Report-out from an NSF Workshop on Advanced Manufacturing for Industrial Decarbonization
Abstract
Advanced manufacturing can largely contribute to industrial decarbonization through at least two strategies. First, traditional manufacturing technologies with low energy efficiency or high emissions can be replaced with advanced manufacturing technologies. Second, advanced manufacturing technologies can be developed to produce novel products that improve energy efficiency or reduce emissions. There are gaps in the literature regarding the state-of-the-art about these two strategies. To help realize the full potential of advanced manufacturing for industrial decarbonization, the NSF Workshop on Advanced Manufacturing for Industrial Decarbonization was recently held. The workshop provided an interaction venue for researchers from different research communities, including advanced manufacturing, energy efficiency, industrial decarbonization, and techno-economic analysis. This paper reports the findings from the workshop, including discussions on the four major pillars of industrial decarbonization (energy efficiency; industrial electrification; low-carbon fuels, feedstocks, and energy sources; and carbon capture, utilization, and storage), existing gaps and challenges in advanced manufacturing for industrial decarbonization, and recommendations regarding how to accelerate research and development on advanced manufacturing for industrial decarbonization. This paper will assist active researchers, scientists, engineers, project leaders, and program directors in identifying the current research focuses, challenges, and future trends in the field.
Keywords
INTRODUCTION
The 2024 United Nations Climate Change Conference, more commonly known as COP29, again emphasized the urgent need for climate actions[1]. According to a report recently published by the Intergovernmental Panel on Climate Change of the United Nations[2], limiting global warming to 1.5 and
(1) Energy efficiency
(2) Industrial electrification
(3) Low-carbon fuels, feedstocks, and energy sources (LCFFES)
(4) Carbon capture, utilization, and storage (CCUS)
Traditionally, researchers in the manufacturing field have mainly aimed to improve part quality, increase productivity, and reduce cost, with inadequate consideration of manufacturing’s carbon footprint. Manufacturing currently contributes to 83% of the energy-related CO2 emissions in the U.S. industrial sector and 25% of total CO2 emissions in the U.S. economy[3]. Mitigation of CO2 emissions from manufacturing is urgently needed. Furthermore, advanced manufacturing can be used to produce novel products that improve energy efficiency or reduce emissions during the usage of these products. Advanced manufacturing can also be used to produce emerging products that aim at mitigating emissions (e.g., sorbents for carbon capture and catalysts for carbon utilization).
To help realize the full potential of advanced manufacturing for industrial decarbonization, the NSF Workshop on Advanced Manufacturing for Industrial Decarbonization was held in August 2023, in Arlington, Virginia[4]. The objectives of the workshop included:
(1) To identify gaps and challenges of advanced manufacturing for industrial decarbonization, including but not limited to technological pathways and their techno-economic analysis (TEA)
(2) To formulate recommendations for research initiatives
The workshop provided an interaction venue for researchers from the communities of advanced manufacturing, energy efficiency, industrial electrification, LCFFES, CCUS, and TEA.
A steering committee of 21 members was formed to help recommend and invite speakers, review abstracts for the selection of idea presentations, moderate workshop sessions (invited talks and panel discussions), and provide input to this report.
A group of six organizers were responsible for communicating with all the stakeholders (i.e., steering committee members, invited speakers, idea presenters, and participants), coordinating the schedule, working out the logistics, and moderating workshop sessions (invited talks and panel discussions).
The workshop lasted for one and a half days. It included three types of activities:
(1) Invited talks. Sixteen invited speakers included experts from industry, national labs, and academia who presented inspiring examples of advanced manufacturing for industrial decarbonization, and representatives from federal agencies who talked about their agencies’ perspectives on advanced manufacturing for industrial decarbonization. These speakers were recommended or invited by workshop steering committee members.
(2) Panel discussions. A group of invited speakers served as panelists for these panel discussions.
(3) Idea presentations. Ten selected participants presented their ideas about advanced manufacturing for industrial decarbonization. These presenters were selected by the workshop steering committee based on their submitted abstracts.
Among the 21 steering committee members, six organizers, and 16 invited speakers, there were 12 women or minorities, as illustrated in Figure 1.
The workshop had more than 100 registered participants, representing academia, industry, national labs, and federal agencies.
This paper reports on the findings from the above-mentioned workshop, including discussions on the four major pillars of industrial decarbonization, existing gaps and challenges in advanced manufacturing for industrial decarbonization, and recommendations regarding how to accelerate research and development on advanced manufacturing for industrial decarbonization.
FOUR MAJOR PILLARS OF INDUSTRIAL DECARBONIZATION
Energy efficiency
Sachin Nimbalkar from Oak Ridge National Laboratory presented Energy Efficiency as a Foundational Technology Pillar for Industrial Decarbonization[5]. He highlighted the industry’s significant energy demand and CO2 emissions, accounting for 33% of the nation’s primary energy use and 30% of the nation’s energy-related CO2 emissions. In the path to net-zero CO2 emissions per DOE’s Industrial Decarbonization Roadmap[3], efficiency is expected to serve as the dominant pillar by 2040 and remain one of the major pillars by 2050, as shown in Figure 2[5].
Figure 2. Emissions reductions by different industrial decarbonization pillars and remaining emissions projected in different years[5].
The pathways in the energy efficiency pillar include:
• Strategic energy management
• System efficiency
• Smart manufacturing
• Materials and life cycle efficiency
• Combined heat and power
Specific strategies were provided according to the five pathways above for the six energy-intensive industries, including iron and steel, chemical, food and beverage, petroleum refining, pulp and paper, and cement. For example, smart manufacturing for the iron and steel industry can involve “shortened smelting time and enhanced smelting efficiency using automated detection of molten steel components, blowing controls, and component analysis” or rely on digital twins[6].
Nilanjan Sarangi from Saint-Gobain Abrasive discussed decarbonization from an abrasive manufacturer’s perspective[7]. A case study was presented on their bonded abrasive products. By adopting a net-shape processing method, the energy demand is significantly reduced.
Further research for the energy efficiency pillar is recommended by Nimbalkar in the following areas[5]:
• Improving the efficiency of process heating, steam, and motor systems
• Addressing big data challenges related to data quality, storage, and computing
• Advanced data analytics for processing the data and improving cybersecurity
• Data integration for facilitating utility efficiency programs that reward manufacturers for energy saved rather than equipment installed
• Demonstrations of plant automation systems that provide real-time energy performance data
Industrial electrification
Cecilia Springer from Global Efficiency Intelligence gave a presentation on Deep Decarbonization of Industry through Electrification of Process Heating[8]. She highlighted that process heating is the largest contributor to the U.S. manufacturing energy use. She analyzed the temperature levels of the process heat and revealed that two thirds of process heat used in the U.S. industry is below 300 °C[9].
Furthermore, Springer presented a case study on the electrification of the beer production industry[8]. This study is based on a state-level analysis because different states have different electricity grid emissions factors and industrial energy prices. By replacing a centralized gas boiler system with heat pumps, all process steps of beer production (including mashing, boiling, pasteurization, cleaning, and production support) are expected to save a significant amount of energy. The total energy saved is as large as 69%. CO2 emissions reductions are also significant, as shown in Figure 3[8]. For example, the beer industry in California is expected to reduce its CO2 emissions by more than 100 kt per year in 2030. As the grid electricity becomes cleaner, the CO2 emissions reductions will be more significant, reaching more than 140 and 160 kt per year in 2040 and 2050, respectively. Other states that expect significant CO2 emissions reductions by replacing a centralized gas boiler system with heat pumps in beer factories include Colorado, Texas, Ohio, Georgia, Wisconsin, Pennsylvania, Florida, and North Carolina. According to the study, “CO2 emissions reductions can be achieved even today using grid electricity in most states studied”. In addition, the energy cost was compared between the conventional and electrified processes. The difference is not large. Some states (such as Pennsylvania, Ohio, and Washington) are projected to lower their energy cost while others are to increase it. However, Springer pointed out that “energy cost is only a small portion of total manufacturing cost for many industrial subsectors” and “a moderate increase in energy cost per unit of product resulting from electrification will have a minimal impact on the price of final product and final consumers”.
Figure 3. Projected CO2 emissions reductions in the beer industry across different states in different years[8].
The key takeaways from Springer’s talk include[8]:
• There is significant potential to decarbonize the U.S. industry with electrification.
• Using grid electricity, CO2 emissions reductions from electrification can be achieved in some states with clean electricity supply today and in many more states in 2030.
• Energy cost per unit of production for electrified processes is higher but can be competitive with conventional processes if low-price renewable electricity is available.
Lastly, Springer had the following recommendations on industrial electrification[8]:
• Identify the sweet spots and start there. Start in states with more favorable conditions (e.g., cleaner grid, lower price ratio of electricity to natural gas, more favorable investment conditions, and local incentives, etc.).
• The industry sector should initiate partnerships with government, academia, think tanks, and other stakeholders to develop and/or scale electrification technologies.
• Six impactful actions that would support increased industrial electrification in the U.S. are:
○ Support demonstration of emerging electrification technologies and new applications of existing technologies
○ Financially incentivize electrification
○ Increase renewable electricity generation capacity
○ Enhance the electricity grid
○ Engage communities
○ Develop the workforce
Low-carbon fuels, feedstocks, and energy sources
Eric C.D. Tan from the National Renewable Energy Laboratory gave a presentation on Energy and Techno-Economic Analysis of Bio-based and Low-carbon Chemicals and Fuels Production Processes[10]. He highlighted two case studies. The first one was on 2,3-butanediol (BDO) separation. BDO is “a biomass-derived intermediate for producing sustainable aviation fuel for commercial aviation decarbonization”[11]. It is produced by the fermentation of sugars. The raw broth includes 10 wt.% BDO, 86 wt.% water, and 4 wt.% byproducts. The challenges in BDO separation include low BDO concentration and water being more volatile than BDO. Tan presented analysis results of seven BDO separation processes. The energy consumption and costs of different BDO separation processes vary across one order of magnitude. The second case study was on methanol production[12]. Methanol can serve as an alternative transportation fuel, power generation fuel, and chemical intermediate. There are many methanol production pathways. For example, it can be produced by gasification of mixed plastic waste. It can also be produced by reactive CO2 capture and conversion. Tan presented analysis results of 12 methanol production pathways. The carbon intensities vary significantly and are dictated by the pathways. These case studies showed the importance of combined TEA and life cycle assessment (LCA) for emerging technologies[10].
Don Stevenson from GTI Energy gave a talk on gaseous fuels produced from biogenic feedstocks[13]. He first presented a comprehensive U.S. energy system modeling effort conducted in collaboration with EPRI[14]. The objective was to evaluate the least-cost pathways to achieve net-zero carbon emissions across the entire U.S. economy by 2050. The modeling shows that, although the share of electricity grows considerably under every scenario, ~50% of all energy is supplied to end-use markets as a fuel, as shown in Figure 4[13]. Stevenson then highlighted the need for renewable propane, one of the main components of liquefied petroleum gas (LPG). LPG is versatile, being used for industrial, agricultural, residential, transportation, and recreational purposes. However, the availability of LPG is expected to decline with the shrinking fossil fuel industry because LPG is a fossil fuel byproduct. Stevenson shared the related R&D activities at GTI Energy. Furthermore, he shared advanced syngas manufacturing possibilities. Finally, he concluded that, among many pathways to sustainable products and biofuels, the most scalable route is from cellulosic feedstocks to syngas through processes such as pyrolysis and gasification.
Figure 4. Projected least-cost pathways to achieve net-zero carbon emissions across the entire U.S. economy by 2050[13].
Jonathan Scheffe from the University of Florida gave a presentation on Strategies for Harnessing Solar Thermal Energy for Industrial Decarbonization[15]. He first emphasized that cheap energy storage is key to the renewable energy transition. The variable solar generation within a day can be smoothed by short-term energy storage, for example, Li-ion batteries. However, seasonally variable solar generation requires long-term energy storage. He then pointed out that concentrating solar power could be a promising solution. First, it offers cheap thermal energy storage for 24/7 utilization. Second, the high-temperature process heat (up to 1,500 °C) can be used for manufacturing (e.g., cement or steel). Third, it allows for the production of energy-dense sustainable fuels, satisfying the transportation sector and enabling seasonal energy storage. Scheffe then introduced a few thermal routes to solar fuel production, including fuel reforming, thermolysis, and redox cycles[16,17]. Finally, he proposed some research opportunities where advanced manufacturing can help enable the higher temperatures required by solar fuel production. For example, novel design and manufacturing of heliostats is needed to reduce their cost. Furthermore, additive manufacturing (AM) may help improve the reactive structures that enable efficient radiative heat transfer.
Carbon capture, utilization, and storage
Volker Sick from the University of Michigan gave a talk on Market and Climate Opportunities for CO2 Utilization[18]. He emphasized the importance of CO2 source and sink combinations of different CCUS technologies. The CO2 sources considered include fossil fuel, fossil carbon (limestone), biomass, and ambient (air and water). The CO2 sinks considered include processes such as enhanced oil recovery and geological sequestration, and products such as construction materials, chemicals, fuels, and food. Construction materials are considered as Track 1 products that allow for removal/storage of carbon for > 100 years. Fuels and food are considered as Track 2 products that decompose to CO2 in < 100 years. Chemicals can be either Track 1 or 2 products. The impact of different combinations of these CO2 sources and sinks is illustrated in Figure 5[18]. The conversion from ambient CO2 to Track 1 products is identified as the sweet spot with negative emissions and economic return. Sick also presented the potential climate and economic significance of different product types in 2050, as shown in Figure 6. Construction materials, fuels, and chemicals are projected to be the largest contributors to annual CO2 consumption, at 1.0-9.5, 0.28-10.80, and 0.26-0.58 gigatonnes, respectively. Their market sizes are estimated to be 800-1,000, 21-2,060, and 100-180 billion dollars, respectively.
Figure 5. Impact of different combinations of CO2 sources and sinks (i.e., process and product types)[18].
Figure 6. Potential climate and economic significance of different product types in 2050[18].
John Shingledecker from EPRI gave a presentation on Opportunities for Advanced Manufacturing and Materials in Improving Carbon Capture Technology[19]. He first presented the cost breakdown for a carbon capture, transport, and storage system for a prototypical natural gas combined cycle power plant. It turned out that carbon capture contributes to more than 70% of the overall cost. Therefore, it is critical to lower the carbon capture cost. He then identified key potential areas for cost reductions, including sorbents and heat exchangers. Afterward, he shared several R&D activities by different organizations. For example, complex structures produced by AM could improve heat and mass transfer. These structures can be used for sorbents or heat exchangers. It is also possible to use advanced manufacturing to integrate heat and mass exchange to improve the efficiency and reduce the size of absorber vessels. Finally, he concluded that applying AM to CCUS systems “is in its infancy with only a limited number of studies being conducted”, suggesting that more research is needed in multiple areas.
Doug Robinson from Solidia Technologies talked about Carbon Utilization in Concrete Manufacturing[20]. He first introduced their cement. Because of a different chemical reaction (illustrated in Figure 7), the production of their cement generates 30% less CO2 than ordinary Portland cement (OPC). In addition, their cement reacts with CO2 instead of H2O during concrete forming, resulting in additional CO2 reductions. Additionally, Robinson introduced their supplementary cementitious materials (SCM). Their SCM can be used to replace OPC, leading to CO2 reductions. For example, a 35% replacement leads to a 23% CO2 reduction. Furthermore, Robinson presented that the projected CO2 reduction through Solidia technologies could reach nearly 1 gigatonnes per year assuming a full-market conversion, including 0.17, 0.17, and 0.6 gigatonnes by their cement, concrete, and SCM, respectively. Finally, Robinson concluded his talk by providing the techno-economic perspectives of the technologies discussed above. Their cement is market-proven to be profitable, and their SCM is expected to be profitable. In addition, governmental incentives (e.g., tax credits) can make the adoption of their technologies more economical.
Figure 7. Comparison between OPC and Solidia cement in terms of their chemistry[20]. OPC: Ordinary Portland cement.
Brad Brennan from Dimensional Energy gave a presentation on Converting CO2 and Water into Hydrocarbons[21]. He first introduced their process that consists of three steps: (1) electrolysis to convert
GAPS, CHALLENGES, AND RECOMMENDATIONS
One large gap identified during the workshop is the lack of communications and interactions between the advanced manufacturing and industrial decarbonization research communities. Most of the talks were focused on industrial decarbonization. A large majority of the workshop participants were from the advanced manufacturing research community. While the workshop was an excellent learning experience for advanced manufacturing researchers, the gap cannot be filled with such a single event. These two research communities need to communicate and interact more frequently in order to identify synergy and conduct convergent research.
One significant challenge in industrial decarbonization is the lack of TEA and LCA of emerging technologies. Researchers tend to focus on the technical performance of their technologies while omitting TEA and LCA. However, TEA and LCA determine if the technologies will survive the market and create positive environmental impacts from a holistic view. More research on TEA and LCA is urgently needed before governments and private investors can make informed decisions on what technologies to invest in. This challenge is confirmed in one of the latest reports by National Academies of Sciences, Engineering, and Medicine[22].
Given the above-mentioned gaps and challenges, a significant amount of future work is needed to continue and augment the momentum generated from this initiative. The following actions are recommended:
• To organize more events (workshops, special sessions, symposia, and conferences) to enable more frequent communications and interactions between the advanced manufacturing community and industrial decarbonization research community
• To organize separate events for each industrial decarbonization pillar to allow for more in-depth discussions on which fundamental research areas funding agencies should direct their investments to help grow this field (advanced manufacturing for industrial decarbonization)
• To organize more events for fundamental and applied research focused on TEA and LCA of emerging industrial decarbonization technologies
• To create funding opportunities that support research on advanced manufacturing for industrial decarbonization, including its TEA and LCA
• To develop educational programs that produce workforces equipped with both advanced manufacturing and industrial decarbonization knowledge and skills
• To form a national coalition at the intersection of advanced manufacturing and industrial decarbonization, connecting people from industry, national labs, and academia
• To establish an innovation and commercialization ecosystem that supports and incentivizes new and existing companies to adopt emerging technologies
DECLARATIONS
Acknowledgments
The authors acknowledge the organizers and steering committee members for organizing the workshop. They also acknowledge the speakers for sharing their valuable insights.
Authors’ contributions
Organized the workshop: Ma, C.; Pei, Z.
Wrote the article: Ma, C.; Pei, Z.; Zhang, H. C.
Availability of data and materials
Not applicable.
Financial support and sponsorship
This material is based upon work supported by the National Science Foundation under Grant No. 2305157. The workshop was also financially supported by SME, Texas A&M Engineering Experiment Station, and Texas A&M Energy Institute.
Conflicts of interest
Zhang, H. C. is Editor in Chief and Pei, Z. is Editorial Board Member of the journal Green Manufacturing Open. Zhang, H. C. and Pei, Z. were not involved in any steps of editorial processing, notably including reviewer selection, manuscript handling and decision-making. The other author declares that there are no conflicts of interest.
Ethical approval and consent to participate
Not applicable.
Consent for publication
Not applicable.
Copyright
© The Author(s) 2025.
REFERENCES
1. UN Climate Change Conference Baku - November 2024. https://unfccc.int/cop29. (accessed 2025-04-17)
2. The United Nations (U.N.) Intergovernmental Panel on Climate Change (IPCC). AR6 synthesis report: climate change 2023. https://www.ipcc.ch/report/ar6/syr/. (accessed 2025-04-17)
3. The U.S. DOE Office of Energy Efficiency and Renewable Energy (EERE). DOE industrial decarbonization roadmap, No. DOE/EE-2635. 2022. https://www.energy.gov/sites/default/files/2022-09/Industrial%20Decarbonization%20Roadmap.pdf. (accessed 2025-04-17).
4. NSF Workshop on Advanced Manufacturing for Industrial Decarbonization. https://na.eventscloud.com/website/52669/home/. (accessed 2025-04-17).
5. Nimbalkar, S. Energy efficiency as a foundational technology pillar for industrial decarbonization. Presentation at the NSF Workshop on Advanced Manufacturing for Industrial Decarbonization, August 3-4, 2023, Arlington, VA. https://na.eventscloud.com/website/52669/agenda/. (accessed 2025-04-17).
6. Price, C. R.; Nimbalkar, S. U.; Thirumaran, K.; Cresko, J. Smart manufacturing pathways for industrial decarbonization and thermal process intensification. Smart. Sustain. Manuf. Syst. 2023, 7, 41-53.
7. Sarangi, N. Decarbonization - an abrasive manufacturer’s perspective. Presentation at the NSF Workshop on Advanced Manufacturing for Industrial Decarbonization, August 3-4, 2023, Arlington, VA. https://na.eventscloud.com/website/52669/agenda/. (accessed 2025-04-17).
8. Springer, C. Deep decarbonization of industry through electrification of process heating. Presentation at the NSF Workshop on Advanced Manufacturing for Industrial Decarbonization, August 3-4, 2023, Arlington, VA. https://na.eventscloud.com/website/52669/agenda/. (accessed 2025-04-17)
9. Hasanbeigi, A. Industrial Electrification in U.S. States. https://www.globalefficiencyintel.com/industrial-electrification-in-us-states. (accessed 2025-04-17).
10. Tan, E. C. D. Energy and techno-economic analysis of bio-based and low-carbon chemicals and fuels production processes. Presentation at the NSF Workshop on Advanced Manufacturing for Industrial Decarbonization, August 3-4, 2023, Arlington, VA. https://na.eventscloud.com/website/52669/agenda/. (accessed 2025-04-17)
11. Kubic, W. L. J.; Tan, E. C. D. Reactive extraction process for separating 2, 3-butanediol from fermentation broth. Ind. Eng. Chem. Res. 2023, 62, 5241-51.
12. Martin, J. A.; Tan, E. C. D.; Ruddy, D. A.; King, J.; To, A. T. Temperature–pressure swing process for reactive carbon capture and conversion to methanol: techno-economic analysis and life cycle assessment. Environ. Sci. Technol. 2024, 58, 13737-47.
13. Stevenson, D. Potential contributions of advanced manufacturing toward industrial decarbonization. Presentation at the NSF Workshop on Advanced Manufacturing for Industrial Decarbonization, August 3-4, 2023, Arlington, VA. https://na.eventscloud.com/website/52669/agenda/. (accessed 17 Apr 2025).
14. Net-Zero 2050: U.S. Economy-Wide Deep Decarbonization Scenario Analysis. https://lcri-netzero.epri.com/. (accessed 17 Apr 2025).
15. Scheffe, J. Strategies for harnessing solar thermal energy for industrial decarbonization. Presentation at the NSF Workshop on Advanced Manufacturing for Industrial Decarbonization, August 3-4, 2023, Arlington, VA. https://na.eventscloud.com/website/52669/agenda/. (accessed 17 Apr 2025).
16. Scheffe, J. R.; Steinfeld, A. Oxygen exchange materials for solar thermochemical splitting of H2O and CO2: a review. Mater. Today. 2014, 17, 341-8.
17. Carrillo, R. J.; Scheffe, J. R. Advances and trends in redox materials for solar thermochemical fuel production. Sol. Energy. 2017, 156, 3-20.
18. Sick, V. Market and climate opportunities for CO2 utilization. Presentation at the NSF Workshop on Advanced Manufacturing for Industrial Decarbonization, August 3-4, 2023, Arlington, VA. https://na.eventscloud.com/website/52669/agenda/. (accessed 17 Apr 2025).
19. Shingledecker, J. Opportunities for advanced manufacturing and materials in improving carbon capture technology. Presentation at the NSF Workshop on Advanced Manufacturing for Industrial Decarbonization, August 3-4, 2023, Arlington, VA. https://na.eventscloud.com/website/52669/agenda/. (accessed 17 Apr 2025).
20. Robinson, D. Carbon utilization in concrete manufacturing. Presentation at the NSF Workshop on Advanced Manufacturing for Industrial Decarbonization, August 3-4, 2023, Arlington, VA. https://na.eventscloud.com/website/52669/agenda/. (accessed 17 Apr 2025).
21. Brennan, B. Converting CO2 and water into hydrocarbons. Presentation at the NSF Workshop on Advanced Manufacturing for Industrial Decarbonization, August 3-4, 2023, Arlington, VA. https://na.eventscloud.com/website/52669/agenda/. (accessed 17 Apr 2025).
Cite This Article
How to Cite
Download Citation
Export Citation File:
Type of Import
Tips on Downloading Citation
Citation Manager File Format
Type of Import
Direct Import: When the Direct Import option is selected (the default state), a dialogue box will give you the option to Save or Open the downloaded citation data. Choosing Open will either launch your citation manager or give you a choice of applications with which to use the metadata. The Save option saves the file locally for later use.
Indirect Import: When the Indirect Import option is selected, the metadata is displayed and may be copied and pasted as needed.
About This Article
Copyright
Data & Comments
Data
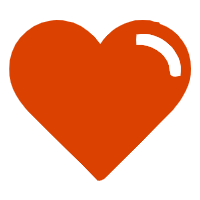
Comments
Comments must be written in English. Spam, offensive content, impersonation, and private information will not be permitted. If any comment is reported and identified as inappropriate content by OAE staff, the comment will be removed without notice. If you have any queries or need any help, please contact us at [email protected].