of venglustat tablets on left ventricular mass index in male and female adult participants with fabry disease (CARAT); 2024. NCT05280548. Available from: https://www.clinicaltrials.gov/study/NCT05280548#eligibility [Last accessed on 19 Nov 2024]
63. A study to evaluate the effect of venglustat tablets on neuropathic and abdominal pain in male and female participants ≥ 16 years of age with fabry disease (PERIDOT); 2024, NCT05206773. Available from: https://www.clinicaltrials.gov/study/NCT05206773?term=NCT05206773&rank=1 [Last accessed on 19 Nov 2024]
64. Guérard N, Morand O, Dingemanse J. Lucerastat, an iminosugar with potential as substrate reduction therapy for glycolipid storage disorders: safety, tolerability, and pharmacokinetics in healthy subjects. Orphanet J Rare Dis 2017;12:9.
65. Guérard N, Oder D, Nordbeck P, et al. Lucerastat, an iminosugar for substrate reduction therapy: tolerability, pharmacodynamics, and pharmacokinetics in patients with Fabry disease on enzyme replacement. Clin Pharmacol Ther 2018;103:703-11.
66. A multicenter, double-blind, randomized, placebo-controlled, parallel-group study to determine the efficacy and safety of lucerastat oral monotherapy in adult subjects with Fabry disease; 2018, NCT03425539. Available from: https://clinicaltrials.gov/study/NCT03425539?term=%20NCT03425539&rank=1 [Last accessed on 19 Nov 2024]
67. Khan A, Barber DL, Huang J, et al. Lentivirus-mediated gene therapy for Fabry disease. Nat Commun 2021;12:1178.
68. Ziegler RJ, Yew NS, Li C, et al. Correction of enzymatic and lysosomal storage defects in Fabry mice by adenovirus-mediated gene transfer. Hum Gene Ther 1999;10:1667-82.
69. An open-label, phase 1/2a trial of gene therapy 4D-310 in adults with Fabry disease and cardiac involvement; 2022, NCT05629559. Available from: https://clinicaltrials.gov/study/NCT05629559?term=NCT05629559&rank=1 [Last accessed on 19 Nov 2024]
70. A phase I/II, multicenter, open-label, single-dose, dose-ranging study to assess the safety and tolerability of ST-920, an AAV2/6 human alpha galactosidase a gene therapy, in subjects with Fabry disease (STAAR); 2019, NCT04046224. Available from: https://clinicaltrials.gov/study/NCT04046224?term=NCT04046224&rank=1 [Last accessed on 19 Nov 2024]
71. A phase 1/2, baseline-controlled, non-randomized, open-label, single-ascending dose study of a novel adeno-associated viral vector (FLT190) in patients with Fabry disease; 2019, NCT04040049. Available from: https://clinicaltrials.gov/study/NCT04040049?term=NCT04040049&rank=1 [Last accessed on 19 Nov 2024]
72. Ortiz A, Germain DP, Desnick RJ, et al. Fabry disease revisited: management and treatment recommendations for adult patients. Mol Genet Metab 2018;123:416-27.
73. Kidney Disease: Improving Global Outcomes (KDIGO) CKD Work Group. KDIGO 2024 clinical practice guideline for the evaluation and management of chronic kidney disease. Kidney Int 2024;105:S117-314.
74. O’Mahony C, Coats C, Cardona M, et al. Incidence and predictors of anti-bradycardia pacing in patients with Anderson-Fabry disease. Europace 2011;13:1781-8.
75. Weidemann F, Maier SK, Störk S, et al. Usefulness of an implantable loop recorder to detect clinically relevant arrhythmias in patients with advanced Fabry cardiomyopathy. Am J Cardiol 2016;118:264-74.
76. Eng CM, Germain DP, Banikazemi M, et al. Fabry disease: guidelines for the evaluation and management of multi-organ system involvement. Genet Med 2006;8:539-48.
77. Politei JM, Bouhassira D, Germain DP, et al. Pain in Fabry disease: practical recommendations for diagnosis and treatment. CNS Neurosci Ther 2016;22:568-76.
78. Mujagic Z, Keszthelyi D, Aziz Q, et al. Systematic review: instruments to assess abdominal pain in irritable bowel syndrome. Aliment Pharmacol Ther 2015;42:1064-81.
79. Hoffmann B, Schwarz M, Mehta A, Keshav S. Fabry Outcome Survey European Investigators. Gastrointestinal symptoms in 342 patients with Fabry disease: prevalence and response to enzyme replacement therapy. Clin Gastroenterol Hepatol 2007;5:1447-53.
80. Svensson CK, Feldt-Rasmussen U, Backer V. Fabry disease, respiratory symptoms, and airway limitation - a systematic review. Eur Clin Respir J 2015;2:26721.
81. Germain DP, Avan P, Chassaing A, Bonfils P. Patients affected with Fabry disease have an increased incidence of progressive hearing loss and sudden deafness: an investigation of twenty-two hemizygous male patients. BMC Med Genet 2002;3:10.
82. Lidove O, Jaussaud R, Aractingi S. Dermatological and soft-tissue manifestations of Fabry disease: characteristics and response to enzyme replacement therapy. In: Mehta A, Beck M, Sunder-Plassmann G, editors. Fabry disease: perspectives from 5 years of FOS. Oxford: Oxford PharmaGenesis; 2006.
Cite This Article

How to Cite
Download Citation
Export Citation File:
Type of Import
Tips on Downloading Citation
Citation Manager File Format
Type of Import
Direct Import: When the Direct Import option is selected (the default state), a dialogue box will give you the option to Save or Open the downloaded citation data. Choosing Open will either launch your citation manager or give you a choice of applications with which to use the metadata. The Save option saves the file locally for later use.
Indirect Import: When the Indirect Import option is selected, the metadata is displayed and may be copied and pasted as needed.
About This Article
Special Issue
Copyright
Data & Comments
Data
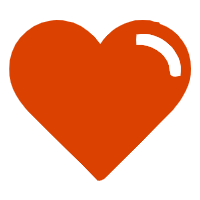
Comments
Comments must be written in English. Spam, offensive content, impersonation, and private information will not be permitted. If any comment is reported and identified as inappropriate content by OAE staff, the comment will be removed without notice. If you have any queries or need any help, please contact us at [email protected].