Rare variants in the FBN1 gene are associated with sporadic dilated cardiomyopathy in a Chinese Han population
Abstract
Introduction: Dilated cardiomyopathy (DCM) represents a diverse set of myocardial diseases characterized by notable genetic heterogeneity. Although over 50 genes have been associated with DCM, these collectively explain 35% of idiopathic DCM cases. Variants in the FBN1 gene encoding fibrillin-1 are primarily linked to connective tissue disorders. Considering the potential of these disorders to impact myocardial tissue, this study probes into the possible association between FBN1 variants and DCM.
Aim: The objective of this study was to investigate the association between FBN1 variants and DCM in a Chinese Han population.
Methods and Results: We performed whole-exome sequencing (WES) to identify rare FBN1 variants among 1,059 DCM cases and 514 controls. Utilizing a case-control strategy and the optimal sequence kernel association test (SKAT-O), we found a significant enrichment of rare deleterious FBN1 variants in DCM patients (19 of 1,059 vs. 0 of 514, PSKAT-O = 7.49E-04). Clinical characteristics analysis indicated a higher occurrence of atrial fibrillation and a higher rate of implantable cardioverter-defibrillator (ICD) implantation among DCM patients carrying FBN1 variants (FBN1+) compared to non-carriers (FBN1-). However, these FBN1 variants did not significantly affect primary endpoints, defined as cardiac mortality or heart transplantation, yet appeared to increase the risk of secondary endpoints, including all-cause mortality or heart failure recurrence.
Conclusion: The findings suggest an association between rare deleterious variants in the FBN1 gene and DCM in a Chinese Han population. Our findings underline the importance of further research to validate these results and elucidate the role of FBN1 in DCM.
Potential Impact of the findings: This research provides fresh insights into the potential role of FBN1 rare variants in DCM, pointing to new directions for future genetic studies and potential therapeutic strategies in DCM management.
Keywords
INTRODUCTION
Dilated cardiomyopathy (DCM) is defined as left ventricular (LV) or biventricular dilatation and systolic dysfunction in the absence of coronary artery disease or abnormal load proportional to the degree of LV injury[1]. DCM is the second-most common cause of heart failure (after coronary artery disease) and the most common indicator for heart transplantation, accounting for 36% of all heart failure cases; the prevalence of DCM in the general population is estimated to be > 0.4%[2,3]. Reports show that approximately 25%-35% of patients with idiopathic DCM have a positive family history, indicative of a major genetic cause[2,4]. Most familial DCMs are inherited in an autosomal dominant pattern. In addition, DCM can be inherited in autosomal recessive, X-linked recessive, and mitochondrial manners[5]. Over 50 genes associated with DCM have been identified[6]. However, the combined genetic contribution of these genes explains about 35% of idiopathic dilated cardiomyopathy cases[7]. This suggests the existence of as-yet-undiscovered genes or mechanisms associated with DCM.
FBN1, the gene that encodes fibrillin-1, a major component of extracellular microfibrils, has been identified as the causal gene for Marfan syndrome (MFS). A total of 1,847 different variants have been reported in the FBN1 mutation database[8]. The “Revised Ghent nosology for the Marfan syndrome” posits that the primary basis for diagnosing MFS is the identification of specific clinical signs, predominantly involving the cardiovascular, musculoskeletal, and ocular systems. Confirmation of a pathogenic FBN1 variant would further substantiate the diagnosis[9]. The primary cardiovascular symptom of MFS, caused by FBN1 variants, is the progressive dilation of the ascending aorta, which can lead to aortic aneurysm or dissection, thus placing patients at a significant risk of cardiovascular mortality[10,11].
While the impact of MFS is most notable on the ascending aorta within the cardiovascular system, there is also evidence suggesting a potential association between FBN1 variants and myocardial dysfunction. Campens et al. (2015) found that mice with FBN1 variants exhibited left ventricular systolic dysfunction[12]. Further ex-vivo studies on the myocardium of these mutant mice suggest a role for microfibrils in influencing the mechanical properties of the myocardium[12]. A recent study by Connor et al., which involved 241 MFS patients without severe valvular disease, revealed evidence of left ventricular (LV) dysfunction in 12% of the participants, suggesting the potential for primary cardiomyopathy in MFS[13].
Given these findings, we employed Whole-exome Sequencing (WES) to scrutinize the variation profile of the FBN1 gene in a cohort of Chinese Han DCM patients, thereby further investigating the potential association between FBN1 variants and DCM.
METHODS
Study population
This study was conducted with the approval of the institutional ethics committee and complied with the International Conference on Harmonization Guidelines for Good Clinical Practice and the Declaration of Helsinki (Ethics Approval Document ID: TJ-C20181101). All participating individuals provided their written informed consent. This investigation is part of an ongoing prospective registry study (NCT03754101) aimed at identifying genetic variants or genes potentially linked with the onset and prognosis of DCM.
A total of 1,059 sporadic DCM patients and 514 ethnically matched controls were recruited from Tongji Hospital, Wuhan, China, between July 2007 and December 2020. The criteria for DCM diagnosis was the presence of left ventricular or biventricular dilatation, characterized by a left ventricular end-diastolic diameter (LVEDD) exceeding 33 mm/m2 in men or 32 mm/m2 in women, coupled with systolic dysfunction, represented by a left ventricular ejection fraction (LVEF) less than 50%[14].
Patients with heart failure due to ischemic heart disease, uncontrolled hypertension, major valvular disease, severe systemic infections, excessive alcohol consumption, insulin-treated diabetes, endocrine disorders such as pheochromocytoma, acromegaly, and thyroid disease, systemic diseases, or previous cancer treatment including radiotherapy were excluded from the study[15].
The control group, despite some individuals with managed hypertension considered as a systemic disease, was free of any specific cardiovascular disease or dilated cardiomyopathy. Detailed characteristics of the participants are presented in [Table 1].
Comparative analysis of baseline clinical characteristics between DCM and control groups, as well as FBN1 variants carriers and non-carriers within DCM patients
Comparison between DCM and control | Comparison between FBN1+ and FBN1- in DCM | |||||
DCM | Control | FBN1+ | FBN1- | |||
(n = 1,059) | (n = 514) | P value | (n = 19) | (n = 1,040) | P value | |
Male (%) | 776 (73.3) | 248 (48.2) | < 0.001 | 12 (63.2) | 764 (73.5) | 0.315 |
Age of onset (years) | 52.04 ± 13.79 | - | - | 54.74 ± 19.57 | 51.99 ± 13.67 | 0.39 |
Age at enrollment (years) | 54.97 ± 14.14 | 60.53 ± 14.18 | < 0.001 | 57.42 ± 18.63 | 54.92 ± 14.05 | 0.445 |
NYHA III/IV (%) | 727 (68.6) | 0 ( 0.0) | < 0.001 | 14 (73.7) | 713 (68.6) | 0.633 |
Smoke (%) | 449 (42.4) | 107 (22.0) | < 0.001 | 5 (26.3) | 444 (42.7) | 0.152 |
Alcohol intake (%) | 138 (15.9) | 78 (16.0) | 0.969 | 0 ( 0.0) | 138 (16.2) | 0.089 |
Non-fatal stroke (%) | 47 ( 4.4) | 7 ( 2.4) | 0.125 | 1 ( 5.3) | 46 ( 4.4) | 0.861 |
Hypertension (%) | 526 (49.7) | 21 ( 4.1) | < 0.001 | 10 (52.6) | 516 (49.6) | 0.794 |
Hyperlipidemia (%) | 106 (10.0) | 12 ( 4.2) | 0.002 | 1 ( 5.3) | 105 (10.1) | 0.487 |
Diabetes mellitus (%) | 176 (16.6) | 1 ( 0.2) | < 0.001 | 4 (21.1) | 172 (16.5) | 0.6 |
Renal insufficiency (%) | 85 ( 9.0) | 12 ( 4.2) | 0.008 | 6 (33.3) | 79 ( 8.5) | < 0.001 |
Body mass index (kg/m2) | 23.88 ± 4.46 | 22.83 ± 3.01 | 0.001 | 24.97 ± 3.36 | 23.84 ± 4.49 | 0.483 |
TC (mmol/L) | 3.90 ± 0.99 | 4.43 ± 0.84 | < 0.001 | 3.89 ± 1.25 | 3.91 ± 0.99 | 0.948 |
TG (mmol/L) | 1.39 ± 0.94 | 1.29 ± 0.77 | 0.073 | 1.40 ± 0.75 | 1.39 ± 0.95 | 0.982 |
HDL-C (mmol/L) | 0.96 ± 0.33 | 1.36 ± 0.34 | < 0.001 | 0.98 ± 0.25 | 0.96 ± 0.33 | 0.855 |
LDL-C (mmol/L) | 2.49 ± 0.82 | 2.46 ± 0.65 | 0.486 | 2.40 ± 1.15 | 2.50 ± 0.82 | 0.668 |
NT-proBNP (pg/mL) | 3,813.00 [1,702.00, 8,915.50] | 66.00 [32.00, 106.75] | < 0.001 | 3,368.00 [1,533.00, 1,0404.00] | 3,817.00 [1,720.00, 8,899.75] | 0.89 |
ALT (U/L) | 26.00 [16.00, 47.00] | 15.00 [11.00, 20.00] | < 0.001 | 30.00 [22.00, 45.50] | 26.00 [16.00, 47.00] | 0.34 |
AST (U/L) | 27.00 [19.00, 41.00] | 20.00 [17.00, 24.00] | < 0.001 | 27.00 [22.00, 34.50] | 27.00 [19.00, 41.50] | 0.895 |
Cr (µmol/L) | 88.00 [73.00, 111.00] | 66.00 [58.00, 77.00] | < 0.001 | 89.00 [73.00, 127.00] | 88.00 [73.00, 111.00] | 0.967 |
Atrial fibrillation (%) | 237 (22.4) | 1 ( 0.4) | < 0.001 | 8 (42.1) | 229 (22.0) | 0.037 |
Non-sustained ventricular tachycardia (%) | 145 (13.7) | 0 ( 0.0) | < 0.001 | 2 (10.5) | 143 (13.8) | 0.685 |
Left bundle branch block (%) | 112 (10.6) | 4 ( 1.4) | < 0.001 | 4 (21.1) | 108 (10.4) | 0.134 |
Any arrhythmia (%) | 450 (42.5) | 5 ( 1.8) | < 0.001 | 12 (63.2) | 438 (42.1) | 0.066 |
IVS (mm) | 9.56 ± 1.42 | 9.13 ± 1.11 | < 0.001 | 9.44 ± 1.34 | 9.56 ± 1.43 | 0.728 |
LVPW (mm) | 9.55 ± 1.34 | 9.00 ± 1.02 | < 0.001 | 9.44 ± 1.04 | 9.55 ± 1.35 | 0.738 |
LVEDD (mm) | 66.64 ± 8.26 | 45.02 ± 3.76 | < 0.001 | 65.42 ± 9.44 | 66.67 ± 8.24 | 0.515 |
LAD (mm) | 45.66 ± 7.90 | 30.62 ± 3.90 | < 0.001 | 46.16 ± 8.08 | 45.65 ± 7.90 | 0.784 |
LVEF (%) | 31.41 ± 10.00 | 65.75 ± 4.96 | < 0.001 | 33.32 ± 8.35 | 31.38 ± 10.03 | 0.403 |
E/A | 1.74 ± 1.54 | 1.03 ± 0.35 | < 0.001 | 1.45 ± 1.00 | 1.74 ± 1.54 | 0.566 |
E/e′ | 22.43 ± 12.48 | 9.45 ± 2.97 | < 0.001 | 24.75 ± 16.80 | 22.49 ± 12.36 | 0.609 |
Aortic root diameter (mm) | 29.60 ± 13.27 | 25.47 ± 3.65 | < 0.001 | 27.88 ± 2.80 | 29.63 ± 13.36 | 0.711 |
Proximal ascending aorta diameter (mm) | 32.64 ± 4.83 | 30.11 ± 3.31 | < 0.001 | 34.58 ± 4.46 | 32.60 ± 4.83 | 0.159 |
Aortic root dilatation (%) | 49 ( 7.4) | 0 ( 0.0) | < 0.001 | 0 ( 0.0) | 49 ( 7.6) | 0.344 |
Proximal ascending aorta dilatation (%) | 189 (30.1) | 12 ( 5.6) | < 0.001 | 6 (42.9) | 183 (29.8) | 0.292 |
Any aorta dilatation (%) | 212 (26.5) | 12 ( 5.0) | < 0.001 | 6 (40.0) | 206 (26.2) | 0.232 |
Pacemaker implantation (%) | 54 ( 5.1) | - | - | 0 ( 0.0) | 54 ( 5.2) | 0.307 |
Implantable cardioverter-defibrillator (%) | 19 ( 1.8) | - | - | 2 (10.5) | 17 ( 1.6) | 0.004 |
Cardiotonic use (%) | 507 (48.1) | - | - | 10 (52.6) | 497 (48.0) | 0.69 |
Diuretic use (%) | 869 (82.4) | - | - | 16 (84.2) | 853 (82.4) | 0.838 |
ACE inhibitor use (%) | 802 (76.0) | - | - | 13 (68.4) | 789 (76.2) | 0.434 |
Beta-blocker use (%) | 555 (52.7) | - | - | 8 (42.1) | 547 (52.9) | 0.353 |
Aldactone use (%) | 814 (77.2) | - | - | 17 (89.5) | 797 (77.0) | 0.199 |
Whole-exome sequencing and variants quality control
Whole-exome sequencing (WES) was conducted on both DCM cases (n = 1,059) and controls (n = 514). Genomic DNA, extracted from peripheral blood leukocytes, was measured for concentration using a Nanodrop 2000 (Thermo Fisher Scientific). We employed the SureSelectXT exon V6 kit (Agilent Technologies) to fragment this DNA into pieces of approximately 300 bp. After adaptor ligation and end repair, these fragments underwent targeted region capture. This captured library was amplified to create clusters suitable for sequencing on a HiseqXten sequencer (Illumina), yielding paired-end reads of 150 bp. Following adapter sequence removal, we aligned the reads to the human reference genome (hg19) using the Burrows-Wheeler algorithm (BWA)[16]. PCR duplicates were marked with Picard, and SAMtools facilitated the classification and management of post-alignment SAM or BAM files[17].
The Genome Analysis Toolkit (GATK) was used for the final steps of base quality score recalibration, indel realignment, and variant calling, all following GATK best-practice guidelines[18]. After a collective call for the study cohort, variants were documented in a variant call format (VCF) employing VCFtools[19]. Any variant with a read depth of less than 20 or a missing rate above 20% across the entire cohort was discarded. The remaining variants were annotated using ANNOVAR[20].
Variants identification and pathogenicity assessment
Rare variants, defined as those with a minor allele frequency (MAF) < 0.001 in East Asian populations according to public databases, became the focus of our study. Out of the 51 genes associated with DCM and classified by the Clinical Genome Resource (ClinGen), we excluded seven due to contentious pathogenic evidence. Thus, our investigation involved the remaining 44 genes (comprising 19 high-evidence genes and 25 low-evidence genes)[7] and the gene FBN1. These 44 genes were incorporated to mitigate potential confounding due to the influence of deleterious variants in known DCM-associated genes during our subsequent analyses.
In the FBN1 gene, missense and truncating variants classified as pathogenic or likely pathogenic (P/LP) by the American College of Medical Genetics and Genomics (ACMG) guidelines were included in subsequent analyses[21]. For missense variants deemed variants of uncertain significance (VUS) by ACMG, the deleteriousness prediction was made using REVEL and VEST3[22,23], the most precise in silico functional prediction methods[24]. The thresholds for a deleterious prediction were a REVEL score ≥ 0.4 and a VEST3 score ≥ 0.5, based on a previous evaluation study of missense variant prediction tools[24].For cross-validation, we utilized CADD and MutationTaster, with a CADD-phred-like score > 20 and a “D” prediction from MutationTaster, indicating a deleterious prediction[25,26].
In our analysis, FBN1 variants were deemed deleterious either when they were predicted to be so by in silico tools or were classified as P/LP by the ACMG. These rare deleterious FBN1 variants were included in the gene-based association test [Figure 1].
Figure 1. Flowchart illustrating the screening criteria for identifying rare deleterious variants in FBN1 and other known DCM-associated genes. DCM: Dilated cardiomyopathy; WES: whole-exome sequencing; BWA: burrows-Wheeler algorithm; GATK: genome analysis toolkit; MAF: minor allele frequency; EAS: East Asian; ACMG: American College of Medical Genetics and Genomics; VUS: variants of uncertain significance; REVEL: rare exome variant ensemble learner; VEST3: variant effect scoring tool version 3; CADD: combined annotation dependent depletion; TTNtv: TTN truncating variants; PSI: percentage spliced in.
Among the 43 selected DCM-associated genes, variants were deemed deleterious if they were classified as
Gene-based association test
Utilizing the optimal sequence kernel association test (SKAT-O)-a nuanced synthesis of unidirectional and variance-component tests-we evaluated the association of rare deleterious FBN1 variants with DCM risk[32]. A P < 0.05 was set as the threshold for statistical significance.
Follow-up and clinical outcomes
Clinical endpoint events were adjudicated by two independent clinicians who were blinded to imaging and genetic data. Primary endpoints were defined as cardiac mortality (verified by official death certificates or relative interviews) or heart transplantation (validated via medical records). Secondary endpoints included all-cause mortality or heart failure recurrence, either in-hospital or post-discharge.
Statistical analysis
Baseline characteristics were represented through suitable descriptive statistics. For normally distributed continuous variables, we reported the mean ± standard deviation (SD), while for non-normally distributed variables, we reported the median with interquartile range [IQR]. Categorical variables were expressed as counts (percentages). Depending on the data distribution, we used independent samples t-tests or Wilcoxon’s tests for continuous variables, and chi-squared or Fisher’s exact tests for categorical ones.
We estimated survival rates via Kaplan-Meier methods, comparing groups with the log-rank test. Cox proportional hazards models assessed the influence of various clinical characteristics on outcome likelihood. All tests were two-tailed with a P less than 0.05 indicating statistical significance. All analyses and data visualizations were performed using R (version 3.6.2).
RESULTS
Profile of the rare variants in the FBN1 gene
In our WES analysis of 1,059 DCM cases and 514 controls, 47 rare FBN1 variants were identified in 53 cases and 9 controls [Supplementary Table 1]. Upon applying the ACMG guidelines, we excluded two likely benign variants, leaving us with 44 VUS missense variants and one likely pathogenic frameshift insertion (p.S932fs). After assessing deleteriousness, we classified 17 of these missense variants as deleterious
Figure 2. Distribution of rare deleterious variants in FBN1. Schematic representation of FBN1 protein domains and the identified variant sites in patients. Frameshift insertion variants are shown in purple, and missense variants are shown in green. Domains include TB (TB domain), cEGF (Complement Clr-like EGF-like domain), vWFA (von Willebrand factor type A domain), and EGF_CA (Calcium-binding EGF domain). The X-axis represents the length of the FBN1 protein, and the Y-axis represents the number of individuals carrying specific variants.
Gene-based association test
To mitigate confounding effects, we excluded individuals carrying pathogenic or likely pathogenic variants, known as P/LP variants, in the 44 known DCM-associated genes. The details of these genes are provided in [Supplementary Table 4]. Among 215 P/LP variants identified in these genes, 197 were in 208 DCM patients and 21 in 22 controls. [Supplementary Table 5]. Within the 208 DCM patients, one also carried a deleterious FBN1 variant and was thus excluded from the subsequent association tests. This exclusion strategy ultimately led to a cohort consisting of 851 DCM patients (including 18 carrying rare deleterious FBN1 variants) and 492 controls for the FBN1 gene-based association test. Using the sequence kernel association test-optimal (SKAT-O), we found a significant enrichment of deleterious FBN1 variant carriers in the DCM cohort (18 of 851 vs. 0 of 492, PSKAT-O = 7.49E-04), even when we adjusted for sex and age at enrollment.
Comparative analysis of clinical characteristics in DCM and controls
To better understand the associations between the FBN1 genotype and DCM phenotype, as well as the differences between DCM patients and controls, we conducted a comprehensive analysis of various clinical characteristics [Table 1]. This analysis not only compared DCM patients to controls but also differentiated DCM patients based on the presence or absence of rare deleterious FBN1 variants.
When comparing the overall DCM group to the control group, we noted several significant differences in clinical features. DCM patients were primarily male (73.3% vs. 48.2%, P < 0.001) and were younger at the time of enrollment (54.97 ± 14.14 vs. 60.53 ± 14.18 years, P < 0.001). Moreover, these patients exhibited a significantly higher proportion of NYHA Class III/IV status (68.6% vs. 0%, P < 0.001), indicating severe impairment of cardiac function, which was further supported by echocardiographic data. Specifically, DCM patients had larger left ventricular end-diastolic diameters (LVEDD: 66.64 ± 8.26 vs. 45.02 ± 3.76 mm,
Within the DCM group, no significant differences were observed in enrollment age, onset age, or sex between FBN1 variant carriers (FBN1+) and non-carriers (FBN1-). However, the occurrence of atrial fibrillation was significantly higher in FBN1+ patients (42.1% vs. 22.0%, P = 0.037). This group also had a higher rate of implantable cardioverter-defibrillator (ICD) implantation (10.5% vs. 1.6%, P = 0.004), possibly due to their increased susceptibility to atrial fibrillation. The incidence of “any arrhythmia,” which is a collective measure of atrial fibrillation, non-sustained ventricular tachycardia (NSVT), and left bundle branch block (LBBB), did not differ significantly between the two groups (63.2% vs. 42.1%, P = 0.066). Moreover, renal insufficiency was more prevalent in FBN1+ patients (33.3% vs. 8.5%, P < 0.001), suggesting a potential link between FBN1 variants and renal impairment, a hypothesis that would require more robust evidence to validate.
Interestingly, no significant differences in echocardiographic parameters, including LVEF, LVEDD, and left atrial diameter (LAD), were found between FBN1+ and FBN1- DCM patients. This indicates that FBN1 variants may not significantly influence these specific cardiac structural and functional parameters. Detailed statistics and additional findings can be found in [Table 1].
Continuing our exploration into the influence of the FBN1 genotype on DCM phenotype, we conducted a more granular stratification of DCM patients into three distinct groups for a detailed baseline comparison: (1) those carrying deleterious variants in FBN1 (FBN1+); (2) those without FBN1 variants but carrying variants in other known DCM-associated genes (FBN1-/DCMGenes+); and (3) those without deleterious variants in either FBN1 or any other known DCM-associated genes (FBN1-/DCMGenes-). Relative to the FBN1-/DCMGenes+ group, the FBN1+ group demonstrated a heightened prevalence of atrial fibrillation (42.1% vs. 20.5%, P = 0.03), left bundle branch block (
FBN1 is known to be associated with MFS, which primarily manifests as dilation of the ascending aorta. We further examined the aortic diameters in DCM patients carrying FBN1 variants. After adjusting for enrollment age and body surface area, the aortic root diameter and proximal ascending aorta diameter showed no significant disparities between FBN1 variant carriers and non-carriers (P = 0.711 and P = 0.159, respectively). Moreover, rates of the aortic root and proximal ascending aorta dilatations, conditions defined by exceeding the upper reference limits (adjusted for age and body surface area), did not differ significantly
Remarkably, none of the DCM patients with rare deleterious FBN1 variants in our cohort were diagnosed with MFS during our follow-up. Instead, cardiac dilatation served as their primary cardiovascular phenotype, deviating from the severe aortic dilatation typical of MFS. Considering patients’ medical histories, it appears that FBN1 rare deleterious variants in our DCM cohort may not result in typical MFS phenotypes, underscoring the need for more research on FBN1 genotype-phenotype correlations in cardiovascular diseases.
Clinical outcomes
We sought to discern the potential impact of FBN1 variants on the prognosis of DCM patients, utilizing sophisticated statistical methodologies including Kaplan-Meier survival analysis and Cox proportional hazards model analysis. A total of 1,056 (99.7%) participants accepted the final evaluation. The mean follow-up time was 47.84 ± 24.73 months. Upon comparing DCM patients with and without deleterious FBN1 variants [Figure 3], we found no significant difference in the risk of primary endpoints
Figure 3. Clinical outcomes in DCM patients with and without rare deleterious variants in FBN1. (A) Kaplan-Meier curve illustrates survival free of primary endpoints, which include cardiac mortality or heart transplantation. (B) The curve represents survival free of secondary endpoints, comprising all-cause mortality or heart failure recurrence. Probability values were calculated using the log-rank test. FBN1+: DCM cases carrying rare deleterious variants in FBN1; FBN1-: DCM cases not carrying rare deleterious variants in FBN1.
Further comparative analysis among pre-defined groups [Supplementary Figure 1] revealed that the FBN1+ group did not exhibit a significantly different risk for primary endpoints (P = 0.28). However, an elevated risk for secondary endpoints was discerned in this group (P = 0.049).
The above findings indicate that FBN1 variants may not significantly affect the primary clinical endpoints in DCM patients, yet they seem to increase the risk of secondary endpoints. These observations underscore the need for further investigation into the specific influence of FBN1 variants on the clinical trajectory and outcome of DCM.
DISCUSSION
Drawing upon existing research, our study significantly enhances our understanding of FBN1’s involvement in dilated cardiomyopathy (DCM). While prior research had implied a possible FBN1-DCM connection, our study represents the first dedicated analysis of FBN1’s impact on DCM susceptibility, clinical features, and prognosis in a sizable DCM cohort. We uncovered 18 rare deleterious FBN1 variants in 19 of 1,059 DCM patients and detailed the variants’ distribution and types. Notably, we observed variations in clinical characteristics between DCM patients with and without FBN1 variants. FBN1 variant carriers displayed higher rates of atrial fibrillation (42.1% vs. 22.0%, P = 0.037) and ICD implantation (10.5% vs. 1.6%,
Previous studies have identified causal or associated genes through rigorous cosegregation and linkage analyses in extensive DCM families[33,34]. Although these conventional methods are reliable, they are limited to identifying variants with a large effect size. For low-penetrance variants, perfect cosegregation with the phenotype is improbable[35]. Hence, we performed a gene-based association analysis on DCM cases and controls, offering robust statistical measures to evaluate the impact of rare variants[36].
Our study reveals an intriguing phenomenon: while some DCM patients with FBN1 rare deleterious variants showed MFS features, most did not present typical MFS phenotypes, such as severe aortic dilatation. Instead, their primary cardiovascular phenotype was left ventricular dilatation and systolic dysfunction, evidenced by reduced LVEF.
Our cohort included a DCM patient with an FBN1 frameshift variant who had significant aortic dilatation. Although her condition and the pathogenic FBN1 variant could suggest MFS, further follow-ups revealed no MFS family history or lens dislocation. However, the potential for her to develop MFS cannot be completely ruled out. On the contrary, most of our patients carrying FBN1 variants did not have pronounced aortic dilatation, indicating that while FBN1 variants in our study are associated with DCM, they may not lead to classic MFS phenotypes. This warrants further investigation into FBN1 genotype-phenotype correlations in FBN1-associated cardiovascular diseases.
Previous studies have reported the frequency of FBN1 variants in MFS to be between 50% and 90%[37,38]. In contrast, we found a lower detection rate of rare deleterious FBN1 variants in our DCM cohort (1.79%). Additionally, like in MFS where missense variants are most common[8], our DCM patients with FBN1 variants primarily had missense variants. These variations in variant prevalence underscore the complex role of FBN1 in cardiac pathophysiology and the need for disease-specific investigations.
With over 50 implicated genes, DCM’s genetic landscape is diverse[39]. Genes such as TTN and LMNA contribute significantly to DCM’s clinical features, causing around 25% and 5% of autosomal dominant DCM cases, respectively[2,40-42]. In our study, we found that 1.79% of DCM patients carried deleterious FBN1 variants, thus prompting further investigation into the FBN1-DCM connection.
Our findings suggest that rare deleterious FBN1 variants may predispose individuals to DCM, highlighting the possible importance of FBN1 in DCM’s genetic structure. This lays the groundwork for future research into FBN1’s role in DCM pathogenesis.
Study limitations
Our study has several limitations, such as potential false positives due to focusing solely on FBN1, difficulty in performing pathogenicity research due to the recruitment of sporadic DCM patients without a family history, and the relatively small sample size. The identification of rare deleterious missense variants was based on computational prediction tools, introducing a possible risk of false positives. Moreover, our study is a single-center investigation conducted exclusively in an East Asian population, possibly limiting its generalizability. FBN1 is at best expressed at low levels in the cardiac myocytes but at high levels in cardiac fibroblasts. Therefore, the deleterious effect of the rare variants in the FBN1 gene on cardiac function could be mediated through their effects on cardiac fibroblasts and the myocardial architecture.
Conclusion
We found enrichment of rare, deleterious variants in the FBN1 gene in patients with sporadic DCM. Our findings indicate that FBN1 variants may increase the risk of atrial fibrillation and secondary endpoints in DCM patients. This discovery could potentially improve DCM diagnostic and therapeutic strategies. However, given the complex nature of DCM and the various factors affecting its phenotype, our findings require further validation in larger, more diverse cohorts.
DECLARATIONS
AcknowledgmentsThe authors thank all study participants and clinicians for their vital contributions to this study. The Graphic Abstract figure was partly generated using Servier Medical Art, provided by Servier, licensed under a Creative Commons Attribution 3.0 unported license.
Author’s contributionsDesigned the study, drafted and revised the manuscript: Wu D
Contributed to analysis and interpretation of data: Sun Y, Li C, Xiao L, Dai J, Chen Y, Shi L
Contribute to the enrollment and follow-up of research subjects, sample and clinical baseline data collection: Chen P, Wang H, Yu B, Wei H, Li R, Song X, Yu T
Contributed to funding, conception, revision, and final approval of the manuscript: Wang DW
Financial support and sponsorshipThis publication was supported by grants from the National Nature Science Foundation of China (NOS. 81630010, 91839302 and 82100442), National Key R&D Program of China (No. 2017YFC0909400), and Shanghai Municipal Science and Technology Major Project (No. 2017SHZDZX01).
Conflict of interestAll authors declared that there are no conflicts of interest.
Ethical approval and consent to participateThis study was approved by the institutional review board of Tongji Hospital, Tongji Medical College, Huazhong University of Science and Technology (IRB TJ-C20181101), and was registered at ClinicalTrials.gov (ID: NCT03754101). All participants provided written informed consent.
Consent for publicationParticipants provided written informed consent.
Copyright© The Author(s) 2023.
Supplementary MaterialsREFERENCES
1. Japp AG, Gulati A, Cook SA, Cowie MR, Prasad SK. The diagnosis and evaluation of dilated cardiomyopathy. J Am Coll Cardiol 2016;67:2996-3010.
3. Rosenbaum AN, Agre KE, Pereira NL. Genetics of dilated cardiomyopathy: practical implications for heart failure management. Nat Rev Cardiol 2020;17:286-97.
4. Fatkin D, Huttner IG, Kovacic JC, Seidman JG, Seidman CE. Precision medicine in the management of dilated cardiomyopathy:
5. McNally EM, Mestroni L. Dilated cardiomyopathy: genetic determinants and mechanisms. Circ Res 2017;121:731-48.
6. Jordan E, Peterson L, Ai T, et al. Evidence-based assessment of genes in dilated cardiomyopathy. Circulation 2021;144:7-19.
7. Hershberger RE, Cowan J, Jordan E, Kinnamon DD. The complex and diverse genetic architecture of dilated cardiomyopathy. Circ Res 2021;128:1514-32.
8. Sakai LY, Keene DR, Renard M, De Backer J. FBN1: the disease-causing gene for Marfan syndrome and other genetic disorders. Gene 2016;591:279-91.
9. Loeys BL, Dietz HC, Braverman AC, et al. The revised Ghent nosology for the Marfan syndrome. J Med Genet 2010;47:476-85.
10. Silverman DI, Burton KJ, Gray J, et al. Life expectancy in the Marfan syndrome. Am J Cardiol 1995;75:157-60.
11. von Kodolitsch Y, De Backer J, Schüler H, et al. Perspectives on the revised Ghent criteria for the diagnosis of Marfan syndrome. Appl Clin Genet 2015;8:137-55.
12. Campens L, Renard M, Trachet B, et al. Intrinsic cardiomyopathy in Marfan syndrome: results from in-vivo and ex-vivo studies of the
13. Connor BS, Algaze CA, Narkevičiūtė A, et al. Prevalence and outcomes of primary left ventricular dysfunction in Marfan syndrome. Am J Cardiol 2022;175:119-26.
14. Pinto YM, Elliott PM, Arbustini E, et al. Proposal for a revised definition of dilated cardiomyopathy, hypokinetic non-dilated cardiomyopathy, and its implications for clinical practice: a position statement of the ESC working group on myocardial and pericardial diseases. Eur Heart J 2016;37:1850-8.
16. Li H, Durbin R. Fast and accurate long-read alignment with Burrows-Wheeler transform. Bioinformatics 2010;26:589-95.
17. Li H, Handsaker B, Wysoker A, et al. The sequence alignment/map format and SAMtools. Bioinformatics 2009;25:2078-9.
18. McKenna A, Hanna M, Banks E, et al. The genome analysis toolkit: a mapreduce framework for analyzing next-generation DNA sequencing data. Genome Res 2010;20:1297-303.
19. Danecek P, Auton A, Abecasis G, et al. The variant call format and VCFtools. Bioinformatics 2011;27:2156-8.
20. Wang K, Li M, Hakonarson H. ANNOVAR: functional annotation of genetic variants from high-throughput sequencing data. Nucleic Acids Res 2010;38:e164.
21. Richards S, Aziz N, Bale S, et al. Standards and guidelines for the interpretation of sequence variants: a joint consensus recommendation of the American College of Medical Genetics and Genomics and the Association for Molecular Pathology. Genet Med 2015;17:405-24.
22. Carter H, Douville C, Stenson PD, Cooper DN, Karchin R. Identifying Mendelian disease genes with the variant effect scoring tool. BMC Genomics 2013;14 Suppl 3:S3.
23. Ioannidis NM, Rothstein JH, Pejaver V, et al. REVEL: an ensemble method for predicting the pathogenicity of rare missense variants. Am J Hum Genet 2016;99:877-85.
24. Li J, Zhao T, Zhang Y, et al. Performance evaluation of pathogenicity-computation methods for missense variants. Nucleic Acids Res 2018;46:7793-804.
25. Schwarz JM, Cooper DN, Schuelke M, Seelow D. MutationTaster2: mutation prediction for the deep-sequencing age. Nat Methods 2014;11:361-2.
26. Rentzsch P, Witten D, Cooper GM, Shendure J, Kircher M. CADD: predicting the deleteriousness of variants throughout the human genome. Nucleic Acids Res 2019;47:D886-94.
27. Schafer S, de Marvao A, Adami E, et al. Titin-truncating variants affect heart function in disease cohorts and the general population. Nat Genet 2017;49:46-53.
28. Walsh R, Thomson KL, Ware JS, et al. Reassessment of mendelian gene pathogenicity using 7,855 cardiomyopathy cases and 60,706 reference samples. Genet Med 2017;19:192-203.
29. Schiabor Barrett KM, Cirulli ET, Bolze A, et al. Cardiomyopathy prevalence exceeds 30% in individuals with TTN variants and early atrial fibrillation. Genet Med 2023;25:100012.
30. Roberts AM, Ware JS, Herman DS, et al. Integrated allelic, transcriptional, and phenomic dissection of the cardiac effects of titin truncations in health and disease. Sci Transl Med 2015;7:270ra6.
31. Li Q, Wang K. InterVar: clinical interpretation of genetic variants by the 2015 ACMG-AMP guidelines. Am J Hum Genet 2017;100:267-80.
32. Lee S, Emond MJ, Bamshad MJ, et al. Optimal unified approach for rare-variant association testing with application to small-sample case-control whole-exome sequencing studies. Am J Hum Genet 2012;91:224-37.
33. Norton N, Li D, Rampersaud E, et al. Exome sequencing and genome-wide linkage analysis in 17 families illustrate the complex contribution of TTN truncating variants to dilated cardiomyopathy. Circ Cardiovasc Genet 2013;6:144-53.
34. Horvat C, Johnson R, Lam L, et al. A gene-centric strategy for identifying disease-causing rare variants in dilated cardiomyopathy. Genet Med 2019;21:133-43.
35. Cooper DN, Krawczak M, Polychronakos C, Tyler-Smith C, Kehrer-Sawatzki H. Where genotype is not predictive of phenotype: towards an understanding of the molecular basis of reduced penetrance in human inherited disease. Hum Genet 2013;132:1077-130.
36. Moutsianas L, Agarwala V, Fuchsberger C, et al. The power of gene-based rare variant methods to detect disease-associated variation and test hypotheses about complex disease. PLoS Genet 2015;11:e1005165.
37. Faivre L, Collod-Beroud G, Loeys BL, et al. Effect of mutation type and location on clinical outcome in 1,013 probands with Marfan syndrome or related phenotypes and FBN1 mutations: an international study. Am J Hum Genet 2007;81:454-66.
38. Turner CL, Emery H, Collins AL, et al. Detection of 53 FBN1 mutations (41 novel and 12 recurrent) and genotype-phenotype correlations in 113 unrelated probands referred with Marfan syndrome, or a related fibrillinopathy. Am J Med Genet A 2009;149A:161-70.
39. McNally EM, Golbus JR, Puckelwartz MJ. Genetic mutations and mechanisms in dilated cardiomyopathy. J Clin Invest 2013;123:19-26.
40. Fatkin D, MacRae C, Sasaki T, et al. Missense mutations in the rod domain of the lamin A/C gene as causes of dilated cardiomyopathy and conduction-system disease. N Engl J Med 1999;341:1715-24.
41. Gerull B, Gramlich M, Atherton J, et al. Mutations of TTN, encoding the giant muscle filament titin, cause familial dilated cardiomyopathy. Nat Genet 2002;30:201-4.
Cite This Article
How to Cite
Download Citation
Export Citation File:
Type of Import
Tips on Downloading Citation
Citation Manager File Format
Type of Import
Direct Import: When the Direct Import option is selected (the default state), a dialogue box will give you the option to Save or Open the downloaded citation data. Choosing Open will either launch your citation manager or give you a choice of applications with which to use the metadata. The Save option saves the file locally for later use.
Indirect Import: When the Indirect Import option is selected, the metadata is displayed and may be copied and pasted as needed.
About This Article
Copyright
Data & Comments
Data
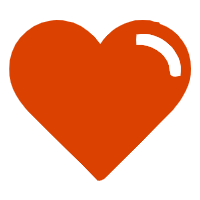
Comments
Comments must be written in English. Spam, offensive content, impersonation, and private information will not be permitted. If any comment is reported and identified as inappropriate content by OAE staff, the comment will be removed without notice. If you have any queries or need any help, please contact us at [email protected].