Aging and rejuvenation of engineered cardiovascular tissues: from research to clinical application
Abstract
Aging is a key contributor to the pathogenesis of cardiovascular diseases (CVDs). However, current methods and models of CVD do not include the factor of aging due to the use of premature cardiomyocytes. There is an urgent need for an engineered cardiovascular tissue (ECT) model that includes aging as the greatest CVD risk factor to facilitate drug development for aged CVD patients. Cell therapy, which transplants pluripotent stem cell-derived cardiomyocytes in patients, was proved to be effective for cardiac repair, while the cell retention rate is limited. Alternatively, implantation of ECT could enable long-term retention of cells after translation and may result in rejuvenation in aged hearts. This review summarizes the key features of aging and the influencing factors in engineered cardiovascular tissues. The applications and challenges of engineered myocardium designed for clinical use are also discussed.
Keywords
INTRODUCTION
The elderly population is at higher risk of cardiovascular diseases (CVDs), with approximately 66% of cases occurring after the age of 75[1]. Given the increasing average life span worldwide, the severe consequence of CVD is expected to notably arouse social concern in the coming years[2,3]. However, cardiomyocytes in post-mitotic heart progressively decline, and adult cardiovascular tissue is difficult to regenerate once injured[4]. Therefore, regenerative therapy has become a research hotspot in recent years. These regenerative strategies include pharmaceutical induction of regeneration in situ and cell therapy via transplantation. Engineered cardiovascular tissue (ECT) may serve as a useful study tool in both directions.
The development of efficient CVD drugs for the aging population is of great importance. However, prevailing models of CVD fall short in presenting the aging features because they use premature cardiomyocytes. It is essential to compare the differential gene expression in young cardiomyocytes versus old cardiomyocytes to reveal the key molecule targets and pathways affected by aging[5]. This comparison can help us to find functional molecules, such as extracellular matrix components, which are capable of reversing the aging features[5,6]. Therefore, the generation of age-appropriate cardiac models is of great research value. However, the challenges of modeling aged cardiovascular tissues are hampering the development of therapies. Some existing models used aged rat cardiomyocytes to construct cardiovascular tissues[7]; however, rodent cardiomyocytes have different molecular expression and electrophysiological properties compared with human cardiomyocytes, which may not fully capture the complexities of aged patients’ tissues. Using induced pluripotent stem cell (iPSC) derived cardiomyocytes (iPSC-CM) may be an alternative strategy, but there is a lack of a cost-effective model simulating the pathogenic process of the aged human heart using iPSC-CM. The existing aged iPSC-CM model requires up to 14-month prolonged culture[5,8]. New strategies that can accelerate the aging of engineered cardiovascular tissues may solve this problem. Understanding the mechanisms implicated in aging and rejuvenation of engineered cardiovascular tissues will reveal crucial insights for developing new therapies or adapting existing therapies. Furthermore, the transplantation of ECT provides the possibility of heart regeneration. This review examines the effect of aging on engineered cardiovascular tissues, focusing on features such as declined cardiac contraction, increased tissue stiffness, and DNA damage. The influencing factors of aging such as oxidative stress, SIRT2 deficiency, and LMNA gene mutation are clarified. The consequences of enhanced rejuvenation in cardiovascular tissues and the underlying mechanisms are summarized. Moreover, the potential application of engineered cardiovascular tissues to rejuvenate aged hearts and associated challenges are discussed in detail.
ENGINEERED CARDIOVASCULAR TISSUE IN VITRO: AN AGING MODEL
Features and influencing factors of aging in engineered cardiovascular tissues
In vitro models of cardiovascular diseases aim to provide insights into pathological progression and assess the rejuvenating effects of pharmacological treatments. However, the contribution of aging is largely underrepresented due to the use of neonatal or premature cardiomyocytes. Therefore, generating ECT models recapitulating the cellular phenotype of aged cardiovascular tissue in patients could greatly improve our understanding of the roles of aging in CVDs. During aging, the cells in the heart develop a senescent phenotype characterized by telomere damage, mitochondrial dysfunction, cardiomyocyte pathological hypertrophy, and fibrosis[9-11]. Therefore, engineered cardiovascular tissues have been generated to reproduce these phenotypes in vitro [Table 1]. For example, an in vitro aged ECT model shows major cellular features of aging and stiffness resembling the aged human heart after prolonged culture[8]. However, prolonged in vitro culture is time-consuming and costly, which largely hinders the application of this model. Therefore, factors that promote aging could be crucial to accelerate aging in vitro [Figure 1].
Figure 1. Aging in engineered cardiovascular tissues. Dox: Doxorubicin; HGPS: hutchinson-gilford progeria syndrome; hCB-EPC: human cord blood-derived endothelial progenitor cells; iPSC: induced pluripotent stem cell; iCM: iPSC-derived cardiomyocyte; iSMC: iPSC-derived smooth muscle cells; NRCM: neonatal rat cardiomyocyte; ROS: reactive oxygen species; TEBV: tissue-engineered blood vessels.
Features of aging in engineered cardiovascular tissues
Aging features | Tissue types | Strategies accelerating aging | Aging indicators | Reference |
Cellular senescence | TEBVs | Oxidative stress | Increased p21 levels and reduced NOS3 expression | [19] |
Cellular senescence and impaired stress response | In vitro aged myocardial tissue | Prolonged culture and enhanced cellular stiffness | P21 staining, β-galactosidase staining | [8] |
Cellular senescence | Cardiac tissue chip model | Acute low-dose doxorubicin treatment | DNA damage response foci, and increased expression of cell cycle inhibitor p16INK4a, p53, and ROS | [7] |
Loss and dysfunction of SMCs in the vasculature | Tissue-engineered blood vessel | Induced pluripotent stem cell (iPSC)-derived SMCs from an HGPS patient | Reduced vasoactivity, increased medial wall thickness, increased calcification and apoptosis | [13] |
Cellular senescence and impaired cardiomyocyte function | Tissue-engineered 3D co-culture system | Aging cardiac fibroblasts | Slower action potential conduction, prolonged action potential duration, weaker contractions, higher tissue stiffness, and reduced calcium transient amplitude | [63] |
Accelerated aging and death | Tissue-engineered blood vessels | Induced pluripotent stem cell (iPSC)-derived SMCs and ECS from an HGPS patient | Reduction in both vasoconstriction and vasodilation | [14] |
A recent study identified SIRT2 as a significantly decreased protein in the hearts of aged monkeys[10]. Interestingly, SIRT2 deficiency leads to senescence features in human induced pluripotent stem cell (iPSC)-derived cardiomyocytes[10], suggesting the possibility of accelerated heart aging in vitro via gene engineering. Hutchinson-Gilford Progeria Syndrome (HGPS) is a genetic disease associated with pro-senescence characteristics. Consequently, other genetically accelerated aging blood vessel models were fabricated using vascular cells derived from HGPS patients’ iPSCs[12-14]. These tissue-engineered blood vessel (TEBV) models exhibited features of vascular aging, including excessive extracellular matrix (ECM) deposition and occurrence of inflammation. However, this model requires iPS cell resources from HGPS patients, which are rare and hinder the application of this model. HGPS is caused by a mutation in the LMNA gene, which encodes the nuclear envelope protein lamin A. This mutation results in the production of a shorter transcript known as progerin[15]. Aberrant accumulation of progerin in the nuclear membrane interferes with DNA damage response, gene transcription, and telomere maintenance[16]. Researchers demonstrated that forced progerin expression could induce aging-related features in iPSC-derived neurons [15], expanding the application possibilities of this in vitro aging model.
Other factors promoting aging include oxidative stress and telomere damage. The connection between oxidative stress and aging has been well established[17]. Studies have revealed that oxidative stress can induce senescence and expression of inflammatory markers in the cardiovascular system of aged patients[18]. Therefore, researchers seek to generate a vascular aging model via oxidative stress stimulation in TEBV[19]. Exposure to H2O2 can significantly increase senescence in endothelial cells and other cell types. Interestingly, compared with other cell types, the aging effect on TEBV is dominated by the endothelium[19]. Accumulating evidence suggests cardiomyocytes develop senescence upon doxorubicin treatment[20,21]. Doxorubicin-induced aging was also achieved in a cardiac tissue chip model through acute low-dose doxorubicin treatment[7], with aging features including enhanced DNA damage response, increased p53 expression and reactive oxygen species (ROS). Recent studies show that telomere damage can activate senescence-associated pathways in cardiomyocytes and lead to pathological fibrosis and hypertrophy[9]. Since this telomere damage can be induced by mitochondrial dysfunction[11,22], reagents causing mitochondrial dysfunction may be used to generate aging models via induced telomere damage.
In summary, to better understand the molecular differences between aged and young cardiovascular tissues, it is required to construct age-appropriate ECT. However, existing aged ECT relies on prolonged culture and is time-consuming. Therefore, the ways to accelerate the aging process in vitro are the key to improvement. Some genetic manipulations, which lead to SIRT2 deficiency and abnormal accumulation of progerin, were proved to be able to accelerate aging. These findings provide a theoretical basis for constructing an ECT model of accelerated aging by genetic manipulations. In addition to intrinsic factors, some extrinsic factors, such as oxidative stress, have also been shown to accelerate the aging process. Based on these findings, a more economical in vitro aging model could be developed through genetic manipulation or external stimulation. It will provide a favorable model for further understanding the molecular characteristics of aging and exploring new therapeutic targets.
Rejuvenation of ECT: lessons from cardiovascular tissues
To rejuvenate engineered cardiovascular tissues, we need to gain insights from the aging and rejuvenation of cardiovascular tissues [Table 2]. Extracellular matrix proteins enriched in young cardiac ECM have been shown to promote heart regeneration[23,24]. A recent study identified a novel ECM molecule, versican, which can induce cardiac regeneration[6]. Since ECM proteins can be delivered as recombinant proteins or modified RNA, they may serve as very suitable rejuvenating targets for cardiac tissue. Endothelium-targeted Sirt7 gene therapy ameliorates aging features in a mouse model of HGPS[25], suggesting that Sirt7 is a potential target for reversing age-related vascular dysfunction. Restoring mitochondrial function through the inhibition of DYRK1B activity has also been shown to rejuvenate mitochondrial bioenergetics and cardiac performance[26] [Figure 2].
Rejuvenation in cardiovascular tissues
Rejuvenation features | Tissue types | Rejuvenation strategies | Potential target | Reference |
Enhanced cardiomyocyte proliferation, reduced fibrosis, and improved cardiac function | Myocardium | Intramyocardial injection of versican | Integrin β1, ERK1/2 and Akt | [6] |
Improves neovascularization and extends life span | Vascular endothelium | Vascular endothelium-targeted Sirt7 gene therapy | Sirt7 | [25] |
Improve in vitro and in vivo vasculogenesis | Vascular endothelium | Conjugation of drug-loaded liposomal nanoparticles directly to the surface of GDM-exposed ECFCs | N/A | [27] |
β-galactosidase, p53, p21, and p16 were remarkably reduced | Myocardium | Exosomes derived from human umbilical cord MSCs | Apaf1 | [28] |
Modulated the aging-related p16 gene and enhanced cell proliferation | Myocardium | Covalently immobilized 2 proangiogenic cytokines (vascular endothelial growth factor and basic fibroblast growth factor) onto porous collagen scaffolds | N/A | [30] |
Rejuvenating mitochondrial bioenergetics | Myocardium | Inhibition of DYRK1B or STAT3 activity using specific inhibitors | PGC-1α | [26] |
Rejuvenating cardiac gene expression, heart function, exercise capacity, and systemic biomarkers | Myocardium | CDC transplantation | N/A | [29] |
Moreover, a recent study reported a novel strategy to rejuvenate vascular progenitor cells via TGF-β inhibitor-loaded liposomal nanoparticles[27], suggesting the therapeutic potential of drug-loaded nanoparticles. Another study shows that young mesenchymal stem cells (MSC)-derived exosomes can revitalize aged MSCs and promote their cardiac repair function via miR-136/Apaf1 axis[28], indicating the protective effect of beneficial factor-loaded exosomes. In another study, human heart cells from older donors were treated with exosomes secreted by cardiosphere-derived cells (CDCs) from young donors[29]. Results show that young-CDC-secreted exosomes could reverse senescent cellular features in aged human heart cells in vitro. Growth factors, such as vascular endothelial growth factor (VEGF) and basic fibroblast growth factor (bFGF), have also been reported to rejuvenate engineering tissues constructed from MSCs of aged donors[30] [Figure 2].
Figure 2. Rejuvenation of cardiovascular tissues. CDC: Cardiosphere-derived cell; GDM: gestational diabetes mellitus; MI: myocardial infarction; MSC: mesenchymal stem cell; ECFC: endothelial colony-forming cells.
Strategies targeting senescent cells are also of great importance in cardiovascular rejuvenation. To induce cytotoxicity specifically in senescent cells, two approaches are commonly applied: genetic methods and immunological methods. The genetic method introduces cellular suicide signals driven by the p16 or p21 promoter[31,32], while the immunological method uses immune cells and antibodies to recognize and eliminate senescent cells[33,34]. Inducible elimination of senescent cells was achieved in mice expressing caspase 8 (a suicide signal activated by a drug named AP20187) in p16INK4a-positive senescent cells[35]. Moreover, precise elimination of type-specific aging cells could be achieved using cell type-specific Cre mice[36]. In another mouse model, herpes simplex virus thymidine kinase (HSV-TK) expression was driven by the p16INK4A promoter, and specific elimination of p16INK4A positive cells was achieved by the antiviral drug Ganciclovir[37]. These genetic clearance strategies of senescent cells suggest that the delivery of inducible suicide construct driven by the p16 or p21 promoter using adeno-associated virus (AAV) may be a possible approach for selective clearance of aging cells in CVD patients. Combining this with tissue-specific Cre, cell type-selective elimination of aging cells will generate novel insights into whether the removal of aging non-cardiomyocytes contributes to the improvement of heart function.
Alternatively, the immunological method usually uses chimeric antigen receptor (CAR) T cells and specific antibodies. CAR T cells are genetically engineered to target cells with specific antigens. For example, urokinase-type plasminogen activator receptor (uPAR) is used as a cell surface marker to eliminate senescent cells[33]. The results show that the removal of aging cells via uPAR CAR T cells can reduce liver fibrosis[33]. Vaccination against senescent antigens has also been proven to be an effective strategy. Glycoprotein nonmetastatic melanoma protein B (GPNMB) is enriched in senescent vascular endothelial cells[34]. Vaccination of mice against GPNMB can reduce GPNMB-positive cells and extend the lifespan of progeroid mice[34]. It is expected that more novel gene delivery and immunological drugs targeting senescent cells will be developed and tested on age-matched in vitro models for preclinical investigation.
Some molecules such as SIRT7 and ECM molecules have been explored and proved to have rejuvenating capacity. In addition, some drug-loaded nanoparticles and exosomes have also been confirmed to promote rejuvenation. The removal of senescent cells by genetic or immunological methods may also be a promising treatment that requires further research and development. The development of novel anti-aging therapies through AAV or RNA-mediated molecular therapy, or delivery of drug-loaded nanoparticles and exosomes, as well as senescent cell depletion, could be followed by preclinical validation in age-appropriate animal and ECT models.
ENGINEERED CARDIOVASCULAR TISSUE IN VIVO FOR REJUVENATION: CLINICAL POTENTIAL AND CHALLENGES
Heart failure is the severe stage or terminal stage of various CVDs and is characterized by apoptosis of cardiomyocytes and a decline in heart function. The loss of cardiomyocytes is irreversible due to the declined regeneration capacity of adult myocardium. The only effective way to cure heart failure is transplant therapy, but this strategy is largely hindered by the lack of donor resources and the complexity of the surgery. The development of stem cell technology in recent years is expected to address this problem. The first generation of cardiac regeneration therapy mainly focused on adult stem cells, such as mesenchymal stem cells. These adult stem cells, which can self-renew and differentiate, were transplanted into patient’s hearts to replace damaged cardiomyocytes. Several clinical trials based on this strategy have been conducted in Europe, the United States, and Japan[38]. However, whether transplanted adult stem cells can replace damaged cardiomyocytes remains unclear. Researchers believe that some of the functional improvements observed in these experiments may be related to paracrine effects or immunomodulatory effects of mesenchymal stem cells[39]. With the deepening of research and technological development in regenerative medicine, the second generation of cardiac regenerative therapy focused on multiple strategies, such as cardiomyocytes and ECTs derived from iPSCs. Clinical trials, such as the HEAL-CHF Trial based on hPSC-CMs[40], have been carried out, providing novel insights into regenerative medicine [Table 3]. The possibility of cardiomyocyte transplantation has been proved in rodent[41-43], pig[44], and macaque[45,46] models of CVD. However, a low cell retention rate remains a key challenge of cell injection strategies[47]. Alternatively, the transplantation of ECT results in improved cell retention [48] without the appearance of arrhythmia[49,50]. Therefore, the decline in heart function and heart failure caused by aging may be reversed by the transplantation of engineered cardiovascular tissues.
Clinical trials
No. | Study title | Conditions | Interventions | Sponsor | Collaborator | Sample size | End point | Preliminary result | Reference |
NCT04396899 | Safety and efficacy of induced pluripotent stem cell-derived engineered human myocardium as biological ventricular assist tissue in terminal heart failure (BioVAT-HF) | Heart failure | Biological: EHM implantation | University Medical Center Goettingen | Deutsches Zentrum für Herz-Kreislauf-Forschung (DZHK), University Medical Center Freiburg | 53 | 2024/10/1 | / | / |
NCT04945018 | A study of iPS cell-derived cardiomyocyte spheroids (HS-001) in patients with heart failure (LAPiS study) (LAPiS) | Heart failure/ischemic heart disease | Biological: HS-001 CSDevice: HS-001-D needle, HS-001-D adaptor | Heartseed Inc. | / | 10 | 2026/1/31 | / | / |
NCT02057900 | Transplantation of human embryonic stem cell-derived progenitors in severe heart failure (ESCORT) | Ischemic heart disease | Biological: human embryonic stem cell-derived CD15 + Isl-1 + progenitors | Assistance Publique-Hôpitaux de Paris | / | 6 | 2018/3/22 | None of the patients presented with arrhythmias or tumors during follow-up. All patients were symptomatically improved with an increased systolic motion. One patient died early post surgery from comorbidities. One patient died of heart failure after 22 months | [58] |
UMIN000003273 | Development of new strategy for severe heart failure using autologous myoblast sheets | Ischemic cardiomyopathy, dilated cardiomyopathy | Culture of autologous skeletal myoblasts and engineering myoblast sheets. Transplantation of the myoblast sheets to the heart | Osaka University Graduate School of Medicine | / | 27 | 2018/9/18 | None of the patients presented with procedure-related major complications during follow-up. The majority of the ischemic cardiomyopathy patients showed significant symptomatic improvement | [64,65] |
NCT03763136 | Treating heart failure with hPSC-CMs (HEAL-CHF) | Heart failure | Biological: hPSC-CM therapy | Help Therapeutics | The Affiliated Nanjing Drum Tower Hospital of Nanjing University Medical School | 20 | 2024/10/30 | / | [40] |
NCT05223894 | Treating heart failure with hiPSC-CMs | Heart failure | Biological: hiPSC-CM therapy | Help Therapeutics | Shanghai East Hospital | 20 | 2025/12/30 | / | / |
NCT05566600 | Allogeneic iPSC-derived cardiomyocyte therapy in patients with worsening ischemic heart failure | Ischemic heart failure, chronic heart failure | Biological: human (allogeneic) iPS-cell-derived cardiomyocyte | Help Therapeutics | Central South University | 32 | 2025/7/31 | / | / |
NCT04982081 | Treating congestive HF with hiPSC-CMs through endocardial injection | Cardiovascular diseases, congestive heart failure, dilated cardiomyopathy | Biological: hiPSC-CM therapy | Help Therapeutics | Xijing Hospital | 20 | 2023/7/31 | / | / |
NCT05647213 | Autologous induced pluripotent stem cells of cardiac lineage for congenital heart disease | Univentricular heart, congenital heart disease, heart failure, NYHA class III heart failure, NYHA Class IV | Biological: iPSC-CL | HeartWorks, Inc. | / | 50 | 2029/2/1 | / | / |
For effective clinical translation, experience could be gained from preclinical studies. The retention and function of ECT engraftment have been demonstrated in rodent transplantation experiments[50,51], and electromechanical integration has been shown in host and graft tissues[52,53]. The electromechanical synchronization of transplanted ECT has also been demonstrated in vivo[50,51,54,55]. However, some reports indicate that the electromechanical synchronization of grafts is variable or absent[41,56]. Therefore, further studies are required to confirm the electromechanical integration of ECT grafts. With the development of ECT transplantation and the initiation of clinical trials[57-59], these outcomes will provide essential information on the safety and efficacy of ECT transplantation.
Considering the increased average life span of our population, there is an urgent demand for state-of-the-art engineered tissue concepts that maintain function throughout life to avoid reoperation[60]. Recent studies show that growth factors can enhance the function of a biodegradable patch seeded with MSCs from old donors, and this functionally enhanced ECT could restore cardiac function after surgery[30]. After transplantation, the old cells were revitalized, suggesting this growth factor-enriched MSC patch system serves as a rejuvenating and repairing platform for aged patients with heart failure. Tissue-engineered vascular grafts (TEVGs) with the ability to regenerate may overcome the growth barriers of non-living artificial tissues. However, the generation of living TEVGs requires multiple steps of in vitro culture, which may lead to accelerated cell aging and telomere shortening. This may result in adverse effects on the long-term function of the TEVGs in vivo. Interestingly, although TEVGs appear to be older than their native counterparts, their growth ability and long-term function were not compromised[61]. TEVG appears to rejuvenate due to endogenous cellular renewal, but the molecular mechanism remains unclear. A deeper understanding of the molecular mechanisms of TEVG rejuvenation in vivo could facilitate the development of renewable engineered cardiovascular tissues.
Although the technical development of engineered cardiovascular tissue has advanced the recovery of CVDs in the aging population, many limitations hinder the clinical application of this technology. For example, the immunogenicity of allogeneic cell transplantation can trigger a host immune response, necessitating patients to receive immunosuppressive drugs[40]. Patient-specific iPSC-derived cardiac cells may be a safer resource for autologous transplantation; however, this strategy requires establishing an iPS cell line for each patient, which is time-consuming and costly. Additionally, clinical applications require a large number of cells (approximately 108) and larger sizes of ECT, presenting further challenges to cell culture and ECT construction systems. A low retention rate is a major challenge of cell transplantation. Recent studies demonstrate enhanced cell retention through tissue engineering[62]. However, some researchers have observed a lack of electrical integration of tissue-engineered patches in the host[41], which may lead to arrhythmia in patients after transplantation. Furthermore, the transplantation of tissue-engineered patches requires thoracotomy, potentially prolonging the recovery process of patients. Therefore, the development of tissue-engineered patches suitable for minimally invasive surgery will significantly promote the clinical application of tissue engineering for cardiovascular rejuvenation and repair.
CONCLUSIONS
The development of age-matched ECT is still in its infancy, with a lack of ready-to-use models. Existing prolonged-culture models are time-consuming and costly. Therefore, it is necessary to accelerate aging through genetic or non-genetic approaches for model construction. Once a new model is established, a comprehensive assessment of the aging features using various measurements is needed. For assessing the rejuvenating efficiency of drugs, insights can be obtained from aged animal models due to the limited knowledge of the assessment of regenerative efficiency on aged ECT models. Young ECM and enhanced mitochondrial function have been demonstrated to play essential roles in rejuvenation and may provide novel drug targets for regenerative therapy. Additionally, drug-loaded nanoparticles and exosomes loaded with miRNAs have also shown rejuvenating effects in cellular models in vitro.
The development of next-generation ECT for transplantation holds significant applicational value. Genetically engineered ECT with improved regenerative ability could enhance ECT function and service life after transplantation. Electrical coupling of ECT with host tissue is crucial to avoid potential arrhythmia. Moreover, developing ECT suitable for minimally invasive surgery is a valuable research direction. In summary, with the advancement of ECT construction, insights from preclinical studies and clinical trials will largely inspire basic and translational research. Optimized and combined therapeutic approaches will be developed to effectively respond to age-matched strategies for CVD treatment and heart rejuvenation.
DECLARATIONS
Authors’ contributions
Participated in research design: Xiao J, Li G, Cretoiu D, Liu C, Hao T
Designed the review and wrote the manuscript: Xiao J, Li G, Cretoiu D, Liu C, Hao T
Collected the literature: Guo J, Zhang Y, and Hu M
Availability of data and materials
Not applicable.
Financial support and sponsorship
This work was supported by grants from the National Natural Science Foundation of China (82020108002 and 82225005 to Xiao J, 82370285 to Liu C), grants from the Science and Technology Commission of Shanghai Municipality (23410750100, 20DZ2255400, and 21XD1421300 to Xiao J), and the “Dawn” Program of Shanghai Education Commission (19SG34 to Xiao J).
Conflicts of interest
All authors declared that there are no conflicts of interest.
Ethical approval and consent to participate
Not applicable.
Consent for publication
Not applicable.
Copyright
© The Author(s) 2024.
REFERENCES
1. Ungvari Z, Kaley G, de Cabo R, Sonntag WE, Csiszar A. Mechanisms of vascular aging: new perspectives. J Gerontol A Biol Sci Med Sci 2010;65:1028-41.
2. Peiyong Z, Junichi S. Cardiomyocyte senescence and the potential therapeutic role of senolytics in the heart. J Cardiovasc Aging 2024;4:18.
3. López-Otín C, Blasco MA, Partridge L, Serrano M, Kroemer G. Hallmarks of aging: An expanding universe. Cell 2023;186:243-78.
4. Bergmann O, Zdunek S, Felker A, et al. Dynamics of cell generation and turnover in the human heart. Cell 2015;161:1566-75.
5. Ozcebe SG, Zorlutuna P. In need of age-appropriate cardiac models: impact of cell age on extracellular matrix therapy outcomes. Aging Cell 2023;22:e13966.
6. Feng J, Li Y, Li Y, et al. Versican promotes cardiomyocyte proliferation and cardiac repair. Circulation 2024;149:1004-15.
7. Budhathoki S, Graham C, Sethu P, Kannappan R. Engineered aging cardiac tissue chip model for studying cardiovascular disease. Cells Tissues Organs 2022;211:348-59.
8. Acun A, Nguyen TD, Zorlutuna P. In vitro aged, hiPSC-origin engineered heart tissue models with age-dependent functional deterioration to study myocardial infarction. Acta Biomater 2019;94:372-91.
9. Anderson R, Lagnado A, Maggiorani D, et al. Length-independent telomere damage drives post-mitotic cardiomyocyte senescence. EMBO J 2019;38:e100492.
10. Ye Y, Yang K, Liu H, et al. SIRT2 counteracts primate cardiac aging via deacetylation of STAT3 that silences CDKN2B. Nat Aging 2023;3:1269-87.
11. Mone P, Agyapong ED, Morciano G, et al. Dysfunctional mitochondria elicit bioenergetic decline in the aged heart. J Cardiovasc Aging 4 2024:13.
12. Abutaleb NO, Atchison L, Choi L, et al. Lonafarnib and everolimus reduce pathology in iPSC-derived tissue engineered blood vessel model of Hutchinson-Gilford Progeria Syndrome. Sci Rep 2023;13:5032.
13. Atchison L, Zhang H, Cao K, Truskey GA. A tissue engineered blood vessel model of hutchinson-gilford progeria syndrome using human iPSC-derived smooth muscle cells. Sci Rep 2017;7:8168.
14. Atchison L, Abutaleb NO, Snyder-Mounts E, et al. iPSC-derived endothelial cells affect vascular function in a tissue-engineered blood vessel model of hutchinson-gilford progeria syndrome. Stem Cell Reports 2020;14:325-37.
15. Miller JD, Ganat YM, Kishinevsky S, et al. Human iPSC-based modeling of late-onset disease via progerin-induced aging. Cell Stem Cell 2013;13:691-705.
16. Dechat T, Pfleghaar K, Sengupta K, et al. Nuclear lamins: major factors in the structural organization and function of the nucleus and chromatin. Genes Dev 2008;22:832-53.
17. Dogacan Y, William TP. From vitality to vulnerability: the impact of oxygen on cardiac function and regeneration. J Cardiovasc Aging 2024;4:15.
18. Ungvari Z, Tarantini S, Kiss T, et al. Endothelial dysfunction and angiogenesis impairment in the ageing vasculature. Nat Rev Cardiol 2018;15:555-65.
19. Salmon EE, Breithaupt JJ, Truskey GA. Application of oxidative stress to a tissue-engineered vascular aging model induces endothelial cell senescence and activation. Cells 2020;9:1292.
20. Spallarossa P, Altieri P, Aloi C, et al. Doxorubicin induces senescence or apoptosis in rat neonatal cardiomyocytes by regulating the expression levels of the telomere binding factors 1 and 2. Am J Physiol Heart Circ Physiol 2009;297:H2169-81.
21. Maejima Y, Adachi S, Ito H, Hirao K, Isobe M. Induction of premature senescence in cardiomyocytes by doxorubicin as a novel mechanism of myocardial damage. Aging Cell 2008;7:125-36.
22. Liu C, Zhang X, Hu M, et al. Metabolic targets in cardiac aging and rejuvenation. J Cardiovasc Aging 2022;2:46.
23. Bassat E, Tzahor E. How can young extracellular matrix promote cardiac regeneration? Circulation 2024;149:1016-8.
24. Tzahor E, Dimmeler S. A coalition to heal-the impact of the cardiac microenvironment. Science 2022;377:eabm4443.
25. Sun S, Qin W, Tang X, et al. Vascular endothelium-targeted Sirt7 gene therapy rejuvenates blood vessels and extends life span in a Hutchinson-Gilford progeria model. Sci Adv 2020;6:eaay5556.
26. Zhuang L, Jia K, Chen C, et al. DYRK1B-STAT3 Drives cardiac hypertrophy and heart failure by impairing mitochondrial bioenergetics. Circulation 2022;145:829-46.
27. Bui L, Edwards S, Hall E, et al. Engineering bioactive nanoparticles to rejuvenate vascular progenitor cells. Commun Biol 2022;5:635.
28. Zhang N, Zhu J, Ma Q, et al. Exosomes derived from human umbilical cord MSCs rejuvenate aged MSCs and enhance their functions for myocardial repair. Stem Cell Res Ther 2020;11:273.
29. Grigorian-Shamagian L, Liu W, Fereydooni S, et al. Cardiac and systemic rejuvenation after cardiosphere-derived cell therapy in senescent rats. Eur Heart J 2017;38:2957-67.
30. Kang K, Sun L, Xiao Y, et al. Aged human cells rejuvenated by cytokine enhancement of biomaterials for surgical ventricular restoration. J Am Coll Cardiol 2012;60:2237-49.
31. Omori S, Wang TW, Johmura Y, et al. Generation of a p16 reporter mouse and its use to characterize and target p16(high) cells in vivo. Cell Metab 2020;32:814-28.
32. Wang B, Wang L, Gasek NS, et al. An inducible p21-Cre mouse model to monitor and manipulate p21-highly-expressing senescent cells in vivo. Nat Aging 2021;1:962-73.
33. Amor C, Feucht J, Leibold J, et al. Senolytic CAR T cells reverse senescence-associated pathologies. Nature 2020;583:127-32.
34. Suda M, Shimizu I, Katsuumi G, et al. Senolytic vaccination improves normal and pathological age-related phenotypes and increases lifespan in progeroid mice. Nat Aging 2021;1:1117-26.
35. Baker DJ, Wijshake T, Tchkonia T, et al. Clearance of p16Ink4a-positive senescent cells delays ageing-associated disorders. Nature 2011;479:232-6.
36. Farr JN, Saul D, Doolittle ML, et al. Local senolysis in aged mice only partially replicates the benefits of systemic senolysis. J Clin Invest 2023;133:e162519.
37. Demaria M, Ohtani N, Youssef SA, et al. An essential role for senescent cells in optimal wound healing through secretion of PDGF-AA. Dev Cell 2014;31:722-33.
38. Ward MR, Abadeh A, Connelly KA. Concise review: rational use of mesenchymal stem cells in the treatment of ischemic heart disease. Stem Cells Transl Med 2018;7:543-50.
39. Zhang K, Cheng K. Stem cell-derived exosome versus stem cell therapy. Nat Rev Bioeng 2023;12:1-2.
40. Zhang H, Xue Y, Pan T, et al. Epicardial injection of allogeneic human-induced-pluripotent stem cell-derived cardiomyocytes in patients with advanced heart failure: protocol for a phase I/IIa dose-escalation clinical trial. BMJ Open 2022;12:e056264.
41. Gerbin KA, Yang X, Murry CE, Coulombe KL. Enhanced electrical integration of engineered human myocardium via intramyocardial versus epicardial delivery in infarcted rat hearts. PLoS One 2015;10:e0131446.
42. Zhao X, Chen H, Xiao D, et al. Comparison of non-human primate versus human induced pluripotent stem cell-derived cardiomyocytes for treatment of myocardial infarction. Stem Cell Reports 2018;10:422-35.
43. Shiba Y, Fernandes S, Zhu WZ, et al. Human ES-cell-derived cardiomyocytes electrically couple and suppress arrhythmias in injured hearts. Nature 2012;489:322-5.
44. Romagnuolo R, Masoudpour H, Porta-Sánchez A, et al. Human embryonic stem cell-derived cardiomyocytes regenerate the infarcted pig heart but induce ventricular tachyarrhythmias. Stem Cell Reports 2019;12:967-81.
45. Chong JJ, Yang X, Don CW, et al. Human embryonic-stem-cell-derived cardiomyocytes regenerate non-human primate hearts. Nature 2014;510:273-7.
46. Liu YW, Chen B, Yang X, et al. Human embryonic stem cell-derived cardiomyocytes restore function in infarcted hearts of non-human primates. Nat Biotechnol 2018;36:597-605.
47. Nguyen PK, Neofytou E, Rhee JW, Wu JC. Potential strategies to address the major clinical barriers facing stem cell regenerative therapy for cardiovascular disease: a review. JAMA Cardiol 2016;1:953-62.
48. Sekine H, Shimizu T, Dobashi I, et al. Cardiac cell sheet transplantation improves damaged heart function via superior cell survival in comparison with dissociated cell injection. Tissue Eng Part A 2011;17:2973-80.
49. Riegler J, Tiburcy M, Ebert A, et al. Human engineered heart muscles engraft and survive long term in a rodent myocardial infarction model. Circ Res 2015;117:720-30.
50. Zimmermann WH, Melnychenko I, Wasmeier G, et al. Engineered heart tissue grafts improve systolic and diastolic function in infarcted rat hearts. Nat Med 2006;12:452-8.
51. Weinberger F, Breckwoldt K, Pecha S, et al. Cardiac repair in guinea pigs with human engineered heart tissue from induced pluripotent stem cells. Sci Transl Med 2016;8:363ra148.
52. Didié M, Christalla P, Rubart M, et al. Parthenogenetic stem cells for tissue-engineered heart repair. J Clin Invest 2013;123:1285-98.
53. Rubart M, Pasumarthi KB, Nakajima H, Soonpaa MH, Nakajima HO, Field LJ. Physiological coupling of donor and host cardiomyocytes after cellular transplantation. Circ Res 2003;92:1217-24.
54. Iseoka H, Miyagawa S, Fukushima S, et al. Pivotal role of non-cardiomyocytes in electromechanical and therapeutic potential of induced pluripotent stem cell-derived engineered cardiac tissue. Tissue Eng Part A 2018;24:287-300.
55. Li J, Minami I, Shiozaki M, et al. Human pluripotent stem cell-derived cardiac tissue-like constructs for repairing the infarcted myocardium. Stem Cell Reports 2017;9:1546-59.
56. Jackman CP, Ganapathi AM, Asfour H, et al. Engineered cardiac tissue patch maintains structural and electrical properties after epicardial implantation. Biomaterials 2018;159:48-58.
57. Chachques JC, Trainini JC, Lago N, et al. Myocardial assistance by grafting a new bioartificial upgraded myocardium (MAGNUM clinical trial): one year follow-up. Cell Transplant 2007;16:927-34.
58. Menasché P, Vanneaux V, Hagège A, et al. Transplantation of human embryonic stem cell-derived cardiovascular progenitors for severe ischemic left ventricular dysfunction. J Am Coll Cardiol 2018;71:429-38.
59. Cyranoski D. 'Reprogrammed' stem cells approved to mend human hearts for the first time. Nature 2018;557:619-20.
60. Fioretta ES, von Boehmer L, Motta SE, Lintas V, Hoerstrup SP, Emmert MY. Cardiovascular tissue engineering: from basic science to clinical application. Exp Gerontol 2019;117:1-12.
61. Kelm JM, Emmert MY, Zürcher A, et al. Functionality, growth and accelerated aging of tissue engineered living autologous vascular grafts. Biomaterials 2012;33:8277-85.
62. Zimmermann W. Tissue engineered heart repair from preclinical models to first-in-patient studies. Current Opinion in Physiology 2020;14:70-7.
63. Li Y, Asfour H, Bursac N. Age-dependent functional crosstalk between cardiac fibroblasts and cardiomyocytes in a 3D engineered cardiac tissue. Acta Biomater 2017;55:120-30.
64. Domae K, Miyagawa S, Yoshikawa Y, et al. Clinical outcomes of autologous stem cell-patch implantation for patients with heart failure with nonischemic dilated cardiomyopathy. J Am Heart Assoc 2021;10:e008649.
Cite This Article
How to Cite
Download Citation
Export Citation File:
Type of Import
Tips on Downloading Citation
Citation Manager File Format
Type of Import
Direct Import: When the Direct Import option is selected (the default state), a dialogue box will give you the option to Save or Open the downloaded citation data. Choosing Open will either launch your citation manager or give you a choice of applications with which to use the metadata. The Save option saves the file locally for later use.
Indirect Import: When the Indirect Import option is selected, the metadata is displayed and may be copied and pasted as needed.
About This Article
Copyright
Data & Comments
Data
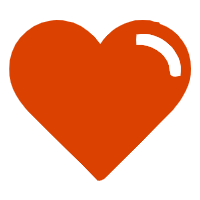
Comments
Comments must be written in English. Spam, offensive content, impersonation, and private information will not be permitted. If any comment is reported and identified as inappropriate content by OAE staff, the comment will be removed without notice. If you have any queries or need any help, please contact us at [email protected].