Neurogenic dysphagia: current pharmacogenomic perspectives
Abstract
Neurogenic dysphagia (ND) is characterized by a swallowing disorder where nervous system, muscle, and neuromuscular diseases are involved. DRD1, COMT, BDNF, and APOE are genes that may have a predictive role in the occurrence and evolution of ND. Many drugs that improve swallowing or can induce or exacerbate swallowing difficulties are related to dopamine metabolism and substance P. These pharmacological treatments for ND include dopamine precursors (levodopa), dopamine agonists (amantadine, apomorphine, cabergoline, and rotigotine), and TRP channel activators (capsaicin, piperine, and menthol). Since treatment outcomes are highly dependent on the genomic profiles of ND patients, personalized treatments should rely on pharmacogenetic procedures to optimize therapeutic interventions. Knowledge of the pharmacogenetic profiles of these drugs would minimize the occurrence of adverse drug reactions (especially to antidopaminergic medications) that may induce dysphagia and optimize pharmacological treatment that can ameliorate it. This knowledge should also be applied to the use of medications that control symptoms associated with dysphagia, such as sialorrhea, xerostomia, reflux, or hiccups.
Keywords
INTRODUCTION
Neurogenic dysphagia (ND) refers to any swallowing disorder associated with central and peripheral nervous system conditions, as well as muscle and neuromuscular diseases. ND is linked to multiple degenerative and nondegenerative congenital, traumatic, vascular, neoplastic, and iatrogenic disorders as diverse as cerebral palsy, traumatic brain injury (TBI), amyotrophic lateral sclerosis (ALS), multiple sclerosis (MS), Parkinson’s syndromes, myasthenia gravis (MG), and myositis[1]. Based on clinical observations, ND can be classified into the following seven distinct phenotypes, which are particularly useful when etiological diagnosis is in doubt: (i) premature bolus spillage; (ii) delayed swallowing reflex, both characteristic of stroke; (iii) predominance of residue valleculae, common in patients with Parkinson’s disease; (iv) predominance of residue in the piriform sinus, characteristic of myositis, motor neuron disease, or brainstem stroke; (v) pharyngolaryngeal movement disorder, observed in patients with parkinsonism and stroke; (vi) fatigable swallowing weakness in individuals with myasthenia gravis; and (vii) complex disorder, as occurs in ALS[2].
The importance of dysphagia stems mostly from the increased risk of death caused by aspiration pneumonia, and conditions related to dehydration or malnutrition[3,4]. In addition to these factors, aging reduces the frequency of spontaneous swallowing[5]. To ensure proper diagnosis and management of ND, it is mandatory to: (i) obtain a complete medical history; (ii) perform screenings that assess the risk of aspiration (e.g., a swallowing test with water and other consistencies); (iii) conduct counseling tests and clinically evaluate dysphagia by videofluoroscopy (VFSS), swallowing endoscopy (FEES), or manometry, and other additional tests such as ultrasonography or electromyography); (iv) perform treatments based on dietary therapeutic interventions, behavioral interventions, oral hygiene measures, neurostimulation, pharmacotherapy, and surgical treatments[6]. In this third step, the management of special groups such as tracheostomized patients and patients with nasogastric tubes is of particular interest[6].
The treatment of ND is mainly based on rehabilitation therapies performed by speech therapists and other non-pharmacological approaches. However, some medications may be effective in improving impairment during the different phases of swallowing[6,7]. The majority of medications used to treat oropharyngeal dysphagia have a general effect on swallowing function that is independent of the underlying neurological disease; this allows for standardized use[8]. Pharmacotherapy, however, produces limited results and should therefore not be used as a stand-alone treatment, but rather as an adjunct to other therapies[8]. Furthermore, medications such as antidopaminergic agents, anticholinergic drugs, or benzodiazepines induce or exacerbate dysphagia[9-12].
In view of these considerations, research into specific ND-related genes may be useful in the prognosis of this condition. Because pharmacogenetics also plays a key role in both the diagnosis and the correct pharmacological management of patients with dysphagia, to increase the benefit of compounds that can improve swallowing difficulty and minimize the risk with the use of dysphagia-inducing drugs, in this review, we highlight these ND mechanisms from a pharmacogenomic perspective.
DOPAMINE AS A NEUROTRANSMITTER
Dopamine is a neurotransmitter of high relevance in the swallowing process. Its precursor, L-DOPA, is synthesized from the essential amino acid tyrosine or indirectly through phenylalanine, a non-essential amino acid. Dopamine β-hydroxylase (DBH) catalyzes the conversion of dopamine to norepinephrine (NE), and NE is then converted into epinephrine by phenylethanolamine N-methyltransferase with
Dopamine is synthesized and acts primarily in the central nervous system (CNS). Dopaminergic neurons project to different brain regions along the mesolimbic, mesocortical, nigrostriatal, and tuberoinfundibular pathways. Dopamine exerts its effects by binding to five G-protein-coupled receptors (D1-D5); of these, D1 receptors are the most abundant in the CNS. These receptors are divided into
DOPAMINE AND SWALLOWING
The swallowing process requires, at least in part, dopamine activity and its binding to its receptors[16]. Although most dopamine receptors would theoretically be relevant to ND, the role of the dopamine
The use of dopaminergic agonists in the treatment of neurogenic dysphagia
Levodopa, rotigotine, cabergoline, apomorphine, and amantadine are dopamine agonists that have been used generically to treat a variety of neurological conditions associated with oropharyngeal dysphagia[8]. The drug that provides the best outcome is controversial because of conflicting outcomes across different studies. However, among these, levodopa is the most widely used, and it is also used to evaluate the swallowing response during the Fiberoptic Endoscopic Evaluation of Swallowing (FEES) test[21,22]. Most studies have focused on the effect of dopaminergic agonists in Parkinson’s disease, and several publications show that these drugs improve dysphagia, especially in the oral phase and, to a lesser extent, in the pharyngeal phase[23-25]. This clinical improvement is related to swallowing alterations due to nigrostriatal dopamine deficits and to other structures such as the pedunculopontine nucleus or the medulla[23]. In a small group of patients, an improvement in bolus fragmentation, vallecular stasis, and laryngeal penetration was observed, together with a shortening of the swallowing phase; these findings are associated with an improvement in bucco-linguo-facial motility[26]. Paradoxically, and despite most articles reporting a beneficial effect, one clinical trial showed that levodopa could worsen dysphagia by inhibiting brainstem reflexes[27]. Overall, however, the results appear to support its use in PD patients despite the lack of high-quality evidence[28]. Although dopaminergic agonists have a modest effect on the motor symptoms of progressive supranuclear palsy, they help some patients improve their swallowing[21]. However, these drugs can also be employed in acquired neurological conditions. Following a lacunar stroke involving the basal ganglia, for example, levodopa decreases the risk of aspiration by shortening the latency of the swallowing reflex, as shown after examining the submental electromyographic activity and the visual observation of the laryngeal movement[29]. This reduction, according to monocentric randomized trials in which imaging and physical signs were evaluated, is also observed with other dopamine agonists such as cabergoline and amantadine; the elderly population, in particular, may benefit from treatment with dopamine agonists[30,31].
The search for new compounds to treat ND also includes natural supplements that contain dopamine, for use mainly in groups where dosage or side effects may be contraindicated, such as children or the elderly. Natural sources of dopamine include Mucuna pruriens, Vicia faba, or Musa cavendishii[32-34]. In fact, several studies in patients with Parkinson’s disease reveal the effectiveness of these treatments with extracts derived from these products; these compounds reduce the risk of adverse effects such as dyskinesias as well as induce epigenetic and pharmacoepigenetic modifications[35,36].
Pharmacogenetics of dopaminergic agonists in the treatment of neurogenic dysphagia
Anti-ND drugs exhibit different specific pharmacogenetic profiles [Table 1][37]. All of the medications used to treat ND show, among others, DRD1 as a mechanistic gene and the binding of drugs to this receptor. All of the anti-ND drugs have COMT as substrates, where COMT shortens the activity of these dopaminergic drugs[38]. Moreover, the COMT rs4680 polymorphism may induce motor complications such as dyskinesia during treatment with levodopa[38-40]. Levodopa also has DBH as substrate[37]. ADORA2A SNPs and HOMER1 variants are associated with L-DOPA-induced adverse motor (e.g., dyskinesia) and psychotic symptoms[41,42]. A haplotype integrating -141CIns/Del, rs2283265, rs1076560, C957T, TaqIA, and rs2734849 polymorphisms at the DRD2/ANKK1 gene region is linked to L-DOPA-induced motor dysfunction[43]. SLC6A3 is a genetic modifier of the treatment response to L-DOPA[44]. The multi-drug resistance gene
Pharmacogenetics of dopaminergic agonists in the treatment of neurogenic dysphagia
Drug | Properties | Pharmacogenetics |
![]() | Name: Levodopa IUPAC Name: l-Tyrosine-3-hydroxy Molecular Formula: C9H11NO4 Molecular Weight: 197.19 g/mol Mechanism: Levodopa circulates in the plasma to the blood–brain barrier, where it crosses and is then converted by striatal enzymes to dopamine. Carbidopa inhibits the peripheral plasma breakdown of levodopa by inhibiting its carboxylation, and thereby increases available levodopa at the blood–brain barrier Effect: Antiparkinsonian agents, dopamine precursors | Pathogenic genes: ANKK1, BDNF, LRRK2, PARK2 Mechanistic genes: CCK, CCKAR, CCKBR, DRD1, DRD2, DRD3, DRD4, DRD5, GRIN2A, GRIN2B, HCRT, HOMER1, LMO3, OPRM1 Metabolic genes: Substrate: COMT, CYP1A2, CYP2B6, CYP2C19, CYP2D6, CYP3A4, CYP3A5, DBH, DDC, G6PD, MAOB, TH, UGT1A1, UGT1A9 Transporter genes: SLC22A1, SLC6A3, SLC15A1 (inhibitor). SLC16A10 (inhibitor), SLC7A5, SLC7A8 Pleiotropic genes: ACE, ACHE |
![]() | Name: Cabergoline IUPAC Name: Ergoline-8β-carboxamide, N-[3-(dimethylamino)propyl]-N-[(ethylamino)carbonil]-6-(2-propenyl) Molecular Formula: C26H37N5O2 Molecular Weight: 451.60 g/mol Mechanism: A long-acting dopamine receptor agonist. Has high binding affinity for dopamine D2-receptors and lesser affinity for D1, α1- and α2-adrenergic, and serotonin (5-HT1 and 5-HT2) receptors. Reduces serum prolactin concentrations by inhibiting release of prolactin from the anterior pituitary gland (agonist activity at D2 receptors) Effect: Antiparkinsonian agents, ergot-derivative dopamine receptor agonists | Pathogenic genes: BDNF, GSK3B Mechanistic genes: ADRA1A, ADRA1B,ADRA1D, ADRA2A, ADRA2B, ADRA2C, ADRB1, ADRB2, AKT1, BDNF, CNR1, DRD1, DRD2, DRD3, DRD4, DRD5, GSK3B, HTR1A, HTR1B, HTR1D, HTR2A, HTR2B, HTR2C, HTR7 Metabolic genes: Substrate: COMT, CYP1A2, CYP2B6, CYP2C19, CYP2D6, CYP3A4 (minor), CYP3A5, DDC Transporter genes: ABCB1 |
![]() | Name: Rotigotine Molecular Formula: C19H25NOs Molecular Weight: 315.47 g/mol Mechanism: A non-ergot dopamine receptor agonist with specificity for D3-, D2-, and D1-dopamine receptors. Although the precise mechanism of action of Rotigotine is unknown, it is believed to be due to stimulation of postsynaptic dopamine D2-type autoreceptors within substantia nigra in brain, leading to improved dopaminergic transmission in motor areas in basal ganglia, notably caudate nucleus/putamen regions Effect: Antiparkinsonian agents, non-ergot-derivative dopamine receptor agonists | Pathogenic genes: ANKK1, BDNF, LRRK2 Mechanistic genes: CCK, CCKAR, CCKBR, DRD1, DRD2, DRD3, DRD4, DRD5, GRIN2A, GRIN2B, HCRT, HOMER1, LMO3, OPRM1, HTR1A, ADRA2B Metabolic genes: Substrate: COMT, MAOB, CYP3A4, CYP2D6 Inhibitor: CYP2D6, CYP2C19 Transporter genes: SLC22A1, SLC6A3 Pleiotropic genes: ACE, APOE |
![]() | Name: Apomorphine Molecular Formula: C17H17NO2HCl1/2H2O Molecular Weight: 312.79 g/mol Mechanism: Stimulates postsynaptic D2-type receptors within the caudate-putamen in the brain Effect: Antiparkinsonian agents, non-ergot-derivative dopamine receptor agonists | Pathogenic genes: PARK2 Mechanistic genes: ADRA2A, ADRA2B, ADRA2C, CALY, DRD1, DRD2, DRD3, DRD4, DRD5, HTR1A, HTR1B, HTR1D, HTR2A, HTR2B, HTR2C Metabolic genes: Substrate: COMT, CYP1A2 (minor), CYP2B6, CYP2C9 (minor), CYP2C19 (minor), CYP2D6, CYP3A4 (minor), CYP3A5, DDC, UGT1A1, UGT1A9, SULT1A1, SULT1A2, SULT1A3, SULT1E1, SULT1B1 Inhibitor: CYP1A2 (weak), CYP2C19 (weak), CYP3A4 (weak) Transporter genes: SLC18A2 |
![]() | Name: Amantadine IUPAC Name: Tricyclo[3.3.1.13,7]decan-1-amine, hydrochloride Molecular Formula: C10H17NHCl Molecular Weight: 187.71 g/mol Mechanism: Antiparkinsonian activity may be due to inhibition of dopamine reuptake into presynaptic neurons or by increasing dopamine release from presynaptic fibers Effect: Antiparkinsonian agents, adamantanes, dopamine agonists | Pathogenic genes: PARK2 Mechanistic genes: CCR5, CXCR4, DRD1, DRD2, GRIN3A, CHRNA3, CHRNA4, CHRNA7 Metabolic genes: Substrate: COMT, CYP1A2, CYP2B6, CYP2C19, CYP2D6, CYP3A4, CYP3A5, DDC, UGT1A1, UGT1A9 Inhibitor: MAOB Transporter genes: SLC22A1 (Substrate/inhibitor), SLC22A2 (Substrate/inhibitor) |
Antidopaminergics and neurogenic dysphagia
In a significant number of cases, the causes of ND can be induced or exacerbated by certain drugs[9-11]. Many patients with different neurological conditions are treated with antidopaminergic medication[10,11]. Adverse reactions are especially frequent in senescence and are relevant since they are reversible, and dysphagia may be the only or the predominant extrapyramidal symptom. Although it is recommended that drug intake be minimized as much as possible, this is not feasible in many cases. It is therefore recommended that the drug dose be adjusted to avoid the aforementioned side effects. Knowing the pharmacogenetic profiles of these drugs is, therefore, very important to therapeutic strategies[37] [Table 2].
Pharmacogenetics of antidopaminergic drugs and the risk of neurogenic dysphagia
Drug | Properties | Pharmacogenetics |
![]() | Name: Haloperidol IUPAC Name: 4-[4-(4-chlorophenyl)-4-hydroxypiperidin-1-yl]-1-(4-fluorophenyl)butan-1-one Molecular Formula: C21H23ClFNO2 Molecular Weight: 375.864223 g/mol Mechanism: Haloperidol is a butyrophenone antipsychotic which blocks postsynaptic mesolimbic dopaminergic D1 and D2 receptors in brain. Depresses release of hypothalamic and hypophyseal hormones. Believed to depress reticular activating system Effect: Antipsychotic agent, Serotonergic antagonist, Dopaminergic antagonist, antiemetic, antidyskinesia agent, sedative effects, hypotension | Pathogenic genes: ADRA1A, ADRA2A, ADRA2B, ADRA2C, BDNF, DRD1, DRD2, DRD3, DRD4, DTNBP1, GRIN2B, HTR2A Mechanistic genes: ANKK1, BDNF, COMT, DRD1, DRD2, DRD3, DTNBP1, GRIN2A, GRIN3B, GRIN2C, GRIN2B, SLC6A3, MCHR1, SLC18A2, HTR2C, SIGMAR1, HRH1, CHRM3, HTR1A, HTR6, HTR7 Metabolic genes: Substrate: CBR1, CYP1A1 (minor), CYP1A2 (minor), CYP2A6, CYP2C8 (minor), CYP2C9 (minor), CYP2C19 (minor), CYP2D6 (major), CYP3A4/5 (major), CYP3A7, GSTP1, UGT1A9 Inhibitor: CYP2D6 (moderate), CYP3A4 (moderate) Transporter genes: ABCB1 (substrate/inhibitor), ABCC1, KCNE1, KCNE2, KCNH2, KCNJ11, KCNQ1, SLC6A3 Pleiotropic genes: CHRM2, FOS, GSK3B, HRH1, HTR2A, HTT, IL1RN |
![]() | Name: Sulpiride. IUPAC Name: N-[(1-ethylpyrrolidin-2-yl)methyl]-2-methoxy-5-sulfamoylbenzamide Molecular Formula: C15H23N3O4S Molecular Weight: 341.42582 g/mol Mechanism: It is a selective antagonist at postsynaptic D2 and D3 receptors. It appears to lack effects on norepinephrine, acetylcholine, serotonin, histamine, or GABA receptors. It also stimulates secretion of prolactin. Effect: Antipsychotic agent, dopaminergic antagonist, antidepressant effect, antiemesis, sedation | Pathogenic genes: DRD2, DRD3, DRD4 Mechanistic genes: DRD2, DRD3, DRD4, PRLH, CA2, CA3 Metabolic genes: Substrate: CYP1A2, CYP2B1, CYP3As Inhibitor: BCHE, CYP1A2, CYP2B1, CYP3As Transporter genes: SLC22A1, SLC22A2, SLC47A1, SLC47A2, SLC22A3, ABCB1, ABCG2 |
![]() | Name: Aripiprazole. IUPAC Name: 7-{4-[4-(2,3-dichlorophenyl) piperazin-1-yl]butoxy}-1,2,3,4-tetrahydroquinolin-2-one Molecular Formula: 448.38538 g/mol Molecular Weight: C23H27Cl2N3O2 Mechanism: Partial agonist at the D2 and 5-HT1A receptors, and as an antagonist at the 5-HT2A receptor Effect: Antipsychotic agent, H1-receptor antagonist, serotonergic agonist | Pathogenic genes: DRD1, DRD2, DRD3, DRD4, HTR1A, HTR2A, HTR2C Mechanistic genes: ADRA1A, ADRA1B, ADRA2A, ADRA2B, ADRA2C, CHRM1, CHRM2, CHRM3, CHRM4, CHRM5, CALy, GSTP1,DRD1, DRD2, DRD3, DRD4, HRHs, HTR1A, HTR1B, HTR1D, HTR1E, HTR2A, HTR2B, HTR2C, HTR3A, HTR6, HTR7, CYP1A1 Metabolic genes: Substrate: CYP1A2, CYP2A6, CYP2D6 (major), CYP3A4 (major), CYP2C8, CYP2C9, CYP2C19, CYP3A5, FMO3, UGT1A4 Inhibitor: CYP2D6, CYP3A4, CYP2C19 Transporter genes: ABCB1 |
![]() | Name: Olanzapine. IUPAC Name: 5-methyl-8-(4-methylpiperazin-1-yl)-4-thia-2,9-diazatricyclo[8.4.0.03,7]tetradeca-1(14),3(7),5,8,10,12-hexaene Molecular Formula: C17H20N4S Molecular Weight: 312.4325 g/mol Mechanism: It displays potent antagonism of serotonin 5-HT2A and 5-HT2C, dopamine D1-4, histamine H1 and α1-adrenergic receptors, moderate antagonism of 5-HT3 and muscarinic M1-5 receptors, and weak binding to GABA-A, BZD, and β-adrenergic receptors. Effect: Antipsychotic agent, GABA modulator, muscarinic antagonist, serotonin uptake inhibitor, dopaminergic antagonist, serotonergic antagonist, histamine antagonist, antiemetic activity | Pathogenic genes: COMT, DRD1, DRD2, DRD3, DRD4, GRM3, HTR2A, HTR2C, LPL Mechanistic genes: ABCB1, ADRA1A, ADRA1B, ADRB3, AHR, BDNF, CHRM1, CHRM2, CHRM3, CHRM4, CHRM5, COMT, DRD1, DRD2, DRD3, DRD4, DRD5, GABRs, GRIN2B, HRH1, HTR2A, HTR2C, HTR3A, HTR6, HRH1,LEP, RGS2, RGS7, SLC6A4, STAT3, TMEM163 Metabolic genes: Substrate: COMT, CYP1A2 (major), CYP2C9, CYP2D6 (major), CYP3A4, CYP3A5, FMO1, FMO3, GSTM3, TPMT, UGT1A1, UGT1A4, UGT2B10 Inhibitor: CYP1A2 (weak), CYP2C9 (weak), CYP2C19 (weak), CYP2D6 (weak), CYP3A4 (weak) Inducer: GSTM1, MAOB, SLCO3A1 Transporter genes: ABCB1 (substrate/inhibitor), KCNH2, SLC6A2, SLC6A4, SLCO3A1 Pleiotropic genes: APOA5, APOC3, GNB3, LEP, LEPR, LPL |
![]() | Name: Quetiapine IUPAC Name: 2-[2-(4-{2-thia-9-azatricyclo[9.4.0.03,8]pentadeca-1(15),3,5,7,9,11,13-heptaen-10-yl}piperazin-1-yl)ethoxy]ethan-1-ol Molecular Formula: C46H54N6O8S2 Molecular Weight: 883.08636 g/mol Mechanism: Antagonist at multiple neurotransmitter receptors: serotonin 5-HT1A and 5-HT2, dopamine D1 and D2, histamine H1, and adrenergic α1- and α2-receptors. Effect: Antipsychotic agent, Adrenergic antagonist, histamine antagonist, serotonergic antagonist, dopaminergic antagonist, sedative activity, orthostatic hypotension | Pathogenic genes: ADRA2A, DRD1, DRD2, DRD4, HTR1A, HTR2A, RGS4 Mechanistic genes: ADRA1s, ADRA2A, ADRA2B, ADRA2C, BDNF, CHRM1, CHRM2, CHRM3, CHRM4, CHRM5, DRD1, DRD2, DRD4, HRH1, HTR1A, HTR1B, HTR1D, HTR1E, HTR2A, HTR2B, HTR2C, HTR6, HTR7 Metabolic genes: Substrate: CYP2D6 (minor), CYP3A4/5 (major), CYP3A7,CYP2C19 Transporter genes: ABCB1 (substrate/inhibitor), KCNE1, KCNE2, KCNH2, KCNQ1, SCN5A, SLC6A2 (inhibitor) |
![]() | Name: Risperdone IUPAC Name: 3-{2-[4-(6-fluoro-1,2-benzoxazol-3-yl)piperidin-1-yl]ethyl}-2-methyl-4H,6H,7H,8H,9H-pyrido[1,2-a]pyrimidin-4-one Molecular Formula: C23H27FN4O2 Molecular Weight: 410.484483 g/mol Mechanism: Antagonist at multiple neurotransmitter receptors: serotonin 5-HT1A and 5-HT2, dopamine D1 and D2, histamine H1, and adrenergic α1- and α2-receptors. Effect: Antipsychotic agent, H1-receptor antagonist, dopaminergic antagonist, alpha-adrenergic antagonist, serotonergic antagonist, somnolence, orthostatic hypotension | Pathogenic genes: ADRA2A, BDNF, COMT, DRD1, DRD2, DRD3, DRD4, GRM3, HTR2A, HTR2C, HTR7, PON1, RGS4 Mechanistic genes: ADRA1A, ADRA1B, ADRA2B, ADRA2C, DRD1, DRD2, DRD3, DRD4, FOS, HRH1, HTR1A, HTR2A, HTR2C, HTR3A, HTR3C, HTR6, HTR7, NR1I2, STAT3 Metabolic genes: Substrate: COMT, CYP2D6 (major), CYP3A4/5 (minor) Inhibitor: CYP2D6 (weak), CYP3A4 (weak) Inducer: MAOB Transporter genes: ABCB1 (substrate/inhibitor), KCNH2, SLC6A4 Pleiotropic genes: APOA5, BDNF, RGS2 |
![]() | Name: Chlorpromazine IUPAC Name: [3-(2-chloro-10H-phenothiazin-10-yl)propyl]dimethylamine Molecular Formula: C17H19ClN2S Molecular Weight: 318.86416 g/mol Mechanism: Blocks postsynaptic mesolimbic dopaminergic receptors in the brain. Has actions at all levels of CNS, particularly at subcortical levels; also acts on multiple organ systems. It also exhibits weak ganglionic blocking, has a strong α-drenergic blocking effect, and depresses the release of hypothalamic and hypophyseal hormones. Depresses the reticular activating system Effect: Antipsychotic agent, dopaminergic antagonist, antiemetic, anticholinergic effects, sedative effects, antihistaminic effects, anti-serotonergic activity, hypotension | Pathogenic genes: BDNF, DRD1, DRD2, DRD3, DRD4, HTR2A Mechanistic genes: ADRA1A, ADRA1B, CHRM1, CHRM2, CHRM3, DRD1, DRD2, DRD3, DRD4, DRD5, HRH1, HRH4, HTR1A, HTR2A, HTR2C, HTR6, HTR7, KCNH2, SMPD1, CALM1 Metabolic genes: Substrate: CYP1A2(minor), CYP2A6, CYP2C9, CYP2C19, CYP2D6(major), CYP3A (minor), FMO1, UGT1A3, UGT1A4 Inhibitor:CYP1A2, CYP2D6(strong), CYP2C19, CYP2E1 (weak), CYP3A4, DAO, BCHE Inductor: CYP3A4 Transporter genes: ABCB1 (substrate/inhibitor), ABCB11 (inhibitor), CFTR Pleiotropic genes: ACACA, BDNF, FABP1, LEP, NPY |
![]() | Name: Metoclopramide IUPAC name: 27. Benzamide, 4-amino- 5-chloro-N-[2-(diethylamino)ethyl]-2-methoxy-, monohydrochloride, monohydrate, Molecular formula: C14H22ClN3O2 HCl H Molecular Weight: : 354.2 g/mol Mechanism: Blocks dopamine receptors and (when given in higher doses) also blocks serotonin receptors in chemoreceptor trigger zone of CNS. Enhances response to acetylcholine of tissue in upper GI tract causing enhanced motility and accelerated gastric emptying without stimulating gastric, biliary, or pancreatic secretions. Increases lower esophageal sphincter tone Effect: Prokinetic agents, antiemetic | Pathogenic genes: DRD2 Mechanistic genes: DRD2, CHRM1, HTR4, HTR3A Metabolic genes: Substrate: CYP2D6 (minor), CYP3A4, CYP1A2 (minor) Inhibitor: CYP2D6 (strong) Transporter genes: ABCB1 Pleiotropic genes: ACHE |
Antipsychotics, as antidopaminergic medications, are primarily metabolized through CYP1A2/2D6/3A4/2C19[47]. Of these, CYP2D6 is the most relevant because 40% of these neuroleptics are major substrates of this enzyme. CYP2D6, however, is associated with side effects. Other genes such as HTR2A, SLC18A2, GRIK3, and DRD2 are linked to extrapyramidal reactions[48]. Drugs that exert an antidopaminergic effect on DRD1 are of particular interest. In ND, DRD1 is the pathogenic gene that is involved in the pharmacogenomic response to haloperidol, aripiprazole, olanzapine, quetiapine, or risperdone. Other DRDs (not DRD1) pathogenic variants mediate the adverse effects of antipsychotic drugs such as sulpiride, domperidone, and metroclopramide, causing oropharyngeal dysphagia; this suggests that other dopamine- and non-dopamine pathways mediate blocking of the swallowing phase[37].
TRANSIENT RECEPTOR POTENTIAL CHANNEL (TRP) GENES
Transient receptor potential (TRP) channel genes encode ion channels that are classified into two broad groups: (i) Group 1 includes TRPC (canonical), TRPV (vanilloid), TRPVL (vanilloid-like), TRPM (melastatin), TRPS (soromelastatin), TRPN (no mechanoreceptor potential C), and TRPA (ankyrin); (ii) Group 2 consists of TRPP (polycystic) and TRPML (mucolipin)[49]. Some of these targets represent a therapeutic strategy of interest for dysphagia by stimulating areas that evoke the swallowing reflex. Group 1 genes are the most relevant where TRPV1, TRPA1, and TRPM8, for example, are involved in stimulation of thermal sensitivity and the release of CGRP and inflammatory mediators[50]. These receptors are expressed on trigeminal, vagal, and glossopharyngeal nerve terminals; these nerves are critical in the swallowing process[51,52]. Three compounds of clinical relevance in ND that stimulate these receptors are capsaicin, piperine, and menthol. Capsaicin increases the frequency of spontaneous swallowing by stimulating TRPV1 receptors, piperine stimulates TRPV1/A1 receptors, and menthol stimulates TRPM8 receptors[53,54]. A recent meta-analysis revealed the effectiveness of TRP channel agonists in treating ND[55]. Capsaicin produces the highest therapeutic outcomes by lowering the risk of laryngeal penetration and pharyngeal residue and increasing bolus velocity[54]. Capsaicin also induces the release of SP, a neurotransmitter involved in amplifying the inflammatory response and nociceptive sensitization. Since DBH inhibits capsaicin, a pharmacogenetic study in patients with variants of interest is mandatory[37]. As mechanistic genes, TRPV1 Val585Ile and UCP2 -866 G/A variants correlate with the capsinoid therapeutic response[56]. All three, but mainly capsaicin, inhibit CYP group enzymes (CYP3A4, CYP2C9, and weak in CYP2D6). Furthermore, capsaicin and piperine inhibit CYP1A2[57]. In silico, piperine weakly inhibits CYP2D6 WT and CYP2D6*53[58]. Capsaicin and the other compounds, in addition to exhibiting large heterogeneity in their metabolic genes, exert anti-inflammatory effects by modulating pleiotropic genes such as TNF and ILs[37] [Table 3].
Pharmacogenetics of other drugs in the treatment of neurogenic dysphagia
Drug | Properties | Pharmacogenetics |
![]() | Name: Capsaicin IUPAC name: 6-Nonenamide, (E)-N-[(4-hydroxy-3-methoxy-phenyl) methyl]-8-methyl. Molecular Formula: C18H27NO3. Molecular Weight: 305.41 g/mol Mechanism: Induces release of substance P (main chemomediator of pain impulses from the periphery) from peripheral sensory neurons, depletes the neuron of substance P (after repeated stimulation), and prevents reaccumulation. Effect: Skin and Mucous Membrane Agents, local anesthetics, topical | Pathogenic genes: DBH, MPO, BCHE, TACR2 Mechanistic genes: TRPV1, PHB2, ABCB1, ACOX1, ACSL3, ALOX5, CFTR, F2, FOS, HTR1D, NOS3, NPC1, PPARA, TAC1, TGFB1, UCP2 Metabolic genes: Substrate: GLU, CYP2E1 (minor), UGT1A1, UGT1A7, UGT1A9, UGT1A10, GSTP1 Inhibitor: CYP3A4 (strong), CYP2C9, CYP2D6 (weak), PTGS2, MPO, CYP1A2 (strong), CYP1A2 (strong), CYP19A2 (strong), CYP2E1, DBH, BCHE Inductor: CYP1A1, CYP1A2 Transporter genes: ABCB1 Pleiotropic genes: TNF |
![]() | Name: Piperine IUAC name: (2E,4E)-5-(2H-1,3-Benzodioxol-5-yl)-1-(piperidin-1-yl)penta-2,4-dien-1-one. Molecular Formula: C17H19NO3 Molecular Weight: 285.34 g/mol Mechanism: An alkaloid isolated from the plant Piper nigrum that has a role as an NF-kappaB inhibitor, a plant metabolite, a food component, and a human blood serum metabolite. It is a member of benzodioxoles, an N-acylpiperidine, a piperidine alkaloid, and a tertiary carboxamide. Effect: Skin and mucous membrane agents, local anesthetics, topical | Mechanistic genes: TRPV1, TRPA1, NR1I2, FOS Metabolic genes: Substrate: CYP1A1 Inhibitor: CYP3A4, CYP2C9, CYP2D6 (weak) Transporter genes: ABCB1 (inhibitor) Pleiotropic genes: TNF, IL1B, IL6 |
![]() | Name: Menthol IUPAC name: (1R,2S,5R)-2-isopropyl-5-methylcyclohexanol Molecular Formula: C10H20O Molecular Weight: 156.26 g/mol Mechanism: A local anesthetic with counterirritant qualities, widely used to relieve minor throat irritation. Menthol also acts as a weak κ-opioid receptor agonist. Effect: Skin and mucous membrane agents, local anesthetics, topical | Mechanistic genes: TRPM8, TOP1, FOS Metabolic genes: Substrate: CYP2A6 |
![]() | Name: Imidapril IUPAC name: (4S)-3-[(2S)-2-[[(2S)-1-ethoxy-1-oxo-4-phenylbutan-2-yl]amino]propanoyl]-1-methyl-2-oxoimidazolidine-4-carboxylic acid;hydrochloride Molecular Formula: C2H27N3O6 Molecular weight: 405,44 g/mol Mechanism: Prevents conversion of angiotensin I to angiotensin II, a potent vasoconstrictor. Effect: Angiotensin-converting enzyme inhibitors | Mechanistic genes: ACE, AGT, AGTR1, BDKRB2, CES1, CES2, NOS3 |
![]() | Name: Lisinopril IUPAC name: L-Proline, 1-[N 2-(1-carboxy-3-phenylpropyl)-L- lysyl]-, dihydrate, (S) Molecular Formula: C21H31N3O52H2O Molecular Weight: 441.52 g/mol Mechanism: Competitive inhibitor of angiotensin-converting enzyme (ACE). Prevents conversion of angiotensin I to angiotensin II, a potent vasoconstrictor. Effect: Angiotensin-converting enzyme inhibitors | Mechanistic genes: ACE, ACE2, REN, AGT; BDKRB2, MMP3, NOS3, NPPA Metabolic genes: Substate: CYP3A4/5 (major) |
![]() | Name: Perindopril IUPAC name: 1H-Indole-2-carboxylic acid, 1-[2-[[1-(ethoxycarbonyl)butyl]amino]-1-oxopropyl]octahydro-, [2S-[1[R*(R*)],2α,3aβ,7aβ]]- Molecular Formula: C19H32N2O5 C4H11N Molecular Weight: 441.60 g/mol Mechanism: A prodrug for perindoprilat, which acts as competitive inhibitor of angiotensin-converting enzyme. Prevents c conversion of angiotensin I to angiotensin II, a potent vasoconstrictor, and causes an increase in plasma renin activity and reduction in aldosterone secretion. Effect: Angiotensin-converting enzyme inhibitors | Mechanistic genes: SFRP4, ACE, AGT, AGTR1, MMP2, TGFB1 Metabolic genes: Substate: BCHE Transporter genes: SLC15A1, SLC15A2 |
![]() | Name: Levetiracetam IUPAC name: 1-Pyrrolidineacetamide, α-ethyl-2-oxo-, (α S)- Molecular Formula: C8H14N2O2 Molecular Weight: 170.21 g/mol Mechanism: The precise mechanism by which levetiracetam exerts its antiepileptic effect is unknown and does not appear to derive from any interaction with known mechanisms involved in inhibitory and excitatory neurotransmission. Effect: Anticonvulsants, miscellaneous | Mechanistic genes: SV2A, CACNA1B, MT-TK Metabolic genes: Unknown: CYP2D6, CYP3A4 Transporter genes: ABCB1 |
OTHER DRUGS USED IN NEUROGENIC DYSPHAGIA
Angiotensin-converting enzyme inhibitors (ACE inhibitors) inhibit substance P degradation[59]. These drugs reduce the cough threshold and subsequently can be used in aspiration prophylaxis; however, results from studies on perindopril, lisinopril, or imidapril are inconclusive[59-61]. Imidapril is effective in controlling dysphagia after stroke[30]. In one study, levetiracetam was beneficial to the recovery of dysphagia in post-stroke patients[62]. Several reports describe the usefulness of cough provocation tests with irritants (citric acid, tartaric acid, and mannitol) as a diagnostic tool[63-65], but it remains to be determined whether such agents are useful for treating dysphagia. Table 3 shows the pharmacogenetic profiles of other drugs used to treat ND[37]. It should furthermore be noted that drugs used to treat ND (including dopaminergic agonists) may influence neuroplasticity and axonal regrowth or sprouting to improve, for example, the level of consciousness that would facilitate swallowing[66].
OTHER GENES RELATED TO NEUROGENIC DYSPHAGIA
Few reports have linked other genes to dysphagia. However, the BDNF gene has been studied the most in this regard; the influence of the COMT gene on symptomatic dysphagia has been previously discussed[20]; rs6265 polymorphisms exert disparate effects on pharyngeal stimulation in healthy subjects[67] and appear to influence a better prognosis in swallowing after stroke or poor tolerance to esophageal electrostimulation in carriers of the Met allele[68-70]. Furthermore, a study with a large sample of elderly individuals showed that e4 homozygous APOE carriers have low swallowing evaluation scores[71]. Finally, The T allele of rs17601696 (parent gene FGFR2) is reported to be associated with ND[72].
PHARMACOGENETICS OF DRUGS EMPLOYED IN OTHER ASSOCIATED OROPHARYNGEAL SYMPTOMS IN NEUROGENIC DYSPHAGIA
Together with strategies aimed at controlling ND, it is also important to manage those factors that may exacerbate symptoms and increase the risk of aspiration. Many patients with CNS conditions exhibit sialorrhea, hiccups, xerostomia, or reflux with swallowing difficulties. Prior to considering systemic drugs, it is recommended that local treatment or physical measures be initiated first [Table 4][37].
Pharmacogenetics of drugs in associated symptoms and neurogenic dysphagia
Drug | Properties | Pharmacogenetics |
![]() | Name: Omeprazole IUPAC name: 1H-Benzimidazole, 5-methoxy-2-[[(4-methoxy-3,5-dimethyl-2-pyridinyl)methyl]sulfinyl] Molecular Formula: C17H19N3O3S Molecular weight: 345.42 g/mol Mechanism: Concentrates in acid conditions of parietal cell secretory canaliculi. Forms active sulfenamide metabolite which irreversibly binds to and inactivates hydrogen-potassium ATPase (proton or acid pump), blocking final step in secretion of hydrochloric acid. Acid secretion is inhibited until additional hydrogen-potassium ATPase is synthesized, resulting in prolonged duration of action. Suppresses H. pylori in duodenal ulcer and/or reflux esophagitis infected with organism. Effect: Antiulcer agents and acid suppressants, proton-pump inhibitors, substituted benzimidazole | Mechanistic genes: ATP4A, AHR, ADH1C, ALDH3A1, AHR, ATP4A, ATP4B, CASR, CBR1, CFTR, CHRM3, FMO1, HRH2, MMP2, NR1I2, NR1I3, RRAS2, SNAP25, SSTR2 Metabolic genes: Substrate: CYP1A1, CYP2C8 (minor), CYP2C9 (minor), CYP2C18 (minor), CYP3A4 (major), CYP2C19 (major), CYP2A6 (minor), CYP2D6 (minor) Inhibitor: CYP1A2 (moderate), CYP2C9 (moderate), CYP2D6 (moderate), CYP3A4 (moderate), CYP2C19 (strong) Inducer: CYP1A1, CYP1A2, CYP1B1, CYP3A4, CYP2B6 Transporter genes ABCG2 (inhibitor), ABCC3 (inducer), ABCB1, ABCC6 (substrate/inhibitor), ABCC6, UGT1A1 |
![]() | Name: Pantoprazole IUPAC name: (1) 1H-Benzimidazole, 5-(difluoromethoxy)-2-[[(3,4-dimethoxy-2-pyridinyl)methyl]sulfinyl] Molecular Formula: C16H15F2N3O4S. Molecular weight: 383.37 g/mol Action: Suppresses gastric acid secretion by inhibiting parietal cell H+/K+ ATP pump Effect: Antiulcer agents and acid suppressants, proton-pump inhibitors, substituted benzimidazole | Mechanistic genes: ATP4A, DDAH1, ABCC2, CASR, CHRM3, HRH2, IL1B, PPAs, SNAP25, SSTR2 Metabolic genes: Substrate: CYP3A4 (major), CYP2C19, CYP2C19 (major), CYP2D6 (minor), SULTs, UGTs Inhibitor: CYP2C19 (strong),CYP1A2 (weak), CYP2C9 (moderate), CYP2D6 (weak), CYP3A4 (moderate) Inducer: CYP1A2, CYP3A4 Transporter genes: ABCB1 (substrate/inhibitor), ABCG2 (substrate/Inhibitor), SLC22A8 (inhibitor) |
![]() | Name: Lansoprazole IUPAC name: 1H-Benzimidazole, 2-[[[3-methyl-4-(2,2,2-trifluoroethoxy)-2-pyridinyl]methyl]sulfinyl]- Molecular Formula: C16H14F3N3O2S Molecular Weight: 369.36 g/mol Mechanism: Decreases acid secretion in gastric parietal cells through inhibition of (H +, K +)-ATPase enzyme system, blocking final step in gastric acid production Effect: Antiulcer agents and acid suppressants, proton-pump inhibitors, substituted benzimidazole | Mechanistic genes: ATP4A; CASR, MAPT, CYP1A1, CYP1B1, HRH2, SNAP25, SSTR2 Metabolic genes: Substrate: CYP2C8 (major), CYP2C9 (major), CYP2C18 (major), CYP2C19 (major), CYP3A4/5 (major); POR Inhibitor: CYP2C9, (moderate), CYP2C19 (strong), CYP3A4 CYP2D6 (moderate), CYP2E1 (moderate), CYP3A4 (moderate), PPA1 Inducer: CYP1A2, CYP1A1, CYP1B1, CYP2C9, CYP3A4 Transporter genes: ABCG2 (inhibitor), ABCB1 (substrate/inhibitor), SLC22A8 (inhibitor), SLC22A1, SLC22A2, SLC22A3 |
![]() | Name: Rabeprazole IUPAC name: 1H-Benzimidazole, 2-[[[4-(3-methoxypropoxy)-3-methyl-2-pyridinyl]methyl]sulfinyl] Molecular Formula: C18H20N3NaO3S Molecular weight: 381.42 g/mol Action: Suppresses gastric acid secretion by inhibiting parietal cell H+/K+ ATP pump Effect: Antiulcer agents and acid suppressants, proton-pump inhibitors, substituted benzimidazole | Mechanistic genes: ATP4A, DDAH1, ATP4B, CASR, CHRM3, HRH2, HTR1D, NR1I2, SNAP25, SSTR2 Metabolic genes: Substrate: CYP3A4 (major), CYP2C19 (major), CYP2D6 (major) Inhibitor: CYP2C9 (moderate), CYP2C8 (moderate), CYP2C19 (strong), CYP2D6 (moderate) Transporter genes: ABCB1 (substrate/ inhibitor), ABCG2 (substrate/inhibitor), SLC22A8 (inhibitor) |
![]() | Name: Famotidine IUPAC name: Propanimidamide, N’-(aminosulfonyl)-3-[[[2-[(diaminomethylene)amino]-4-thiazolyl]methyl]thio]- Molecular formular: C8H15N7O2S3 Molecular weight: 337.45 g/mol Action: Famotidine works by reducing the amount of acid in the stomach, thereby reducing pain and allowing the ulcer to heal, and through a competitive inhibition of histamine at H2 receptors of gastric parietal cells, which inhibits gastric acid secretion. Effect: Antiulcer agents and acid suppressants, histamine H2-antagonists | Pathogenic genes: HRH2 Mechanistic genes: HRH2, CAT, FOS Metabolic genes: Inhibitor: CYP1A2 Transporter genes: SLC22A6, SLC22A8 (Substrate/inhibitor), SCL22A2 (Inhibitor), SLC47A1 (Inhibitor) |
![]() | Name: Pilocarpine IUPAC name:. 2(3H)-Furanone, 3-ethyldihydro-4-[(1-methyl-1H-imidazol-5-yl)methyl]-, monohydrochloride, (3S-cis)- Molecular Formula: C11H16N2O2 Molecular weight: 244.72 g/mol Mechanism: Directly stimulates cholinergic receptors in eye causing miosis (by contraction of iris sphincter) and loss of accommodation (by constriction of ciliary muscle) and lowering of intraocular pressure (with decreased resistance to aqueous humor outflow) Effect: Antiglaucoma agents, miotics, cholinergic agonists | Pathogenic genes: BDNF Mechanistic genes:CHRM3,CHRM1, CHRM2, CHRM4 BDNF; CHRNs; FOS; GRIA3 Metabolic genes: Substrate: CYP1A2 (minor), CYP2C9 (minor), CYP2C19 (minor), CYP2D6 (minor), CYP3A4 (minor) Inhibitor: CYP2A6, CYP3A4 (weak), CYP2A6 (weak), CYP2E1 (weak) |
![]() | Name: Amitriptyline IUPAC Name: dimethyl(3-{tricyclo[9.4.0.03,8]pentadeca-1(15),3,5,7,11,13-hexaen-2-ylidene}propyl)amine Molecular Formula: C20H24ClN Molecular Weight: 313.86426 g/mol Mechanism: Increases synaptic concentration of serotonin and/or norepinephrine in the central nervous system by inhibiting their reuptake in the presynaptic neuronal membrane Effect: Adrenergic uptake inhibition, antimigraine activity, analgesic (nonnarcotic) activity, antidepressant action | Pathogenic genes: ABCB1, GNB3, HTRs, NTRK2, SLC6A4, TNF Mechanistic genes: ADRA1A, ADRA1B, ADRA1D, ADRA2A, HTRs, HRH1, HRH2, HRH4, SIGMAR1, NTRK1, NTRK2, OPRD1, OPRK1, OPRM1 Metabolic genes: Substrate: CYP1A2 (minor), CYP2B6 (minor), CYP2C8, CYP2C9 (minor), CYP2C19 (minor), CYP2D6 (major), CYP3A4/5 (major), GSTP1, UGT1A3, UGT1A4, UGT2B10 Inhibitor: CYP1A2 (moderate), CYP2C9 (moderate), CYP2C19 (moderate), CYP2D6 (moderate), CYP2E1 (weak) Transporter genes: ABCB1 (substrate/inhibitor), ABCC2 (inhibitor), ABCG2 (inhibitor), KCNA1, KCNE2, KCNH2, KCNQ1, KCNQ2, KCNQ3, SCN5A, SLC6A2, SLC6A4 Pleiotropic genes: FABP1, GNAS, GNB3, NTRK1, TNF |
![]() | Name: Scopolamine IUPAC Name: Benzeneacetic acid, α-(hydroxymethyl)-, 9-methyl-3-oxa-9-azatricyclo[3.3.1.02,4]non-7-yl ester, hydrobromide, trihydrate, [7(S)-(1α,2β,4β,5α,7β)]- Molecular Formula: C17H21NO4HBr3H2O Molecular weight: 438.31 g/mol Mechanism: Competitively inhibits acetylcholine and other cholinergic stimuli at autonomic effectors innervated by postganglionic cholinergic nerves and, to a lesser extent, on smooth muscles that lack cholinergic innervation. Doses used to decrease gastric secretions likely to cause dryness of mouth (xerostomia). Antagonizes histamine and serotonin Effect: Anticholinergic agents, antimuscarinics/antispasmodics | Mechanistic genes: CHRM1, CHRM2, CHRM3, CHRM4, CHRM5, CHRNA4, CHRNB2, SI Metabolic genes: Substrate:CYP3A4 |
![]() | Name: Glycopyrrolate IUPAC Name: Pyrrolidinium, 3-[(cyclopentylhydroxyphenylacetyl)oxy]-1,1-dimethyl-, bromide Molecular Formula: C19H28BrNO3 Molecular Weight: 398.33 g/mol Mechanism: Blocks action of acetylcholine at parasympathetic sites in smooth muscle, secretory glands, and CNS Effect: Anticholinergic agents, antimuscarinics/antispasmodics | Mechanistic genes: CHRM1, CHRM2, CHRM3, CHRM4, CHRM5 Metabolic genes: Substrate: CYP1A2, CYP2B6, CYP2C9, CYP2D6,CYP2C18, CYP2C19, CYP3A4 Transporter genes: SLC22A2, SLC47A1 |
![]() | Name: Trihexyphenidyl IUPAC Name: 1-Piperidinepropanol,α-cyclohexyl-α-phenyl Molecular Formula: C20H31NO Molecular Weight: 301,46 g/mol Mechanism: Exerts direct inhibitory effect on parasympathetic nervous system. It also has a relaxing effect on smooth musculature, exerted both directly on muscle itself and indirectly through parasympathetic nervous system (inhibitory effect) Effect: Antiparkinsonian agents, anticholinergic agents | Pathogenic genes: PARK2 Mechanistic genes: CHRM1, CHRM2, CHRM3, CHRM4, CHRM5 |
![]() | Name: Atropine IUPAC Name: Benzeneacetic acid, α-(hydroxymethyl)-8-methyl-8-azabicyclo[3.2.1]oct-3-yl ester, endo-(–) Molecular Formula: C17H23NO3 Molecular Weight: 289.37 g/mol Mechanism: Blocks the action of acetylcholine at parasympathetic sites in smooth muscle, secretory glands, and CNS. Increases cardiac output, dries secretions. Reverses the muscarinic effects of cholinergic poisoning Effect: Mydriatics, anticholinergic agents, antimuscarinics/antispasmodics, antidote | Mechanistic genes: CHRM1, CHRM2; CHRM3, CHRM4, CHRM5, CHRNA4, CHRNB2, FOS, GLRA1, PTGS2, TP53 Transporter genes: ABCB11 Pleiotropic genes: ACHE, CES1 |
![]() | Name: Domperidone IUPAC name: 2H-Benzimidazol-2-one, 5-chloro-1-[1-[3-(2,3-dihydro-2-oxo-1H-benzimidazol-1-yl)propyl]-4-piperidinyl]-1,3-dihydro- Molecular Formula: C 22H24ClN5O2 Molecular weight: 425.91 g/mol Mechanism: Has peripheral dopamine receptor blocking properties. Increases esophageal peristalsis; lowers esophageal sphincter pressure, gastric motility, and peristalsis; and enhances gastroduodenal coordination, therefore facilitating gastric emptying and decreasing small bowel transit time Effect: Prokinetic agents, dopamine antagonist | Pathogenic genes: DRD2, DRD3 Mechanistic genes: DRD2, DRD3 Metabolic genes: Substrate: CYP3A5 (major), CYP3A7, CYP3A4 (major), CYP1A2 (minor), CYP2B6 (minor), CYP2C8 (minor), CYP2D6 (minor), CYBs (major) Transporter genes: ABCB1 |
![]() | Name: Baclofen IUPAC name: Butanoic acid, 4-amino-3-(4-chlorophenyl)- Molecular Formula: C10H12ClNO Molecular weight: 213.66 g/mol Mechanism: Inhibits the transmission of mono/polysynaptic reflexes at the spinal cord level, possibly by hyperpolarization of primary afferent fiber terminals Effect: GABA-derivative skeletal muscle relaxants | Mechanistic genes: GABBR1, GABBR2, CXCR4, CFTR Transporter genes: ABCC9, ABCC12, SLC28A1 |
Sialorrhea
The most used treatments for the control of hypersalivation in patients with neurological damage are based on their anticholinergic profiles. This includes a heterogeneous group of drugs such as amitriptyline, scopolamine, glycopyrronium chloride, trihexyphenidyl, atropine, or thiopium bromide. These anticholinergic agents present an added benefit in the control of other motor symptoms, as occurs in patients with Parkinson’s disease[73]. However, their main drawback is the occurrence of frequent side effects that include sedation, cognitive deficits, constipation, urinary retention, tremor, and blurred vision. Within a population where the prevalence of dementia is high, elderly patients often use drugs with anticholinergic effects, and frequently in combination. Furthermore, in this patient population, polymedication may mask symptoms that are misdiagnosed as pathology unrelated to drug toxicity[74].
Concerning the pharmacogenetic profile, anticholinergic drug exposure shows associated variants located at chromosome 3p21.1 locus, with the top
Xerostomia
The first line of treatment for xerostomia is to employ local therapies (artificial saliva, sialogogues), avoiding the use of systemic medications (pilocarpine) as the first choices due to their common negative effects. Side effects include blurred vision, bronchoconstriction, hiccup, sweating, hypotension, bradycardia, cutaneous vasodilatation, nausea, diarrhea, or increased urinary frequency[78]. Polymorphisms in CYP2A6 modify the pharmacokinetics of this drug, where the clearance of pilocarpine is significantly lower. In vivo, these slow metabolizers have two inactive CYP2A6 alleles: CYP2A6*4A, CYP2A6*7, CYP2A6*9, or CYP2A6*10[79].
Pharyngolaryngeal reflux
Proton-pump inhibitors (PPI) and H2 receptor antagonists show improvements in gastro‐esophageal reflux disease‐like symptoms, being PPIs more effective in subjects with negative endoscopic findings[80]. CYP2C19 is the most prominent of the PPI-metabolizing enzymes; CYP2C19-specific single nucleotide polymorphisms reduce clearance proportionally and increase exposure and prolong proton-pump inhibition. Differences in CYP2C19-mediated metabolism lead to marked interpatient variability in acid suppression, drug–drug interaction potential, and clinical efficacy[81-84]. This phenomenon has also been observed with CYP3A4, but to a lesser degree[82].
Hiccup
Pharmacologically, multiple drugs with different targets are available to control hiccups. Baclofen is a drug commonly used in intractable hiccups[85]. The ABCC9 SNP (rs11046232, heterozygous AT versus reference TT genotype) is associated with a two-fold increase in oral baclofen clearance[86]. Allelic variants with the ABCC12, SLC28A1, and PPARD SNPs generate variable responses in cerebral palsy[86]. Chlorpromazine, domperidone, and metoclopramide can also be useful. However, since these are antidopaminergic drugs, they should be prescribed with caution because they may worsen dysphagia. Domperidone would be recommended amongst these medications because of its limited transit through the blood–brain barrier and exceptional central effects[87]. Paradoxically, metoclopramide and other antidopaminergic drugs may be beneficial by reducing nausea and vomiting in patients with ND, and therefore the risk of aspiration. In these cases, dose adjustment and patient selection are essential due to the risk of adverse effects[45].
CONCLUSION
Treatment of ND must be comprehensive and multidisciplinary. Pharmacological treatments are support tools for other therapeutic measures. Dopamine is the main neurotransmitter implicated in these swallowing disorders. Of the genes that encode dopaminergic receptors, DRD1 is the most important in the prediction and treatment of ND. Other genes such as COMT and DBH have also been considered in the management of ND. Polymorphisms in dopaminergic and antidopaminergic agents are associated, respectively, with undesired or insufficient effects and increased risk of swallowing impairment. SP is another main factor in the treatment of ND, which can be altered with antidopaminergic agents. SP degradation is blocked with TRP channel agonists such as capsaicin, piperine, menthol, and ACE inhibitors. Genetic variants influence the therapeutic response of TRP channel agonists. When symptoms coexist that can worsen dysphagia and increase the risk of aspiration (e.g., reflux, xerostomia, sialorrhea, and hiccups), it is recommended to carefully associate other medications with ND treatment due to the risk of adverse effects, which may even include swallowing disorders. Dose adjustment and choice of drug in polypharmacy patients is one of the main objectives of a pharmacogenetic analysis.
DECLARATIONS
AcknowledgmentsJoaquin Guerra would like to thank Dante Doncel Guerrero for being the source of inspiration to develop this article.
Authors’ contributionsMade substantial contributions to conception and design of the review and interpretation: Guerra J
Read, adjusted and approved the final manuscript: Naidoo V, Cacabelos R
Availability of data and materialsNot applicable.
Financial support and sponsorshipNone.
Conflicts of interestAll authors declared that there are no conflicts of interest.
Ethical approval and consent to participateNot applicable.
Consent for publicationNot applicable.
Copyright© The Author(s) 2022.
REFERENCES
1. Panebianco M, Marchese-Ragona R, Masiero S, Restivo DA. Dysphagia in neurological diseases: a literature review. Neurol Sci 2020;41:3067-73.
2. Warnecke T, Labeit B, Schroeder J, et al. Neurogenic dysphagia: systematic review and proposal of a classification system. Neurology 2021;96:e876-89.
3. Sura L, Madhavan A, Carnaby G, Crary MA. Dysphagia in the elderly: management and nutritional considerations. Clin Interv Aging 2012;7:287-98.
4. Wirth R, Dziewas R, Beck AM, et al. Oropharyngeal dysphagia in older persons - from pathophysiology to adequate intervention: a review and summary of an international expert meeting. Clin Interv Aging 2016;11:189-208.
5. Nascimento W, Tomsen N, Acedo S, et al. Effect of aging, gender and sensory stimulation of TRPV1 Receptors with capsaicin on spontaneous swallowing frequency in patients with oropharyngeal dysphagia: a proof-of-concept study. Diagnostics (Basel) 2021;11:461.
6. Dziewas R, Allescher HD, Aroyo I, et al. Diagnosis and treatment of neurogenic dysphagia - S1 guideline of the German society of neurology. Neurol Res Pract 2021;3:23.
7. Speyer R, Baijens L, Heijnen M, Zwijnenberg I. Effects of therapy in oropharyngeal dysphagia by speech and language therapists: a systematic review. Dysphagia 2010;25:40-65.
8. Warnecke T, Dziewas R, Langmore S. Neurogenic dysphagia. 1st ed. Cham: Springer, 2021.
11. Crouse EL, Alastanos JN, Bozymski KM, Toscano RA. Dysphagia with second-generation antipsychotics: A case report and review of the literature. Ment Health Clin 2017;7:56-64.
12. Wolf U, Eckert S, Walter G, et al. Prevalence of oropharyngeal dysphagia in geriatric patients and real-life associations with diseases and drugs. Sci Rep 2021;11:21955.
13. Eisenhofer G, Kopin IJ, Goldstein DS. Catecholamine metabolism: a contemporary view with implications for physiology and medicine. Pharmacol Rev 2004;56:331-49.
14. Vidal P-P, Sans A. Chapter 30: Vestibular system. In: Paxinos G, editor. The rat nervous system, 3dr ed. London: Academic Press, 2004; pp. 965-96.
15. Tritsch NX, Sabatini BL. Dopaminergic modulation of synaptic transmission in cortex and striatum. Neuron 2012;76:33-50.
16. Bieger D, Giles S, Hockman C. Dopaminergic influences on swallowing. Neuropharmacology 1977;16:245-52.
17. Jia YX, Sekizawa K, Ohrui T, Nakayama K, Sasaki H. Dopamine D1 receptor antagonist inhibits swallowing reflex in guinea pigs. Am J Physiol 1998;274:R76-80.
18. Gantois I, Fang K, Jiang L, et al. Ablation of D1 dopamine receptor-expressing cells generates mice with seizures, dystonia, hyperactivity, and impaired oral behavior. Proc Natl Acad Sci USA 2007;104:4182-7.
19. Park HY, Kim Y, Oh HM, Kim TW, Park GY, Im S. Potential prognostic impact of dopamine receptor d1 (. rs4532 ;12:675060.
20. Nimmons D, Pendleton N, Payton A, et al. A novel association between COMT and BDNF gene polymorphisms and likelihood of symptomatic dysphagia in older people. Neurogastroenterol Motil 2015;27:1223-31.
21. Warnecke T, Oelenberg S, Teismann I, et al. Endoscopic characteristics and levodopa responsiveness of swallowing function in progressive supranuclear palsy. Mov Disord 2010;25:1239-45.
22. Warnecke T, Suttrup I, Schröder JB, et al. Levodopa responsiveness of dysphagia in advanced Parkinson’s disease and reliability testing of the FEES-Levodopa-test. Parkinsonism Relat Disord 2016;28:100-6.
23. Hunter PC, Crameri J, Austin S, Woodward MC, Hughes AJ. Response of parkinsonian swallowing dysfunction to dopaminergic stimulation. J Neurol Neurosurg Psychiatry 1997;63:579-83.
24. Hirano M, Isono C, Sakamoto H, Ueno S, Kusunoki S, Nakamura Y. Rotigotine transdermal patch improves swallowing in dysphagic patients with parkinson’s disease. Dysphagia 2015;30:452-6.
25. Hirano M, Isono C, Fukuda K, Ueno S, Nakamura Y, Kusunoki S. Effects of the rotigotine transdermal patch versus oral levodopa on swallowing in patients with Parkinson’s disease. J Neurol Sci 2019;404:5-10.
26. Tison F, Wiart L, Guatterie M, et al. Effects of central dopaminergic stimulation by apomorphine on swallowing disorders in Parkinson’s disease. Mov Disord 1996;11:729-32.
27. Michou E, Hamdy S, Harris M, et al. Characterization of corticobulbar pharyngeal neurophysiology in dysphagic patients with Parkinson’s disease. Clin Gastroenterol Hepatol 2014;12:2037-45.e1.
28. Chang MC, Park JS, Lee BJ, Park D. Effectiveness of pharmacologic treatment for dysphagia in Parkinson’s disease: a narrative review. Neurol Sci 2021;42:513-9.
29. Kobayashi H, Nakagawa T, Sekizawa K, Arai H, Sasaki H. Levodopa and swallowing reflex. Lancet 1996;348:1320-1.
30. Arai T, Sekizawa K, Yoshimi N, Toshima S, Fujiwara H. Cabergoline and silent aspiration in elderly patients with stroke. J Am Geriatr Soc 2003;51:1815-6.
31. Nakagawa T, Wada H, Sekizawa K, Arai H, Sasaki H. Amantadine and pneumonia. Lancet 1999;353:1157.
32. Apaydin H, Ertan S, Ozekmekçi S. Broad bean (Vicia faba) - A natural source ofL-dopa-Prolongs “on” periods in patients with Parkinson’s disease who have “on-off” fluctuations. Mov Disord 2000;15:164-6.
33. Kanazawa K, Sakakibara H. High content of dopamine, a strong antioxidant, in Cavendish banana. J Agric Food Chem 2000;48:844-8.
34. Pulikkalpura H, Kurup R, Mathew PJ, Baby S. Levodopa in Mucuna pruriens and its degradation. Sci Rep 2015;5:11078.
35. Katzenschlager R, Evans A, Manson A, et al. Mucuna pruriens in Parkinson’s disease: a double blind clinical and pharmacological study. J Neurol Neurosurg Psychiatry 2004;75:1672-7.
36. Cacabelos R, Carrera I, Martínez O, et al. Atremorine in parkinson’s disease: from dopaminergic neuroprotection to pharmacogenomics. Med Res Rev 2021;41:2841-86.
37. Cacabelos R. World guide for drug use and pharmacogenomics. Corunna, Spain: EuroEspes Publishing Co., 2012.
38. Soler-Alfonso C, Motil KJ, Turk CL, et al. Potocki-Lupski syndrome: a microduplication syndrome associated with oropharyngeal dysphagia and failure to thrive. J Pediatr 2011;158:655-659.e2.
39. Michałowska M, Chalimoniuk M, Jówko E, et al. Gene polymorphisms and motor levodopa-induced complications in Parkinson’s disease. Brain Behav 2020;10:e01537.
40. Zhao C, Wang Y, Zhang B, Yue Y, Zhang J. Genetic variations in catechol-O-methyltransferase gene are associated with levodopa response variability in Chinese patients with Parkinson’s disease. Sci Rep 2020;10:9521.
41. Rieck M, Schumacher-Schuh AF, Callegari-Jacques SM, et al. Is there a role for ADORA2A polymorphisms in levodopa-induced dyskinesia in Parkinson’s disease patients? Pharmacogenomics 2015;16:573-82.
42. Schumacher-Schuh AF, Altmann V, Rieck M, et al. Association of common genetic variants of HOMER1 gene with levodopa adverse effects in Parkinson’s disease patients. Pharmacogenomics J 2014;14:289-94.
43. Rieck M, Schumacher-Schuh AF, Altmann V, et al. DRD2 haplotype is associated with dyskinesia induced by levodopa therapy in Parkinson’s disease patients. Pharmacogenomics 2012;13:1701-10.
44. Moreau C, Meguig S, Corvol JC, et al. Polymorphism of the dopamine transporter type 1 gene modifies the treatment response in Parkinson’s disease. Brain 2015;138:1271-83.
45. Ahmed SS, Husain RS, Kumar S, Ramakrishnan V. Association between MDR1 gene polymorphisms and Parkinson’s disease in Asian and Caucasian populations: a meta-analysis. J Neurol Sci 2016;368:255-62.
46. Lohr KM, Masoud ST, Salahpour A, Miller GW. Membrane transporters as mediators of synaptic dopamine dynamics: implications for disease. Eur J Neurosci 2017;45:20-33.
47. Pouget JG, Shams TA, Tiwari AK, Müller DJ. Pharmacogenetics and outcome with antipsychotic drugs. Dialogues Clin Neurosci 2014;16:555-66.
48. Yoshida K, Müller DJ. Pharmacogenetics of antipsychotic drug treatment: update and clinical implications. Mol Neuropsychiatry 2020;5:1-26.
50. Kichko TI, Neuhuber W, Kobal G, Reeh PW. The roles of TRPV1, TRPA1 and TRPM8 channels in chemical and thermal sensitivity of the mouse oral mucosa. Eur J Neurosci 2018;47:201-10.
51. Alvarez-Berdugo D, Rofes L, Farré R, et al. Localization and expression of TRPV1 and TRPA1 in the human oropharynx and larynx. Neurogastroenterol Motil 2016;28:91-100.
52. Hossain MZ, Ando H, Unno S, Masuda Y, Kitagawa J. Activation of TRPV1 and TRPM8 channels in the larynx and associated laryngopharyngeal regions facilitates the swallowing reflex. Int J Mol Sci 2018;19:4113.
53. Peier AM, Moqrich A, Hergarden AC, et al. A TRP channel that senses cold stimuli and menthol. Cell 2002;108:705-15.
54. Alvarez-Berdugo D, Rofes L, Arreola V, Martin A, Molina L, Clavé P. A comparative study on the therapeutic effect of TRPV1, TRPA1, and TRPM8 agonists on swallowing dysfunction associated with aging and neurological diseases. Neurogastroenterol Motil 2018;30:e13185.
55. Cheng I, Sasegbon A, Hamdy S. Effects of pharmacological agents for neurogenic oropharyngeal dysphagia: A systematic review and meta-analysis. Neurogastroenterol Motil 2022;34:e14220.
56. Snitker S, Fujishima Y, Shen H, et al. Effects of novel capsinoid treatment on fatness and energy metabolism in humans: possible pharmacogenetic implications. Am J Clin Nutr 2009;89:45-50.
57. Shamsi S, Tran H, Tan RS, Tan ZJ, Lim LY. Curcumin, piperine, and capsaicin: a comparative study of spice-mediated inhibition of human cytochrome P450 isozyme activities. Drug Metab Dispos 2017;45:49-55.
58. Don CG, Smieško M. In Silico Pharmacogenetics CYP2D6 Study Focused on the Pharmacovigilance of Herbal Antidepressants. Front. Pharmacol 2020;11:683.
59. Ikeda J, Kojima N, Saeki K, Ishihara M, Takayama M. Perindopril increases the swallowing reflex by inhibiting substance P degradation and tyrosine hydroxylase activation in a rat model of dysphagia. Eur J Pharmacol 2015;746:126-31.
60. Shinohara Y, Origasa H. Post-stroke pneumonia prevention by angiotensin-converting enzyme inhibitors: results of a meta-analysis of five studies in Asians. Adv Ther 2012;29:900-12.
61. Lee JS, Chui PY, Ma HM, et al. Does low dose angiotensin converting enzyme inhibitor prevent pneumonia in older people with neurologic dysphagia - a randomized placebo-controlled trial. J Am Med Dir Assoc 2015;16:702-7.
62. Chen D, Xing H, Jiang Q, Xiang Y, Hu W, Guo H. Role of levetiracetam in the rehabilitation of dysphagia due to stroke. Int J of Pharmacol 2017;13:603-11.
63. Monroe MD, Manco K, Bennett R, Huckabee M. Citric acid cough reflex test: Establishing normative data. J Speech Lang Hear 2014;17:216-24.
64. Fujiwara K, Kawamoto K, Shimizu Y, et al. A novel reflex cough testing device. BMC Pulm Med 2017;17:19.
65. Nurmi HM, Lätti AM, Brannan JD, Koskela HO. Comparison of mannitol and citric acid cough provocation tests. Respir Med 2019;158:14-20.
66. Krieger DW. Therapeutic drug approach to stimulate clinical recovery after Brain injury. Front Neurol Neurosci 2013:76-87.
67. Jayasekeran V, Pendleton N, Holland G, et al. Val66Met in brain-derived neurotrophic factor affects stimulus-induced plasticity in the human pharyngeal motor cortex. Gastroenterology 2011;141:827-836.e1.
68. Oh HM, Kim TW, Park HY, Kim Y, Park GY, Im S. Role of rs6265 BDNF polymorphisms and post-stroke dysphagia recovery-A prospective cohort study. Neurogastroenterol Motil 2021;33:e13953.
69. Vasant DH, Payton A, Mistry S, Thompson DG, Hamdy S. The val66met polymorphism of brain-derived neurotrophic factor is associated with human esophageal hypersensitivity. Neurogastroenterol Motil 2013;25:162-e85.
70. Essa H, Vasant D, Raginis-zborowska A, Payton A, Michou E, Hamdy S. PTU-182 Can response to pharyngeal stimulation in dysphagic stroke be predicted by bdnf genetic polymorphisms? Gut 2015;64:A143.1-A143.
71. Mentz H, Horan M, Payton A, Ollier W, Pendleton N, Hamdy S. Homozygosity in the ApoE 4 polymorphism is associated with dysphagic symptoms in older adults. Dis Esophagus 2015;28:97-103.
72. Raginis-Zborowska A, Mekli K, Payton A, Ollier W, Hamdy S, Pendleton N. Genetic determinants of swallowing impairments among community dwelling older population. Exp Gerontol 2015;69:196-201.
73. Katzenschlager R, Sampaio C, Costa J, Lees A. Anticholinergics for symptomatic management of Parkinson’s disease. Cochrane Database Syst Rev 2003;2002:CD003735.
74. Schuh MJ, Crosby S. Chronic anticholinergic toxicity discovered in a pharmacogenomics, polypharmacy patient. Sr Care Pharm 2021;36:304-10.
75. Eum S, Hill SK, Alliey-Rodriguez N, et al. Genome-wide association study accounting for anticholinergic burden to examine cognitive dysfunction in psychotic disorders. Neuropsychopharmacology 2021;46:1802-10.
76. Kersten H, Wyller TB, Molden E. Association between inherited CYP2D6/2C19 phenotypes and anticholinergic measures in elderly patients using anticholinergic drugs. Ther Drug Monit 2014;36:125-30.
77. Gurocak S, Konac E, Ure I, et al. The impact of gene polymorphisms on the success of anticholinergic treatment in children with overactive bladder. Dis Markers 2015;2015:732686.
78. Villa A, Connell CL, Abati S. Diagnosis and management of xerostomia and hyposalivation. Ther Clin Risk Manag 2015;11:45-51.
79. Endo T, Nakajima M, Fukami T, et al. Genetic polymorphisms of CYP2A6 affect the in vivo pharmacokinetics of pilocarpine. Pharmacogenet Genomics 2008;18:761-72.
80. Sigterman KE, van Pinxteren B, Bonis PA, Lau J, Numans ME. Short-term treatment with proton pump inhibitors, H2-receptor antagonists and prokinetics for gastro-oesophageal reflux disease-like symptoms and endoscopy negative reflux disease. Cochrane Database Syst Rev 2013;5:CD002095.
81. Addington WR, Stephens RE, Gilliland K, Miller SP. Tartaric acid-induced cough and the superior laryngeal nerve evoked potential. Am J Phys Med Rehabil 1998;77:523-6.
82. Ward RM, Kearns GL. Proton pump inhibitors in pediatrics: mechanism of action, pharmacokinetics, pharmacogenetics, and pharmacodynamics. Paediatr Drugs 2013;15:119-31.
83. Rouby N, Lima JJ, Johnson JA. Proton pump inhibitors: from CYP2C19 pharmacogenetics to precision medicine. Expert Opin Drug Metab Toxicol 2018;14:447-60.
84. Harris DM, Stancampiano FF, Burton MC, et al. Use of pharmacogenomics to guide proton pump inhibitor therapy in clinical practice. Dig Dis Sci 2021;66:4120-7.
85. Patial RK. Baclofen in the treatment of intractable hiccups. J Assoc Physic India 2002;50:1312-3.
86. McLaughlin MJ, He Y, Brunstrom-Hernandez J, et al. Pharmacogenomic variability of oral baclofen clearance and clinical response in children with cerebral palsy. PMR 2018;10:235-43.
Cite This Article
How to Cite
Guerra, J.; Naidoo V.; Cacabelos R. Neurogenic dysphagia: current pharmacogenomic perspectives. J. Transl. Genet. Genom. 2022, 6, 304-21. http://dx.doi.org/10.20517/jtgg.2022.08
Download Citation
Export Citation File:
Type of Import
Tips on Downloading Citation
Citation Manager File Format
Type of Import
Direct Import: When the Direct Import option is selected (the default state), a dialogue box will give you the option to Save or Open the downloaded citation data. Choosing Open will either launch your citation manager or give you a choice of applications with which to use the metadata. The Save option saves the file locally for later use.
Indirect Import: When the Indirect Import option is selected, the metadata is displayed and may be copied and pasted as needed.
About This Article
Special Issue
Copyright
Data & Comments
Data
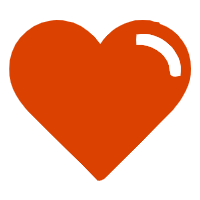
Comments
Comments must be written in English. Spam, offensive content, impersonation, and private information will not be permitted. If any comment is reported and identified as inappropriate content by OAE staff, the comment will be removed without notice. If you have any queries or need any help, please contact us at support@oaepublish.com.